DOI:
10.1039/C9FO01519A
(Paper)
Food Funct., 2020,
11, 1063-1073
Dietary resveratrol attenuated colitis and modulated gut microbiota in dextran sulfate sodium-treated mice
Received
12th July 2019
, Accepted 4th November 2019
First published on 11th December 2019
Abstract
Accumulating evidence suggests that the gut microbiota plays an important role in the pathogenesis of colitis and that its composition could be modulated by exposure to dietary components. Thus, it may be possible to ameliorate the severity of colitis through administration of dietary components. Herein, we determined the effects of orally administered resveratrol on the gut microbiota composition and the resulting inflammatory status of a dextran sodium sulfate (DSS)-induced colitis mouse model. Our results supported our hypothesis that dietary resveratrol altered the microbial composition and restored microbial community diversity in DSS-treated mice. Specifically, resveratrol effectively decreased the abundance of the genera Akkermansia, Dorea, Sutterella and Bilophila, and increased the proportion of Bifidobacterium in colitic mice. Resveratrol was also able to prevent mouse body weight loss, reduce the disease activity index, attenuate tissue damage, and down-regulate the expression of pro-inflammatory cytokines such as IL-2, IFN-γ, GM-CSF, IL-1β, IL-6, KC/GRO, and TNF-α in the colon of DSS-treated mice. Pearson's correlation analysis indicated significant correlations between the relative levels of these pro-inflammatory cytokines and alterations of the gut microbiota. Our results demonstrated that dietary resveratrol attenuated the inflammatory status and alleviated gut microbiota dysbiosis in a colitis mouse model.
Introduction
Inflammatory bowel disease (IBD), encompassing relapsing–remitting Crohn's disease and ulcerative colitis, is a chronic inflammatory disease of the gastrointestinal tract.1 Although the precise etiology of IBD remains unknown, the most accepted hypothesis to date is that genetically predisposed hosts experience continual immune and inflammatory responses to their gut microbiota or ingested matieral.1,2 The possible role of the gut bacterial community in the pathogenesis of IBD has been highlighted only recently. Existing lines of evidence for such a relationship include: noted microbial markers for the progression of IBD such as reduced community diversity and altered bacterial composition when compared to healthy subjects,3–5 germ-free dextran sodium sulfate (DSS)-treated mice exhibiting resistance against colitis development compared to their infected counterparts,6 and IL-10-deficient mice (a murine model of IBD) not developing colitis under germ-free conditions.7 These results suggest that microbial factors might directly contribute to the development of colonic inflammation and adverse metabolic consequences. Therefore, the gut microbiota has become an important target in the treatment of IBD, and therapy has focused on correcting intestinal microbial imbalances and dysbiosis.
As the sole source of energy input for bacteria residing in the gastrointestinal tract, an individual's diet has a large influence on the gut microbiota composition. Food components (e.g. fiber, polysaccharides, and polyphenols) that are natively non-digestible are subjected to intestinal microbial metabolism.8,9 Previous studies have estimated that 90–95% of ingested polyphenols may accumulate in the large intestine where they interact with the gut microbial community.10 Resveratrol (3,5,4′-trihydroxy-trans-stilbene), a polyphenol found in various berries and peanuts, is highly accumulated in the gastrointestinal tract (65.1%).11 Studies have reported that dietary resveratrol alleviates gut dysbiosis caused by a high-fat diet, namely by increasing the Firmicutes/Bacteroidetes ratio, inhibiting the growth of Enterococcus faecalis, and increasing the prevalence of probiotics Lactobacillus and Bifidobacterium in the gut.12,13
The protective effects of resveratrol against acute or chronic colitis in different models were demonstrated in previous studies.14–17 In several instances, dietary resveratrol down-regulated inflammatory biomarkers, reduced oxidative stress, and attenuated clinical symptoms in experimental murine colitis models.14,16,18 A human study revealed that six weeks of dietary resveratrol could alleviate clinical colitis.19 A separate study reported increases in Lactobacillus and Bifidobacterium by resveratrol treatment and a moderating effect on the growth of Enterobacteria in an acute colitis rat model.17 Recently, Alrafas et al. reported that resveratrol modulated the gut microbiota to prevent colitis in an acute 2,4,6-trinitrobenzenesulfonic acid-induced colitis mouse model.20 In this study, 16S rRNA sequencing was used to identify gut microbiota community shifts by resveratrol treatment in DSS-induced chronic colitis mice. Our study provides insights into the effects of resveratrol on the gut microbiota and their interaction to affect chronic colitis.
Materials and methods
Animals and experimental design
This study was performed in strict accordance with the NIH guidelines for the care and use of laboratory animals and was approved by the Institutional Animal Care and Use Committee of the University of Massachusetts Amherst. 40 male CD-1 mice (6–8 weeks old) were purchased from Charles River Laboratories (Wilmington, MA, USA). Animals were randomly assigned to four groups and housed at 3–4 mice per cage in a temperature-controlled environment (22 ± 2 °C) with 65% relative humidity and a fixed 12 h light/dark cycle. For the first week of standard diet feeding, cage rotation was performed to minimize individual variation. After one-week diet acclimation, the four groups, each with n = 10, were designated as follows: the control group (DSS−/RES−) fed with standard chow AIN93G diet (Dyets Inc., Bethlehem, PA, USA); the resveratrol group (DSS−/RES+) fed with standard diet supplemented with 0.025% resveratrol (w/w, >99% purity) (Quality Phytochemicals, East Brunswick, NJ, USA); the DSS group (DSS+/RES−) fed with standard diet and 1.5% DSS water (w/v, salt form) (International Lab, Chicago, IL, USA); and the DSS-resveratrol group (DSS+/RES+) fed with 0.025% resveratrol (w/w) supplemented diet and 1.5% DSS drinking water (w/v). DSS was added to sterilized drinking water and administered ad libitum for 4 days, followed by 7 days of pure water for recovery. This cycle was repeated four times. Food and water consumption per cage were recorded and averaged to each mouse throughout the entire experimental period. At the end of the fourth cycle of DSS treatment, all mice were sacrificed by CO2 asphyxiation. The spleen was quickly excised and weighed. The colon from each mouse was dissected, measured in length, and cut open longitudinally. Fecal samples were collected from the colon immediately, snap-frozen, and then stored at −80 °C for later analysis.
