DOI:
10.1039/C9FO02424G
(Paper)
Food Funct., 2020,
11, 932-943
Consumption of a green tea extract–curcumin drink decreases blood urea nitrogen and redox iron in β-thalassemia patients†
Received
16th October 2019
, Accepted 26th December 2019
First published on 26th December 2019
Abstract
The most important cause of death in β-thalassemia major patients is organ dysfunction due to iron deposits. Non-transferrin bound iron (NTBI), labile plasma iron (LPI) and labile iron pool are redox-active forms of iron found in thalassemia. Iron chelation therapy is adopted to counteract the resulting iron overload. Extracts of green tea (GTE) and curcumin exhibit iron-chelating and antioxidant activities in iron-loaded cells and β-thalassemic mice. We have used our GTE-CUR drink to investigate the potential amelioration of iron overload and oxidative stress in transfusion-dependent β-thalassemia (TDT) patients. The patients were enrolled for a control group without and with GTE-CUR treatments (17.3 and 35.5 mg EGCG equivalent). Along with regular chelation therapy, they were daily administered the drink for 60 d. Blood samples were collected at the beginning of the study and after 30 d and 60 d for biochemical and hematological tests. Interestingly, we found a decrease of blood urea nitrogen levels (P < 0.05), along with a tendency for a decrease of NTBI and LPI, and a delay in increasing lipid-peroxidation product levels in the GTE-CUR groups. The findings suggest that GTE-CUR could increase kidney function and diminish redox-active iron in iron overloaded β-thalassemia patients.
1. Introduction
A short red blood cell (RBC) lifespan is mainly caused by ineffective erythropoiesis and excessive iron in β-thalassemia patients. Many patients have to receive continual blood transfusions which lead to the inevitable consequence of iron accumulation over time. Toxic iron forms such as non-transferrin bound iron (NTBI) and labile plasma iron (LPI) in the plasma compartment, and intracellular labile iron pools (LIP) present redox activity via the Fenton reaction. Such iron catalyzes the production of reactive oxygen species (ROS) which can oxidize biomolecules causing tissue damage and organ dysfunction.1 Oxidative renal tubular damage in β-thalassemia patients may be caused by iron overload-mediated lipid peroxidation.2
Desferrioxamine, deferiprone (DFP) and deferasirox (DFX) are effective iron chelators used for the treatment of β-thalassemia patients with iron overload.3 However, they cause adverse effects and can induce serious complications. Combination therapy is a possible option of interest while adjunctive treatment can minimize the side effect.4 Modified medical regimens involving iron chelator combinations, adjunctive iron chelators and antioxidants, and intermittent oral drug administration are currently under investigation in patients.5–9
Green tea extract (GTE) made from tea (Camellia sinensis) leaves contain mostly catechin (C), epicatechin (EC), epicatechin-3-gallate (ECG), epigallocatechin (EGC) and epigallocatechin-3-gallate (EGCG). EGCG is the most abundant and exhibits potent antioxidant and iron-chelating activity.10–12Curcuma longa Linn rhizomes is abundant with curcuminoids (CUR) as curcumin (C), demethoxycurcumin (DMC) and bis-demethoxycurcumin (BDMC) which exhibit anti-inflammatory and iron-chelating activities.13 Our studies show that GTE and CUR can effectively lower the levels of NTBI, LPI, LIP and lipid-peroxidation products in iron loaded-cells and β-thalassemia subjects.10,14–19 Indeed, the quality of tea is mainly attributed to its polyphenol and caffeine contents, which depend upon the strain of the tea, the season of collection, the altitude of the growing location, the extraction methods and the batch-to-batch preparation procedure. Therefore, the evaluation process needs to be fully verified. Catechin contents in GTE are varied as 5–287 mg L−1 EGC, 17–94 mg L−1 EC, 18–408 mg L−1 EGCG, and 8–85 mg L−1 ECG, while other phenolic compounds are present in lesser amounts and are less effective at iron chelation. In particular, the ability of EGCG to exhibit important free-radical scavenging and iron-chelating activities has been recognized. It has been suggested that EGCG can serve as a specific biomarker for the evaluation of the consumption of tea. With regard to therapeutic efficacy, we have paid a good deal of attention to the quality, safety and consumption acceptance of our green tea–curcumin (GTE-CUR) drink at different EGCG doses (17.3 mg and 35.5 mg EGCG equivalent). In this study, we developed a GTE-CUR drink and investigated its influence on the improvement of iron overload, oxidative stress and erythropoiesis in β-thalassemia patients.
2. Methods and materials
2.1. Chemicals
2,2′-Azino-bis(3-ethylbenzthiazoline-6-sulphonic acid) diammonium (ABTS); butylated hydroxytoluene (BHT); 6-hydroxy-2,5,7,8-tetramethylchroman-2-carboxylic acid (trolox); 3-(N-morpholino)sulfonate (MOPS); nitrilotriacetic acid (NTA); 1,1,3,3-tetramethoxypropane; thiobarbituric acid (TBA) and trichloroacetic acid (TCA) were obtained from Sigma-Aldrich Chemicals Company (St Louis, MO, USA). Standard caffeine, curcuminoids, epicatechin (EC), epicatechin-3-gallate (ECG) and epigallocatechin-3-gallate (EGCG) were also purchased from Sigma-Aldrich Chemicals Company (St Louis, MO, USA). Elecsys® ferritin test kits were purchased from Roche Diagnostics International Ltd, Rotkreuz, Switzerland. Clinical chemistry reagents were purchased from Randox® Laboratories Ltd, County Antrim, UK. Hematology reagents were supplied by Beckman Coulter, Inc., Atlanta, Georgia, USA.
2.2. Preparation of oolong tea and GTE
Dry oolong tea and green tea (C. sinensis var. assamica) were purchased from Suwiroon Thai Tea Factory in Chiang Rai. The tea was finely ground using an ultra-centrifugal milling machine (Retsch Model ZM200) and passed through a nylon mesh screen (polyester, 60–100 μm particle size) that was fitted in a vibration separator machine. The tea samples were then stored separately in plastic bags in a vacuum at 4 °C for further use. Fine oolong tea powder was extracted with hot water (5 g per 100 mL) at 80 °C for 3 min and filtered through cellulose paper (Whatman No. 4, Maidstone, UK) in a vacuum to obtain the oolong tea concentrate.15 Fine green tea powder was extracted in 70% ethanol (1.0 g per 5 mL) with shaking (110 rpm, 4.5 h), and filtered through cellulose-type paper in a vacuum. Ethanol was then removed using a rotatory evaporator (60 °C, 180 rpm), and the tea was dried with a freeze-drying machine.20 Finally, GTE (1%, w/v) was mixed with maltodextrin (4%, w/v) and cyclodextrin (3% w/v) and then dried using a spray-drying machine (inlet temperature 155–160 °C, outlet temperature 85–90 °C). Catechin derivatives were analyzed using the high performance liquid chromatography/ultraviolet (HPLC/UV) detection method with the inclusion of a column (C18, 250 mm × 4.6 mm, 5 μm, temperature at 25–30 °C) using linear-gradient elution by varying the proportion of the mobile-phase solvent A (0.2% o-phosphoric acid/acetonitrile/tetrahydrofuran (THF) (86/12.5/1.5, v/v/v) and the mobile-phase solvent B (0.2% o-phosphoric acid/acetonitrile/THF (73.5/25/1.5, v/v/v). The elution protocol was followed by adding 0–100% of mobile phase A (30 min) while the volume of mobile phase B was gradually increased from 0–100% (10 min, hold 20 min) at a flow rate of 1.0 mL min−1. Mobile phase B was then slowly decreased to an initial condition of 100% mobile phase A (10 min, hold 20 min). Additionally, the detection wavelength was set at 280 nm and the running time was 90 min (Fig. S1†).21
2.3. Preparation of the CUR extract
Tumeric (C. longa) was finely ground, extracted with 75% ethanol and freeze-dried. The CUR extract was mixed with the GTE and other ingredients in order to prepare the GTE-CUR drink (1×, 2.5× and 5× concentrate). The curcuminoid contents were then analyzed by HPLC, in which the system was composed of the isocratic elution with a mobile phase of acetonitrile and 2% acetic acid (40
:
60, v/v), a flow rate of 2.0 mL min−1, a detection wavelength of 425 nm and a running time of 16 min (Fig. S2†).22 The eluted curcuminoids were compared with authentic curcuminoids for appropriate positioning and to calculate their concentrations.
