DOI:
10.1039/C9RA10848C
(Paper)
RSC Adv., 2020,
10, 11808-11815
Mg3N2-assisted one-pot synthesis of 1,3-disubstituted imidazo[1,5-a]pyridine†
Received
24th December 2019
, Accepted 3rd March 2020
First published on 23rd March 2020
Abstract
A novel Mg3N2-assisted one-pot annulation strategy has been developed via cyclo-condensation reaction of 2-pyridyl ketones with alkyl glyoxylates or aldehydes, allowing the formation of imidazo[1,5-a]pyridines exclusively with an exellent yield.
Introduction
The design and synthesis of new azaheterocyclic ring systems are highly necessary in modern drug discovery to achieve specific drug–receptor interactions.1,20 Among them, imidazo[1,5-a]pyridine is one of the most important and medicinally fascinating heterocyclic ring systems, which functions as a building block for the synthesis of important bio-conjugates. Additionally, it plays a crucial role in many areas of research including pharmaceuticals2 and materials science.3 Furthermore, the applications of its derivatives have also been actively probed in organic light-emitting diodes (OLED),4 precursors of N-heterocyclic carbenes5 and the design of different metal complexes.6 Thus, due to the superior activity of imidazo[1,5-a]pyridine and its derivatives, the development of new versatile and efficient protocols for their synthesis has attracted increasing interest. The existing methods7 mainly rely on the traditional dehydrative,8,18 desulfurative9 and oxidative10,13a,19 (Fig. 1, eqn (a)) intra-/intermolecular cyclization of 2-pyridinylmethylamine derivatives (with carbonyl compounds). Another striking tool is the direct C–H amination/cyclization strategy.11 The research groups of Wang11a and Wei11c independently investigated the synthesis of imidazo[1,5-a]pyridines via the sequential dual oxidative amination of the C sp3–H bonds under metal-free conditions. Moreover, the latest reports on the synthesis of imidazo[1,5-a]pyridine present a straightforward way to construct this particular heterocyclic ring system via the decarboxylative cyclic annulation of amines12 (Fig. 1, eqn (b)) or α-amino acids13,19 (Fig. 1, eqn (c)) with 2-pyridyl carbonyl compounds. Encouraged by these previous achievements and in continuation of our exploits on the development of novel approaches towards biologically active compounds,14 we hypothesized the development of a rapid, more practical and eco-friendly technique by utilizing a secondary nitrogen source for the shaping of the imidazo[1,5-a]pyridine ring structure (Fig. 1, eqn (d)).
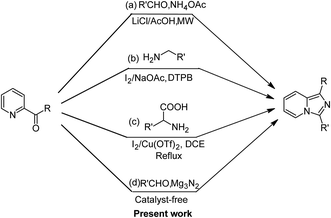 |
| Fig. 1 Synthetic methods for the formation of imidazo[1,5-a]pyridine. | |
To accomplish this goal, we searched for a substance that can act as a substitute for ammonia. A literature review revealed that magnesium nitride (Mg3N2) can act as a convenient source of ammonia when used in protic media and forms a magnesium salt with the potential to act as a catalyst.15a Moreover, recent reports on Mg3N2 highlight its applicability in the synthesis of diverse azaheterocyclic ring systems.15 Thus, herein, we report a new methodology for the Mg3N2-assisted one-pot annulation reaction towards the synthesis of 1,3-disubstituted imidazo[1,5-a]pyridines using 2-pyridyl ketone and ethyl/methyl glyoxylates in a protic solvent. The rationale behind the use of glyoxylate is based on its high degree of electrophilic character and the production of imidazo[1,5-a]pyridine carboxylates that can be synthetically manipulated into complex architectures. So far, to the best of our knowledge, there is no report describing the applicability of Mg3N2 in the synthesis of imidazo[1,5-a]pyridinyl carboxylates.
Our study began with the two-step synthesis of commercially unavailable 2-pyridyl ketones, which were derived from the Grignard reaction of 2-pyridylmagnesium chloride and N,N-dialkyl alkyl/aryl amides according to a known procedure,16a and their characterization data were in accordance with the literature.16 Having oven-ready 2-pyridyl ketone as a key substrate in hand, the next investigation commenced with establishing the best reaction conditions, where dehydrative annulation occurred smoothly. Initially, in an attempt for the stepwise formation of the desired 1,3-disubstituted imidazo[1,5-a]pyridines via the intermediate 2-pyridinylmethyl imine, we chose 2-pyridyl phenyl ketone (1a) and Mg3N2 as model substrates without their isolation, which were immediately treated for cyclization with ethyl glyoxylate (2a) (Scheme 1, route I). However, the above reaction only resulted in partial conversion under ambient conditions and at high temperatures (Table 1, entries 1–4). This indicates that the incomplete formation of ketoimine may be due to the slow evolution of ammonia or ammonia simply escaping out. Another possibility is that the complexation of the free Mg-salt with 2-imido pyridine17 prevents its nucleophilic attack on the aldehyde.
