DOI:
10.1039/C9FO02622C
(Paper)
Food Funct., 2020,
11, 944-954
Effects of three cooking methods on content changes and absorption efficiencies of carotenoids in maize
Received
7th November 2019
, Accepted 5th January 2020
First published on 7th January 2020
Abstract
Maize is a staple source of certain carotenoids for the human diet, but food processing is an important factor affecting the carotenoid content and absorption. In this study, we investigated the content changes of carotenoids in maize under three cooking methods (boiling kernels, preparing porridge and preparing tortilla). Also, using the in vitro digestion model, we assessed the effects of the cooking methods on carotenoid absorption efficiencies (digestion stability, micellization efficiency and bioaccessibility). The results indicated that the carotenoid content obviously increased in the boiled kernels, but its carotenoid bioaccessibility was the lowest compared to that of porridge and tortilla. Tortilla presented the highest digestion stability of β-carotene (309 ± 63%) and bioaccessibility of xanthophylls (22.4 ± 0.5% for lutein and 18.5 ± 1.0% for zeaxanthin) among the three cooked products during in vitro digestion. The contents of carotenoids in the porridge were all the lowest among the three cooked products; however, the low concentration level of xanthophylls reduced the competitive effect on β-carotene micellization, which increased the bioaccessibility of β-carotene to 52.1 ± 5.0%. Additionally, the content of xanthophylls (lutein + zeaxanthin) in digesta significantly and positively correlated with the β-carotene content in digesta, whereas it negatively correlated with the micellization of β-carotene. This correlation between the xanthophylls and β-carotene was not affected by the cooking methods. These results together suggest that tortilla and porridge are better dietary choices for the intake of xanthophylls and β-carotene, respectively, among maize-based foods. Furthermore, the absorption of β-carotene was influenced by the content of xanthophylls whatever the cooking method.
Introduction
Carotenoids, a group of isoprene derivatives found in a variety of fruits and crops, impart visible colors to these agricultural commodities.1 In some photosynthetic plant tissues, such as dark-green leafy vegetables, carotenoids are bound with proteins in the chloroplasts, while carotenoids can also accumulate in non-photosynthetic plant tissues, such as fruits, roots and seeds. Carotenoids are considered to provide protection against several chronic diseases, including cancer, cardiovascular disorder and impaired vision.2,3 Zeaxanthin and its isomer lutein are the dietary carotenoids actively absorbed and accumulated in the human macula.4 Additionally, according to the epidemiologic studies, these two carotenoids are preferentially accumulated in the human brain and benefit in maintaining cognitive health.5 β-Carotene, exhibiting provitamin A (PVA) activity, is an important nutrient required to prevent vitamin A deficiency (VAD). VAD is a leading cause of blindness and early childhood deaths in low-income countries.6
The bioaccessibility of carotenoids is governed by the characteristics of the food matrix, and the efficiency of physical, enzymic and chemical digestion.7 Several studies have established that bioaccessibility is correlated with bioavailability in humans.8,9 Digestion stability can directly present the retention of carotenoids after digestion. Micellization efficiency is defined as the percentage of carotenoids transferred to mixed micelles from the crude digesta, and often used as a measure of bioaccessibility. In recent years, an in vitro digestion model has been developed and modified for assessing food bioaccessibility, instead of animal and human models. The in vitro method, simulating digestion processes, attempts to mimic physiological and physical–chemical conditions. It typically includes the oral, gastric and small intestinal phases, and large intestinal fermentation occasionally.10 Although the absorption efficiencies of phytochemicals under the in vitro model cannot fully explain the bioavailability observed in in vivo situations,11–13 the in vitro methodology is widely used for studying the pre-absorptive processes of carotenoids.9,14,15
The carotenoids in maize are present at substantial levels in amyloplasts which are specialized for storing starch granules.16 Generally, common varieties of yellow maize contain high amounts of lutein and zeaxanthin.17 Increasing the pro-VA carotenoids, such as β-carotene, is also the primary goal of selective breeding. The absorption of carotenoids depends on the dietary constituents and their food particle size.18 Preparing porridge, boiling and preparing tortilla (nixtamalization) are the widely used cooking methods, and the usage of these cooking techniques depends on the geographical localization and the different dietary cultures. Boiling corn is the most common method in China and the Mediterranean, and can increase the overall carotenoid content by 36% in the cooked products.19 Maize porridge is the most popular dietary mode all over the world; the final carotenoid content in the porridge is determined by a variety of cooking parameters (temperature, time and pH).20 Nixtamalization is a traditional cooking method in Mexico and Central America, and is suitable for maize snacks, such as table tortillas or tortilla chips.21,22
This study attempted to investigate the content changes of maize carotenoids in different cooked products (boiled kernels, porridge and tortilla). The effects of different cooking methods on the carotenoid absorption efficiency were also studied by using the in vitro digestion model. Additionally, we analyzed the correlation between the content of xanthophylls and β-carotene in the three cooked products during micellization.
Materials and methods
Plant material
The maize, line Tianta 5, is a commercial cultivar with extreme salt tolerance and its salt-tolerant ability is much better than its parent inbred lines: salt-tolerant HOO-108 and salt-sensitive 825. The plants were grown during the growing season in 2018 at the experimental garden of Tianjin University, located at Nankai District, Tianjin (117°10′ 18.98′′ E; 39°06′ 33.02′′ N). The experimental samples were harvested in September, and then stored at −20 °C until the analysis.
Chemicals and standards
HPLC grade acetone, methanol, methyl tert-butyl ether and all analytical grade reagents (petroleum ether, acetone, and methanol) were procured from Jiangtian Chemical (Tianjin, China). α-Amylase (EC 3.2.1.1), porcine pepsin (EC 3.4.23.1), cholesterol esterase (EC 3.1.1.13), porcine pancreatin (EC 232-468-9), porcine bile extract (B8631), analytical standards of β-apo-8′-carotenal (CAS 1107-26-2), lutein (CAS 127-40-2), zeaxanthin (CAS 144-68-3), and β-carotene (CAS 7235-40-7) and all other chemicals were procured from Dingguo Chemical Co. (Beijing, China).
Preparation of boiled kernels
For boiling treatment, 2 g maize kernels and 10 mL distilled water were added into a 50 mL conical sterile tube. According to the Chinese traditional cooking method, the sample was boiled for 15 min at 95 °C. Then the cooled boiled kernels were dried with filter paper and immediately evaluated for carotenoid contents. The in vitro digestion experiments were performed simultaneously. Each preparation process was performed in triplicate.