2.4. Formulation of the GTE-CUR drink
We used the Design Expert version 6.2.10 Program (Stat Ease Inc., MN), Product- and Consumer-Oriented tests to optimize the ingredient composition and obtain the most suitable product. The ingredients shown in Table 1 were mixed and adjusted to achieve the finished GTE-CUR product coded as 2.5× and 5× when the concentrations were equivalent to 17.3 mg and 35.5 mg EGCG, respectively, by calculation. Caffeine content was determined using reverse-phase HPLC with the following conditions: a column (ODS-type, 4.6 mm × 250 mm, 5 μm), isocratic mobile-phase solvent (0.2% phosphoric acid and acetonitrile, 90
:
10 by volume), a flow rate of 1.0 mL min−1, and achieved detection at 275 nm (Fig. S3†).23 The inductively coupled plasma-mass spectrometry method under the specified conditions was used for the quantitation of aluminum (Al) and iron (Fe).24,25 The physiochemical properties and microbiological contamination were determined (Table 2). In addition, the 9-point hedonic scaling-based sensory test for the GTE-CUR drink was used in the evaluation of two hundred healthy volunteers.
Table 1 Appropriate compositions of the GTE-CUR drink (45 mL) (2.5× = 17.3 mg EGCG equivalent, 5× = 35.5 mg EGCG equivalent)
Additive ingredients |
GTE-CUR (1×) |
GTE-CUR (2.5×) |
GTE-CUR (5×) |
5% oolong concentrate (mL) |
31.2 |
21.4 |
14.4 |
Honey (mL) |
6.7 |
11.4 |
13.33 |
GTE powder (g) |
0.99 |
1.70 |
3.5 |
Curcuminoid extract (mg) |
60 |
110 |
140 |
L-Ascorbic acid (g) |
0.20 |
0.34 |
0.44 |
Table salt (g) |
0.03 |
0.05 |
0.07 |
Oligofructose (mL) |
4.5 |
7.7 |
10.1 |
Inulin (g) |
1.35 |
2.31 |
3.03 |
Table 2 Analysis of the experimental GTE-CUR drink (45 mL serving)
Presentation |
GTE-CUR (1×) |
GTE-CUR (2.5×) |
GTE-CUR (5×) |
Abbreviations/symbols: CFU = colony-forming unit; MPN = most probable numbers; ND = not determined. |
Physicochemical properties
|
pH |
3.65 ± 0.02 |
ND |
ND |
Total acidity (%) |
4.28 ± 0.36 |
ND |
ND |
Soluble solid (°Brix) |
30.67 ± 0.26 |
ND |
ND |
Color |
L* |
22.83 ± 1.91 |
ND |
ND |
a* |
−0.81 ± 0.14 |
ND |
ND |
b* |
8.25 ± 0.52 |
ND |
ND |
Microbiological analysis
|
Total plate count (CFU g−1) |
<10 |
ND |
ND |
Yeast and mold (CFU g−1) |
<10 |
ND |
ND |
Total coliform bacteria (MPN g−1) |
<3 |
ND |
ND |
2.5. Ethics statement
The study protocol has been approved by the Research Ethical Committee for Human Study of Faculty of Medicine, Chiang Mai University, Chiang Mai, Thailand (Study Code: MED 2557-02201). All patients gave their informed consent before their inclusion in the study.
2.6. Patients and study design
Thirty-five transfusion-dependent Thai β-thalassemia patients (TDT) who regularly came for their physical and blood examinations at the Adult Thalassemia Clinic, Maharaj Nakorn Chiang Mai Hospital, Faculty of Medicine, Chiang Mai University, Chiang Mai, Thailand were enrolled in this study under inclusion and exclusion criteria. The subjects had not received any treatment (except for blood transfusion and iron chelation therapy) for at least 3 m prior to the study. Results of physical examinations including height, body weight, body mass index (BMI), and liver and spleen palpability were recorded. Using stratified sampling, the subjects were divided into three groups: no GTE-CUR (n = 12), GTE-CUR (17.3 mg EGCG equivalent, n = 12), and GTE-CUR (35.5 mg EGCG equivalent, n = 11), and received the product daily for 60 d. The patients were requested to cease receiving iron chelation for 72 h before blood collection and to fast overnight. Blood was collected from 8.00–9.00 am before the next blood transfusion in order to obtain the baseline data and after receiving the drink for 1 and 2 m for the analysis of hematological and biochemical parameters.
2.7. Assay of hematological and biochemical parameters
Blood cell indices were analyzed by using a Beckman Coulter Automate cell counter according to the manufacturer's instruction. Blood biochemical parameters, including urea nitrogen, creatinine (CRE), total protein, albumin, cholesterol, alanine aminotransferase (ALT), aspartate aminotransferase (AST), alkaline phosphatase (ALP) and bilirubin levels, were analyzed by using a Randox® Automated machine according to the manufacturer's instruction.
2.8. Quantification of thiobarbituric acid-reactive substances (TBARS)
TBARS, which are lipid peroxidation products, were measured by using a colorimetric method.26,27 Serum was deproteinized with the reagent containing 10% (w/v) TCA and 50 mg L−1 (w/v) BHT and heated for 30 min at 90 °C. After centrifugation, the supernatant was mixed with the chromogenic solution containing 0.44 M H3PO4 and 0.6% (w/v) TBA. The mixture was heated for 30 min at 90 °C to produce a pink colored product that was represented as TBARS, which was measured photometrically at 532 nm. TBARS concentrations were determined from a standard curve constructed by varied concentrations of 1,1,3,3-tetramethoxypropane (0–100 μM).
2.9. Determination of serum antioxidant activity
Antioxidant activity was determined using the method established by Re and colleagues based on decolorization of the 2,2′-azino-bis(3-ethylbenzthiazoline-6-sulphonic acid) diammonium radical cation (ABTS˙+).28 Briefly, working ABTS˙+ solution was incubated with serum or standard trolox solution for exactly 6 min and measured photometrically at 734 nm against the reagent blank. The antioxidant value was determined from a standard curve constructed from varied concentrations of 6-hydroxy-2,5,7,8-tetramethylchroman-2-carboxylic acid (trolox) solution and expressed as trolox equivalent antioxidant capacity (TEAC).
2.10. Quantification of serum NTBI
NTBI assay was performed using an iron-sensitive fluorescent bead method established by Ma and colleagues.29 Serum was incubated with 800 mM nitrilotriacetic acid (NTA), pH 7.0, solution at room temperature for 30 min and centrifuged through a filtration membrane (10 kDa cutoff, polysulfone type) at 10
000 rpm and 15 °C for 50 min to obtain the Fe-NTA filtrate. The filtrate and standard iron solution were then incubated with an iron-sensitive probe (2.8 × 106 beads in 50 mM MOPS buffer, pH 7.4, at 37 °C for 16 h and the fluorescence intensity (FI) was measured with a flow cytometer (Guava easyCyte 6HT-2L System set up at λex 495 nm, λem 525 nm). Guava InCyte software was used for data acquisition and analysis. The population gates were selected from dot-plots of the control bead area and the FI of 5000 events. In the calibration, the beads mixed with a normal human serum as a control were used to set up the FI at 100% and the relative FI of the beads with patient serum is calculated accordingly. The Fe calibration curve was produced between peak height values against a standard iron solution (0–10 μM ferric-NTA in 80 mM NTA solution). Non-linear regression assuming variable slope sigmoidal dose–response function was used to determine the NTBI concentration.30
2.11. Measurement of LPI
LPI assay was performed using a fluorogenic probe method.31 In the assay, serum was incubated with or without 5 mM DFP at 37 °C for 30 min and the dihydrorhodamine (DHR) solution containing ascorbic acid was added. Kinetics of increasing FI were followed immediately for 40 min with every 2 min with readings at 37 °C using a spectrofluorometer (λex 485 nm, λem 538 nm). The slope of the FI was plotted against the reaction time. A calibration curve was constructed from standard ferrous ammonium sulfate solution (0–20 μM). Differences in the rate of DHR oxidation represent a component of redox active LPI. The LPI concentration was calculated from the curve of the relative differences in the slope with or without DFP versus the standard iron concentration, as has been described in the established method.
