DOI:
10.1039/C9RA10031H
(Paper)
RSC Adv., 2020,
10, 36212-36218
A biocompatible PAA-Cu-MOP hydrogel for wound healing†
Received
30th November 2019
, Accepted 12th September 2020
First published on 1st October 2020
Abstract
Wounds infected by bacteria are dangerous for human beings. However, along with the emergence of new strains and strong bacterial resistance, traditional antibiotics are unable to meet the medical needs for treating bacterial infections. Thus, new antibacterial substances with superior antimicrobial properties are urgently needed. Herein, a hydrogel containing poly acrylic acid (PAA), glycerol and Cu-MOP (named PAA-Cu-MOP hydrogel) is obtained by a facile mixing and ultrasonic procedure for wound healing treatment. This PAA-Cu-MOP hydrogel with high biocompatibility exhibits excellent wound healing behavior and is even better than the one of recombinant human epidermal growth factor. Tissue experiment results reveal that the PAA-Cu-MOP hydrogel accelerates the wound healing process by promoting angiogenesis, stimulating cell proliferation, and up-regulating cell factors.
1. Introduction
Full-thickness or partial skin defect wounds are one of the most common, complex and costly diseases.1–3 And combined with infection, especially for the most superficial wounds, treatment is difficult with the negative effect on cicatrization and high rates of complications. Bacteria-associated wound infection especially Pseudomonas aeruginosa (P.aeruginosa) and Staphylococcus aureus (S. aureus) can prolong the process of wound healing which will cause abscess formation and even death due to necrosis, sepsis and so on.4,5 Wound infections caused by bacteria not only bring pain to patients, but also impose significant burdens to the economy and healthcare systems with high morbidity and mortality.6,7 Conventional treatments for infected wounds use topical or systemic antibiotics. However, the abuse and misapplication of antibiotics have led to the enhancement of bacterial resistance, which seriously threatens human health. Therefore, it is an urgent need to develop other antibacterials with continuous long-term antibacterial activity and broad-spectrum antibacterial properties to avoid wound infection.
Metal–organic polyhedrons (MOPs) are a diverse class of metal–organic molecular assemblies with well-defined cavities which facilitate people to regulate and control their structures, functional groups and morphology by some chemical routes.8,9 Copper has been reported to possess anti-inflammatory, anti-microbial properties and could stimulate the processes of angiogenesis and collagen deposition that could accelerate wound healing.3,10–14 However, the excess of copper ions are considered to be cytotoxic that would pose a major challenge for clinical application.15–18 By means of abundant organic ligands, the chemical and physical properties of MOPs can be modulated for suitable to storage and release metal ions to eliminate cytotoxicity.3,19–21 In our previous work, we have reported a Cu-MOP hydrogel exhibits excellent antibacterial activity with favorable biocompatibility, non-toxicity and no irritation.22 However, the Cu-MOP hydrogel cannot maintain moist environment for a very long time, which is not benefit for the bio-medicine application, such as would healing.
Herein, a hydrogel composite incorporated PAA and glycerol into Cu-MOP (named PAA-Cu-MOP hydrogel) was developed to improve moist stability for the treatment of wound healing. Poly (acrylic acid) (PAA, named Carbomer) is an adhesive material with non-toxicity, higher water absorbency that has been used as biological barrier and drug carrier.23–27 Moreover, glycerol is usually been applied in the medical field, food industry and some other fields due to its excellent hygroscopics, antifreezes properties. The PAA-Cu-MOP hydrogel showed excellent antibacterial activity and broad antibacterial spectrum. Moreover, the PAA-Cu-MOP hydrogel accelerated the process of wound healing in full-thickness skin defect model, which was even better than the one of commercial drug, such as recombinant human epidermal growth factor.