Preparation of maize porridge
According to an earlier protocol23 with several modifications, 2 g milled maize flour and 10 mL distilled water were added into a 50 mL conical sterile tube. The sample was vortexed adequately and boiled for 15 min at 95 °C. The mixture was continuously stirred by a glass rod during the heating process. Then the cooled porridge was immediately evaluated for carotenoid contents, and the in vitro digestion experiments were performed simultaneously. Each preparation process was performed in triplicate.
Preparation of tortillas
According to an earlier protocol21 with several modifications, 2 g maize kernels were weighed into a 50 mL conical sterile tube, and 10 mL distilled water and 35 mg calcium hydroxide were added into the tube. The sample was boiled for 5 min at 95 °C and then steeped for 16 h at room temperature in the dark. The lime-cooked kernels were washed to rinse the residual pericarp and calcium hydroxide residues. After that, the washed sample was roughly and manually ground in a mortar, and then dried at 50 °C for 48 h in a dark drying oven. The resulting sample was thoroughly milled using a commercial mill. Water (1.2 mL/2 g) was gradually added into the nixtamalized flour to form a dough, and the dough was pressed into a flat disc with 1 mm thickness and 4 cm diameter. The disc was baked on a hot griddle (200–210 °C) for 10 s on one side, turned over and heated for 15 s on the other side and an additional 5 s on the initial side. Finally, the carotenoid contents of the tortilla were measured, and the in vitro digestion experiments were performed simultaneously. Each preparation process was performed in triplicate.
In vitro digestion model
The in vitro digestion procedure was adopted according to the standardized COST Infogest protocol24 with several modifications. 1.25× concentrated digestion electrolyte stock solutions were prepared according to the protocol proposed by Minekus et al. Simulated digestion fluids were used as follows: Simulated Salivary Fluid (SSF), Simulated Gastric Fluid (SGF) and Simulated Intestinal Fluid (SIF). Additionally, the corresponding enzymes, CaCl2, and water are added according to the detailed experiments below.
Oral phase of digestion
Because the liquid food is generally exposed to the oral environment in around 1–3 s,25 the porridge sample transferred to the gastric phase directly. Briefly, the prepared solid samples (tortillas or boiled kernels) were added into a conical sterile tube, and mixed with 3.5 mL of SSF electrolyte stock solution, 0.075 g α-amylase (75 U mL−1 in the final mixture) and 25 μL of CaCl2 solution (0.3 M). We put the tube in a 37 °C water bath and minced the sample quickly to simulate mastication in the human mouth. Finally, distilled water was added to a final volume of 10 mL, and then the tube was blanketed with nitrogen gas and incubated at 37 °C with continuous shaking (95 strokes per min for 10 min).
Gastric phase of digestion
The oral bolus or the porridge sample (10 mL) was mixed with 7.5 mL of SGF electrolyte stock solution, 1.328 g pepsin (2000 U mL−1 in the final digestion mixture) and 5 μL of CaCl2 solution (0.3 M). Then distilled water was added to a final volume of 20 mL and the pH was adjusted to 3 using 1 M HCl. The reaction vessel was blanketed with nitrogen gas and incubated at 37 °C with continuous shaking (95 strokes per min for 2 h). The pH of the reaction solution was readjusted manually during incubation.
Intestinal phase of digestion
According to a previous report,10 cholesterol esterase could hydrolyze xanthophylls (zeaxanthin and lutein), which was important to assess the bioaccessibilities of xanthophylls (zeaxanthin and lutein); thus this enzyme was added to the simulated intestinal phase. Briefly, 20 mL of gastric chyme was mixed with 11 mL of SIF electrolyte stock solution, 1 mL of cholesterol esterase solution (3.08 U mL−1), 1 g pancreatin (100 U mL−1 of trypsin in the final mixture), 0.275 g bile salt (10 mM in the final mixture) and 40 μL of CaCl2 solution (0.3 M). Distilled water was added to a final volume of 40 mL and the pH was adjusted to 7 with 1 M NaOH. The reaction vessel was blanketed with nitrogen gas and incubated at 37 °C with continuous shaking (95 strokes per min for 2 h). The pH of the reaction solution was readjusted manually during incubation.
Isolation of the micellar fraction
Based on a method described previously,23 the micelles were separated from the digesta phase by centrifuging at 20
000 rpm for 30 min at 4 °C. Then the aqueous fraction was transferred to a 50 mL syringe and filtered through a 0.22 μm filter. The collected aliquots were stored at −20 °C with a blanket of nitrogen and analyzed within 2–3 days.
Extraction of carotenoids from a raw maize sample and food products
The carotenoid extraction from a raw maize sample and food products were accomplished as described in an earlier report.23 Briefly, 2 g raw maize flour or food products were weighed into a 50 mL polypropylene centrifuge tube, and 20 mg ascorbic acid and 10 mL methanol were added into the tube. Then the solid food product, such as the boiled kernels or tortilla, was adequately ground. An additional 10 mL of 60% KOH solution was added to the mixture, and the tube was placed in a water bath maintained at 60 °C for 20 min. After the sample cooled down to room temperature, 100 μL β-apo-8′-carotenal (0.1 mg mL−1) and 10 mL of petroleum ether
:
acetone (2
:
1, v/v) with 0.1% BHT were added to the mixture, then vortexed (30 s) and centrifuged at 5000 rpm for 5 min. The upper organic phase was carefully transferred to another centrifuge tube. The extracts of raw maize and cooked products were completely colorless after repeating the process three times. Then the combined organics were dried under nitrogen. Finally, the dried extract was stored at −20 °C until further analysis.
Extraction of carotenoids from digesta and micellar fraction
Briefly, 10 mL of diethyl petroleum ether
:
acetone (2
:
1, v/v) with 0.1% BHT and the digestion phase (digesta or micellar fraction) with 100 μL β-apo-8′-carotenal (0.1 mg mL−1) were mixed in a polypropylene centrifuge tube. Then the sample was vortexed (3 min) and centrifuged at 5000 rpm for 5 min. The upper organic phase was carefully transferred to a new centrifuge tube. The extraction was repeated three times and the combined organics were dried under nitrogen. Finally, the dried extract was stored at −20 °C until further analysis.