2.12. Measurement of serum ferritin
Serum ferritin concentrations were measured by using the chemiluminescence immune assay (ECLIA, COBAS Elecsys®) technology according to the manufacturer's instructions.
2.13. Analysis of serum catechins
Serum levels of catechin derivatives were measured using the reverse-phase HPLC/UV method with slight modification.32 During the assay, the serum, without β-glucuronidase treatment was deproteinized by centrifugation on a filter membrane (polyethersulfone type, 30 kDa cutoff) and analyzed using the HPLC system. The conditions included a column (Agilent 1260 Infinity Quaternary LC Model, Agilent Technologies; ODS type, dimension 150 mm × 3.0 mm ID, 5 μm pore size), and the mobile phase was composed of an aqueous solution of 0.2% (v/v) acetonitrile (A) and methanol (B), a linear gradient elution of 0–50% B for 0–12 min and 50–100% B for 13–20 min, a flow rate of 1.0 mL min−1, and detection at 280 nm. The post-running period for the washing step was 5 min, and the column temperature was set at 25 °C, and while the sample injection volume was 20 μL. Additionally, HPLC ChemStation software was used to integrate and analyze the corresponding peaks. Authentic catechins were used to construct a standard curve for the calculation of serum catechin concentrations. Chromatographic peaks of analytes were identified by comparison with the retention time of the standards. The recovery and detection limit for catechins and gallic acid were 77–94% and 3 ng mL−1 as has been described in the original method.
2.14. Statistical analysis
Data were analyzed using IBM SPSS Statistics 20 program and are expressed as mean ± SD. Statistical significance was determined using Student's unpaired t test or one-way analysis of variance (ANOVA). A P value <0.05 was considered significant.
3. Results and discussion
3.1. The GTE-CUR drink
We found that 1.0 g of GTE contained 2.98 ± 0.15 mg GC, 9.74 ± 0.24 mg EGC, 2.74 ± 0.09 mg EC, 10.15 ± 0.11 mg EGCG and 4.46 ± 0.22 mg ECG, whereas 1.0 g of the curcuminoid extract contained 179.5 ± 3.9 mg C, 97.8 ± 2.4 mg DMC and 52.0 ± 1.5 mg BDMC. Some amounts of caffeine, Fe and Al were detectable in the GTE-CUR product (Table 3). Interestingly, most of the consumers were satisfied with the product (77%) and were likely to purchase it (60%).
Table 3 Composition analysis of the experimental GTE-CUR drink (45 mL serving)
Presentation |
GTE-CUR (1×) |
GTE-CUR (2.5×) |
GTE-CUR (5×) |
Abbreviations/symbols: ABTS = 2,2′-azino-bis(3-ethylbenzthiazoline-6-sulphonic acid, Al = aluminum; BDMC = bis-demethoxycurcumin, C = curcumin, DMC = demethoxycurcumin, EC = epicatechin, EGC = epigallocatechin, ECG = epicatechin-3-gallate, EGCG = epigallocatechin-3-gallate; Fe = iron; GAE = gallic acid equivalent; GTE = green tea extract; ND = not determined; TE = trolox equivalent; TPC = total phenolic content; TTC = total tannin content. |
TTC (%) |
79.54 ± 1.42 |
ND |
ND |
ABTS˙-scavenging (mg TE per mL) |
61.05 ± 0.33 |
ND |
ND |
TPC (mg GAE per mL) |
27.05 ± 0.52 |
ND |
ND |
Curcuminoids: |
C (mg) |
10.8 |
19.7 |
25.1 |
DMC (mg) |
5.9 |
10.8 |
13.7 |
BDMC (mg) |
3.12 |
5.7 |
7.3 |
Catechins: |
GC (mg) |
2.95 |
5.07 |
10.43 |
EGC (mg) |
9.64 |
16.56 |
34.09 |
EC (mg) |
2.71 |
4.66 |
9.59 |
EGCG (mg) |
10.04 |
17.26 |
35.53 |
ECG (mg) |
4.41 |
7.58 |
9.59 |
Caffeine (mg) |
ND |
5.4 |
7.7 |
Al (μg) |
ND |
51 |
ND |
Fe (μg) |
ND |
58 |
ND |
3.2. Demographic characteristics of patients
A total of thirty-five Thai patients were selected who are transfusion-dependent β-thalassemia major and β-thalassemia/HbE patients that routinely visited the hospital to check their physical state and undergo blood examinations at the Adult Thalassemia Clinic, Maharaj Nakorn Chiang Mai Hospital, Faculty of Medicine, Chiang Mai University during the data collection period of this study. The main inclusion criteria were serum NTBI > 2 μM, LPI > 2 μM, and ferritin > 1500 ng mL−1. All patients were assessed by experienced hematologists. Most of the patients were splenectomized and were regularly administered with either monotherapy (DFO, DFP and DFX) or DFO-DFP combination therapy (Table 4). Interestingly, there were no significant differences in numbers, gender, age, height, body weight, liver span and number of blood transfusion among these three groups.
Table 4 Demographic and therapeutic data of transfusion-dependent β-thalassemia patients (n = 35) according to study variables. Data are expressed as mean ± SD (range)
Information |
DI |
GTE-CUR (17.26 mg EGCG eq.) |
GTE-CUR (35.53 mg EGCG eq.) |
Abbreviations: B = β-thalassemia; BE = β-thalassemia HbE; BM = β-thalassemia major; BW = body weight; CUR = curcumin; DFO = desferrioxamine; DFP = deferiprone; DFX = deferasirox; EGCG eq. = epigallocatechin-3-gallate equivalent, F = female; GTE = green tea extract; Ht = height; M = male; NA = not available; T = thalassemia; Tx = blood transfusion. |
Gender |
3 M, 9 F |
4 M, 8 F |
5 M, 6 F |
Age (year) |
30.3 ± 12.9 (19–62) |
28.3 ± 7.1 (18–43) |
26.1 ± 6.9 (18–42) |
Type |
3 B, 7 BE, 2 BM |
5 B, 7 BE |
8 B, 3 BE |
Chelation |
1 DFO, 7 DFP, 3 DFO ± DFP |
2 DFO, 5 DFP, 3 DFX, 1 DFO ± DFP |
2 DFO, 5 DFP, 3 DFX, 1 DFO ± DFP |
Splenectomy |
8/12 |
9/12 |
10/11 |
Ht (cm) |
153.8 ± 6.4 (145–165) |
150.8 ± 6.1 (136–158) |
153.6 ± 9.7 (136–167) |
BW (kg) |
47.9 ± 6.0 (39–56) |
42.8 ± 5.9 (32–53) |
48.0 ± 6.5 (39–57) |
Liver span (cm) |
13.4 ± 1.8 (11–15) |
11.5 ± 2.0 (10–15) |
9.6 ± 2.8 (8–15) |
Tx (unit) |
1.29 ± 0.54 |
1.27 ± 0.47 |
1.38 ± 0.52 |
3.3. Hematological parameters
As shown in Tables 5 and 6, the GTE-CUR drink in both concentrations (17.3 and 35.5 mg EGCG equivalent) did not change the levels of RBC indices during the course of this study when compared with the control. The findings revealed that RBC numbers in the GTE-CUR (35.5 mg EGCG equivalent) treated groups tended to be decreased significantly whereas other red cell indices remain unchanged. Not surprisingly, the numbers of white blood cells (WBC) and platelets (PLT) were not found to be different between either group even though the white blood cells seemed to have increased in the GTE-CUR treated groups.