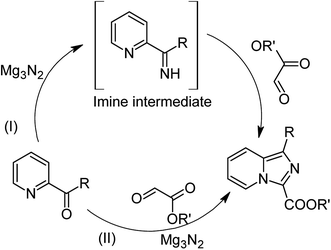 |
| Scheme 1 Mg3N2-assisted (I) stepwise and (II) one-pot annulation reaction for the synthesis of imidazo[1,5-a]pyridine. | |
Table 1 Optimization study for the one pot synthesis of 3a
Thus, to achieve complete conversion, in next attempt we used a sealed tube and tested the one-pot annulation (Scheme 1, route II). To our delight, the employment of a closed system ended with satisfactory conversion but extended the reaction time at ambient temperature (Table 1, entry 5). Thus, to enhance the rate of the reaction, moderate heating was applied, which stimulated the formation of 3a in excellent yields (Table 1, entry 6) and the product structure was finally unambiguously established by analyzing its IR, and 1H and 13C NMR spectra.
The results shown in Table 1 indicate that the solvent combination plays a crucial role in the present transformation (Table 1, entries 7–11). Attempts to employ other nitrogen sources (Table 1, entries 9 and 10) did not result in an improvement in percentage yield of 3a against Mg3N2, while increasing the amount of Mg3N2 to 1.5 equivalents resulted in the same yield (Table 1, entry 11). Thus, the optimal reaction conditions were achieved using 1a (1 equiv.), Mg3N2 (1 equiv.) and methyl glyoxylate 2a (1 equiv.) in EtOH
:
water (8
:
2) as the solvent system to obtain the maximum yield of 3a (Table 1, entry 8), and hence the same combination was chosen for further studies.
With these results in hand, we sought to examine the scope and generality of the method by employing a wide range of 2-pyridyl ketones. As shown in Table 2, this methodology tolerates a wide range of 2-pyridyl ketones (1a–k) bearing alkyl/aryl and hetero aryl functionalities. All these substrates were smoothly transformed into the expected imidazo[1,5-a]pyridines (3a–m) exclusively by reacting with aldehydes (2a–c) in the same pot and no anomalies were observed. Pleasingly, the sterically hindered 2-(3-methyl)pyridyl ketones (Table 2, entries 2, 4, 6, 8, and 10) also worked well under the standard conditions by affording the corresponding products in good yields. It is noteworthy that the electronic nature of the substituent on aroyl (Table 2, entries 1 and 2 and 11 and 12), heteroaroyl (Table 2, entries 3 and 4) and acyl (Table 2, entries 5–10) did not influence the rate of product formation significantly, which clearly demonstrates the high efficiency and wide generality of the present protocol. Notably, in the long run, up to five-gram-scale synthesis of 3a was achieved successfully, confirming the synthetic practicality of the present method.
Table 2 One-pot synthesis of imidazo[1,2-a]pyridinea
Mechanism
Based on the abovementioned experimental results and the latest mechanistic studies on the role of Mg3N2 in azaheterocyclic chemistry,15 we rationalized the plausible mechanism, as depicted in Scheme 2. The reasonable explanation for the one-pot annulation between 2-pyridyl ketone and aldehyde is attributed to the simultaneous formation of two types of imines such as keto imine A (Path a) and aldimine D (Path b). Owing to the higher degree of electrophilic character of aldehyde compared to ketone, the formation of aldimines derived from ethyl glyoxylate/aldehyde seems to be very convenient, and hence Path (b) seems to be more feasible than Path (a). Consequently, the previously released Mg-salt may co-ordinates with 2-pyridyl ketone,17d which upon graceful nucleophilic attack of aldimine D, leads to the formation of intermediate E. This upon successive intramolecular cyclization to intermediate F followed by the release of a water molecule, generates G. Finally, the intermediate G quickly tautomerizes to rearrange into the more stable imidazo[1,5-a]pyridine (3) as the only product.
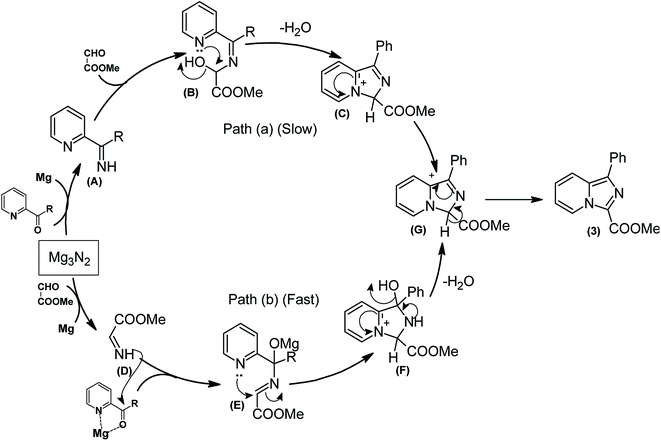 |
| Scheme 2 Plausible mechanistic rationalization for the synthesis of imidazo[1,5-a]pyridine. | |
Conclusion
In summary, we demonstrated a novel and efficient strategy in terms of cost and ease of use for the synthesis of diverse and functionalized imidazo[1,5-a]pyridines through an unprecedented annulation of readily available 2-pyridyl ketones and Mg3N2 as a secondary nitrogen/amine source. We believe that any comparison between Mg3N2 and other precious amines (particularly amino acids) should indicate that Mg3N2 has valuable and unique reactivity with an entirely different set of chemical transformations, which fulfills the demands of academia and industry. Moreover, the synthesized imidazo[1,5-a]pyridine-3-carboxylates can be converted into more complex heterocycles via a systematic approach, which is ongoing in our laboratory.