Quantification and absorption efficiency of carotenoids
The content of carotenoids in different steps of the experiment (raw maize sample, cooked products, digesta and micellar fraction) was assessed as shown in Fig. 1A and B. The total carotenoid content was quantified using a spectrophotometer at 450 nm using the following formula26 (Abs = the absorbance at 450 nm, ε (specific absorption coefficient) = 2500, V = total volume of the sample (mL), W = the weight of the sample (g), 104 is the conversion factor):
Total carotenoid content (μg g−1) = (Abs × V × 104)/ε × W |
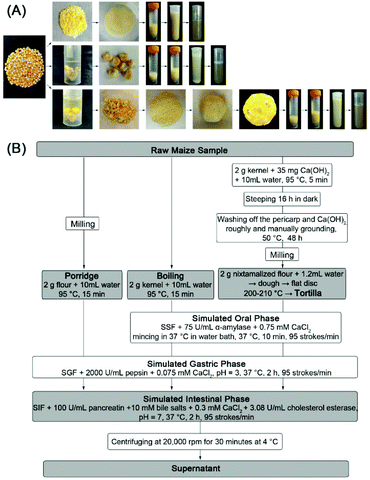 |
| Fig. 1 Flow chart of cooking processes and in vitro digestion (A); the contents of carotenoids in the grey boxes will be extracted (B). | |
The HPLC analysis of carotenoids was based on our previous study;27 the frozen extract was dissolved in 200 μL of HPLC grade acetone, and then filtered through a 0.22 μm filter. The final injection volume was 20 μL. The carotenoids were separated by using a C30 YMC column (250 × 4.6 mm, 5 μm; YMC Co., Japan). The mobile phase consisted of mixtures of methanol, methyl tert-butyl ether, and water as solvents A (81
:
15
:
4, v/v/v) and B (6
:
90
:
4, v/v/v). The gradient elution was performed under the following conditions: 100% A to 100% B in 90 min. The flow rate was 1 mL min−1, and the column temperature was set at 32 °C. The chromatogram was monitored at 450 nm, and the identification of carotenoids was based on the retention times of authentic standards. The content of carotenoids was quantified using external calibration curves, and the linear regression factor was higher than 0.9996. β-Apo-8′-carotenal was used as the internal standard, and the efficiency of extraction was used to calculate the final carotenoid concentration value. Each experiment was performed in triplicate.
The absorption efficiency of carotenoids was calculated, using the following formulas: digestion stability % = (the content in digesta/the content in cooking product) × 100%; micellization efficiency % = (the content in micellar fraction/the content in digesta) × 100%; and bioaccessibility % = (the content in micellar fraction/the content in cooking product) × 100%.
Statistical analysis
All analyses were performed using three biological and three technical replicates. The data were subjected to statistical analysis using IBM SPSS Statistics version 20.0. The mean difference was analyzed by one-way ANOVA followed by Duncan's test at p < 0.05. The results were expressed as means ± standard deviation. The linear regression analysis and Pearson's coefficients were calculated to determine the relationship between the β-carotene and xanthophylls. The content of xanthophylls in digesta was the independent variable, the β-carotene content in digesta and the β-carotene content in the micellar fraction were the dependent variables. The principal component analysis (PCA) and the cluster analysis of the carotenoid content were performed using MetaboAnalyst 4.0.28
Results and discussion
The carotenoid concentration in different cooked products of maize
The contents of total carotenoids in raw maize kernels and the three cooked products are shown in Fig. 2. The highest content of total carotenoids was found in boiled kernels (101 ± 4 μg g−1), and the contents of lutein and zeaxanthin also significantly increased (p < 0.05) after the boiling process compared with those in raw kernels (Table 1). It has been reported that the carotenoid content of maize increased after heat treatment.29 In other crops, the concentrations of β-carotene in spinach and pumpkin were also increased by boiling.30,31 Similarly, the contents of lutein and zeaxanthin in orange-fleshed sweet potato also increased after thermal treatment.32 Many authors considered that the increased content of carotenoids would be attributed to the improved solubility of carotenoids due to heat treatment.33 It was suggested that the carotenoids in the majority of vegetables would be increased after the boiling process, while the actual situation was determined by the type of carotenoid. In addition, it has been reported that the heat treatment might break down the cell walls, which further enhanced the release of carotenoids from the food matrix.34
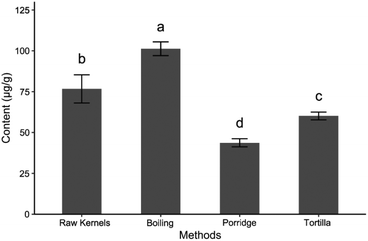 |
| Fig. 2 The total carotenoid contents in raw maize kernels, boiled kernels, porridge and tortilla. Error bars represent standard deviations (n = 3). Different letters in the columns represent statistically significant differences (p < 0.05). | |
Table 1 The contents of individual carotenoids in raw maize, boiled kernels, porridge and tortilla (μg g−1)a,b
|
Raw maize |
Boiling |
Porridge |
Tortilla |
Data are average ± standard deviation of triplicate analyses.
Different letters indicate a statistically significant difference (p < 0.05) among the maize samples for the same carotenoid.
|
Lutein |
61.5 ± 3.22b |
75.5 ± 8.11a |
15.3 ± 0.59d |
49.4 ± 3.07c |
Zeaxanthin |
7.99 ± 0.27b |
9.85 ± 1.50a |
2.05 ± 0.12c |
7.65 ± 0.63b |
β-Carotene |
2.26 ± 0.19a |
2.64 ± 0.77a |
0.45 ± 0.07b |
0.45 ± 0.05b |
The content of total carotenoids in porridge (43.7 ± 4.2 μg g−1) presented the lowest level among the three cooked products. Compared with the raw maize sample, the contents of three individual carotenoids in porridge all dramatically (p < 0.05) decreased with an average ratio of 76.5% (Table 1). This result was in contrast to the reports which illustrated that the contents of carotenoids maintained high retention after the porridge-making process.20,35 However, a previous study suggested that the porridge cooking method resulted in higher consumption of the total and individual carotenoids compared with the other cooking methods.36 Obviously, in this study, the only difference between the boiling and porridge cooking methods was the milling process (Fig. 1A and B). Consequently, we assumed that the dramatic decrease of the carotenoid content in porridge was due to oxidation, which easily occurred when the milled maize sample was exposed thoroughly to thermal air.
Tortilla retained 78.4% of the total carotenoid content of the raw sample (Fig. 2), which was consistent with a previous study reporting that the traditional tortilla retained 72.0–87.6% of the total carotenoid content of raw kernels.37 Similar to the result of porridge, the content of β-carotene in the tortilla dramatically decreased to 0.45 ± 0.05 μg g−1 (Table 1). The autoxidation of β-carotene occurred under an atmosphere of oxygen in the dark with an induction period of less than one hour. During the autoxidation, the twisting of the molecule during the isomerization process might lead to an unpaired spin state, which could react easily with oxygen to form a carbon-peroxyl triplet biradical.38 Then the products might go on to form endo-peroxides or react with a neutral β-carotene molecule, forming an epoxide and a carotene alkoxyl radical.38,39 In the tortilla preparing process, the autoxidation of β-carotene might occur during the drying process of the wet ground sample (Fig. 1A and B).