Table 5 Red blood cell parameters of transfusion-dependent β-thalassemia patients with or without GTE-CUR drink treatment for 2 months. Data are expressed as mean ± SD. *P < 0.05 when compared with M0
Parameter |
Time |
DI |
GTE-CUR (17.26 mg EGCG eq.) |
GTE-CUR (35.53 mg EGCG eq.) |
Abbreviations: CUR = curcumin, DI = deionized water, EGCG eq. = epigallocatechin-3-gallate equivalent, GTE = green tea extract, Hb = hemoglobin, Hct = hematocrit, MCH = mean corpuscular hemoglobin, MCHC = mean corpuscular hemoglobin concentration, MCV = mean corpuscular volume, RBC = red blood cell, RDW = red cell distribution width. |
Hb (g dL−1) |
M0 |
7.45 ± 1.35 |
8.49 ± 1.21 |
7.10 ± 1.09 |
M1 |
7.24 ± 0.94 |
8.00 ± 1.25 |
7.03 ± 1.16 |
M2 |
7.78 ± 1.12 |
7.67 ± 1.54 |
6.73 ± 0.86 |
|
Hct (%) |
M0 |
24.13 ± 5.58 |
25.7 ± 3.61 |
22.0 ± 3.66 |
M1 |
23.90 ± 2.98 |
24.3 ± 3.56 |
21.6 ± 4.10 |
M2 |
25.28 ± 3.72 |
22.9 ± 4.04 |
20.7 ± 2.71 |
|
RBC (cells per mm3) |
M0 |
3.13 ± 0.61 |
3.31 ± 0.41 |
3.01 ± 0.53 |
M1 |
3.05 ± 0.41 |
3.13 ± 0.42 |
4.94 ± 6.37 |
M2 |
3.21 ± 0.36 |
2.91 ± 0.51 |
2.68 ± 0.43* |
MCV (fL) |
M0 |
78.2 ± 6.4 |
76.6 ± 2.6 |
74.7 ± 4.5 |
M1 |
78.6 ± 5.0 |
77.3 ± 2.7 |
75.5 ± 4.1 |
M2 |
78.6 ± 5.7 |
78.2 ± 3.0 |
77.2 ± 5.2 |
|
MCH (pg) |
M0 |
24.0 ± 2.2 |
25.3 ± 1.3 |
24.1 ± 2.7 |
M1 |
23.9 ± 2.3 |
25.5 ± 1.6 |
24.8 ± 2.9 |
M2 |
24.3 ± 2.3 |
26.1 ± 1.5 |
25.2 ± 3.0 |
|
MCHC (%) |
M0 |
30.7 ± 2.4 |
33.1 ± 1.1 |
32.3 ± 1.9 |
M1 |
30.4 ± 2.6 |
33.0 ± 1.6 |
32.7 ± 2.5 |
M2 |
30.9 ± 2.1 |
33.4 ± 1.1 |
32.6 ± 2.1 |
|
RDW (%) |
M0 |
23.4 ± 6.2 |
22.1 ± 2.2 |
21.6 ± 4.0 |
M1 |
22.0 ± 5.8 |
21.6 ± 2.1 |
21.8 ± 4.0 |
M2 |
22.9 ± 5.3 |
20.6 ± 2.3 |
20.9 ± 4.8 |
Table 6 White blood cell and platelet parameters of transfusion-dependent β-thalassemia patients with or without GTE-CUR treatment for 2 months. Data are expressed as mean ± SD. *P < 0.05 when compared with M0
Parameter |
Time |
DI |
GTE-CUR (17.26 mg EGCG eq.) |
GTE-CUR (35.53 mg EGCG eq.) |
Abbreviations: CUR = curcumin, DI = deionized water, EGCG eq. = epigallocatechin-3-gallate equivalent, GTE = green tea extract, PLT = platelet, WBC = white blood cell. |
WBC (×103 cell per mm3) |
M0 |
9.48 ± 4.62 |
16.02 ± 6.03 |
17.44 ± 8.41 |
M1 |
10.03 ± 6.24 |
16.41 ± 7.06 |
15.83 ± 6.95 |
M2 |
9.99 ± 4.53 |
16.30 ± 6.15 |
16.90 ± 8.19 |
|
Neutrophil (%) |
M0 |
52 ± 8 |
54 ± 10 |
43 ± 10 |
M1 |
48 ± 13 |
54 ± 11 |
41 ± 12 |
M2 |
51 ± 18 |
49 ± 13 |
44 ± 12 |
|
Eosinophil (%) |
M0 |
8 ± 14 |
2 ± 2 |
3 ± 2 |
M1 |
2 ± 3 |
2 ± 1 |
3 ± 2 |
M2 |
2 ± 2 |
2 ± 1 |
3 ± 2 |
|
Basophil (%) |
M0 |
1 ± 2 |
5 ± 1 |
6 ± 2 |
M1 |
1 ± 2 |
5 ± 2 |
7 ± 2 |
M2 |
1 ± 3 |
6 ± 2 |
6 ± 2 |
|
Lymphocyte (%) |
M0 |
33 ± 16 |
37 ± 10 |
46 ± 11 |
M1 |
42 ± 9 |
37 ± 10 |
46 ± 12 |
M2 |
39 ± 15 |
41 ± 12 |
44 ± 13 |
|
Monocyte (%) |
M0 |
5 ± 4 |
2 ± 1 |
2 ± 1 |
M1 |
7 ± 4 |
2 ± 1 |
2 ± 1 |
M2 |
6 ± 5 |
2 ± 1 |
2 ± 1 |
|
PLT (×105 cells per mm3) |
M0 |
5.01 ± 2.03 |
6.21 ± 3.31 |
6.33 ± 2.36 |
M1 |
5.19 ± 2.52 |
6.64 ± 3.43 |
5.99 ± 2.38 |
M2 |
5.48 ± 2.54 |
6.72 ± 3.73 |
5.95 ± 2.40 |
The GTE-CUR drink only induced small changes in hematopoietic activity indicators during the course of this study when compared with the control. Notably, RBC numbers in the treated groups tended to decrease whereas other red cell indices remained unchanged, which likely affected erythropoiesis. Marouani and colleagues reported that polyphenol- and aluminum-rich green tea decoction significantly decreased body iron reserves and lowered the blood hemoglobin concentration in rats, possibly due to an interference with dietary iron absorption by green tea aluminum.33 A previous study reported on a toxic effect of curcumin at 100 mg per kg body weight on hematopoietic activity in rats with chemically induced hepatocarcinogenesis.34 Beneficially, curcumin improved anemia and extramedullary hematopoiesis in the livers and spleens of tumor-bearing mice, potentially improving the quality of life of human cancer patients.35
3.4. Blood biochemical parameters
Conclusively, the level of blood urea nitrogen decreased in a time-dependent manner in both GTE-CUR treated groups, whose value was significant at 35.5 mg EGCG equivalent (Fig. 1). Concomitantly, the GTE-CUR treatments seem to prevent increases in serum creatinine levels as was found in the control group. TDT patients in all three groups were positive for hypocholesterolemia, for which we found tentative prevention of increasing serum cholesterol levels only in the high-dose GTE-CUR group. Either or both of the major ingredients in the GTE-CUR drink would promote the uptake of plasma cholesterol via low-density-lipoprotein (LDL) receptor-mediated endocytosis and/or inhibit de novo synthesis of endogenous cholesterol synthesis in the liver. Our findings determine that the serum levels of ALP in the GTE-CUR treated groups (17.3 and 35.5 mg EGCG equivalent) were found to decrease (Δ = 1.8 ± 24.6 and −25.6 ± 22.6 U L−1, respectively) when compared with the DI treated group (Δ = 63.5 ± 50.2 U L−1). This result confirmed a degree of safety in drinking the GTE-CUR product containing 17.3 and 35.5 mg EGCG equivalent for 2 m. Nevertheless, the serum levels of AST, ALT, total protein, albumin, total bilirubin and direct bilirubin were not altered in the DI and GTE-CUR groups over the course of the 2 m study.