Experimental
General information
Commercially available reagents were purchased from commercial suppliers and used without further purification. Reactions were monitored by thin layer chromatography (TLC) using MERCK precoated silica gel plates. Column chromatography was performed over silica gel (200–300 mesh). 1H NMR and 13C NMR spectra were recorded on 400 MHz NMR and 100 MHz spectrometers, respectively. Chemical shifts (δ) are expressed in parts per million (ppm), and coupling constants (J) are given in hertz (Hz). Chemical shifts are referenced to CDCl3 (δ = 7.27 for 1H and δ = 77.16 for 13C NMR) as an internal standard. Infrared spectra were recorded on an FTIR spectrometer. Samples were examined as KBr discs with ∼5% w/w. Elemental analyses were performed on a EURO EA3000 Vector model. Melting points were determined on a melting point apparatus and are uncorrected. High-resolution mass spectra were obtained using a GCT-TOF instrument with an ESI source.
General procedure for the preparation of imidazo[1,5-a]pyridines 3(a–m)
A mixture of 2-pyridyl ketones 1 (1 mol), aldehyde 2 (1 mol) and Mg3N2 (0.150 g, 1 mol) in EtOH
:
water (8
:
2) (3 mL) in a 5 mL sealed tube was stirred at 80 °C for the time indicated in Table 1. Upon complete consumption of ketone 1 (monitored by TLC), the reaction mixture was allowed to cool to room temperature, quenched in ice-cold water (10 mL), and extracted with EtOAc (3 × 15 mL). The combined organic layer was dried over Na2SO4, the was solvent removed in vacuo and the product was purified through silica gel column chromatography using a mixture of EtOAc and n-hexane as the eluent to afford the corresponding product 3.
1) Methyl 1-phenylimidazo[1,5-a]pyridine-3-carboxylate (3a). Yellow solid; 92% yield; mp 139–141 °C; Rf 0.45 (n-hexane/EtOAc 8
:
2); 1H NMR (400 MHz, CDCl3) δ 9.42 (ddd, J = 6.8, 1.2, 0.8 Hz, 1H), 7.78–7.73 (m, 3H), 7.47–7.43 (m, 4H), 7.05 (td, J = 7.2, 1.2 Hz, 1H), 3.8 (s, 3H) ppm; 13C NMR (100 MHz, CDCl3) δ 161.5, 153.5, 147.1, 134.3, 130.0, 128.7, 128.3, 128.1, 127.7, 117.4, 114.2, 51.3 ppm; FT-IR (KBr, thin film): ν 3044.8, 2955.9, 1674.4, 1492.1, 1473.6, 1385.5, 1340.6, 1224.3, 1159.8, 917.5, 753.4, 743.3, 700.3 cm−1; anal. calc. for C15H12N2O2: % C, 71.42; % H, 4.79; % N, 11.10, observed: % C, 71.40; % H, 4.80; % N, 11.08; MS (EI): m/z = 252 (M+).
2) Methyl 8-methyl-1-phenylimidazo[1,5-a]pyridine-3-carboxylate (3b). Beige solid; 85% yield; mp 148–150 °C; Rf 0.50 (n-hexane/EtOAc 8
:
2); 1H NMR (400 MHz, CDCl3) δ 9.29 (dd, J = 6.8, 1.2 Hz, 1H), 7.76–7.74 (m, 2H), 7.47–7.41 (m, 3H), 7.26–7.24 (m, 1H), 6.99 (t, J = 6.8 Hz, 1H), 3.80 (s, 3H), 2.69 (s, 3H) ppm; 13C NMR (100 MHz, CDCl3) δ 161.6, 152.9, 147.2, 134.4, 130.1, 128.6, 127.4, 127.1, 126.0, 114.2, 51.2, 17.1 ppm; FT-IR (KBr, thin film): ν 2954.4, 2892.6, 1680.3, 1521.0, 1491.4, 1378.9, 1328.4, 1243.8, 1221.8, 1152.5, 784.7, 754.7 cm−1; anal. calc. for C16H14N2O2: % C, 72.16; % H, 5.30; % N, 10.52, observed: % C, 72.19; % H, 5.34; % N, 10.49; MS (EI): m/z = 266 (M+).