Overall, the cooking method had a large impact on the content of total and individual carotenoids. Although carotenoids were vulnerable to the high-temperature environment, the heat treatment was beneficial in increasing the carotenoid content through disrupting the food matrix (cell walls), facilitating their liberation in appropriate solvents.31,40 In this study, comparing with the three cooking methods (Fig. 1A and B), it was suggested that the milling process and its order in the cooking process played an important role in the retention of the carotenoids. It was obvious that the carotenoid contents in the intact kernel were much higher than those of the milled samples after the boiling process. The oxidation might be the main factor influencing the carotenoid losses, which was stimulated by light and heat. In addition, carotenoid oxidation also depended on available oxygen and the type of carotenoid.20 After the milling process, the exposed carotenoids, especially β-carotene, were more sensitive and vulnerable to heat treatment.
The content and absorption efficiency of carotenoids during in vitro digestion
The total carotenoid content of boiled kernels showed the largest decline from that of the cooked product (101 ± 4 μg g−1) to the micellar fraction (2.39 ± 0.20 μg g−1) (Fig. 3A). The micellization efficiency (6.66 ± 0.13%) and the bioaccessibility (2.36 ± 0.10%) of total carotenoids in the boiled kernels were the lowest among the three cooked products (Fig. 3C and D). Although the result of the in vitro method for partial carotenoids might not comprehensively predict the result of the in vivo experiment,12 the early studies have reported that the micellization efficiency and bioaccessibility measured by in vitro experiments were the positive predictors of carotenoid bioavailability.9,11 The in vitro method is widely used to assess the absorption efficiency of carotenoids in different food products.36,41 Additionally, a previous study reported that the micellization efficiency of carotenoids was positively correlated with the uptake of the carotenoids in Caco-2 cells.23 Comparing the three cooking methods, both porridge and tortilla underwent the milling step in their preparation processes. However, the boiling method maintained the intactness of the kernels until the simulated mastication process. Although the boiling method could prevent the carotenoids from oxidation during the cooking process, the incomplete mastication might negatively influence the absorption of carotenoids. The carotenoids were liberated from the food matrix during digestion (acidic conditions and enzymatic hydrolysis) in the stomach.42 Then the carotenoids were emulsified in lipid droplets which further develop into micelles in the intestinal lumen before absorption.43 The milling process could concentrate the desirable grain components and remove the poorly digested compounds.44 Moreover, dietary fiber could reduce the bioavailability of macronutrients and affected the absorption of carotenoids.45 They can enhance the viscosity of gastric fluids to restrict the peristaltic mixing process, which limits the transport of enzymes to their substrates.46 Dietary fiber also negatively influenced the soluble carotenoids being transported to the gut wall.47 Similar to most cereal grains, the fiber in maize was predominantly located in the hull (51%) and germ (16%) fractions.48 We assumed that the boiled kernels contain plenty of dietary fiber in digestion, which affects the absorption of carotenoids. This might be the reason why the cooking method with the milling process could increase the bioaccessibility of carotenoids.
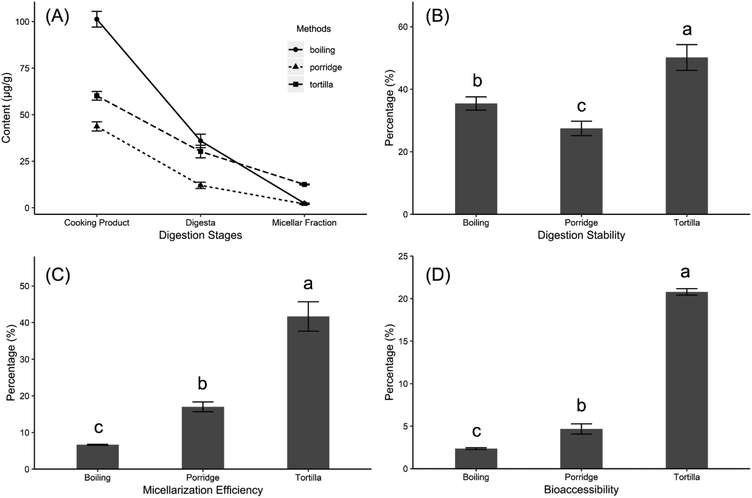 |
| Fig. 3 The content and absorption efficiency of total carotenoids among the three cooked products. The stability of the total carotenoid content of the three cooked products at different stages during digestion (A). The digestion stability (B), micellization efficiency (C) and bioaccessibility (D) of the total carotenoids in the three cooked products. Error bars represent standard deviations (n = 3). Different letters in the columns represent statistically significant differences (p < 0.05). | |
Additionally, in the digesta phase, the lowest concentrations of lutein (6.94 ± 0.59 μg g−1), zeaxanthin (0.84 ± 0.07 μg g−1) and β-carotene (0.47 ± 0.03 μg g−1) were all found in the porridge (Table 2). Interestingly, the β-carotene contents of porridge and tortilla in digesta were all higher than the contents before digestion, which directly caused the digestion stability of β-carotene of porridge and tortilla to reach 104 ± 11% and 309 ± 63%, respectively (Fig. 4A). The high retention of β-carotene in porridge was likely induced by the formation of small amounts of isomers, which could co-elute with β-carotene.36,41 Similarly, in tomato and red pepper, the concentrations of lycopene were also enhanced in digesta.49 Noteworthily, in this study, the digestion stability of β-carotene in tortilla reached 309%, which was about 3-fold higher than that of porridge (Fig. 4A). It had been reported that the bioavailability of β-carotene would be decreased by the pectin which mainly presented in the pericarp.50,51 During the tortilla preparing process, the kernels were washed to rinse off the residual pericarp after overnight steeping (Fig. 1B). This washing process removed the pericarp and the other poorly digested compounds (such as fibers), and effectively increased the digestive stability of β-carotene. On the other hand, the crucial part of carotenoid metabolism occurred in the small intestine, and the carotenoids need to be incorporated into the lipid fraction during digestion before being absorbed.52 Furthermore, dietary lipids were considered as the main factor for the formation of micelles,53,54 and cooking kernels with a suitable concentration of calcium hydroxide solution could retain abundant lipids.55 Therefore, the lime-cooking process might be an important reason why the digestion stability of β-carotene in tortilla could reach 309%.