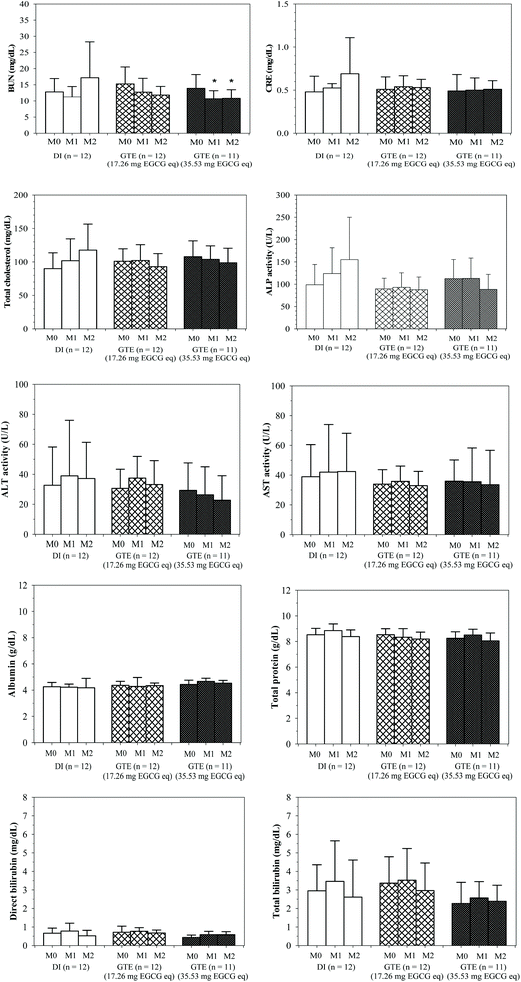 |
| Fig. 1 Levels of blood biochemical parameters of transfusion-dependent β-thalassemia patients with or without GTE-CUR drink treatment for 2 months. Data are expressed as mean ± SD. *P < 0.05 when compared with M0. Abbreviations: ALP = alkaline phosphatase, ALT = alanine aminotransferase, AST = aspartate aminotransferase, BUN = blood urea nitrogen, CRE = creatinine, DI = deionized water, EGCG eq = epigallocatechin-3-gallate equivalent, GTE = green tea extract, M = month. | |
Levels of BUN and serum creatinine in pediatric β-thalassemia patients were found not be different from those of the normal control; however, in contrast, their renal tubular function may be normal or impaired.36,37 In our study, as a consequence of potent diuretic activity, the GTE-CUR treatment lowered BUN levels and prevented increases in serum creatinine levels as was found in the control group. Coffee consumption (2.3 cups per d) is inversely related to serum uric acid concentration levels; however, it was not found to be dependent on the age, systolic blood pressure, serum creatinine, or serum lipid profile.38 Consistently, GTE was able to reduce the serum levels of uric acid in the healthy volunteer, possibly as a consequence of diuretic activity.39 A nutrient mixture containing GTE potentially prevented liver and kidney damage in acetaminophen-induced mice.40 Green tea could relieve antibiotic-induced nephrotoxicity and oxidative renal damage by improving the degree of antioxidant defense and integrity of the kidneys.41 Levels of BUN and plasma creatinine in experimental chronic-renal-failure rats were significantly reduced by curcumin via mechanisms of anti-inflammation, anti-oxidation and antagonistic activity of tumor necrotic factor-alpha (TNFα) elicited nuclear factor-kappa B (NFκB) activation.42
Liver oxidative damage and inflammation are usually found in thalassemia patients. Serum levels of ALP activity and other liver function markers in hepatitis C virus-infected β-thalassemia patients were not significantly changed after DFP treatment, whereas the ALP activity was found to have been significantly increased in β-thalassemia patients with chelation therapy due to bone resorption and hypofunction of bone metabolism.43–45 Serum levels of liver function biomarkers, except for ALP activity, were not altered in the GTE-CUR treated groups in our 2 m study. Importantly, a recent study has demonstrated a decrease in liver ALP in mice that have been treated with a tea-fortified alcoholic beverage due to the hepatoprotective mechanism that is associated with the endogenous anti-oxidation activity of tea.46 Green tea supplementation increased bone-specific ALP, leading to improvement in bone turnover and muscular strength, among postmenopausal women with osteopenia.47 Consumption of EGCG at 300 mg d−1 for 3 m did not cause any adverse effects on liver function in female volunteers.48 Consequently, this confirms the safety of drinking the GTE-CUR products containing 17.3 and 35.5 mg EGCG equivalent for 2 m.
Serum lipid profiles were significantly lowered in β-thalassemia patients when compared with healthy persons due to oxidative liver damage.49 Consistently, the TDT patients in all three groups of this study were positive for hypocholesterolemia, for which we found tentative prevention by increasing serum cholesterol levels only in the high-dose GTE-CUR group. Either or both of the major ingredients in the GTE-CUR drink would promote the uptake of plasma cholesterol via LDL receptor-mediated endocytosis and/or by inhibiting the de novo synthesis of endogenous cholesterol in the liver. Curcumin and green tea (particularly EGCG) were reported to be dose-dependent by increasing LDL receptor mRNA and upregulating sterol-regulatory element-binding protein gene expression in human hepatocellular carcinoma cells (HepG2).50–52
3.5. Iron overload and oxidative stress parameters
NTBI and LPI are detectable in β-thalassemia patients with iron overload and can be removed by iron chelators. LPI levels were almost unchanged by GTE-CUR (17.3 mg EGCG equivalent) treatment; however, these levels tended to decrease (Δ = −1.45 ± 2.84 and −1.63 ± 2.34 μM, respectively) by the GTE-CUR (35.5 mg EGCG equivalent) treatment after 1 and 2 m. Clearly, we found that serum NTBI levels were slightly changed (Δ = 0.13 ± 4.51 and −1.95 ± 8.28 μM, respectively) for the GTE-CUR (17.3 mg EGCG equivalent) treatment, and time-dependently decreased (Δ = −2.01 ± 5.86 and −3.93 ± 3.83 μM, respectively) by the GTE-CUR (35.5 mg EGCG equivalent) treatment (Fig. 2). Unexpectedly, the findings indicate that serum ferritin concentration levels tended to increase slightly in both the GTE-CUR treatment groups at month 1 and 2 for unknown reasons.
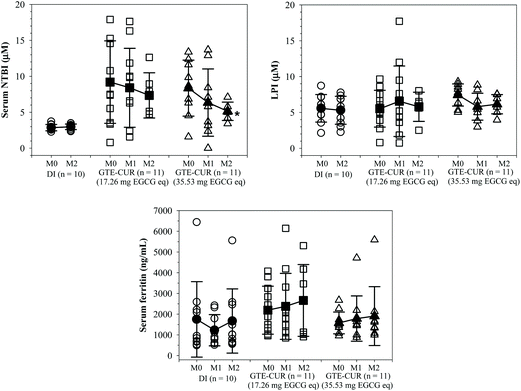 |
| Fig. 2 Levels of iron parameters in the serum of transfusion-dependent β-thalassemia patients with or without GTE-CUR drink treatment for 2 months. Data are shown as mean ± SD. *P < 0.05 when compared with M0. Abbreviations: EGCG eq = epigallocatechin-3-gallate equivalent, LPI = labile plasma iron, NTBI = non-transferrin bound iron. | |
Apparently, the levels of the serum lipid-peroxidation product TBARS tended to increase in members of the β-thalassemia patient control group (no GTE-CUR drink), whereas they were unchanged in the GTE-CUR groups throughout the course of 2 m. The findings of this study revealed that GTE-CUR treatments prevented an increase of serum TBARS levels in TDT patients. Nonetheless, serum antioxidant capacities over the course of several months were not different among members of these three groups (Fig. 3).
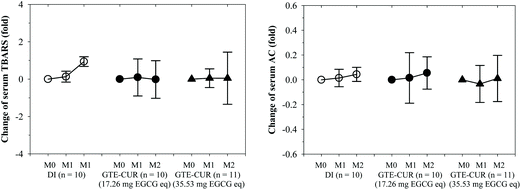 |
| Fig. 3 Change of thiobarbituric acid-reactive substance and antioxidant capacity levels in the serum of transfusion-dependent β-thalassemia patients with or without GTE-CUR drink treatment for 2 months. Data are expressed as mean ± SD. Abbreviations: AC = antioxidant capacity, TBARS = thiobarbituric acid-reactive substances. | |
We have demonstrated that GTE, particularly EGCG, improved iron overload in cells and animals by decreasing serum NTBI and LPI levels, and tissue iron concentrations.10,11,17 Curcumin also effectively decreased the levels of serum NTBI in thalassemia subjects with iron overload.53 Chelation therapy and antioxidant supplementation decreased serum ferritin, serum iron (SI) and total iron-binding capacity (TIBC) levels in β-thalassemia major patients when compared with the control.54–57 Rather than SI and TIBC, serum NTBI can be used as an alternative iron parameter as well as a form of redox-active iron to assess the efficiency of a treatment and it is positively correlated with serum lipid-peroxidation products.58–60 In this study, we found that GTE-CUR supplementation could decrease the levels of serum NTBI and LPI, and inhibit the increase of serum TBARS levels. However, it did not influence serum ferritin levels. Ferritin, which is an acute-phase protein and widely used as an iron overload parameter, is found to be highly increased in the serum of thalassemia patients with iron overload and among hepatitis patients. Levels of serum ferritin were not changed, before and during GTC-CUR supplementation in this study.