3) Ethyl 1-(2-thionyl)imidazo[1,5-a]pyridine-3-carboxylate (3c). Beige solid; 90% yield; mp 98–100 °C; Rf 0.46 (n-hexane/EtOAc 8
:
2); 1H NMR (400 MHz, CDCl3) δ 9.36 (dd, J = 7.2, 1.2 Hz, 1H), 8.04 (dd, J = 3.6, 1.2 Hz, 1H), 7.70–7.68 (m, 1H), 7.47 (dd, J = 4, 1.2 Hz, 1H), 7.42–7.38 (m, 1H), 7.15–7.13 (m, 1H), 7.00–6.96 (m, 1H), 4.55 (q, J = 7.2 Hz, 2H), 1.47 (t, J = 7.2 Hz, 3H) ppm; 13C NMR (100 MHz, CDCl3) δ 160.8, 146.8, 146.6, 136.6, 129.8, 128.5, 128.2, 128.1, 127.3, 117.1, 113.9, 110.8, 60.8, 14.4 ppm; FT-IR (KBr, thin film): ν 3034.6, 1694.8, 1496.0, 1446.0, 1338.4, 1231.6, 1228.1, 1157.9, 1086.3, 1046.0, 963.5, 852.9, 720.4 cm−1; anal. calc. for C14H12N2O2S: % C, 61.75; % H, 4.44; % N, 10.29, observed: % C, 61.79; % H, 4.40; % N, 10.25; MS (EI): m/z = 272 (M+).
4) Ethyl 1-(2-thionyl)-8-methylimidazo[1,5-a]pyridine-3-carboxylate (3d). Off-white powder; 83% yield; mp 99–101 °C; Rf 0.51 (n-hexane/EtOAc 8
:
2); 1H NMR (400 MHz, CDCl3) δ 9.23 (d, J = 6.8 Hz, 1H), 7.99 (dd, J = 3.6, 1.2 Hz, 1H), 7.47 (dd, 1H), 7.21 (d, J = 6.8 Hz, 1H), 7.15 (dd, J = 4, 1.2 Hz, 1H), 6.92 (t, J = 6.8 Hz, 1H), 4.50 (q, J = 7.2 Hz, 2H), 2.67 (s, 3H), 1.46 (t, J = 7.2 Hz, 3H) ppm; 13C NMR (100 MHz, CDCl3) δ 160.9, 147.1, 146.1, 136.8, 129.7, 127.9, 127.2, 127.1, 127.0, 126.1, 114.0, 60.6, 16.9, 14.3 ppm; FT-IR (KBr, thin film): ν 2984.2, 2969.7, 2945.1, 1683.0, 1458.3, 1442.7, 1394.0, 1328.0, 1271.1, 1154.9, 1068.4, 1001.0, 952.3, 928.6, 807.6, 746.3, 697.3 cm−1; anal. calc. for C15H14N2O2S: % C, 62.92; % H, 4.93; % N, 9.78, observed: % C, 62.95; % H, 5.00; % N, 9.80; MS (EI): m/z = 286 (M+).
5) Ethyl 1-methylimidazo[1,5-a]pyridine-3-carboxylate (3e). Off-white powder; 87% yield; mp 119–120 °C; Rf 0.41 (n-hexane/EtOAc 8
:
2); 1H NMR (400 MHz, CDCl3) δ 9.26 (d, J = 6.8 Hz, 1H), 7.57 (d, 1H), 7.34–7.30 (m, 1H), 6.93 (t, J = 6.8 Hz, 1H), 4.41 (q, J = 7.2 Hz, 2H), 2.67 (s, 3H), 1.41 (t, J = 7.2 Hz, 3H) ppm; 13C NMR (100 MHz, CDCl3) δ 161.3, 152.7, 146.8, 127.8, 127.4, 116.5, 113.5, 112.5, 60.2, 16.6, 14.4 ppm; FT-IR (KBr, thin film): ν 2979.3, 2951.4, 2928.9, 1692.5, 1497.3, 1459.1, 1385.1, 1267.0, 1153.6, 1060.0, 951.8, 879.2, 756.9, 704.1 cm−1; anal. calc. for C11H12N2O2: % C, 64.69; % H, 5.93; % N, 13.72, observed: % C, 64.70; % H, 5.90; % N, 13.70; MS (EI): m/z = 204 (M+).
6) Ethyl 1,8-dimethylimidazo[1,5-a]pyridine-3-carboxylate (3f). Off-white powder; 75% yield; mp 110–112 °C; Rf 0.41 (n-hexane/EtOAc 8
:
2); 1H NMR (400 MHz, CDCl3) δ 9.18 (d, J = 6.8 Hz, 1H), 7.18 (dt, J = 7.2, 1.2 Hz,1H), 6.89 (t, J = 7.2 Hz, 1H), 4.45 (q, J = 7.2 Hz, 2H), 2.74 (s, 3H), 2.62 (s, 3H), 1.43 (t, J = 7.2 Hz, 3H) ppm; 13C NMR (100 MHz, CDCl3) δ 161.6, 152.1, 147.0, 128.6, 126.4, 125.7, 113.5, 112.9, 60.2, 17.0, 16.7, 14.4 ppm; FT-IR (KBr, thin film): ν 2994.0, 2980.6, 2957.7, 2947.5, 1718.5, 1693.8, 1546.6, 1484.6, 1452.5, 1393.4, 1345.1, 1291.0, 1140.2, 1092.1, 1058.5, 1021.0, 748.3, 710.3 cm−1; anal. calc. for C14H14N2O2: % C, 66.04; % H, 6.47; % N, 12.84, observed: % C, 66.00; % H, 6.50; % N, 12.89, MS (EI): m/z = 218 (M+).