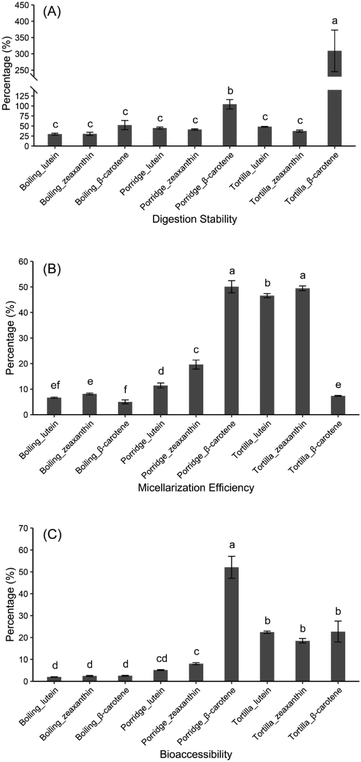 |
| Fig. 4 Digestion stability (A), micellization efficiency (B), and bioaccessibility (C) of three individual carotenoids in different cooked products, respectively. Error bars represent standard deviations (n = 3). Different letters in the columns represent statistically significant differences (p < 0.05). | |
Table 2 The individual carotenoid contents of the three cooked products in the digesta and micellar fractiona,b
|
Carotenoids in digesta (μg g−1) |
Carotenoids in micellar fraction (μg g−1) |
Boiling |
Porridge |
Tortilla |
Boiling |
Porridge |
Tortilla |
Data are average ± standard deviation of triplicate analyses.
Different letters indicate a statistically significant difference (p < 0.05) among the three different cooking products for the same carotenoid in their digestive phase.
|
Lutein |
22.4 ± 1.38a |
6.94 ± 0.59b |
23.8 ± 1.72a |
1.49 ± 0.13b |
0.79 ± 0.01b |
11.1 ± 0.65a |
Zeaxanthin |
2.94 ± 0.09a |
0.84 ± 0.07b |
2.86 ± 0.19a |
0.24 ± 0.02b |
0.16 ± 0.00b |
1.41 ± 0.07a |
β-Carotene |
1.32 ± 0.12a |
0.47 ± 0.03b |
1.36 ± 0.18a |
0.07 ± 0.01c |
0.23 ± 0.01a |
0.10 ± 0.01b |
Although the carotenoid contents of the three cooked products dramatically decreased during micellization (Table 2), the micellization efficiencies of lutein and zeaxanthin in tortilla stayed at high levels, which were, respectively, 46.5 ± 0.8% and 49.5 ± 1.0% (Fig. 4B). Additionally, in the boiled kernels and tortilla, the micellization efficiencies of the carotenoids were in the order of zeaxanthin > lutein > β-carotene (Fig. 4B), and this result was consistent with an earlier conclusion which suggested that xanthophylls had a higher micellization efficiency level than carotenes.17,23,41 However, in this study, the micellization efficiency of β-carotene in the porridge (approximately 50.1%) was significantly higher (p < 0.05) than that of the other two methods, and even higher than the micellization efficiencies of xanthophylls in porridge (Fig. 4B). A previous study reported that the micellization efficiencies of carotenes in porridge were markedly higher than those of xanthophylls,36 which suggested that preparing porridge was a better cooking method for the intake of β-carotene.
Effects of xanthophyll content on the absorption of β-carotene
The principal component analysis (PCA) of the carotenoid contents in the three cooking methods (including the cooked products, digesta and micellar fraction) indicated that PC 1, explaining 92.7% of the total variance, separated the three cooking methods, respectively. PC 2, accounting for 7.1% of the total variance, separated tortilla from boiled products and porridge (Fig. 5A). The cluster analysis of the carotenoid contents revealed that all contents clustered into two major clusters. According to the heatmap, the concentrations of lutein, zeaxanthin and xanthophylls (lutein + zeaxanthin) in the digesta were in contrast to the β-carotene content in the micellar fraction (Fig. 5B). The published result reported that the type of carotenoid was the major factor affecting the transfer of carotenoids to the aqueous micellar fraction.56 According to a previous clinical study, oxygenated carotenoids (such as lutein and zeaxanthin) could interfere with the absorption of β-carotene.57 In this study, in the digesta phase, the content of xanthophylls (lutein + zeaxanthin) in boiled kernels and tortilla was almost 3-fold higher than the content in porridge. However, in the micellar fraction, the β-carotene contents of boiled kernels and tortilla were, respectively, 3.3-fold and 2.3-fold lower than the content of porridge (Table 2). It was proved that the absorption of β-carotene was correlated with the content of xanthophylls.
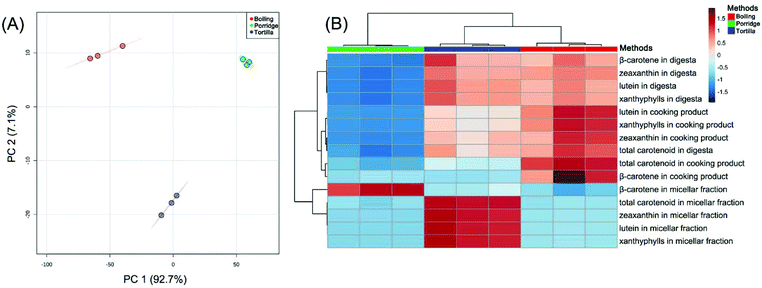 |
| Fig. 5 The principal component analysis of the contents of the total and individual carotenoids in three cooked products at all experimental stages (A). Three points of each colour are the three replicates. The cluster analysis of the contents of the total and individual carotenoids in the three cooked products at all experimental stages (B). Heatmap representation of the clustered data in which cells denote the glog (generalized logarithm transformation) values of the normalized concentration. The colour scale ranges from red (higher than the mean concentration) to blue (lower than the mean concentration). Three columns of each color are the three replicates. | |
Subsequently, the three cooking methods were analyzed simultaneously, and it was found that the correlation between the content of xanthophylls (lutein + zeaxanthin) and β-carotene was positive and significant (r = 0.994, p < 0.001; Fig. 6A) in the digesta phase. However, in the micellar fraction, the content of xanthophylls was slightly and negatively correlated with the content of β-carotene, but the correlation was statistically insignificant (r = −0.382, p = 0.55). Furthermore, β-carotene micellization was negatively and significantly correlated with the content of xanthophylls in digesta (r = −0.961, p < 0.001; Fig. 6B). This result was consistent with the previous study,23 and verified the conclusion that there was a competitive effect between xanthophylls and β-carotene during micellization.58 It has been reported that the micellization of carotenoids could be influenced by the polarity.59,60 In the gastrointestinal phase, carotenoids would be solubilized in the lipid emulsions which were a kind of phospholipid-triglyceride droplet, a triglyceride core surrounded by phospholipids.61 The solubility of apolar β-carotene in a pure triglyceride was much higher than that of polar xanthophylls, and it was easier for xanthophylls to reside at the surface of the droplets.61 Therefore, a significant percentage of xanthophylls was spontaneously transferred to the micellar fraction from the surface phase of the droplets. However, the carotenes that remained in the core part would transfer to the micellar fraction after triglyceride hydrolysis being catalyzed by pancreatic lipase.56,61 Therefore, in this study, the xanthophyll contents of boiled kernels and tortilla in digesta were much higher than the β-carotene content, which resulted in the absorption of β-carotene being restricted during the micellization process. However, in the digesta phase of porridge, the low concentration level of xanthophylls reduced the competitive effect on β-carotene micellization. Additionally, the correlations between xanthophylls and β-carotene were not influenced by the different cooking methods. This result suggested that the competition between xanthophylls and β-carotene during micellization was mainly affected by the type of carotenoid instead of the cooking methods.