2. Results and discussion
2.1. Synthesis and characterization of the PAA-Cu-MOP hydrogel
The synthesis procedure of PAA-Cu-MOP hydrogel was described in the Experimental section.22,28 As shown in Fig. 1A, after ultrasonic for 3–5 minutes and placement for 2–5 hours at room temperature, a gel-like sample was obtained. Scanning electronic microscopy images were taken to observe the morphology of the sample. As shown in Fig. 1B, it is obviously to see that the sample shows thin long strip morphology with the scale of 5 μm and fibrous morphology with the length of 2 μm and the diameter of 124 nm (Fig. S2A†). In addition, the Cu-MOP was homogeneously dispersed in the PAA-Cu-MOP hydrogel (Fig. S2B–F†). Then, the viscoelastic properties of the as-prepared hydrogel were then assessed using oscillatory rheology. As shown in Fig. 1C, the storage modulus (G′) of the sample was approximately an order of magnitude greater than the loss modulus (G′′), which was indicative of an elastic gel rather than a viscous material (Fig. 1C).29,30 Powder X-ray diffraction results indicated that the PAA-Cu-MOP hydrogel could maintain crystal structure (Fig. S1A†). For Fourier transform infrared spectroscopy (FTIR) (Fig. 1D), the peak of 3450 cm−1 in PAA-Cu-MOP hydrogel widened and showed a little right shifted compared to the Cu-MOP hydrogel, indicating the associated stretching vibration of –OH in glycerol and PAA. Moreover, the new peaks of 2950 cm−1 and 2900 cm−1 in PAA-Cu-MOP hydrogel could be ascribed to the stretching vibration of C–H (–CH2 and –CH) in glycerol and PAA. In addition, the new peaks of 1115 cm−1 and 1030 cm−1 in PAA-Cu-MOP hydrogel could also be ascribed to the stretching vibration and bending vibration of C–O and O–H in glycerol. Finally, the new peak of 1750 cm−1 in PAA-Cu-MOP hydrogel was from the stretching vibration of C
O in PAA.31,32 All these results showed that the addition of glycerol and PAA only mixed with the Cu-MOP hydrogel and did not change the structure of Cu-MOP hydrogel. Thermogravimetry Analysis (TGA) was used to determine the thermal stability of the PAA-Cu-MOP hydrogel. As shown in Fig. S1B,† it is obvious to see that the PAA-Cu-MOP hydrogel has a large mass loss at about 200 °C, indicating the good stability of the PAA-Cu-MOP hydrogel.
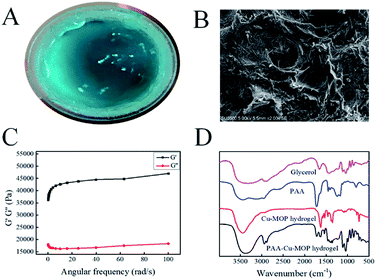 |
| Fig. 1 (A) The picture of our PAA-Cu-MOP hydrogel. (B) The SEM images of the PAA-Cu-MOP hydrogel in 5.00 μm scale. (C) Frequency sweeps in oscillatory rheometry of the PAA-Cu-MOP hydrogel. (D) The FTIR spectra of this PAA-Cu-MOP hydrogel. | |
2.2. Hygroscopicity and moisture retention of the PAA-Cu-MOP hydrogel
Hygroscopicity and moisture retention are two important factors in hydrogel for clinical medicine application.33–35 Previous study revealed that the Cu-MOP hydrogel was formed based on forming hydrogen bonds between Cu-MOPs and water molecules. Unfortunately, the ability of Cu-MOP hydrogel to maintain moist microenvironment was only for a very short time. Therefore, the PAA and glycerol were added to improve the hygroscopicity of Cu-MOP hydrogel. The hygroscopicity retention was evaluated in dry environment of silica gel and relative humidity (RH 43%, 81%) from 0 h to 312 h. In dry environment, the moisture ability of PAA-Cu-MOP hydrogel and Cu-MOP hydrogel were mainly similar from 0 h to 27 h, whereas the moisture ability of PAA-Cu-MOP hydrogel was better than the one of Cu-MOP hydrogel from 30 h to 312 h. The moisture ability of Cu-MOP hydrogel was close to zero after place them in the three conditions within 48 h (Fig. 2A). In addition, when these samples were treated with RH 43% and 81%, the glycerol showed strong hygroscopicity activity; whereas the PAA-glycerol hydrogel, PAA-Cu-MOP hydrogel and Cu-MOP hydrogel did not absorb water from ambient environment. From 0 to 35 hours, it was obvious to see that Cu-MOP hydrogel lost almost all water; however, PAA-Cu-MOP hydrogel only lost 42.3% water and could maintain a balance weight after 35 hours under RH 43% condition (Fig. 2B). Moreover, when RH was 81%, the hygroscopic tendency was similar to the one of RH 43% condition. In detail, all water molecules escaped from the Cu-MOP hydrogel within 52 hours; in the meantime, PAA-Cu-MOP hydrogel was able to sustain 64.5% water at 52 hours (Fig. S3†). These results indicated that the addition of PAA and glycerol in Cu-MOP hydrogel could improve its moisture retention ability which favored for prolonging the humidity.