7) Ethyl 1-cyclopropylimidazo[1,5-a]pyridine-3-carboxylate (3g). Pale yellow solid; 88% yield; mp 98–100 °C; Rf 0.42 (n-hexane/EtOAc 8
:
2); 1H NMR (400 MHz, CDCl3) δ 9.31 (ddd, J = 7.2, 1.2, 0.8 Hz, 1H), 7.56 (dd, 1H), 7.36–7.32 (m, 1H), 6.94 (td, J = 7.2, 1.2 Hz, 1H), 4.48 (q, 2H), 2.87 (quin, 1H), 1.46 (t, J = 7.2 Hz, 3H), 1.22 (dt, 2H), 1.10 (dt, 2H) ppm; 13C NMR (100 MHz, CDCl3) δ 161.7, 158.1, 147.1, 127.9, 127.7, 116.4, 113.2, 60.2, 14.5, 9.9 ppm; FT-IR (KBr, thin film): ν 3082.4, 2984.3, 2955.6, 1675.2, 1536.8, 1413.4, 1341.6, 1088.3, 1055.8, 768.9 cm−1; anal. calc. for C13H14N2O2: % C, 67.81; % H, 6.13; % N, 12.17, observed: % C, 67.85; % H, 6.10; % N, 12.15; MS (EI): m/z = 230 (M+).
8) Ethyl 1-cyclopropyl-8-methylimidazo[1,5-a]pyridine-3-carboxylate (3h). Off-white powder; 80% yield; mp 105–108 °C; Rf 0.38 (n-hexane/EtOAc 8
:
2); 1H NMR (400 MHz, CDCl3) δ 9.15–9.13 (m, 1H), 7.11 (dd, 1H), 6.82 (t, J = 6.8 Hz, 1H), 4.47 (q, J = 7.2 Hz, 2H), 2.84 (quin, 1H), 2.54 (s, 3H), 1.46 (t, J = 7.2 Hz, 3H), 1.30–1.19 (m, 3H), 1.07–1.04 (m, 2H) ppm; 13C NMR (100 MHz, CDCl3) δ 161.9, 157.4, 147.4, 126.4, 125.6, 113.1, 60.1, 16.9, 14.5, 10.1 ppm; FT-IR (KBr, thin film): ν 2999.3, 2976.5, 2934.4, 1676.7, 1559.1, 1412.3, 1381.0, 1338.6, 1236.3, 1185.7, 1086.2, 901.6, 779.3, 756.4 cm−1; anal. calc. for C14H16N2O2: % C, 68.83; % H, 6.60; % N, 11.47, observed: % C, 68.80; % H, 6.65; % N, 11.50; MS (EI): m/z = 244 (M+).
9) Methyl 1-tert-butylimidazo[1,5-a]pyridine-3-carboxylate (3i). Pale yellow solid; 78% yield; mp 126–128 °C; Rf 0.32 (n-hexane/EtOAc 8
:
2); 1H NMR (400 MHz, CDCl3) δ 9.32 (ddd, J = 7.2, 1.2, 0.8 Hz), 7.71–7.68 (m, 1H), 7.38–7.34 (m, 1H), 6.98 (td, J = 6.8, 1.2 Hz, 1H), 3.99 (s, 3H), 1.53 (s, 9H) ppm; 13C NMR (100 MHz, CDCl3) δ 163.8, 161.5, 145.4, 128.2, 121.1, 117.2, 113.7, 112.1, 51.1, 34.6, 29.5 ppm; FT-IR (KBr, thin film): ν 2984.3, 2946.3, 1683.0, 1516.2, 1474.3, 1344.0, 1250.1, 1140.6, 1052.2, 1029.8, 914.0, 885.7, 750.3, 747.6, 722.1 cm−1; anal. calc. for C13H16N2O2: % C, 67.22; % H, 6.94; % N, 12.06, observed: % C, 67.20; % H, 7.00; % N, 12.10; MS (EI): m/z = 232 (M+).
10) Methyl 1-tert-butyl-8-methylimidazo[1,5-a]pyridine-3-carboxylate (3j). Beige solid; 72% yield; mp 112–114 °C; Rf 0.32 (n-hexane/EtOAc 8
:
2); 1H NMR (400 MHz, CDCl3) δ 9.16 (dd, J = 6.8, 1.2 Hz, 1H), 7.14–7.12 (m, 1H), 6.86 (t, J = 6.8 Hz, 1H), 3.98 (s, 3H), 2.63 (s, 3H), 1.53 (s, 9H) ppm; 13C NMR (100 MHz, CDCl3) δ 163.1, 161.7, 145.5, 127.2, 125.9, 113.6, 112.3, 52.9, 51.0, 34.7, 29.5, 26.1 ppm; FT-IR (KBr, thin film): ν 2952.4, 2929.3, 1759.2, 1714.9, 1651.1, 1474.4, 1392.6, 1343.9, 1237.7, 1185.7, 1098.3, 1074.6, 773.7, 747.1 cm−1; anal. calc. for C14H18N2O2: % C, 68.27; % H, 7.37; % N, 11.37, observed: % C, 68.30; % H, 7.40; % N, 11.40; MS (EI): m/z = 246 (M+).