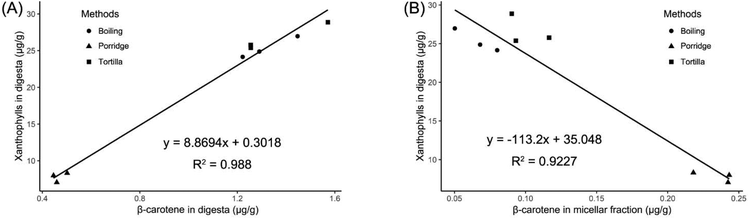 |
| Fig. 6 Linear regression analysis and Pearson's correlation of xanthophylls (lutein + zeaxanthin) and β-carotene. The regression analysis of the β-carotene content in digesta vs. xanthophyll content in digesta (r = 0.994, p < 0.001) (A). The regression analysis of the micellization of β-carotene vs. xanthophyll content in digesta (r = −0.961, p < 0.001) (B). The r and p values represent Pearson's correlation and significance, respectively. | |
Conclusions
In the present study, the effects of three different cooking methods (boiling kernels, preparing porridge and preparing tortilla) on the content changes of carotenoids in maize were investigated. The results indicated that the concentration of carotenoids in the intact kernels was much higher than that in the milled samples after the boiling process, and the oxidation might be the main factor influencing the carotenoid losses. Furthermore, through the in vitro digestion model, it was found that the absorption of β-carotene was negatively correlated with the xanthophyll content in digesta, and the competition between xanthophylls and β-carotene during micellization was mainly affected by the type of carotenoid instead of the cooking method. This is the first report in which different cooking methods are compared to investigate the content changes and absorption efficiencies of carotenoids. Future studies should focus on optimizing the cooking method to increase the absorption of carotenoids in humans.
Conflicts of interest
There are no conflicts to declare.
Acknowledgements
This work was supported by the Tianjin Science and Technology Research and Development Plan Project (19YFZCSN00280) and the Tianjin Rice Industry Technology System Innovation Team Construction (ITTRRS2018007).
References
- E.-S. M. Abdel-Aal, J. C. Young, I. Rabalski, P. Hucl and J. Fregeau-Reid, Identification and quantification of seed carotenoids in selected wheat species, J. Agric. Food Chem., 2007, 55, 787–794 CrossRef CAS PubMed.
- M. Shareck, M.-C. Rousseau, A. Koushik, J. Siemiatycki and M.-E. Parent, Inverse association between dietary intake of selected carotenoids and vitamin C and risk of lung cancer, Front. Oncol., 2017, 7, 23 Search PubMed.
- B. Kulczyński, A. Gramza-Michałowska, J. Kobus-Cisowska and D. Kmiecik, The role of carotenoids in the prevention and treatment of cardiovascular disease—current state of knowledge, J. Funct. Foods, 2017, 38, 45–65 CrossRef.
- N. P. Davies and A. B. Morland, Macular pigments: their characteristics and putative role, Prog. Retinal Eye Res., 2004, 23, 533–559 CrossRef CAS PubMed.
- E. J. Johnson, A possible role for lutein and zeaxanthin in cognitive function in the elderly, Am. J. Clin. Nutr., 2012, 96, 1161S–1165S CrossRef CAS PubMed.
- G. Giuliano, Provitamin A biofortification of crop plants: a gold rush with many miners, Curr. Opin. Biotechnol., 2017, 44, 169–180 CrossRef CAS PubMed.
- R. M. Faulks and S. Southon, Challenges to understanding and measuring carotenoid bioavailability, Biochim. Biophys. Acta, 2005, 1740, 95–100 CrossRef CAS PubMed.
- M. L. Failla, T.-Y. Huo and S. K. Thakkar, In vitro screening of relative bioaccessibility of carotenoids from foods, Asia Pac. J. Clin. Nutr., 2008, 17, 200–203 CAS.
- E. Reboul, M. Richelle, E. Perrot, C. Desmoulins-Malezet, V. Pirisi and P. Borel, Bioaccessibility of carotenoids and vitamin E from their main dietary sources, J. Agric. Food Chem., 2006, 54, 8749–8755 CrossRef CAS PubMed.
- R. Estévez-Santiago, B. Olmedilla-Alonso and I. Fernández-Jalao, Bioaccessibility of provitamin A carotenoids from fruits: application of a standardised static in vitro digestion method, Food Funct., 2016, 7, 1354–1366 RSC.
- F. Granado-Lorencio, C. Herrero-Barbudo, I. Blanco-Navarro, B. Pérez-Sacristán and B. Olmedilla-Alonso, Bioavailability of carotenoids and α-tocopherol from fruit juices in the presence of absorption modifiers: in vitro and in vivo assessment, Br. J. Nutr., 2008, 101, 576–582 CrossRef PubMed.
- F. Granado, B. Olmedilla, C. Herrero, B. Perez-Sacristan, I. Blanco and S. Blazquez, Bioavailability of carotenoids and tocopherols from broccoli: in vivo and in vitro assessment, Exp. Biol. Med., 2006, 231, 1733–1738 CrossRef CAS PubMed.
- A. G. Oomen, A. Hack, M. Minekus, E. Zeijdner, C. Cornelis, G. Schoeters, W. Verstraete, T. Van de Wiele, J. Wragg and C. J. Rompelberg, Comparison of five in vitro digestion models to study the bioaccessibility of soil contaminants, Environ. Sci. Technol., 2002, 36, 3326–3334 CrossRef CAS PubMed.
- F. Granado-Lorencio, B. Olmedilla-Alonso, C. Herrero-Barbudo, B. Pérez-Sacristán, I. Blanco-Navarro and S. Blázquez-García, Comparative in vitro bioaccessibility of carotenoids from relevant contributors to carotenoid intake, J. Agric. Food Chem., 2007, 55, 6387–6394 CrossRef CAS PubMed.