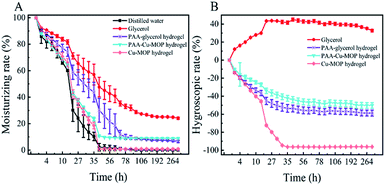 |
| Fig. 2 Hygroscopicity and moisture retention of the PAA-Cu-MOP hydrogel. (A) Dry environment of silica gel. (B) Relative humidity of 43%. | |
2.3. Antibacterial activity of the PAA-Cu-MOP hydrogel
Wound infection is mainly caused by bacteria,36–38 thus the antibacterial activity of the hydrogel should be evaluated before applying it for wound healing in vivo.39,40 The Gram-negative bacteria P. aeruginosa is an opportunistic pathogen which causes severe acute and chronic infection that delays wound healing efficiency and is generally difficult to treat.5,41 Herein, the antibacterial activities of two hydrogel were compared on P. aeruginosa by calculating the diameter of inhibition zone on nutrient agar plates from 18 h to 72 h. All the two hydrogels showed high antibacterial effect on P. aeruginosa, and the antibacterial activity of the PAA-Cu-MOP hydrogel was better than that of the Cu-MOP hydrogel and Cu2+ with the same concentration (Fig. 3A and S5A†). Obviously, Cu2+ alone showed a rapid reduce of antibacterial activity with the time, suggesting that pure Cu2+ was unsuitable for long term bacterial-infection wound treatment. Furthermore, Cu-MOP hydrogel released 5.528 μg ml−1 and 16.795 μg ml−1, PAA-Cu-MOP hydrogel released 4.238 μg ml−1 and 7.885 μg ml−1 copper ions after 2 days and 5 days (Fig. S4†). These results indicated that the incorporation of PAA and glycerol into Cu-MOP hydrogel maintained moisture more effectively and enabled the slow release of copper ion which prolonged the acting time, had no toxicity to animals and enhanced the antibacterial efficiency. Next, the details of time-dependent growth inhibitions for PAA-Cu-MOP hydrogel on P. aeruginosa and Bacillus subtilis (B. subtilis, Gram-positive bacteria) were investigated (Fig. S5B–D†). There is a manifest inhibition effect on P. aeruginosa (96%) and B. subtilis (93%) growth after interacting with PAA-Cu-MOP hydrogel for 50 min and 30 min, respectively. This phenomenon confirmed the conclusion of our previous work that a much faster antibacterial response for Gram-positive bacteria than Gram-negative bacteria, which was ascribed to the differences in the structure and composition of bacterial cell walls.22 Furthermore, the antimicrobial spectrum of PAA-Cu-MOP hydrogel was investigated by increasing the species of bacteria including Escherichia coli (E. coli, Gram-negative bacteria), S. aureus (Gram-positive bacteria) and Staphylococcus epidermidis (S. epidermidis, Gram-positive bacteria) (Fig. 3B, C, S6 and S7†). Obviously, after contacting with PAA-Cu-MOP hydrogel, the original smooth surface of E. coli and S. aureus has been changed and the structure collapsed compared to the native bacteria, which indicating the excellent antibacterial property of the PAA-Cu-MOP hydrogel. In addition, for E. coli, S. aureus and S. epidermidis, after 10 min, most of the bacteria have been killed. On the other hand, for B. subtilis and P. aeruginosa, antibacterial efficiency were 93% and 96% after 30 min and 50 min, respectively. All above results revealed that this PAA-Cu-MOP hydrogel showed a wide range of antibiosis scope and high antibacterial activity which was in favour of further applications.
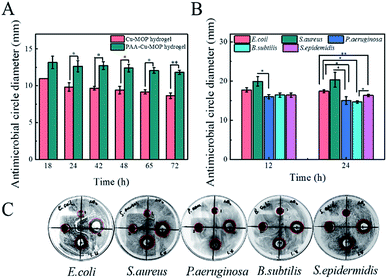 |
| Fig. 3 Antibacterial activity of PAA-Cu-MOP hydrogel. (A) The antibacterial effect of Cu-MOP hydrogel and PAA-Cu-MOP hydrogel on P. aeruginosa by calculating the diameter of inhibition zone on nutrient agar plates. (B) The antibacterial effect of PAA-Cu-MOP hydrogel on five different bacterial from 12 h to 24 h, n = 3, *P < 0.05, **P < 0.01. (C) The antibacterial circle of these five bacteria, n = 3, *P < 0.05, **P < 0.01. | |
2.4. Biocompatibility of the PAA-Cu-MOP hydrogel
The biocompatibility and bio-safety are two very important factors in bio-medicine applications.42–45 The biocompatibility of Cu-MOP hydrogel has been examined by MTT test, acute toxicity test and skin irritation test in our previous work.22 Consequently, the biological safety of PAA-Cu-MOP hydrogel was only assessed by haemolyticus test on human erythrocytes and tissue histology analysis.46 The hemolysis ratio of PAA-Cu-MOP hydrogel was 1.17% with the concentration of 33.4 mg ml−1, while that of Cu-MOP hydrogel, PAA, glycerol and copper irons also showed a lower hemolysis ratio (Fig. S8†). Meanwhile, the histological analysis of the major organs including heart, liver, kidney, lung and spleen of SD rat showed that there were not any abnormal effects and damages after the treatment with PAA-Cu-MOP hydrogel (Fig. S9†). These results demonstrated that PAA-Cu-MOP hydrogel had excellent biological safety and compatibility that was suitable for biomaterial in clinic application.