11) Ethyl 1-(2,4-difluorophenyl)imidazo[1,5-a]pyridine-3-carboxylate (3k). Beige solid; 83% yield; mp 177 °C; Rf 0.32 (n-hexane/EtOAc 8
:
2); 1H NMR (400 MHz, CDCl3) δ 9.41 (d, J = 7.2 Hz,1H), 7.77 (d, J = 6.8 Hz, 1H), 7.64–7.52 (m, 1H), 7.49–7.42 (m, 1H), 7.10–7.07 (m, 1H), 7.01–9.98 (m, 1H), 6.93–6.88 (m, 1H), 4.31 (q, J = 7.2 Hz, 2H), 1.26 (t, J = 7.2 Hz, 3H) ppm; 13C NMR (100 MHz, CDCl3) δ 160.0, 155.4, 145.4, 124.4, 123.6, 111.1, 60.8, 14.4 ppm; FT-IR (KBr, thin film): ν 2978.4, 2939.3, 1685.6, 1497.2, 1393.4, 1337.1, 1217.9, 1169.2, 1135.9, 1043.0, 1008.6, 768.5, 753.4 cm−1; anal. calc. for C16H12F2N2O2: % C, 63.57; % H, 4.00; % N, 9.27, observed: % C, 63.60; % H, 4.05; % N, 9.30; MS (EI): m/z = 302 (M+).
12) 1,3-Diphenylimidazo[1,5-a]pyridine19 (3l). Yellow solid; 85% yield; mp 112–114 °C (lit. mp 110–111 °C); Rf 0.35 (n-hexane/EtOAc 8
:
2); 1H NMR (400 MHz, CDCl3) δ 8.25 (d, 1H), 7.89 (d, J = 5.5 Hz, 2H), 7.80 (s, 5H), 7.55 (d, J = 5.5 Hz, 2H), 7.50 (d, J = 5.5 Hz, 2H), 7.29 (s, 1H), 6.80 (s, 1H), 6.52 (s, 1H) ppm; 13C NMR (100 MHz, CDCl3) δ 139.0, 134.5, 132.0, 130.1, 129.0, 128.6, 128.3, 128.2, 127.5, 126.3, 126.1, 121.4, 120.0, 119.4, 113.2 ppm; FT-IR (KBr, thin film): ν 2978.4, 2939.3, 1655.6, 1497.2, 1393.4, 1337.1, 1217.9, 1169.2, 1135.9, 1043.0, 1008.6, 768.5, 753.4 cm−1; anal. calc. for C19H14N2: % C, 84.42; % H, 5.22; % N, 10.36, observed: % C, 84.49; % H, 5.20; % N, 10.40; MS (EI): m/z = 270 (M+).
13) 1-Methyl-3-phenylimidazo[1,5-a]pyridine18 (3m). Pale yellow oil; 63% yield; Rf 0.40 (n-hexane/EtOAc 8
:
2); 1H NMR (400 MHz, CDCl3) δ 8.23 (d, J = 6.8, Hz, 1H), 8.14 (s, 1H), 7.91 (d, J = 7.4 Hz, 2H), 7.37 (t, J = 7.4 Hz, 2H), 7.26 (t, J = 6.8 Hz, 1H), 6.97 (d, J = 6.8 Hz, 1H), 6.72 (t, J = 6.8 Hz, 1H), 2.53 (s, 3H) ppm; 13C NMR (100 MHz, CDCl3) δ 145.4, 143.7, 133.7, 128.8, 127.9, 126.4, 126.0, 124.6, 124.2, 112.7, 109.6, 17.1 ppm; FT-IR (KBr, thin film): ν 3024.2, 2982.5, 1648.0, 1495.5, 1370.1, 1256.5, 1078.9, 943.7, 741.6, 719.7, 692.2 cm−1; anal. calc. for C14H12N2: % C, 80.74; % H, 5.81; % N, 13.45, observed: % C, 80.75; % H, 5.79; % N, 13.50; MS (EI): m/z = 208 (M+).
Conflicts of interest
There are no conflicts to declare.
References
-
(a) J. A. Joule and K. Mills, Heterocyclic Chemistry, BlackwellScience, Oxford, U.K., 4th edn, 2000, ch. 25 Search PubMed;
(b) M. V. B. Unnamatla, A. Islas-Jácome, A. Quezada-Soto, S. C. Ramárez-López, M. Flores-Álamo and R. Gámez-Montaño, J. Org. Chem., 2016, 81, 10576 CrossRef CAS PubMed;
(c) S. Murru and A. Nefzi, ACS Comb. Sci., 2014, 16, 39 CrossRef CAS PubMed;
(d) S. Kurhade, P. A. Ramaiah, P. Prathipati and D. Bhuniya, Tetrahedron, 2013, 69, 1354 CrossRef CAS.