DAI and histological assessment
Disease activity index (DAI) was scored based on the extent of rectal bleeding: 0 (none), 1 (hemoccult positive), 2 (blood), and 3 (gross bleeding); stool consistency: 0 (normal), 1 (soft but formed), 2 (loose and formless), and 3 (diarrhea); and weight loss: 0 (0%), 1 (1–5%), 2 (5–10%), 3 (10–20%), and 4 (>20%).21 The final macroscopic score for each animal is the sum of these three separate scores.
Formalin-fixed colon tissue was processed for paraffin embedding, with a section thickness of 5 μm, and haematoxylin and eosin (H&E) staining as described previously.22–24 Three parameters were graded: surface epithelial loss, crypt destruction, and inflammatory cell infiltration into the mucosa based on the established criteria.25 A score of 0–4 was assigned to each parameter (maximum macroscopic score = 12).
Enzyme-linked immunosorbent assay (ELISA)
30 mg of colonic mucosa was scraped and homogenized in 200 μL of Tris lysis buffer (Meso Scale Discovery, Rockville, MD, USA) containing 1% protease inhibitor cocktail (Boston Bioproducts, Ashland, MA, USA). The supernatant was collected by centrifugation at 16
000g for 20 m at 4 °C, and then loaded in sandwich ELISA kits (Meso Scale Discovery, Rockville, MD, USA) to determine the concentrations of cytokines: granulocyte-macrophage colony-stimulating factor (GM-CSF), interferon gamma (IFN-γ), interleukin 10 (IL-10), interleukin 2 (IL-2), interleukin 1 beta (IL-1β), interleukin 6 (IL-6), keratinocyte chemoattractant/human growth-regulated oncogene (KC/GRO), and tumor necrosis factor alpha (TNF-α), according to the manufacturer's instructions.
Fecal DNA extraction, 16S rRNA analysis and Illumina Mi-Seq sequencing
Fecal bacterial DNA was extracted using the PowerFecal DNA isolation kit (MoBio Laboratories, Inc., Carlsbad, CA, USA) following the supplier's protocol. The DNA was quantified and quality checked using a NanoDrop spectrophotometer (Thermo Scientific, Waltham, MA, USA) and the samples were normalized to 20 ng μL−1. The V3–V4 regions of the 16S rRNA gene was targeted for amplification by PCR using forward primer 5′TCGTCGGCAGCGTCAGATGTGTATAAGAGACAGCCTACGGGNGGCWGCAG and reverse primer 5′GTCTCGTGGGCTCGGAGATGTGTATAAGAGACAGGACTACHVGGGTATCTAATCC as described by Klindworth et al.26 Successful amplification was confirmed by gel electrophoresis. PCR products were then purified with AMPure XP beads (Beckman Coulter, Danvers, MA, USA). Dual indices and Illumina sequencing adapters were attached by PCR using the Nextera XT Index Kit (Illumina, San Diego, CA, US). The quantity and size of the PCR products were determined and verified using the Qubit dsDNA BR Assay kit (Life Technologies Corporation, Carlsbad, CA, USA) and ScreenTape Assay on Tape Station 2200 (Agilent Technologies, Santa Clara, CA, USA). Final PCR products were pooled in equimolar volumes and diluted to 4 nM. After combining the amplicon library and PhiX control, the samples were loaded onto the 600-cycle MiSeq Reagent kit v3 cartridge and sequenced using the Illumina MiSeq platform (Illumina Inc, San Diego, CA, USA).
Statistical analysis
Automatically demultiplexed Illumina sequence reads were processed using Quantitative Insights Into Microbial Ecology (QIIME) software pipeline v1.9.1.27 QIIME-generated operational taxonomic units (OTUs) clustered at ≥97% sequence similarity were further subjected to alpha- and beta-diversity analyses and principal coordinate analysis (PCoA). Cladogram construction and linear discriminant analysis (LDA) were performed using the LEfSe tool from the Huttenhower Lab.28 Correlation analyses were performed with SPSS 17.0 software (Chicago, IL, USA). Data were presented as mean ± standard deviation (SD). The statistical significance of differences among groups was determined using one-way ANOVA followed by Tukey's post hoc test or the one-way nonparametric ANOVA Kruskal–Wallis test.
Results and discussion
Dietary resveratrol attenuated colitis symptoms
There were no significant differences in drink and food consumption among cages and groups. The resveratrol dose applied is equivalent to ca. 2.3 mg kg−1 day−1 in humans, which is achievable through dietary supplementation. Administration of DSS water in four cycles successfully induced chronic colitis and the development of colorectal dysplasia in mice.29 Body weight was monitored at the end of every DSS and recovery cycle as an indicator of colitis severity. As the DSS-treatment cycle number increased, body weight increased correspondingly but to a lesser extent in DSS+/RES− mice compared to the control group, with the difference reaching statistical significance at and following the fourth DSS cycle (P < 0.05). Dietary resveratrol prevented this trend in DSS+/RES+ mice (Fig. 1A). DSS+/RES+ mice experienced average final body weight gain from 36.92 ± 3.05 g to 44.78 ± 1.61 g (P < 0.01) (Fig. 1B).