2.5. Wound healing effect in vivo
The wound healing ability of PAA-Cu-MOP hydrogel was evaluated by using a dorsal wound model of rat.19,47–49 The full-thickness round wound with areas of 1 cm2 was on the back of each Sprague-Dawley (SD) male rat. The traumas were photographed with increasing days post-operation to expressly research the wound healing activity of physiological saline (PS, negative control), recombinant human epidermal growth factor (RHEGF, positive control) and PAA-Cu-MOP hydrogel. As show in Fig. 4A and S10,† all the areas of wounds reduced along with time. For physiological saline and recombinant human epidermal growth factor, the wound healing ratio were 1.81%, 28.35%, 87.31% and 5.58%, 30.96%, 92.14%, respectively, after 3, 7, 14 days. However, the wound healing ratio for PAA-Cu-MOP hydrogel were 9.29%, 50.33% and 94.97% (Fig. S10†). The wound healing ratio of PAA-Cu-MOP hydrogel showed significantly accelerating wound healing ability especially on day 3 and day 7. In addition, the time for 50% wound healing ratio was 5 days for PAA-Cu-MOP hydrogel, but the PS and RHEGF for 50% wound area closure were 9 days and 10 days, respectively (Fig. 4A). For the wound healing, the recombinant human epidermal growth factor was accumulated which obstructed proliferation and differentiation of keratinocytes and fibroblast to slow down healing rate.50–52 Therefore, the recombinant human epidermal growth factor group performed similar to the physiological saline group from day 7 to day 9. The wound was fully recured after treatment with PAA-Cu-MOP hydrogel for 10 day, however the traumas treated by PS and RHEGF were only partly recovered. These results demonstrated that PAA-Cu-MOP hydrogel has excellent wound healing ability.
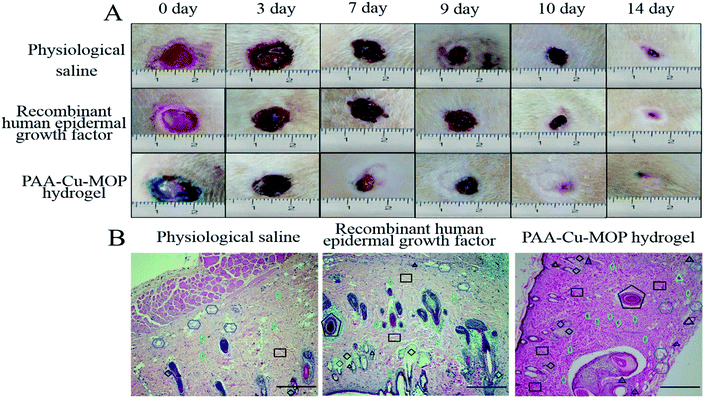 |
| Fig. 4 In vivo assessments of the PAA-Cu-MOP hydrogel for wound healing. (A) Digital images of wounds treated with physiological saline, recombinant human epidermal growth factor and PAA-Cu-MOP hydrogel. (B) Histological images of tissues stained with H&E after treating on day 14. Black triangle: blood vessel; green oval: fibroblast; black square: collagen fiber; black rhombus: hair follicle; purple hexagon: fat cell; black pentagon: sensory corpuscles (scale bar: 10 μm, magnification: 100×). | |
2.6. The mechanism of wound healing effect
The major steps in the process of wound healing are collagen deposition, re-epithelialization and granulation tissue formation.53–55 The higher degree of collagens in wounds, the better proliferated granulation of tissues, and the faster wounds healing efficiency.10,56,57 After operation, we can see that on day 7 the epithelial tissue of the negative control group begins to repair, and is in the early stage of repair. In the meantime, the positive control group is also in the repair stage, but its repair speed is faster than that of the negative control group. The PAA-Cu-MOP hydrogel treated experimental group is in the late stage of repair which shows the best repair efficiency (Fig. S11A†). Histomorphological determination on wound regeneration on day 14 was conducted by H&E staining (Fig. 4B and S11B†). After 14 days treatment, there are more fibroblasts and collagen fiber in wounds treated with PAA-Cu-MOP hydrogel than the ones of treated with PS and RHEGF. Histopathologic analysis confirmed that PAA-Cu-MOP hydrogel could contribute to significantly enhance wound healing. To gain insight into the accelerating wound healing mechanism of the PAA-Cu-MOP hydrogel, the microvessel density (MVD), expression of Ki-67 positive cells, and the expression level of relative mRNA including vascular endothelial growth factor (VEGH), transforming growth factor beta 1 (TGF-β1) were evaluated.