3.6. Serum catechin concentrations
As is shown in Table 7, the levels of all serum catechins were found to be independent of dose and consumption time; surprisingly, EGCG concentrations were the lowest (mostly undetectable) and EGC was not found in the serum of the GTE-CUR treatment groups. Apparently, the levels of serum phenolic acids were dose- and time-dependently increased during the course of the intervention.
Table 7 Levels of serum catechins of transfusion-dependent β-thalassemia patients treated with the GTE-CUR drink for 2 months. Data are shown as mean ± SD. Total catechin concentrations are defined as the sum of ECG, EGCG, C and EC values
Parameter |
Time |
GTE-CUR (17.26 mg EGCG eq.) |
GTE-CUR (35.53 mg EGCG eq.) |
Abbreviations: C = catechin, CG = catechin glucuronides, CUR = curcumin, EC = epicatechin, ECG = epicatechin-3-gallate, EGCG = epigallocatechin-3-gallate, EGCG eq. = epigallocatechin-3-gallate equivalent, GTE = green tea extract, TC = total catechins. |
CG (μg mL−1) |
M0 |
1077 ± 1089 |
1104 ± 532 |
M1 |
1609 ± 551 |
2305 ± 472 |
M2 |
1395 ± 669 |
1922 ± 499 |
|
ECG (μg mL−1) |
M0 |
75.8 ± 49.8 |
36.1 ± 50.6 |
M1 |
58.2 ± 47.1 |
63.3 ± 119.4 |
M2 |
75.2 ± 41.0 |
92.4 ± 67.3 |
|
EGCG (μg mL−1) |
M0 |
2.21 ± 0.11 |
ND |
M1 |
ND |
ND |
M2 |
ND |
ND |
|
C (μg mL−1) |
M0 |
ND |
2.68 ± 8.03 |
M1 |
8.25 ± 17.47 |
2.57 ± 8.54 |
M2 |
3.48 ± 9.84 |
9.06 ± 19.83 |
|
EC (μg mL−1) |
M0 |
0.19 ± 0.02 |
0.91 ± 2.73 |
M1 |
1.55 ± 1.96 |
0.97 ± 1.79 |
M2 |
2.93 ± 5.34 |
3.35 ± 2.76 |
|
TC (μg mL−1) |
M0 |
80.99 ± 52.11 |
72.19 ± 59.10 |
M1 |
66.62 ± 54.63 |
64.17 ± 117.42 |
M2 |
53.50 ± 53.81 |
85.36 ± 78.50 |
We believe that tea catechins are readily absorbed into blood circulation; however, free and conjugated forms of C, EC, ECG and EGCG were not detectable simultaneously in most of our serum samples. Possibly, the HPLC/UV method that we used for detecting these compounds displayed low limit of detection (LOD) and limit of quantification (LOQ) values (0.2–1.4 and 1.3–4.8 μg mL−1, respectively). Additionally, the method was not sensitive enough for this type of analysis.61 Without certain enzyme (e.g. β-glucuronidase and sulfatase) treatments, catechin conjugates could not be detected in the samples either. The outcome was in accordance with the fact that we measured the samples in the fasted state after 8–9 h of the subjects’ last meal and 22–24 h of their last consumption of the GTE-CUR product. For instance, HPLC/MS analysis of β-glucuronidase-treated plasma demonstrated that ECG and its conjugate (such as O-methyl-ECG) appeared in the plasma at the highest point 30 min after oral administration and disappeared by 6 h.62 Due to its short half-life in the plasma, EGCG tends to be degraded in aqueous solution of pH 7.0, but it could be stabilized by its potent antioxidant activity under physiological pH levels by forming non-covalent bonding to serum albumin sulfhydryl groups.63 In addition, galloyl moieties of EGCG and ECG are believed to be linked to the sulfhydryl groups, inferring their availability and activities in the plasma compartment.64 Daily administration of the EGCG-concentrated GTE-CUR drink could maintain its effective dose and potent activities in the plasma, although the elimination half-life of EGCG was approximately 3.4 h.65 The findings of human studies supported an increase of serum and urinary phenolic acids, for which catechins were included among male subjects who regularly drink green tea.66 A plasma kinetic study has shown that oral consumption of EGCG at up to 800 mg d−1 for 10 d was safe for healthy volunteers and beneficial for thalassemia patients as has been shown in this study.67 Furthermore, the solid-phase extraction technique together with high performance liquid chromatography/mass spectrometry analysis which is more sensitive than the HPLC/UV method will be accessible for the quantification of free catechins and their metabolites in serum and urine samples.68
4. Conclusions
An edible green tea–curcumin drink could decrease the levels of serum redox iron and lipid peroxidation product, along with blood urea nitrogen in iron overloaded β-thalassemia patients through relevant consecutive iron-chelating and diuretic effects (Fig. 4). Moreover, the drink did not influence any potential biochemical and hematological parameters.
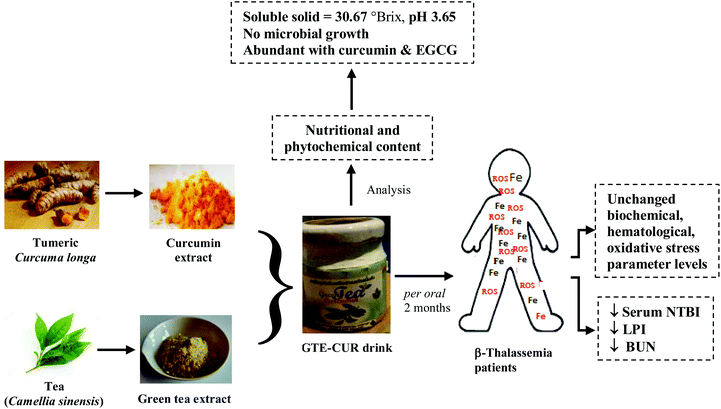 |
| Fig. 4 Graphical conclusion for restoration of iron-overloaded oxidative stress in β-thalassemia patients by the GTE-CUR drink. | |
Author contributions
SS conceived and designed the study and experiments, and contributed to the discussion; AT and SH designed and took the responsibility for the clinical study; NU produced the GTE-CUR drink; PK conducted and designed the experiments, analyzed the biomarker levels and the data, and wrote the manuscript; MC measured the catechin concentrations in the thalassemia serum; and SF and JP provided helpful comments and revised the manuscript. All authors have read and approved the manuscript.
Funding
This work was supported by the Royal Golden Jubilee Ph.D. Program (PHD0060/2554), Thailand Science Research and Innovation through Miss Pimpisid Koonyosying; National Research Council of Thailand; Medical Faculty Endowment Fund, Chiang Mai University through Associate Professor Somdet Srichairatanakool, PhD; and Chair Professor Grant through Professor Suthat Fucharoen, MD of the National Science and Technology Development Agency, Thailand.
Abbreviations
ABTS | 2,2′-Azino-bis(3-ethylbenzthiazoline-6-sulphonic acid) diammonium |
ALT | Alanine aminotransferase |
ALP | Alkaline phosphatase |
ANOVA | Analysis of variance |
AST | Aspartate aminotransferase |
BDMC | Bis-demethoxycurcumin |
BHT | Butylated hydroxytoluene |
BUN | Blood urea nitrogen |
C | Curcumin |
C
max
| Maximum concentration |
CG | Catechin glucuronide |
CRE | Creatinine |
CUR | Curcuminoids |
DFO | Desferrioxamine |
DFP | Deferiprone |
DFX | Deferasirox |
DHR | Dihydrorhodamine |
DMC | Demethoxycurcumin |
EC | Epicatechin |
ECG | Epicatechin-3-gallate |
EGC | Epigallocatechin |
EGCG | Epicatechin-3-gallate |
FI | Fluorescence intensity |
GC | Gallocatechin |
GTE | Green tea extract |
HDL | High density lipoprotein cholesterol |
HPLC/MS | High performance liquid chromatography/mass spectrometry |
HPLC/UV | High performance liquid chromatography/ultraviolet detection |
LDL | Low density lipoprotein-cholesterol |
LIP | Labile iron pools |
LPI | Labile plasma iron |
NFκB | Nuclear factor-kappa B |
NTA | Nitrilotriacetic acid |
NTBI | Non-transferrin bound iron |
PLT | Platelet |
RBC | Red blood cell |
ROS | Reactive oxygen species |
TBA | Thiobarbituric acid |
TBARS | Thiobarbituric acid reactive substances |
TC | Total catechins |
TCA | Trichloroacetic acid |
TDT | Transfusion-dependent β-thalassemia |
TEAC | Trolox equivalent antioxidant capacity |
TNFα | Tumor necrotic factor-alpha |
WBC | White blood cell |
Conflicts of interest
The authors report that they hold no conflicts of interest in this study.