-
(a) D. R. Mohbiya and N. Sekar, ChemistrySelect, 2018, 3, 1635 CrossRef CAS;
(b) R. Nirogi, A. R. Mohammed, A. K. Shinde, S. R. Gagginapally, D. M. Kancharla, V. R. Middekadi, N. Bogaraju, S. R. Ravella, P. Singh, S. R. Birangal, R. Subramanian, R. C. Palacharla, V. Benade, N. Muddana and P. Jayarajan, J. Med. Chem., 2018, 61, 4993 CrossRef CAS PubMed;
(c) D. Kim, L. Wang, J. J. Hale, C. L. Lynch, R. J. Budhu, M. MacCoss, S. G. Mills, L. Malkowitz, S. L. Gould, J. A. DeMartino, M. S. Springer, D. Hazuda, M. Miller, J. Kessler, R. C. Hrin, G. Carver, A. Carella, K. Henry, J. Lineberger, W. A. Schleifc and E. A. Eminic, Bioorg. Med. Chem. Lett., 2005, 15, 2129 CrossRef CAS PubMed;
(d) H. Nakamura and H. Yamamoto, PCT Int. Appl. WO 2005043630, Chem. Abstr., 2005, 142, 440277.
-
(a) H. Sheng, Y. Hu, Yi. Zhou, S. Fan, Y. Caod, X. Zhao and W. Yang, Dyes Pigm., 2019, 160, 48 CrossRef CAS;
(b) F. Yagishita, C. Nii, Y. Tezuka, A. Tabata, H. Nagamune, N. Uemura, Y. Yoshida, T. Mino, M. Sakamoto and Y. Kawamura, Asian J. Org. Chem., 2018, 7, 1614 CrossRef CAS;
(c) M. D. Weber, C. Garino, G. Volpi, E. Casamassa, M. Milanesio, C. Barolo and R. D. Costa, Dalton Trans., 2016, 45, 8984 RSC.
- L. Salassa, C. Garino, A. Albertino, G. Volpi, C. Nervi, R. Gobrtto and K. I. Hardcastle, Organometallics, 2008, 27, 1427 CrossRef CAS.
-
(a) M. Alcarazo, S. J. Roseblade, A. R. Cowley, R. Fernandez, J. M. Brown and J. M. Lassaletta, J. Am. Chem. Soc., 2005, 127, 3290 CrossRef CAS PubMed;
(b) F. E. Hahn, Angew. Chem., Int. Ed., 2006, 45, 1348 CrossRef CAS PubMed.
-
(a) E. Y. Tsui and T. Agapie, Polyhedron, 2014, 84, 103 CrossRef CAS PubMed;
(b) G. A. Ardizzoia, S. Brenna, S. Durini, B. Therrien and M. Veronelli, Eur. J. Inorg. Chem., 2014, 26, 4310 CrossRef;
(c) M. Kriechbaum, D. Otte, M. List and U. Monkowius, Dalton Trans., 2014, 43, 8781 RSC;
(d) A. M. Blanco-Rodriguez, H. Kvapilova, J. Sykora, M. Towrie, C. Nervi, G. Volpi, S. Zalis and A. Vlcek, J. Am. Chem. Soc., 2014, 136, 5963 CrossRef CAS PubMed;
(e) S. Roy, S. Javed, M. M. Olmstead and A. K. Patra, Dalton Trans., 2011, 40, 12866 RSC.
-
(a) M. Qin, Y. Tian, X. Guo, X. Yuan, X. Yang and B. Chen, Asian J. Org. Chem., 2018, 7, 1591 CrossRef CAS;
(b) Z. Yan, C. Wan, Y. Yang, Z. Zha and Z. Wang, RSC Adv., 2018, 8, 23058 RSC;
(c) G. Volpi, G. Magnano, I. Benesperi, D. Saccone, E. Priola, V. Gianotti, M. Milanesio, E. Conterosito, C. Barolo and G. Viscardi, Dyes Pigm., 2017, 137, 152 CrossRef CAS.
-
(a) J. D. Bower and C. R. Ramage, J. Chem. Soc., 1955, 2834 RSC;
(b) G. Pelletier and A. B. Charette, Org. Lett., 2013, 15, 2290 CrossRef CAS PubMed;
(c) Y. Shi, A. V. Gulevich and V. Gevorgyan, Angew. Chem., Int. Ed., 2014, 53, 14191 CrossRef CAS PubMed.
- F. Shibahara, A. Kitagawa, E. Yamaguchi and T. Murai, Org. Lett., 2006, 8, 5621 CrossRef CAS PubMed.
-
(a) F. Shibahara, R. Sugiura, E. Yamaguchi, A. Kitagawa and T. Murai, J. Org. Chem., 2009, 74, 3566 CrossRef CAS PubMed;
(b) L. Hu, L. Gao, C. Wan and Z. Wang, Acta Chim. Sin., 2013, 71, 1603 CrossRef CAS.
-
(a) Y. Yan, Y. Zhang, Z. Zha and Z. Wang, Org. Lett., 2013, 15, 2274 CrossRef CAS PubMed;
(b) D. C. Mohan, S. N. Rao, C. Ravi and S. Adimurthy, Org. Biomol. Chem., 2015, 13, 5602 RSC;
(c) J. Sheng, J. Liu, H. Zhao, L. Zheng and X. Wei, Org. Biomol. Chem., 2018, 16, 5570 RSC.