Angiogenesis is a particularly important phase in wound healing.58,59 Platelet endothelial cell adhesion molecule-1 (CD31) is used primarily to evaluate the degree of angiogenesis.60 The MVD significantly increased on day 7 and day 14 for wounds treated with RHEGF and PAA-Cu-MOP hydrogel (Fig. 5A). On day 7, CD31 immunostaining quantification results showed that MVD in wounds treated with PS, RHEGF and PAA-Cu-MOP hydrogel was 152.4 ± 17.1, 260.0 ± 14.9, 386.0 ± 18.6 microvessels per mm2, respectively. On day 14, the MVD in wounds treated with PS and RHEGF increased to 219.8 ± 15.8 and 329.6 ± 15.6 microvessels per mm2 to promote neoangiogenesis. The value of PAA-Cu-MOP hydrogel in MVD reduced which might be ascribed to wound closure (Fig. 6). The Ki-67 is a cellular marker for proliferation.53,61 The cell proliferation of tissues in wound was detected by the expression level of Ki-67 positive cells. It was found that the tissues in wound treated with RHEGF and PAA-Cu-MOP hydrogel express higher levels of Ki-67 positive cells than that of PS (Fig. 5B and S12†). These results indicated that PAA-Cu-MOP hydrogel displayed the best performer in promoting cell proliferation among three groups.
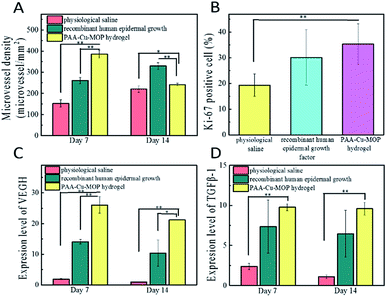 |
| Fig. 5 Analysis of granulation tissues on wounds at day 14 from SD rat. (A) Quantification of microvessel density observed. (B) Quantification of Ki-67 positive cells. (C) Expression level of VEGF and (D) expression level of TGF-β1 in wound healing process with different treatments, n = 5, *P < 0.05, **P < 0.01. | |
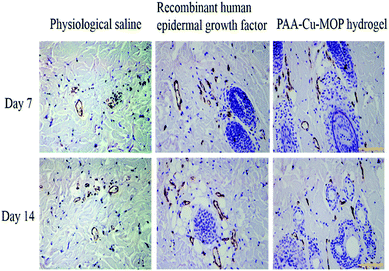 |
| Fig. 6 Immunohistological images of tissues on day 7 and day 14 from wound stained with CD31 (dark brown, endothelial cell positive; scale bar: 10 μm, magnification: 200×). | |
VEGH and TGF-β1 are important cell factors to promote cell proliferation, angiogenesis, and extracellular matrix (ECM) synthesis in wound healing process.61,62 VEGH adjusts multiple pathways to induce endothelial cell proliferation, promote cell proliferation, and create new blood vessels during wound healing.58,63 TGF-β1 is able to regulate cell growth and proliferation, especially promotes cell migration during early healing process.61 Determining these cell factors could explore the molecular mechanism of PAA-Cu-MOP hydrogel for wound healing. VEGH and TGF-β1 were obviously upregulated in the wound treated with PAA-Cu-MOP hydrogel compared to the wounds treated with PS and RHEGF. Furthermore, these two relative mRNA expressions decreased with time (Fig. 5C and D). The PAA-Cu-MOP hydrogel group showed 13.8 times, 1.84 times and 22.7 times, 2.1 times larger than the groups treated with PS, RHEGF for VEGF expression level on day 7 and day 14, respectively (Fig. 5C). Similar to VEGF, the expression level of TGF-β1 in PAA-Cu-MOP hydrogel group was 4.1 times, 1.3 times and 8.7 times, 1.5 times larger than PS group and RHEGF group on day 7 and day 14, respectively (Fig. 5D). These results were consistent with the results of the MVD and Ki-67 positive cells. These results demonstrated that the PAA-Cu-MOP hydrogel accelerated wound healing process by promoting angiogenesis, stimulating cell proliferation, and up-regulating cell factors, such as VEGF and TGF-β1. Due to the viscosity and elasticity of PAA-Cu-MOP hydrogel, the procoagulant ability of PAA-Cu-MOP hydrogel was evaluated by using mouse liver