Acknowledgements
This research was supported by the Royal Golden Jubilee Ph.D. Program (PHD0060/2554), Thailand Science Research and Innovation (formerly Thailand Research Fund) through Miss Pimpisid Koonyosying; National Research Council of Thailand; Medical Faculty Endowment Fund, Chiang Mai University; and Chair Professor Grant through Professor Suthat Fucharoen, MD of the National Science and Technology Development Agency, Thailand. We would like to express our sincere appreciation to the nurses and medical personnel at the Adult Thalassemia Clinic, Maharaj Nakorn Chiang Mai Hospital, Faculty of Medicine, Chiang Mai University for their effective patient management techniques. We thank Emeritus Professor Robert C. Hider, PhD, Institution of Pharmaceutical Sciences, King's College London, United Kingdom for his helpful comments and English proofreading.
References
- Y. Kohgo, K. Ikuta, T. Ohtake, Y. Torimoto and J. Kato, Int. J. Hematol., 2008, 88, 7–15 CrossRef CAS PubMed.
- B. Aldudak, A. Karabay Bayazit, A. Noyan, A. Ozel, A. Anarat, I. Sasmaz, Y. Kilinc, E. Gali, R. Anarat and N. Dikmen, Pediatr. Nephrol., 2000, 15, 109–112 CrossRef CAS PubMed.
- A. T. Taher, J. B. Porter, A. Kattamis, V. Viprakasit and M. D. Cappellini, Drug Des., Dev. Ther., 2016, 10, 4073–4078 CrossRef CAS PubMed.
- J. B. Porter, Blood Rev., 2009, 23(Suppl 1), S3–S7 CrossRef CAS PubMed.
- A. Eghbali, H. Kazemi, H. Taherahmadi, Y. Ghandi, M. Rafiei and B. Bagheri, Eur. J. Haematol., 2017, 99, 577–581 CrossRef CAS PubMed.
- M. Athanassiou-Metaxa, A. Kousi, E. S. Hatzipantelis, I. Tsatra, M. Ikonomou, V. Perifanis and H. Tsantali, Haematologica, 2004, 89, ELT07 Search PubMed.
- Y. Aydinok, A. Kattamis, M. D. Cappellini, A. El-Beshlawy, R. Origa, M. Elalfy, Y. Kilinc, S. Perrotta, Z. Karakas, V. Viprakasit, D. Habr, N. Constantinovici, J. Shen and J. B. Porter, Blood, 2015, 125, 3868–3877 CrossRef CAS PubMed.
- H. Cario, G. Janka-Schaub, G. Janssen, A. Jarisch, G. Strauss and E. Kohne, Klin. Padiatr., 2007, 219, 158–165 CrossRef CAS PubMed.
- E. Cassinerio, N. Orofino, A. Roghi, L. Duca, E. Poggiali, M. Fraquelli, L. Zanaboni and M. D. Cappellini, Blood Cells, Mol., Dis., 2014, 53, 164–167 CrossRef CAS PubMed.
- S. Ounjaijean, C. Thephinlap, U. Khansuwan, C. Phisalapong, S. Fucharoen, J. B. Porter and S. Srichairatanakool, Med. Chem., 2008, 4, 365–370 CrossRef CAS PubMed.
- C. Thephinlap, S. Ounjaijean, U. Khansuwan, S. Fucharoen, J. B. Porter and S. Srichairatanakool, Med. Chem., 2007, 3, 289–296 CrossRef CAS PubMed.
- S. Srichairatanakool, S. Ounjaijean, C. Thephinlap, U. Khansuwan, C. Phisalpong and S. Fucharoen, Hemoglobin, 2006, 30, 311–327 CrossRef CAS PubMed.
- G. N. Roth, A. Chandra and M. G. Nair, J. Nat. Prod., 1998, 61, 542–545 CrossRef CAS PubMed.
- R. W. Kalpravidh, N. Siritanaratkul, P. Insain, R. Charoensakdi, N. Panichkul, S. Hatairaktham, S. Srichairatanakool, C. Phisalaphong, E. Rachmilewitz and S. Fucharoen, Clin. Biochem., 2009, 43, 424–429 CrossRef PubMed.
- P. Koonyosying, S. Kongkarnka, C. Uthaipibull, S. Svasti, S. Fucharoen and S. Srichairatanakool, Biomed. Pharmacother., 2018, 108, 1694–1702 CrossRef CAS PubMed.
- P. Koonyosying, C. Uthaipibull, S. Fucharoen, E. V. Koumoutsea, J. B. Porter and S. Srichairatanakool, Pancreas, 2019, 48, 636–643 CrossRef CAS PubMed.
- T. Saewong, S. Ounjaijean, Y. Mundee, K. Pattanapanyasat, S. Fucharoen, J. B. Porter and S. Srichairatanakool, Med. Chem., 2010, 6, 57–64 CrossRef CAS PubMed.
- C. Thephinlap, C. Phisalaphong, S. Fucharoen, J. B. Porter and S. Srichairatanakool, Med. Chem., 2009, 5, 474–482 CrossRef CAS PubMed.
- C. Thephinlap, C. Phisalaphong, N. Lailerd, N. Chattipakorn, P. Winichagoon, J. Vadolas, S. Fucharoen, J. B. Porter and S. Srichairatanakool, Med. Chem., 2011, 7, 62–69 CrossRef CAS PubMed.
- P. Maisuthisakul, M. H. Gordon, R. Pongsawatmanit and M. Suttajit, Asia Pac. J. Clin. Nutr., 2007, 16, 37–42 CAS.
- P. Sirisa-Ard, N. Peerakam, S. Sutheeponhwiroj, T. Shimamura and S. Kiatkarun, J. Food Nutr. Res., 2017, 5, 48–53 CAS.
- W. Wichitnithad, N. Jongaroonngamsang, S. Pummangura and P. Rojsitthisak, Phytochem. Anal., 2009, 20, 314–319 CrossRef CAS PubMed.
- I. Trandafir, V. Nour and M. E. Ionica, Arch. Latinoam. Nutr., 2013, 63, 87–94 CAS.
- J. A. Bornhorst, J. W. Hunt, F. M. Urry and G. A. McMillin, Am. J. Clin. Pathol., 2005, 123, 578–583 CrossRef CAS PubMed.
- M. S. Wheal, E. DeCourcy-Ireland, J. R. Bogard, S. H. Thilsted and J. C. Stangoulis, Food Chem., 2016, 201, 222–229 CrossRef CAS PubMed.
- A. Meral, P. Tuncel, E. Surmen-Gur, R. Ozbek, E. Ozturk and U. Gunay, Pediatr. Hematol. Oncol., 2000, 17, 687–693 CrossRef CAS PubMed.
- I. Chakraborty, S. Mitra, R. Gachhui and M. Kar, Ann. Acad. Med. Singapore, 2010, 39, 13–16 Search PubMed.
- R. Re, N. Pellegrini, A. Proteggente, A. Pannala, M. Yang and C. Rice-Evans, Free Radical Biol. Med., 1999, 26, 1231–1237 CrossRef CAS PubMed.
- Y. Ma, M. Podinovskaia, P. J. Evans, G. Emma, U. E. Schaible, J. Porter and R. C. Hider, Biochem. J., 2014, 463, 351–362 CrossRef CAS PubMed.
- J. P. Lu and K. Hayashi, Pathol. Int., 1994, 44, 194–199 CrossRef CAS PubMed.
- Q. He, K. Yao, D. Jia, H. Fan, X. Liao and B. Shi, Nat. Prod. Res., 2009, 23, 93–100 CrossRef CAS PubMed.
- K. Narumi, J. Sonoda, K. Shiotani, M. Shigeru, M. Shibata, A. Kawachi, E. Tomishige, K. Sato and T. Motoya, J. Chromatogr. B: Anal. Technol. Biomed. Life Sci., 2013, 945–946, 147–153 Search PubMed.