-
(a) Q. Wang, S. Zhang, F. Guo, B. Zhang, P. Hu and Z. Wang, J. Org. Chem., 2012, 77, 11161 CrossRef CAS PubMed;
(b) A. Joshi, D. C. Mohan and S. Adimurthy, Org. Lett., 2016, 18, 464 CrossRef CAS PubMed;
(c) H. Wang, W. Xu, L. Xin, W. Liu, Z. Wang and K. Xu, J. Org. Chem., 2016, 81, 3681 CrossRef CAS PubMed.
-
(a) M. Li, Y. Xie, Y. Ye, Y. Zou, H. Jiang and W. Zeng, Org. Lett., 2014, 16, 6232 CrossRef CAS PubMed;
(b) H. T. H. Nguyen, O. T. K. Nguyen, T. Truong and N. T. S. Phan, RSC Adv., 2016, 6, 36039 RSC;
(c) A. K. Gupta, D. De, K. Tomar and P. K. Bharadwaj, Dalton Trans., 2018, 47, 1624 RSC;
(d) Z. Hu, J. Hou, J. Liu, W. Yu and J. Chang, Org. Biomol. Chem., 2018, 16, 5653 RSC.
-
(a) J. Jadhav, V. Gaikwad, R. Kurane, R. Salunkhe and G. Rashinkar, Synlett, 2012, 2511 CAS;
(b) J. Jadhav, S. Khanapaure, R. Kurane, R. Salunkhe and G. Rashinkar, Tetrahedron Lett., 2013, 54, 6858 CrossRef CAS;
(c) J. Jadhav, A. Juvekar, R. Kurane, S. Khanapure, R. Salunkhe and G. Rashinkar, Eur. J. Med. Chem., 2013, 65, 232 CrossRef CAS PubMed;
(d) P. Bansode, J. Jadhav, R. Kurane, P. Choudhari, M. Bhatia, S. Khanapure, R. Salunkhe and G. Rashinkar, RSC Adv., 2016, 6, 90597 RSC.
-
(a) K. L. Bridgwood, G. E. Veitch and S. V. Ley, Org. Lett., 2008, 10, 3627 CrossRef CAS PubMed;
(b) G. E. Veitch, K. L. Bridgwood, K. R. Trevor and S. V. Ley, Synlett, 2008, 7, 2597 Search PubMed;
(c) S. Long, M. Panunzio, A. Petroli, W. Qin and Z. Xia, Synthesis, 2011, 7, 1071 Search PubMed;
(d) C. K. Banerjee, J. D. Umarye and P. R. Kanjilal, Synth. Commun., 2013, 43, 2208 CrossRef CAS.
-
(a) G. V. Rao, B. N. Swamy, P. H. Kumar and G. C. Reddy, Synth. Commun., 2009, 39, 1835 CrossRef CAS;
(b) K. Colas, A. C. V. D. dos Santos and A. Mendoza, Org. Lett., 2019, 21(19), 7908 CrossRef CAS PubMed;
(c) Q. Wu, S. Han, X. Ren, X. Ren, H. Lu, J. Li, D. Zou, Y. Wu and Y. Wu, Org. Lett., 2018, 20, 6345 CrossRef PubMed;
(d) S. Ağar and O. T. Gunkara, J. Turk. Chem. Soc., Sect. A, 2017, 5, 247 CrossRef.
-
(a) Y. Baia, W. Chen, J. Li and C. Cui, Coord. Chem. Rev., 2019, 383, 132 CrossRef;
(b) J. J. Sandoval, P. Palma, E. Álvarez, J. Cámpora and A. R. Delgado, Organometallics, 2016, 35, 3197 CrossRef CAS;
(c) A. R. Sayed, M. M. Youssef and Y. S. Al- Faiyz, J. Appl. Sci., 2015, 15, 884 CrossRef CAS;
(d) G. S. Papaefstathiou and S. P. Perlepes, Comments Inorg. Chem., 2002, 23, 249 CrossRef CAS.
- J. M. Crawforth and M. Paoletti, Tetrahedron Lett., 2009, 50, 4916 CrossRef CAS.
-
(a) Z. Xie, J. Peng and Q. Zhu, Org. Chem. Front., 2016, 3, 82 RSC;
(b) H. Wang, W. Xu, Z. Wang, L. Yu and K. Xu, J. Org. Chem., 2015, 80, 2431 CrossRef CAS PubMed.
- A. R. Katritzky, C. A. Ramsden, E. F. V. Scriven and R. J. K. Taylor, Comprehensive Heterocyclic Chemistry III, Elsevier, Oxford, U.K., 2008, vol. 11 Search PubMed.
Footnote |
† Electronic supplementary information (ESI) available. See DOI: 10.1039/c9ra10848c |
|
This journal is © The Royal Society of Chemistry 2020 |
Click here to see how this site uses Cookies. View our privacy policy here.