bleeding model.39,64 The PAA-Cu-MOP hydrogel showed excellent procoagulant ability that the bleeding position indicated rapid control after treating with the hydrogel. The bleeding position treated with PAA-Cu-MOP hydrogel stopped bleeding within 30 s. The stopped bleeding time of negative control (without any treatment), RHEGF and hydrogel matrix (PAA-glycerol hydrogel) were about 120 s, 90 s and 60 s, respectively. The amount blood loss for PAA-Cu-MOP hydrogel, negative control, RHEGF and hydrogel matrix at 30 s and 120 s were 24.73 ± 2.21 mg, 168.67 ± 8.95 mg, 75.52 ± 2.98 mg, 47.83 ± 12.95 mg, and 28.57 ± 1.07 mg, 323.50 ± 16.37 mg, 138.17 ± 2.24 mg, 70.20 ± 4.81 mg, respectively (Fig. S13†). The procoagulant ability of PAA-Cu-MOP hydrogel may come from two aspects. On the one hand, the PAA-Cu-MOP hydrogel formed a network barrier via its viscosity and elasticity to prevent bleeding from bleeding position. On the other hand, the copper ion had been shown to stimulate angiogenesis that contributed to the procoagulant capacity of liver. Thus, we have introduced PAA and glycerol into original Cu-MOP hydrogel to enhance its hygroscopicity and moisture retention activities which are beneficial for wound healing. This PAA-Cu-MOP hydrogel showed excellent antibacterial property and was nontoxic to cells that could be applied for treating bacterial infected wound and accelerating the healing process. PAA-Cu-MOP hydrogel could promote cells to produce more collagen deposition, re-epithelialization, granulation tissue, microvessel density and so on, which displayed better wound healing property than the one of recombinant human epidermal growth factor. Moreover, this PAA-Cu-MOP hydrogel also showed procoagulant effect which facilitated its further applications.
3. Conclusion
In summary, we have successfully prepared hydrogel directly from Cu-MOP, glycerol and PAA by a facile procedure. The PAA-Cu-MOP hydrogel with favorable moisture retention, antibacterial activity and procoagulant ability was used to accelerate the wound healing process in vivo without any toxicity. These important insights gained in our work will shed light on the design principles for efficient biomaterials for wound healing and also open a new avenue to the utilization of Cu-MOP for various biomedicine applications, such as emergency hemostasis.
Ethical statement
All experiments were performed in accordance with the Guidelines including care and use of laboratory animals and biomedical research involving human ethics of QuanZhou Medical College. Experiments were approved by the ethics committee at Quanzhou Medical College. Informed consents were obtained from human participants of this study.
Conflicts of interest
There are no conflicts to declare.
Acknowledgements
The research was supported by the National Natural Science Foundation of China (NSFC, 21765024) and Science and Technology Program of Quanzhou (2018N118S).
References
- P. S. Briquez, J. A. Hubbell and M. M. Martino, Adv. Wound Care, 2015, 4, 479 CrossRef
. - M. B. Dreifke, A. A. Jayasuriya and A. C. Jayasuriya, Mater. Sci. Eng., C, 2015, 48, 651 CrossRef CAS
. - J. Xiao, Y. Zhu, S. Huddleston, P. Li, B. Xiao, O. K. Farha and G. A. Ameer, ACS Nano, 2018, 12, 1023 CrossRef CAS
. - B. Hu, C. Owh, C. P. Lin, W. R. Leow, X. Liu, Y.-L. Wu, P. Guo, X. J. Loh and X. Chen, Chem. Soc. Rev., 2018, 47, 6917 RSC