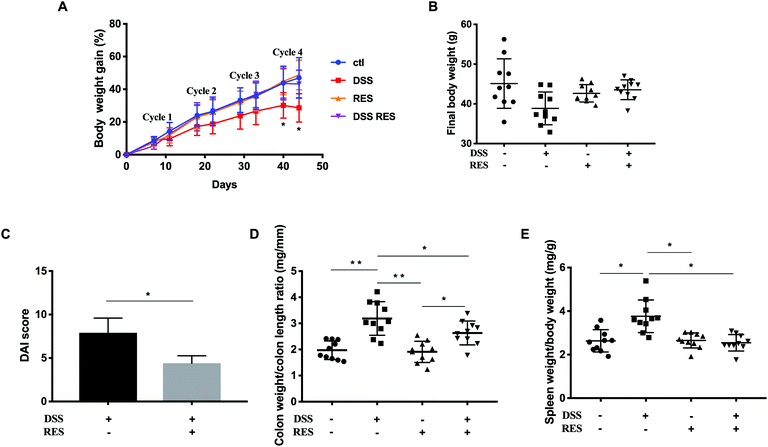 |
| Fig. 1 Dietary resveratrol attenuated colitis symptoms. (A) Percentage of body weight gain during the entire experiment; (B) final body weight; (C) disease activity index (DAI); (D) colon weight to colon length ratio; and (E) spleen weight to body weight ratio. Data are presented as mean ± standard deviation (SD) (n = 10). Significant differences are indicated: *P < 0.05 and **P < 0.01. | |
The DAI score is commonly used to evaluate the inflammatory severity in mice with colitis, which encompasses fecal consistency, hematochezia, and body weight loss.21 The DAI score rated as high as 7.9 ± 1.9 in the DSS+/RES− group, whereas it was markedly attenuated 1.8-fold with resveratrol treatment (P < 0.05) (Fig. 1C). Colon weight/length ratio is another metric correlated with the progression of colorectal inflammation. As shown in Fig. 1D, the difference between DSS+/RES− and DSS−/RES− colon weight/length ratio was highly significant (P < 0.05) while the administration of resveratrol nullified this effect in DSS-treated mice. Furthermore, remarkable spleen enlargement was observed in DSS-treated mice compared to control mice. The consumption of dietary resveratrol was demonstrated to restrict the spleen to final body weight ratio compared to that of the control mice (P < 0.05) (Fig. 1E). These results are in agreement with previous research,14,15,17,18 which demonstrated that dietary resveratrol prevented colitis progression indicators.
Dietary resveratrol ameliorated colonic tissue damage
H&E staining of the colonic segments from DSS+/RES− mice showed severe inflammatory damage, characterized by significant distortion of the crypt structure, edema of the submucosa, and instances of immune cell dysplasia (Fig. 2A). Resveratrol supplementation significantly mitigated the morphological alterations observed in the DSS+/RES− group, protecting colonic tissue integrity. The majority of colonic epithelium and mucosal architecture remained intact (Fig. 2A), which was consistent with previous studies.12,14 Damage of colonic mucosa shown by the overall histological score was 1.7-fold higher (P < 0.01) in the DSS+/RES− group compared to that in the DSS+/RES+ group (Fig. 2B).
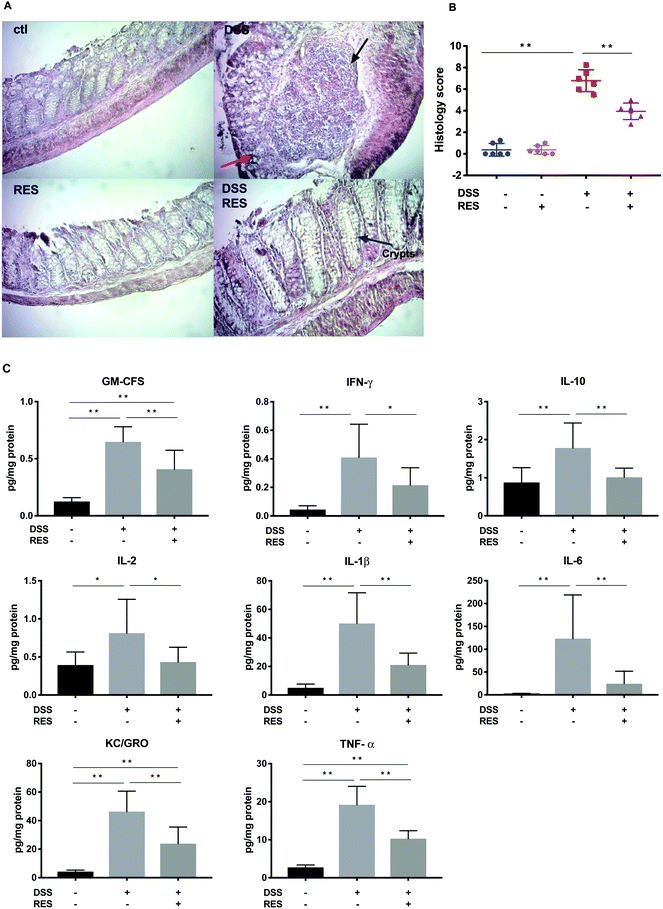 |
| Fig. 2 Dietary resveratrol improved colonic tissue damage and suppressed the overexpression of inflammatory cytokines. (A) Representative colon tissue images of H&E-stained colorectum sections (300× magnification). Crypt destruction associated with epithelial damage (red arrow) and inflammatory cell infiltrations (black arrow) were evident in the DSS group; (B) histological score of colonic abnormality (n = 6); and (C) quantification of inflammatory cytokines (GM-CSF, IFN-γ, IL-10, IL-2, IL-1β, IL-6, KC/GRO, and TNF-α) in the colonic mucosa (n = 8). Data are presented as mean ± SD. Significant differences are indicated as: *P < 0.05 and **P < 0.01. | |
Dietary resveratrol mediated increases of colonic pro-inflammatory cytokines caused by DSS treatment
Cytokines play a crucial role in driving and mediating intestinal inflammation. The severity of IBD is associated with the up-regulation of pro-inflammatory cytokines and down-regulation of anti-inflammatory cytokines. Hence, restoring the balance of these cytokines is a potential mode to alleviate IBD.30 The expression levels of inflammatory cytokines GM-CSF, IFN-γ, IL-10, IL-2, IL-1β, IL-6, KC/GRO, and TNF-α in colon mucosa increased by 5.2, 8.7, 2.0, 2.1, 10.0, 47.9, 10.9, and 7.0-fold, respectively, in DSS+/RES− mice (P < 0.05) compared to the control group, while dietary resveratrol in the DSS+/RES+ group markedly reduced their maximum expression by 44.2%, 48.5%, 43.2%, 46.8%, 58.0%, 79.9%, 48.5%, and 46.5%, respectively (P < 0.05) (Fig. 2C). These results suggested that the anti-inflammatory effect of resveratrol was at least partially due to improvements in immune homeostasis.