- F. Granado-Lorencio, B. Olmedilla-Alonso, C. Herrero-Barbudo, I. Blanco-Navarro, B. Pérez-Sacristán and S. Blázquez-García, In vitro bioaccessibility of carotenoids and tocopherols from fruits and vegetables, Food Chem., 2007, 102, 641–648 CrossRef CAS.
- C. A. Howitt and B. J. Pogson, Carotenoid accumulation and function in seeds and non-green tissues, Plant, Cell Environ., 2006, 29, 435–445 CrossRef CAS PubMed.
- S. K. Thakkar and M. L. Failla, Bioaccessibility of pro-vitamin A carotenoids is minimally affected by non pro-vitamin A xanthophylls in maize (Zea mays sp.), J. Agric. Food Chem., 2008, 56, 11441–11446 CrossRef CAS PubMed.
- J. Díaz-Gómez, R. M. Twyman, C. Zhu, G. Farré, J. C. Serrano, M. Portero-Otin, P. Muñoz, G. Sandmann, T. Capell and P. Christou, Biofortification of crops with nutrients: factors affecting utilization and storage, Curr. Opin. Biotechnol., 2017, 44, 115–123 CrossRef PubMed.
- R. Hoffman and M. Gerber, Food processing and the Mediterranean diet, Nutrients, 2015, 7, 7925–7964 CrossRef CAS PubMed.
- J. Díaz-Gómez, A. J. Ramos, C. Zhu, O. Martín-Belloso and R. Soliva-Fortuny, Influence of cooking conditions on carotenoid content and stability in porridges prepared from high-carotenoid maize, Plant Foods Hum. Nutr., 2017, 72, 113–119 CrossRef PubMed.
- N. Lozano-Alejo, G. V. Carrillo, K. Pixley and N. Palacios-Rojas, Physical properties and carotenoid content of maize kernels and its nixtamalized snacks, Innovative Food Sci. Emerging Technol., 2007, 8, 385–389 CrossRef CAS.
-
S. O. Serna-Saldivar and L. W. Rooney, in Tortillas, Elsevier, 2015, pp. 247–281 Search PubMed.
- N. Dube, P. C. Mashurabad, F. Hossain, R. Pullakhandam, L. Thingnganing and D. K. Bharatraj, β-Carotene bioaccessibility from biofortified maize (Zea mays) is related to its density and is negatively influenced by lutein and zeaxanthin, Food Funct., 2018, 9, 379–388 RSC.
- M. Minekus, M. Alminger, P. Alvito, S. Ballance, T. Bohn, C. Bourlieu, F. Carriere, R. Boutrou, M. Corredig and D. Dupont, A standardised static in vitro digestion method suitable for food–an international consensus, Food Funct., 2014, 5, 1113–1124 RSC.
- J. Chen, Food oral processing—A review, Food Hydrocolloids, 2009, 23, 1–25 CrossRef CAS.
-
J. Gross, Pigments in vegetables: chlorophylls and carotenoids, Springer Science & Business Media, 2012 Search PubMed.
- J. Chao, J. Jing, Q. Zhao, M. Ran, C. Guan and W. Gang, Characterization of lycopene β-cyclase gene from Lycium chinense conferring salt tolerance by increasing carotenoids synthesis and oxidative stress resistance in tobacco : new strategies in plant improvement, Mol. Breed., 2015, 35, 1–18 CrossRef.
- J. Chong, O. Soufan, C. Li, I. Caraus, S. Li, G. Bourque, D. S. Wishart and J. Xia, MetaboAnalyst 4.0: towards more transparent and integrative metabolomics analysis, Nucleic Acids Res., 2018, 46, W486–W494 CrossRef CAS PubMed.
- T. Muzhingi, K. J. Yeum, J. Qin and G. Tang, Determination of carotenoids in yellow maize, the effects of saponification and food preparations, Int. J. Vitam. Nutr. Res., 2008, 78, 112–120 CrossRef CAS PubMed.
- A. Azizah, K. Wee, O. Azizah and M. Azizah, Effect of boiling and stir frying on total phenolics, carotenoids and radical scavenging activity of pumpkin (Cucurbita moschato), Int. Food Res. J., 2009, 16, 45–51 CAS.
- A. Bunea, M. Andjelkovic, C. Socaciu, O. Bobis, M. Neacsu, R. Verhé and J. Van Camp, Total and individual carotenoids and phenolic acids content in fresh, refrigerated and processed spinach (Spinacia oleracea L.), Food Chem., 2008, 108, 649–656 CrossRef CAS PubMed.
- C. M. Donado-Pestana, J. M. Salgado, A. de Oliveira Rios, P. R. dos Santos and A. Jablonski, Stability of carotenoids, total phenolics and in vitro antioxidant capacity in the thermal processing of orange-fleshed sweet potato (Ipomoea batatas Lam.) cultivars grown in Brazil, Plant Foods Hum. Nutr., 2012, 67, 262–270 CrossRef CAS PubMed.
- E. Mayer-Miebach and W. Spiess, Influence of cold storage and blanching on the carotenoid content of Kintoki carrots, J. Food Eng., 2003, 56, 211–213 CrossRef.
- E. S. Hwang, M. Stacewicz-Sapuntzakis and P. E. Bowen, Effects of heat treatment on the carotenoid and tocopherol composition of tomato, J. Food Sci., 2012, 77, C1109–C1114 CrossRef CAS PubMed.
- L. Mugode, B. Ha, A. Kaunda, T. Sikombe, S. Phiri, R. Mutale, C. Davis, S. Tanumihardjo and F. F. De Moura, Carotenoid retention of biofortified provitamin A maize (Zea mays L.) after Zambian traditional methods of milling, cooking and storage, J. Agric. Food Chem., 2014, 62, 6317–6325 CrossRef CAS PubMed.
- E. G. Kean, B. R. Hamaker and M. G. Ferruzzi, Carotenoid bioaccessibility from whole grain and degermed maize meal products, J. Agric. Food Chem., 2008, 56, 9918–9926 CrossRef CAS PubMed.
- A. B. Corrales-Bañuelos, E. O. Cuevas-Rodríguez, J. A. Gutiérrez-Uribe, E. M. Milán-Noris, C. Reyes-Moreno, J. Milán-Carrillo and S. Mora-Rochín, Carotenoid composition and antioxidant activity of tortillas elaborated from pigmented maize landrace by traditional nixtamalization or lime cooking extrusion process, J. Cereal Sci., 2016, 69, 64–70 CrossRef.
- C. S. Boon, D. J. McClements, J. Weiss and E. A. Decker, Factors influencing the chemical stability of carotenoids in foods, Crit. Rev. Food Sci. Nutr., 2010, 50, 515–532 CrossRef CAS PubMed.