. - E. A. Grice, H. H. Kong, C. Sean, C. B. Deming, D. Joie, A. C. Young, G. G. Bouffard, R. W. Blakesley, P. R. Murray and E. D. Green, Science, 2009, 324, 1190 CrossRef CAS
. - E. M. Hetrick and M. H. Schoenfisch, Chem. Soc. Rev., 2006, 35, 780 RSC
. - C. K. Sen, G. M. Gordillo, S. Roy, R. Kirsner, L. Lambert, T. K. Hunt, F. Gottrup, G. C. Gurtner and M. T. Longaker, Wound Repair Regen., 2010, 17, 763 CrossRef
. - H. Vardhan, M. Yusubov and F. Verpoort, Cheminform, 2016, 47, 171 CrossRef
. - B. Li, H. M. Wen, Y. Cui, W. Zhou, G. Qian and B. Chen, Adv. Mater., 2016, 28, 8819 CrossRef CAS
. - A. P. Kornblatt, V. G. Nicoletti and A. Travaglia, J. Inorg. Biochem., 2016, 161, 1 CrossRef CAS
. - A. Gopal, V. Kant, A. Gopalakrishnan, S. K. Tandan and D. Kumar, Eur. J. Pharmacol., 2014, 731, 8 CrossRef CAS
. - G. Borkow, J. Gabbay, R. Dardik, A. I. Eidelman, Y. Lavie, Y. Grunfeld, S. Ikher, M. Huszar, R. C. Zatcoff and M. Marikovsky, Wound Repair Regen., 2010, 18, 266 CrossRef
. - D. Han, Y. Han, J. Li, X. Liu, K. W. K. Yeung, Y. Zheng, Z. Cui, X. Yang, Y. Liang and Z. Li, Appl. Catal., B, 2020, 261, 118248 CrossRef CAS
. - M. Li, X. Liu, L. Tan, Z. Cui, X. Yang, Z. Li, Y. Zheng, K. W. K. Yeung, P. K. Chu and S. Wu, Biomater. Sci., 2018, 6, 2110 RSC
. - Z. Chen, H. Meng, G. Xing, C. Chen, Y. Zhao, G. Jia, T. Wang, H. Yuan, C. Ye and F. Zhao, Toxicol. Lett., 2006, 163, 109 CrossRef CAS
. - G. J. Brewer, Chem. Res. Toxicol., 2010, 23, 319 Search PubMed
. - K. Jomova and M. Valko, Tosicology, 2011, 283, 65 Search PubMed
. - X. X. Xu, M. H. Ding, J. X. Zhang, W. Zheng, L. Li and Y. F. Zheng, J. Biomed. Mater. Res., Part B, 2014, 101, 1428 CrossRef
. - J. Xiao, S. Chen, J. Yi, H. Zhang and G. A. Ameer, Adv. Funct. Mater., 2017, 27, 1604872 CrossRef
. - R. Reshmi, B. Bharaniraja and A. Abi Santhosh, Contraception, 2015, 92, 585 CrossRef
. - G. Liangran, P. Irene, D. D. Yan, S. Kevin, L. Yajuan, C. Yi-Tzai, M. Hang, D. B. Niesen, S. Navindra and A. Aftab, ACS Nano, 2013, 7, 8780 CrossRef
. - Y. Qin, L.-L. Chen, W. Pu, P. Liu, S.-X. Liu, Y. Li, X.-L. Liu, Z.-X. Lu, L.-Y. Zheng and Q.-E. Cao, Chem. Commun., 2019, 55, 2206 RSC
. - D. Caroline, D. L. S. Stéphanie Bruneau, B. Françoise, R. Patrice, W. Jean-Michel and B. Christophe, Invest. Ophthalmol. Vis. Sci., 2002, 43, 3409 Search PubMed
. - G. M. Khan and J. B. Zhu, J. Controlled Release, 1999, 57, 197 CrossRef CAS
. - Q. Hui, L. Zhang, X. Yang, B. Yu, Z. Huang, S. Pang, Q. Zhou, R. Yang, W. Li, L. Hu, X. Li, G. Cao and X. Wang, ACS Biomater. Sci. Eng., 2018, 4, 1661 CAS
. - Z. F. Li, E. T. Kang, K. G. Neoh and K. L. Tan, Biomaterials, 1998, 19, 45 CrossRef CAS
. - J. G. Franchina, W. M. Lackowski, D. L. Dermody, R. M. Crooks, D. E. Bergbreiter, K. Sirkar, R. J. Russell and M. V. Pishko, Anal. Chem., 1999, 71, 3133 CrossRef CAS
. - Z. Niu, S. Fang, X. Liu, J. G. Ma, S. Q. Ma and P. Cheng, J. Am. Chem. Soc., 2015, 137, 14873 CrossRef CAS
. - I. Irwansyah, Y. Q. Li, W. Shi, D. Qi, W. R. Leow, M. B. Tang, S. Li and X. Chen, Adv. Mater., 2015, 27, 648 CrossRef CAS
. - X. Li, J. Fei, Y. Xu, D. Li, T. Yuan, G. Li, C. Wang and J. Li, Angew. Chem., 2018, 57, 1903 CrossRef CAS
. - A. Falase, K. Garcia, C. Lau and P. Atanassov, Electrochem. Commun., 2011, 13, 1488 CrossRef CAS
. - F. Rossi, X. Chatzistavrou, G. Perale and A. R. Boccaccini, J. Appl. Polym. Sci., 2012, 123, 398 CrossRef CAS
. - I. Elsayed and M. H. H. Aboughaly, Expert Opin. Drug Delivery, 2015, 8, 1 Search PubMed