Although the significant mitigation of TNF-α, IFN-γ, IL-1β and IL-6 overexpression by resveratrol in DSS-treated mice (Fig. 2C) is in line with several previous studies,16,18,31 the modulating effects of dietary resveratrol on colonic GM-CSF, KC/GRO and IL-2 have not been as widely reported. The production of GM-CSF is related to delayed neutrophil apoptosis, which contributes to the tissue injury in IBD.32 Indeed, an increased secretion of GM-CSF had been found in mucosal lesions of mouse models and IBD patients.32,33 Our present study reports that dietary resveratrol down-regulated the enhanced GM-CSF production in DSS-induced colitis mice (Fig. 2C) for the first time. KC/GRO is a CXC chemokine, also known as chemokine (C-X-C motif) ligand 1 (CXCL1). During inflammation, it is involved in neutrophil activation and recruitment.34 It has been reported that the level of CXCL1 in serum is highly correlated with the severity of disease in IBD patients.34 The regulating effects of resveratrol on GM-CSF and KC/GRO suggest that the polyphenol may play a role in neutrophil apoptosis and infiltration in the colon.35 IL-2 supports the proliferation and differentiation of regulatory T cells. Subsequently, the aberrant expression of IL-2 promotes the imbalance of effector and regulatory T cells, which leads to multi-organ autoimmune disease and inflammation.36 Our results supported the regulating effects of dietary resveratrol on T cell-associated immune disorders.20
In our study, the observed differential expression of IL-10 in DSS+/RES− and DSS+/RES+ groups (Fig. 2C) was contradictory to previous studies.37 IL-10 exerts anti-inflammatory effects by inhibiting the production of pro-inflammatory cytokines such as IL-1, IL-6, IL-12, and TNF-α as well as chemokines.38 We speculate that the possible cause of increased IL-10 in our findings could be due to a triggered response to severe inflammation and increased expression of pro-inflammatory cytokines.39
Resveratrol partially ameliorated gut microbiota dysbiosis induced by DSS
Given the critical role of the gut microbiota in the pathogenesis of colitis, dietary resveratrol was investigated for its ability to counteract the state of chronic gut microbiota dysbiosis in DSS-treated mice. The influences of dietary resveratrol on the richness and evenness of the gut microbiota were assessed. The OTU number, Shannon diversity and Chao1 indices were measured as they are common measures of α-diversity that indicate the depth of sequence coverage and community diversity within samples.40 As shown in Fig. 3A, dietary resveratrol significantly enriched and diversified the gut microbiota of DSS−/RES+ mice (P < 0.05), while colitic, DSS-treated mice that did not receive resveratrol were found to have decreased abundance, richness, and diversity (P < 0.05) of the gut microbiota, which supported the prebiotic effects of dietary resveratrol. The relative similarity in the microbiota of the four treatment groups was visualized by PCoA (Fig. 3B) following β-diversity analysis. β-Diversity analysis was performed on a rarified sequence set, with a sampling depth equal to the smallest value of the filtered sequences.40 A significant difference between DSS−/RES− and DSS+/RES− groups was observed (P = 0.009), which suggested that DSS treatment dramatically shifted the gut microbiota. Dietary resveratrol shifted this grouping back toward the control group, clearly distinguishable from the DSS+/RES− group (P = 0.008) (Fig. 3B), which implied that the administration of resveratrol partially relieved the dysbiosis caused by DSS and the resulting inflammatory status. The presence of overlapping clusters between DSS−/RES− and DSS−/RES+ groups illustrated the relative similarity in the microbial composition between these two groups (Fig. 3B). The above results indicated that dietary resveratrol partially recovered the richness, abundance, and evenness of the gut microbiota in DSS-treated mice, potentially by protecting numerous specific commensal bacterial clades.
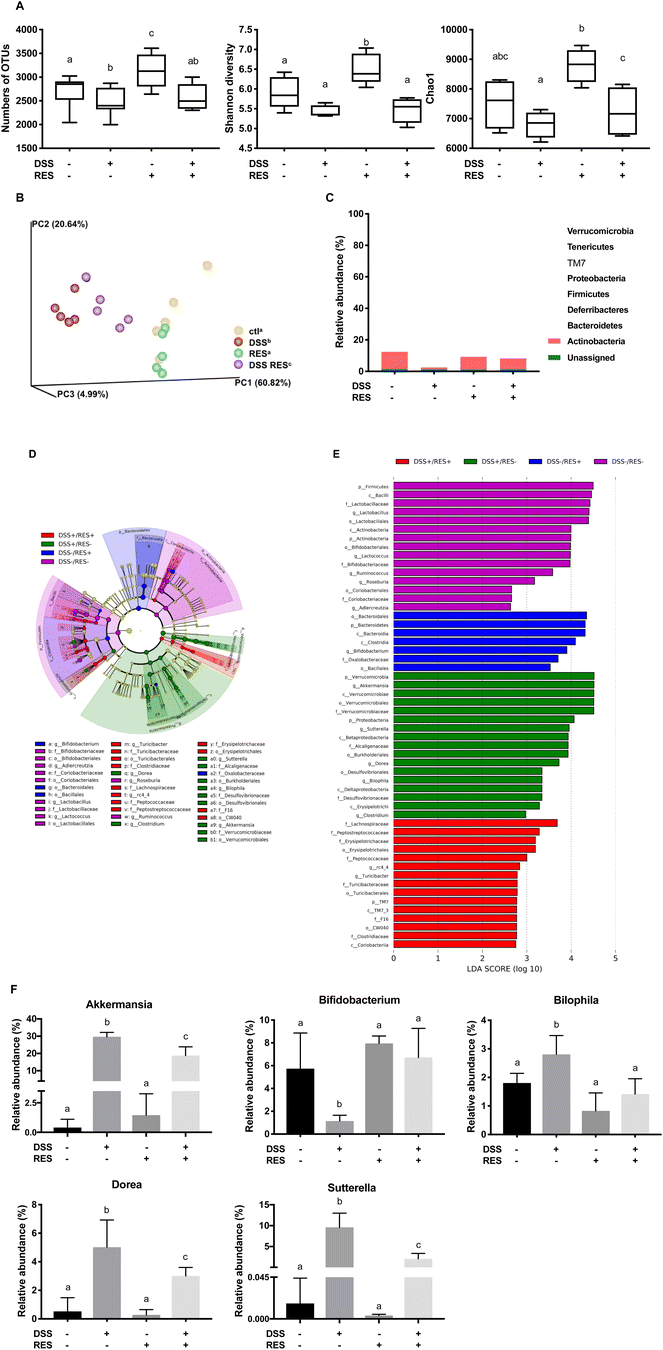 |
| Fig. 3 Dietary resveratrol partially relieved gut microbiota dysbiosis in colitic mice. (A) Effects of resveratrol on the α-diversity of the gut microbiota with different indices – numbers of OTUs, Shannon index, and Chao1 (P < 0.05); (B) effects of resveratrol on the β-diversity of the gut microbiota, visualized with PCoA plots (P < 0.01); (C) relative abundance of the gut microbiota at the phylum level; (D) taxonomic cladogram generated from default LEfSe analysis (α < 0.1 for the factorial Kruskal–Wallis test); (E) LDA scores of the differentially abundant taxa (with a LDA score of >2 and a significance of α < 0.1); (F) relative abundance of the gut microbiota at the genus level (P < 0.05). Data are presented as mean ± SD (n = 5). Different letters represent statistically significant differences. | |