- R. C. Mordi, J. C. Walton, G. W. Burton, L. Hughes, I. U. Keith, L. A. David and M. J. Douglas, Oxidative degradation of β-carotene and β-apo-8′-carotenal, Tetrahedron, 1993, 49, 911–928 CrossRef CAS.
- G. Maiani, M. J. Periago-Castón, G. Catasta, E. Toti, I. G. Cambrodón, A. Bysted, F. Granado-Lorencio, B. Olmedilla-Alonso, P. Knuthsen and M. Valoti, Carotenoids: actual knowledge on food sources, intakes, stability and bioavailability and their protective role in humans, Mol. Nutr. Food Res., 2009, 53, S194–S218 CrossRef PubMed.
- E. G. Kean, N. Bordenave, G. Ejeta, B. R. Hamaker and M. G. Ferruzzi, Carotenoid bioaccessibility from whole grain and decorticated yellow endosperm sorghum porridge, J. Cereal Sci., 2011, 54, 450–459 CrossRef CAS.
- F. Khachik, L. Carvalho, P. S. Bernstein, G. J. Muir, D.-Y. Zhao and N. B. Katz, Chemistry, distribution, and metabolism of tomato carotenoids and their impact on human health, Exp. Biol. Med., 2002, 227, 845–851 CrossRef CAS PubMed.
- M. Alminger, A. M. Aura, T. Bohn, C. Dufour, S. El, A. Gomes, S. Karakaya, M. Martínez-Cuesta, G. McDougall and T. Requena, In vitro models for studying secondary plant metabolite digestion and bioaccessibility, Compr. Rev. Food Sci. Food Saf., 2014, 13, 413–436 CrossRef CAS.
- J. L. Slavin, D. Jacobs and L. Marquart, Grain processing and nutrition, Crit. Rev. Food Sci. Nutr., 2000, 40, 309–326 CrossRef CAS PubMed.
- H. Palafox-Carlos, J. F. Ayala-Zavala and G. A. González-Aguilar, The role of dietary fiber in the bioaccessibility and bioavailability of fruit and vegetable antioxidants, J. Food Sci., 2011, 76, R6–R15 CrossRef CAS PubMed.
- M. Viuda-Martos, R. Lucas-Gonzalez, C. Ballester-Costa, J. A. Perez-Alvarez, L. A. Muñoz and J. Fernández-López, Evaluation of protective effect of different dietary fibers on polyphenolic profile stability of maqui berry (Aristotelia chilensis (Molina) Stuntz) during in vitro gastrointestinal digestion, Food Funct., 2018, 9, 573–584 RSC.
- L. Montagne, J. Pluske and D. Hampson, A review of interactions between dietary fibre and the intestinal mucosa, and their consequences on digestive health in young non-ruminant animals, Anim. Feed Sci. Technol., 2003, 108, 95–117 CrossRef.
-
S. Watson, Structure and Composition in Corn Chemistry and Technology, ed. S. A. Watson and P. E. Ramstad, American Association of Cereal Chemists, St, Paul, MN, 1987 Search PubMed.
- L. Ryan, O. O′connell, L. O′sullivan, S. Aherne and N. O′brien, Micellarisation of carotenoids from raw and cooked vegetables, Plant Foods Hum. Nutr., 2008, 63, 127–133 CrossRef CAS PubMed.
- L. A. Bello-Perez, P. C. Flores-Silva, E. Agama-Acevedo, J. de Dios Figueroa-Cárdenas, J. A. Lopez-Valenzuela and O. H. Campanella, Effect of the nixtamalization with calcium carbonate on the indigestible carbohydrate content and starch digestibility of corn tortilla, J. Cereal Sci., 2014, 60, 421–425 CrossRef CAS.
- C. L. Rock and M. E. Swendseid, Plasma β-carotene response in humans after meals supplemented with dietary pectin, Am. J. Clin. Nutr., 1992, 55, 96–99 CrossRef CAS PubMed.
- L. Lemmens, I. Colle, S. Van Buggenhout, P. Palmero, A. Van Loey and M. Hendrickx, Carotenoid bioaccessibility in fruit-and vegetable-based food products as affected by product (micro) structural characteristics and the presence of lipids: A review, Trends Food Sci. Technol., 2014, 38, 125–135 CrossRef CAS.
- H. C. Furr and R. M. Clark, Intestinal absorption and tissue distribution of carotenoids, J. Nutr. Biochem., 1997, 8, 364–377 CrossRef CAS.
- A. J. Roodenburg, R. Leenen, K. H. van het Hof, J. A. Weststrate and L. B. Tijburg, Amount of fat in the diet affects bioavailability of lutein esters but not of α-carotene, β-carotene, and vitamin E in humans, Am. J. Clin. Nutr., 2000, 71, 1187–1193 CrossRef CAS PubMed.
- H. Martinez-Flores, M. Garnica-Romo, V. Romero and J. Yahuaca, Evaluating the quality of lipids during alkaline cooking of corn, J. Food Lipids, 2006, 13, 177–185 CrossRef CAS.
- V. Tyssandier, B. Lyan and P. Borel, Main factors governing the transfer of carotenoids from emulsion lipid droplets to micelles, Biochim. Biophys. Acta, 2001, 1533, 285–292 CrossRef CAS.
- D. Kostic, W. S. White and J. A. Olson, Intestinal absorption, serum clearance, and interactions between lutein and beta-carotene when administered to human adults in separate or combined oral doses, Am. J. Clin. Nutr., 1995, 62, 604–610 CrossRef CAS PubMed.
- H. van Den Berg, Carotenoid interactions, Nutr. Rev., 2010, 57, 1–10 Search PubMed.
- T. Huo, M. G. Ferruzzi, S. J. Schwartz and M. L. Failla, Impact of fatty acyl composition and quantity of triglycerides on bioaccessibility of dietary carotenoids, J. Agric. Food Chem., 2007, 55, 8950–8957 CrossRef CAS PubMed.
- P. C. Mashurabad, R. Palika, Y. W. Jyrwa, K. Bhaskarachary and R. Pullakhandam, Dietary fat composition, food matrix and relative polarity modulate the micellarization and intestinal uptake of carotenoids from vegetables and fruits, J. Food Sci. Technol., 2017, 54, 333–341 CrossRef CAS PubMed.
- P. Borel, P. Grolier, M. Armand, A. Partier, H. Lafont, D. Lairon and V. Azais-Braesco, Carotenoids in biological emulsions: solubility, surface-to-core distribution, and release from lipid droplets, J. Lipid Res., 1996, 37, 250–261 CAS.
|
This journal is © The Royal Society of Chemistry 2020 |
Click here to see how this site uses Cookies. View our privacy policy here.