. - A. C. Rumondor and L. S. Taylor, Mol. Pharm., 2010, 7, 477 CrossRef CAS
. - N. A. Visalakshi, T. T. Mariappan, H. Bhutani and S. Singh, Pharm. Dev. Technol., 2005, 10, 489 CrossRef CAS
. - M. C. Robson, Surg. Clin. North Am., 1997, 77, 637 CrossRef CAS
. - J. D. Oliver, Epidemiol. Infect., 2005, 133, 383 CrossRef CAS
. - M. C. Robson, B. D. Stenberg and J. P. Heggers, Clin. Plast. Surg., 1990, 17, 485 CrossRef CAS
. - R. Wang, J. Li, W. Chen, T. Xu, S. Yun, Z. Xu, Z. Xu, T. Sato, B. Chi and H. Xu, Adv. Funct. Mater., 2017, 27, 1604894 CrossRef
. - Z. Fan, B. Liu, J. Wang, S. Zhang, Q. Lin, P. Gong, L. Ma and S. Yang, Adv. Funct. Mater., 2014, 24, 3933 CrossRef CAS
. - H. Williams, R. A. Crompton, H. A. Thomason, L. Campbell, G. Singh, A. J. McBain, S. M. Cruickshank and M. J. Hardman, J. Invest. Dermatol., 2017, 137, 2427 CrossRef CAS
. - L. Shang, S. Dong and G. U. Nienhaus, Nano Today, 2011, 6, 401 CrossRef CAS
. - K. Savolainen, H. Alenius, H. Norppa, L. Pylkkänen, T. Tuomi and G. Kasper, Toxicology, 2010, 269, 92 CrossRef CAS
. - G. Oberdorster, J. Intern. Med., 2010, 267, 89 CrossRef CAS
. - P. Cai, X. Zhang, M. Wang, Y. L. Wu and X. Chen, ACS Nano, 2018, 12, 5078 CrossRef CAS
. - B. W. Neun and M. A. Dobrovolskaia, Nano Lett., 2011, 8, 2180 Search PubMed
. - A. S. Colwell, T. M. Krummel, M. T. Longaker and L. H Peter, Plast. Reconstr. Surg., 2006, 117, 2292 CrossRef CAS
. - R. D. Galiano, J. Michaels, M. Dobryansky, J. P. Levine and G. C. Gurtner, Wound Repair Regen., 2004, 12, 485 CrossRef
. - Y. Zhu, R. Hoshi, S. Chen, J. Yi, C. Duan, R. D. Galiano, H. F. Zhang and G. A. Ameer, J. Controlled Release, 2016, 238, 114 CrossRef CAS
. - J. S. Choi, K. W. Leong and H. S. Yoo, Biomaterials, 2008, 29, 587 CrossRef CAS
. - L. A. R. Cho, H. Leem, J. Lee and K. C. Park, Biomaterials, 2005, 26, 4670 CrossRef
. - K. Breuing, C. Andree, G. Helo, J. Slama, P. Y. Liu and E. Eriksson, Plast. Reconstr. Surg., 1997, 100, 657 CrossRef CAS
. - A. J. Singer and R. A. F. Clark, N. Engl. J. Med., 1999, 341, 738 CrossRef CAS
. - R. F. Diegelmann, Front. Biosci., 2004, 9, 283 CrossRef CAS
. - I. A. Silver, Equine Vet. J., 1973, 5, 47 CrossRef CAS
. - W. L. Garner, Plast. Reconstr. Surg., 1998, 102, 135 CrossRef CAS
. - J. F. Woessner, Arch. Biochem. Biophys., 1961, 93, 440 CrossRef CAS
. - N. N. Nissen, P. J. Polverini, A. E. Koch, M. V. Volin, R. L. Gamelli and L. A. DiPietro, Am. J. Pathol., 1998, 152, 1445 CAS
. - M. G. Tonnesen, X. Feng and R. A. F. Clark, J. Invest. Dermatol. Symp. Proc., 2000, 5, 40 CrossRef CAS
. - H. M. DeLisser, P. J. Newman and S. M. Albelda, Immunol. Today, 1994, 15, 490 CrossRef CAS
. - S. Barrientos, O. Stojadinovic, M. Golinko, H. Brem and M. Tomic-Canic PhD, Wound Repair Regen., 2008, 16, 585 CrossRef
. - S. Werner, R. Grose, S. Werner and R. Grose, Physiol. Rev., 2003, 83, 835 CrossRef CAS
. - M. Gaudry, O. Brégerie, V. Andrieu, J. El Benna, M. A. Pocidalo and J. Hakim, Blood, 1997, 90, 4153 CrossRef CAS
. - J. H. Ryu, Y. Lee, W. H. Kong, T. G. Kim, T. G. Park and H. Lee, Biomacromolecules, 2011, 12, 2653 CrossRef CAS
.
Footnotes |
† Electronic supplementary information (ESI) available. See DOI: 10.1039/c9ra10031h |
‡ These authors contributed equally to this work. |
|
This journal is © The Royal Society of Chemistry 2020 |
Click here to see how this site uses Cookies. View our privacy policy here.