To understand which bacterial clades were affected by dietary resveratrol, we evaluated the relative abundance of the predominant taxa within and among groups (Fig. 3C–F). Linear discriminant analysis effect size (LEfSe) algorithm with significance at p < 0.01 was then used to visualize the differentially representative bacterial clades for the microbiota of each experimental group (Fig. 3D and E). Consistent with previous studies, at the phylum level, we found that Firmicutes, Bacteroidetes, and Actinobacteria accounted for more than 95.0% of the whole gut microbial composition in the DSS−/RES− group.41,42 Bacteroidetes were significantly more abundant in DSS−/RES+ mice compared with the control group (P < 0.05) (Fig. 3C–E), which was in agreement with previous studies,43 and were mostly found in the class Bacteroidia and order Bacteroidales (Fig. 3D and E). Notably, DSS treatment significantly altered the bacterial structure at the phylum level by contributing to the expansion of Verrucomicrobia and Proteobacteria populations and reduction of Actinobacteria and Firmicutes populations versus DSS−/RES− mice (P < 0.05) (Fig. 3C–E), which was also in accordance with previous studies.44 Firmicutes, Bacteroidetes, and Actinobacteria only occupied 56.5% of the total gut microbial community in the DSS-treated group, which is 38.5% lower in terms of relative abundance than in the DSS−/RES− group. Dietary resveratrol partially reversed this phylum-level shift by limiting the abundance of Verrucomicrobia from 29.7% to 20.8% and Proteobacteria from 11.7% to 3.6% and increasing the abundance of Actinobacteria from 1.2% to 6.9% (P < 0.05) (Fig. 3C–E).
Akkermansia, Bifidobacterium, Bilophila, Dorea, and Sutterella were the most affected genera among the treatment groups (Fig. 3D–F). It was observed that there was atypical dominance of Akkermansia in the DSS+/RES− group, accounting for 29.7% of the mean population, while it only accounted for less than 1.5% of the microbial composition in DSS−/RES− and DSS−/RES+ groups. Importantly, dietary resveratrol significantly inhibited the excessive abundance of Akkermansia (18.8%) in DSS+/RES+ mice (P < 0.05) (Fig. 3D–F). Akkermansia is a Gram-negative, mucin-degrading bacteria of the Verrucomicrobia phylum, with only one species, Akkermansia muciniphila, identified to date.45 Its potential role in the development of colitis remains controversial. A. muciniphila was reported to be a potent anti-inflammatory intestinal bacterium that adhered to intestinal enterocytes, strengthened the integrity of the epithelial cell layer, and was inversely correlated with inflammation.46,47 However, A. muciniphila has also been reported to be increased in mice with DSS-induced colitis and patients suffering from colorectal cancer.48,49 Seregin et al. concluded that A. muciniphila acted as a pathobiont to promote colitis in a genetically susceptible host under the regulation of nod-like receptor 6 (NLRP6).50 Ganesh et al. demonstrated that the presence of A. muciniphila exacerbated Salmonella-induced colitis in gnotobiotic mice.51 The mechanism by which A. muciniphila promoted colitis will clearly need to be elucidated in future studies. Given its mucin-degrading properties, we speculated that A. muciniphila invaded and degraded the colon mucosa, which allowed for higher permeability and access to other microbes which drive inflammation.9,50
Dietary resveratrol also significantly inhibited the abnormal bloom of Bilophila, Sutterella, and Dorea observed in DSS-fed mice (P < 0.05) (Fig. 3D–F). Bilophila, belonging to the phylum Proteobacteria, is a sulfate-reducing bacteria associated with IBD and appendicitis.9,52Sutterella wadsworthensis, also belonging to the phylum Proteobacteria, has been reported to induce inflammation cascades by occupying the mucus layer and provoking host immune responses.53 Limited research on Dorea, belonging to the phylum Firmicutes, has been published. A clinical study indicated that Dorea was lower in Crohn's disease sufferers compared to healthy subjects.54 However, its potential role in the pathogenesis of IBD has not been discussed yet. In this work, we observed that dietary resveratrol significantly suppressed the relative abundance of Dorea from 5.0% to 3.8% in DSS-treated mice (Fig. 3F) and their abundance was positively correlated with higher expression of pro-inflammatory cytokines (shown below). These contradictory results lead to a need for future investigation to clarify the role of Dorea in the gut and its potential relationship with colon dysbiosis and inflammation. The above results indicated that dietary resveratrol partially suppressed the abnormal growth of potential pathobionts associated with colitis, including Akkermansia, Bilophila, Sutterella and Dorea (Fig. 3F).
Meanwhile, dietary resveratrol in DSS+ mice led to significantly increased counts of Bifidobacterium, with a 5-fold gain in its relative abundance compared to that in DSS+/RES− mice (P < 0.05). From the DSS−/RES− mean level of Bifidobacterium at 6.7%, its relative abundance dropped to 1.2% in DSS+/RES− mice and returned to a similar level to that of the control group upon resveratrol administration (P < 0.05) (Fig. 3D–F). The protective effects of Bifidobacterium against DSS-induced colitis, such as reduced pro-inflammatory cytokine expression and oxidative damage, has been demonstrated in other research studies.55 These results support the trend that dietary resveratrol alleviates microbial community perturbations by selectively restoring the abundance of probiotics and suppressing the growth or otherwise resisting out-competition of potential pathogens in a DSS-induced colitis mouse model.