- N. Marouani, A. Chahed, A. Hedhili and M. H. Hamdaoui, Eur. J. Nutr., 2007, 46, 453–459 CrossRef CAS PubMed.
- M. Sreepriya and G. Bali, Mol. Cell. Biochem., 2006, 284, 49–55 CrossRef CAS PubMed.
- Z. Fu, X. Chen, S. Guan, Y. Yan, H. Lin and Z. C. Hua, Oncotarget, 2015, 6, 19469–19482 Search PubMed.
- V. Smolkin, R. Halevy, C. Levin, M. Mines, W. Sakran, K. Ilia and A. Koren, Pediatr. Nephrol., 2008, 23, 1847–1851 CrossRef PubMed.
- H. M. Jafari, K. Vahidshahi, M. Kosaryan, H. Karami, M. Reza Mahdavi and S. Ehteshami, Bratisl. Lek. Listy, 2011, 112, 278–281 CAS.
- C. Kiyohara, S. Kono, S. Honjo, I. Todoroki, Y. Sakurai, M. Nishiwaki, H. Hamada, H. Nishikawa, H. Koga, S. Ogawa and K. Nakagawa, Br. J. Nutr., 1999, 82, 125–130 CrossRef CAS PubMed.
- K. Jatuworapruk, S. Srichairatanakool, S. Ounjaijean, N. Kasitanon, S. Wangkaew and W. Louthrenoo, J. Clin. Rheumatol., 2014, 20, 310–313 CrossRef PubMed.
- M. W. Roomi, T. Kalinovsky, V. Ivanov, M. Rath and A. Niedzwiecki, Hum. Exp. Toxicol., 2008, 27, 223–230 CrossRef CAS PubMed.
- E. A. Salem, N. A. Salem, M. Kamel, A. M. Maarouf, N. K. Bissada, W. J. Hellstrom and M. Eladl, Renal Failure, 2010, 32, 1210–1215 CrossRef CAS PubMed.
- S. S. Ghosh, H. D. Massey, R. Krieg, Z. A. Fazelbhoy, S. Ghosh, D. A. Sica, I. Fakhry and T. W. Gehr, Am. J. Physiol. Renal Physiol., 2009, 296, F1146–F1157 CrossRef CAS PubMed.
- A. C. Chen, C. T. Peng, S. F. Wu, K. H. Wu, I. P. Chiang and C. H. Tsai, Hemoglobin, 2006, 30, 209–214 CrossRef CAS PubMed.
- L. Pratelli, E. Verri, M. Fortini, S. Marconi, C. Zolezzi, P. M. Fornasari, M. R. Gamberini and V. De Sanctis, J. Pediatr. Endocrinol. Metab., 2006, 19, 1335–1342 CAS.
- N. K. Basha, B. Shetty and U. V. Shenoy, J. Clin. Diagn. Res., 2014, 8, 24–26 CrossRef PubMed.
- S. O. Ochanda, K. Rashid, J. K. Wanyoko, M. Ngotho, A. K. Faraj, C. A. Onyango, F. N. Wachira and D. N. Maranga, BMJ Open Gastroenterol, 2016, 3, e000058 CrossRef CAS PubMed.
- C. L. Shen, M. C. Chyu, J. K. Yeh, Y. Zhang, B. C. Pence, C. K. Felton, J. M. Brismee, B. H. Arjmandi, S. Doctolero and J. S. Wang, Osteoporosis Int., 2011, 23, 1541–1552 CrossRef PubMed.
- J. Mielgo-Ayuso, L. Barrenechea, P. Alcorta, E. Larrarte, J. Margareto and I. Labayen, Br. J. Nutr., 2013, 111, 1263–1271 CrossRef PubMed.
- L. M. Sherief, O. Dawood, A. Ali, H. S. Sherbiny, N. M. Kamal, M. Elshanshory, O. A. Alazez, M. A. Alhady, M. Nour and W. A. Mokhtar, BMC Pediatr., 2017, 17, 69 CrossRef PubMed.
- D. Peschel, R. Koerting and N. Nass, J. Nutr. Biochem., 2007, 18, 113–119 CrossRef CAS PubMed.
- C. Bursill, P. D. Roach, C. D. Bottema and S. Pal, J. Agric. Food Chem., 2001, 49, 5639–5645 CrossRef CAS PubMed.
- C. A. Bursill and P. D. Roach, J. Agric. Food Chem., 2006, 54, 1621–1626 CrossRef CAS PubMed.
- E. Mohammadi, A. Tamaddoni, D. Qujeq, E. Nasseri, F. Zayeri, H. Zand, M. Gholami and S. M. Mir, Phytother. Res., 2018, 32, 1828–1835 CrossRef CAS PubMed.
- P. Mazza, B. Amurri, G. Lazzari, C. Masi, G. Palazzo, M. A. Spartera, R. Giua, A. M. Sebastio, V. Suma, S. De Marco, F. Semeraro and R. Moscogiuri, Haematologica, 1998, 83, 496–501 CAS.
- B. Moayedi, M. Gharagozloo, N. Esmaeil, M. R. Maracy, H. Hoorfar and M. Jalaeikar, Eur. J. Haematol., 2013, 90, 202–209 CrossRef CAS PubMed.
- A. A. Hagag, M. A. Hamam, O. A. Taha and S. M. Hazaa, Infect. Disord.: Drug Targets, 2015, 15, 98–105 CAS.
- S. S. Bhagat, P. D. Sarkar, A. N. Suryakar, R. K. Padalkar, R. A. Ghone, S. M. Patil and P. S. Hundekar, Indian J. Physiol. Pharmacol., 2014, 57, 189–194 Search PubMed.
- F. N. al-Refaie, D. G. Wickens, B. Wonke, G. J. Kontoghiorghes and A. V. Hoffbrand, Br. J. Haematol., 1992, 82, 431–436 CrossRef CAS PubMed.
- A. Araujo, M. Kosaryan, A. MacDowell, D. Wickens, S. Puri, B. Wonke and A. V. Hoffbrand, Br. J. Haematol., 1996, 93, 835–837 CrossRef CAS PubMed.
- G. Cighetti, L. Duca, L. Bortone, S. Sala, I. Nava, G. Fiorelli and M. D. Cappellini, Eur. J. Clin. Invest., 2002, 32(Suppl 1), 55–60 CrossRef CAS PubMed.
- P. L. Fernández, M. J. Martín, A. G. González and F. Pablos, Analyst, 2000, 125, 421–425 RSC.
- T. Kohri, M. Suzuki and F. Nanjo, J. Agric. Food Chem., 2003, 51, 5561–5566 CrossRef CAS PubMed.
- M. J. Bae, T. Ishii, K. Minoda, Y. Kawada, T. Ichikawa, T. Mori, M. Kamihira and T. Nakayama, Mol. Nutr. Food Res., 2009, 53, 709–715 CrossRef CAS PubMed.
- K. Minoda, T. Ichikawa, T. Katsumata, K. Onobori, T. Mori, Y. Suzuki, T. Ishii and T. Nakayama, J. Nutr. Sci. Vitaminol., 2011, 56, 331–334 CrossRef PubMed.
- M. J. Lee, P. Maliakal, L. Chen, X. Meng, F. Y. Bondoc, S. Prabhu, G. Lambert, S. Mohr and C. S. Yang, Cancer Epidemiol. Biomarkers Prev., 2002, 11, 1025–1032 CAS.
- S. M. Henning, P. Wang, N. Abgaryan, R. Vicinanza, D. M. de Oliveira, Y. Zhang, R. P. Lee, C. L. Carpenter, W. J. Aronson and D. Heber, Mol. Nutr. Food Res., 2013, 57, 483–493 CrossRef CAS PubMed.
- U. Ullmann, J. Haller, J. D. Decourt, J. Girault, V. Spitzer and P. Weber, Int. J. Vitam. Nutr. Res., 2004, 74, 269–278 CrossRef CAS PubMed.
- Y. B. Zhou, X. C. Wan, Y. Y. Shang, J. W. Hu, L. Shao, W. Chen and D. X. Li, J. Agric. Food Chem., 2012, 60, 1619–1627 CrossRef CAS PubMed.
Footnote |
† Electronic supplementary information (ESI) available. See DOI: 10.1039/c9fo02424g |
|
This journal is © The Royal Society of Chemistry 2020 |
Click here to see how this site uses Cookies. View our privacy policy here.