Gut microbiota alterations are strongly correlated with the aberrant expression of cytokines
From these findings that dietary resveratrol partially resolved the gut microbiota dysbiosis in colitic mice, we then examined whether these changes were associated with the anti-colitis effects of dietary resveratrol. Correlations between the alterations of the gut microbiota and expressions of inflammatory cytokines were analyzed with Pearson's correlation analysis and visualized in a heatmap as shown in Fig. 4.
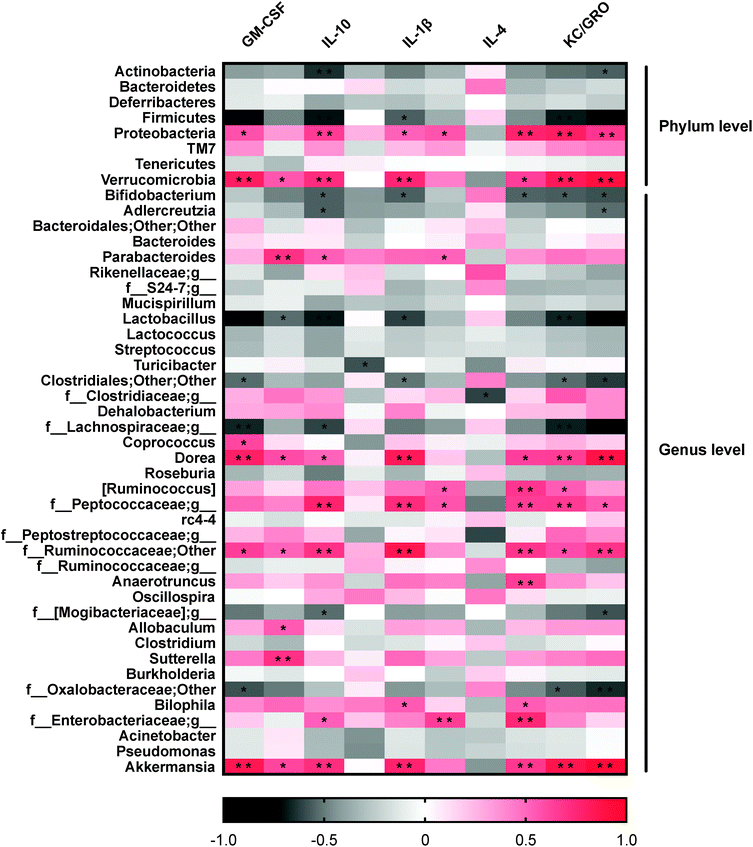 |
| Fig. 4 Heatmap of correlations between the gut microbiota and inflammatory cytokines. Positive correlation is represented in red. Negative correlation is represented in black. Significant differences are indicated as: *P < 0.05 and **P < 0.01. | |
Consistent positive correlations were observed between potential pathogenic organisms and pro-inflammatory cytokine expression levels. Proteobacteria abundance was significantly positively correlated with GM-CSF, IL-1β, IL-2, IL-6, KC/GRO and TNF-α (p < 0.05) expression levels (Fig. 4). Accordingly, at the genus level, the abundance of Bilophila and Sutterella of the same phylum were positively correlated with pro-inflammatory cytokines, including IL-1β, IL-6 and IFN-γ (p < 0.05). In general, Verrucomicrobia and one of its genera, Akkermansia, were significantly positively correlated with the expression of GM-CSF, IFN-γ, IL-1β, IL-6, KC/GRP, and TNF-α (P < 0.05) (Fig. 4). These results indicated that gut microbial variations may affect mucosal autoimmunity through regulating or inducing inflammatory cytokine expression.
It was also observed that certain bacterial clades negatively correlated with pro-inflammatory cytokines and positively correlated with anti-inflammatory cytokines. Specifically, Bifidobacterium was highly negatively correlated with IL-1β, IL-6, KC/GRO and TNF-α (P < 0.05), and positively correlated with IL-4. The same was true for Lactobacillus; negative correlations were observed with pro-inflammatory cytokines GM-CSF, IFN-γ, IL-1β, KC/GRO, and TNF-α (P < 0.05). The protective effect of Lactobacillus and Bifidobacterium in DSS-induced colitis against inflammation by reducing TNF-α levels has been discussed previously.55 These data provide additional clues to the causality between these observations and the illustrated potential modes for the pathogenesis of IBD.
Conclusion
In summary, our results corroborated the anti-colitis effects of dietary resveratrol (at a dose of 2.3 mg kg−1 day−1 human equivalent) in DSS-induced colitic mice and provided evidence for the modulatory effects of dietary resveratrol on the gut microbiota, including the restoration of bacterial community diversity and the rebalance of probiotics and pathobionts. It was revealed that resveratrol administration not only prevented losses in body weight associated with IBD and reduced the degree of disease activity and other markers of colon inflammation but also protected colonic tissue from structure deterioration and dysplasia, and reduced the expression of several pro-inflammatory cytokines when compared to the respective traits in colitic mice that did not receive dietary resveratrol. Based on these results, we further revealed that the alterations in the abundance of select gut microbiota were significantly correlated with the expression of inflammatory cytokines, leading us to propose that dietary resveratrol might prevent colitis to a large extent by modulating the gut microbiota.
Abbreviations
DSS | Dextran sulfate sodium |
RES | Resveratrol |
IBD | Inflammatory bowel disease |
DAI | Disease activity index |
H&E | Hematoxylin & eosin |
OTUs | Operational taxonomic units |
PCoA | Principal coordinate analysis |
TNF-α | Tumor necrosis factor alpha |
IL-1β | Interleukin 1 beta |
GM-CSF | Granulocyte-macrophage colony-stimulating factor |
IL-2 | Interleukin 2 |
IL-6 | Interleukin 6 |
IL-10 | Interleukin 10 |
IFN-γ | Interferon gamma |
KC/GRO | Keratinocyte chemoattractant/human growth-regulated oncogene |
CXCL1 | Chemokine (C-X-C motif) ligand 1 |
LDA | Linear discriminant analysis |
Conflicts of interest
There are no conflicts to declare.
Acknowledgements
This work was supported in part by the USDA/NIFA, the Hatch Fund, and the National Institutes of Health (R01 AT010229).
References
- B. Khor, A. Gardet and R. J. Xavier, Genetics and pathogenesis of inflammatory bowel disease, Nature, 2011, 474(7351), 307–317 CrossRef CAS.
- A. Nishida,
et al., Gut microbiota in the pathogenesis of inflammatory bowel disease, Clin. J. Gastroenterol., 2018, 11(1), 1–10 CrossRef PubMed.
- K. Takahashi,
et al., Reduced Abundance of Butyrate-Producing Bacteria Species in the Fecal Microbial Community in Crohn's Disease, Digestion, 2016, 93(1), 59–65 CAS.
- T. Fujimoto,
et al., Decreased abundance of Faecalibacterium prausnitzii in the gut microbiota of Crohn's disease, J. Gastroenterol. Hepatol., 2013, 28(4), 613–619 CrossRef CAS.
- C. Manichanh,
et al., Reduced diversity of faecal microbiota in Crohn's disease revealed by a metagenomic approach, Gut, 2006, 55(2), 205 CrossRef CAS.
- W. Zhu,
et al., Precision editing of the gut microbiota ameliorates colitis, Nature, 2018, 553, 208–211 CrossRef CAS.
- R. K. Sellon,
et al., Resident Enteric Bacteria Are Necessary for Development of Spontaneous Colitis and Immune System Activation in Interleukin-10-Deficient Mice, Infect. Immun., 1998, 66(11), 5224–5231 CrossRef CAS.
- J. M. Laparra and Y. Sanz, Interactions of gut microbiota with functional food components and nutraceuticals, Pharmacol. Res., 2010, 61(3), 219–225 CrossRef CAS PubMed.
-
Y. Han, et al., Dietary Intake of Whole Strawberry Inhibited Colonic Inflammation in Dextran-Sulfate-Sodium-Treated Mice via Restoring Immune Homeostasis and Alleviating Gut Microbiota Dysbiosis, 2019 Search PubMed.
- F. Cardona,
et al., Benefits of polyphenols on gut microbiota and implications in human health, J. Nutr. Biochem., 2013, 24(8), 1415–1422 CrossRef CAS.
- M. Azorin-Ortuno,
et al., Metabolites and tissue distribution of resveratrol in the pig, Mol. Nutr. Food Res., 2011, 55(8), 1154–1168 CrossRef CAS.
- Y. Qiao,
et al., Effects of resveratrol on gut microbiota and fat storage in a mouse model with high-fat-induced obesity, Food Funct., 2014, 5(6), 1241–1249 RSC.
- U. Etxeberria,
et al., Reshaping faecal gut microbiota composition by the intake of trans-resveratrol and quercetin in high-fat sucrose diet-fed rats, J. Nutr. Biochem., 2015, 26(6), 651–660 CrossRef CAS.
- J. Yao,
et al., Anti-oxidant effects of resveratrol on mice with DSS-induced ulcerative colitis, Arch. Med. Res., 2010, 41(4), 288–294 CrossRef CAS.
- X. Cui,
et al., Resveratrol Suppresses Colitis and Colon Cancer Associated with Colitis, Cancer Prev. Res., 2010, 3(4), 549–559 CrossRef CAS.
- U. P. Singh,
et al., Resveratrol (trans-3,5,4′-trihydroxystilbene) induces silent mating type information regulation-1 and down-regulates nuclear transcription factor-kappaB activation to abrogate dextran sulfate sodium-induced colitis, J. Pharmacol. Exp. Ther., 2010, 332(3), 829–839 CrossRef CAS.
- M. Larrosa,
et al., Effect of a low dose of dietary resveratrol on colon microbiota, inflammation and tissue damage in a DSS-induced colitis rat model, J. Agric. Food Chem., 2009, 57(6), 2211–2220 CrossRef CAS.
- X. Cui,
et al., Resveratrol suppresses colitis and colon cancer associated with colitis, Cancer Prev. Res., 2010, 3(4), 549–559 CrossRef CAS.
- M. Samsami-Kor,
et al., Anti-Inflammatory Effects of Resveratrol in Patients with Ulcerative Colitis: A Randomized, Double-Blind, Placebo-controlled Pilot Study, Arch. Med. Res., 2015, 46(4), 280–285 CrossRef CAS.
- H. R. Alrafas,
et al., Resveratrol modulates the gut microbiota to prevent murine colitis development through induction of Tregs and suppression of Th17 cells, J. Leukoc. Biol., 2019, 106(2), 467–480 CrossRef CAS.
- J. J. Kim,
et al., Investigating Intestinal Inflammation in DSS-induced Model of IBD, J. Visualized Exp., 2012,(60), 3678 Search PubMed.
- W. Xian,
et al., Chemopreventive effects of nobiletin and its colonic metabolites on colon carcinogenesis, Mol. Nutr. Food Res., 2015, 59(12), 2383–2394 CrossRef.
- S. Yue,
et al., Identification of pinostilbene as a major colonic metabolite of pterostilbene and its inhibitory effects on colon cancer cells, Mol. Nutr. Food Res., 2016, 60(9), 1924–1932 CrossRef PubMed.
- M. Song,
et al., Dietary 5-demethylnobiletin inhibits cigarette carcinogen NNK-induced lung tumorigenesis in mice, Food Funct., 2017, 8(3), 954–963 RSC.
- Y. Araki,
et al., Development of dextran sulphate sodium-induced experimental colitis is suppressed in genetically mast cell-deficient Ws/Ws rats, Clin. Exp. Immunol., 2000, 119(2), 264–269 CrossRef CAS.
- A. Klindworth,
et al., Evaluation of general 16S ribosomal RNA gene PCR primers for classical and next-generation sequencing-based diversity studies, Nucleic Acids Res., 2013, 41(1), e1 CrossRef CAS.
- J. G. Caporaso,
et al., QIIME allows analysis of high-throughput community sequencing data, Nat. Methods, 2010, 7(5), 335–336 CrossRef CAS.
- N. Segata,
et al., Metagenomic biomarker discovery and explanation, Genome Biol., 2011, 12(6), R60 CrossRef PubMed.
- M. L. Clapper, H. S. Cooper and W. C. Chang, Dextran sulfate sodium-induced colitis-associated neoplasia: a promising model for the development of chemopreventive interventions, Acta Pharmacol. Sin., 2007, 28(9), 1450–1459 CrossRef CAS.
- Q. Guan and J. Zhang, Recent Advances: The Imbalance of Cytokines in the Pathogenesis of Inflammatory Bowel Disease, Mediators Inflammation, 2017, 2017, 8 Search PubMed.
- J. Yao,
et al., Polydatin ameliorates DSS-induced colitis in mice through inhibition of nuclear factor-kappaB activation, Planta Med., 2011, 77(5), 421–427 CrossRef CAS.
- M. Noguchi,
et al., Increased Secretion of Granulocyte-Macrophage Colony-Stimulating Factor in Mucosal Lesions of Inflammatory Bowel Disease, Digestion, 2001, 63(suppl 1), 32–36 CAS.
- L. A. Dieleman,
et al., Dextran sulfate sodium-induced colitis occurs in severe combined immunodeficient mice, Gastroenterology, 1994, 107(6), 1643–1652 CrossRef CAS.
- K. Mitsuyama,
et al., Increased circulating concentrations of growth-related oncogene (GRO)-alpha in patients with inflammatory bowel disease, Dig. Dis Sci., 2006, 51(1), 173–177 CrossRef CAS.
-
Y. Mayangsari and T. Suzuki, Resveratrol Ameliorates Intestinal Barrier Defects and Inflammation in Colitic Mice and Intestinal Cells, 2018, vol. 66(48), pp. 12666–12674 Search PubMed.
- D. Klatzmann and A. K. Abbas, The promise of low-dose interleukin-2 therapy for autoimmune and inflammatory diseases, Nat. Rev. Immunol., 2015, 15, 283 CrossRef CAS.
- S. Sánchez-Fidalgo,
et al., Dietary supplementation of resveratrol attenuates chronic colonic inflammation in mice, Eur. J. Pharmacol., 2010, 633(1), 78–84 CrossRef.
- M. Saraiva and A. O'Garra, The regulation of IL-10 production by immune cells, Nat. Rev. Immunol., 2010, 10, 170 CrossRef CAS.
- W. H. Peranteau,
et al., IL-10 Overexpression Decreases Inflammatory Mediators and Promotes Regenerative Healing in an Adult Model of Scar Formation, J. Invest. Dermatol., 2008, 128(7), 1852–1860 CrossRef CAS PubMed.
- T. Odamaki,
et al., Age-related changes in gut microbiota composition from newborn to centenarian: a cross-sectional study, BMC Microbiol., 2016, 16, 90 CrossRef.
- P. M. Munyaka,
et al., Acute dextran sulfate sodium (DSS)-induced colitis promotes gut microbial dysbiosis in mice, J. Basic Microbiol., 2016, 56(9), 986–998 CrossRef CAS.
- S. M. Jandhyala,
et al., Role of the normal gut microbiota, World J. Gastroenterol., 2015, 21(29), 8787–8803 CrossRef CAS.
- M.-l. Chen,
et al., Resveratrol attenuates trimethylamine-N-oxide (TMAO)-induced atherosclerosis by regulating TMAO synthesis and bile acid metabolism via remodeling of the gut microbiota, mBio, 2016, 7(2), e02210–e02215 CrossRef CAS.
- L. E. Ritchie,
et al., Polyphenol-rich sorghum brans alter colon microbiota and impact species diversity and species richness after multiple bouts of dextran sodium sulfate-induced colitis, FEMS Microbiol. Ecol., 2015, 91(3), fiv008 CrossRef.
- M. Derrien,
et al., The Mucin Degrader Akkermansia muciniphila Is an Abundant Resident of the Human Intestinal Tract, Appl. Environ. Microbiol., 2008, 74(5), 1646–1648 CrossRef CAS.
- J. Reunanen,
et al., Akkermansia muciniphila adheres to enterocytes and strengthens the integrity of epithelial cell layer, Appl. Environ. Microbiol., 2015, 81(11), 3655–3662 CrossRef CAS.
- F. F. Anhe,
et al., A polyphenol-rich cranberry extract protects from diet-induced obesity, insulin resistance and intestinal inflammation in association with increased Akkermansia spp. population in the gut microbiota of mice, Gut, 2015, 64(6), 872–883 CrossRef CAS.
- C. Schwab,
et al., Longitudinal study of murine microbiota activity and interactions with the host during acute inflammation and recovery, ISME J., 2014, 8, 1101 CrossRef CAS.
- T. L. Weir,
et al., Stool Microbiome and Metabolome Differences between Colorectal Cancer Patients and Healthy Adults, PLoS One, 2013, 8(8), e70803 CrossRef CAS.
- S. S. Seregin,
et al., NLRP6 Protects Il10(-/-) Mice from Colitis by Limiting Colonization of Akkermansia
muciniphila, Cell Rep., 2017, 19(4), 733–745 CrossRef CAS.
- B. P. Ganesh,
et al., Commensal Akkermansia muciniphila exacerbates gut inflammation in Salmonella Typhimurium-infected gnotobiotic mice, PLoS One, 2013, 8(9), e74963 CrossRef CAS.
- J. Loubinoux,
et al., Sulfate-reducing bacteria in human feces and their association with inflammatory bowel diseases, FEMS Microbiol. Ecol., 2002, 40(2), 107–112 CrossRef CAS.
- K. Hiippala,
et al., Mucosal Prevalence and Interactions with the Epithelium Indicate Commensalism of Sutterella spp, Front. Microbiol., 2016, 7, 1706 Search PubMed.
- G. Liguori,
et al., Fungal Dysbiosis in Mucosa-associated Microbiota of Crohn's Disease Patients, J. Crohn's Colitis, 2016, 10(3), 296–305 CrossRef.
- L. J. Fooks and G. R. Gibson, Probiotics as modulators of the gut flora, Br. J. Nutr., 2002, 88(Suppl 1), S39–S49 CrossRef CAS.
Footnote |
† These authors contributed equally to this work. |
|
This journal is © The Royal Society of Chemistry 2020 |
Click here to see how this site uses Cookies. View our privacy policy here.