DOI:
10.1039/D0RA00020E
(Paper)
RSC Adv., 2020,
10, 18044-18053
Retracted Article: The PI3K/Akt and NF-κB signaling pathways are involved in the protective effects of Lithocarpus polystachyus (sweet tea) on APAP-induced oxidative stress injury in mice
Received
2nd January 2020
, Accepted 25th April 2020
First published on 12th May 2020
Abstract
Acetaminophen (APAP)-induced acute liver injury (ALI) is a health issue that has gradually attracted attention, and is often regarded as a model of drug-induced hepatotoxicity. The leaves of Lithocarpus polystachyus Rehd. (named as “sweet tea”, ST) usually serve as tea drink and folk medicine for healthcare in the southwest part of China. In previous reports, it has been proven to protect various animal models, except for APAP-induced liver injury model. Therefore, this study initially explored the protective effect of ST leaf extract (STL-E) on hepatotoxicity induced by APAP in ICR mice. STL-E of 50 and 100 mg kg−1 were given to each group for 7 days. ALI was intraperitoneally induced by APAP treatment (i.p. 250 mg per kg body weight). Biochemical markers, levels of inflammatory factors, histopathological staining and western blotting were used to analyze the inflammation and apoptosis of liver tissues. Interestingly, the treatment with STL-E significantly attenuated APAP-induced liver injury (p < 0.05). Moreover, STL-E partially mitigated APAP-induced liver injury by effectively activating the PI3K/Akt pathway and inhibiting the NF-κB pathway. In a word, STL-E protected liver against APAP-induced hepatotoxicity by inhibiting the PI3K/Akt-mediated apoptosis signal pathway and inhibiting the NF-κB-mediated signaling pathway.
1. Introduction
The liver is an important organ for metabolism, which has several functions including glycogen conservation, red cell decomposition, plasma protein synthesis and detoxification.1 On most occasions, liver injury is an extensively spread pathology involving a gradual evolution of oxidative stress and steatosis in chronic hepatitis, fibrosis, cirrhosis, and hepatocellular carcinoma.2 Acetaminophen (APAP) is a widely used over-the-counter analgesic for pain and fever relief. However, APAP can cause severe liver injury and can develop fulminant hepatic failure when applied at high doses or at lower doses to particularly sensitive people.3 It is a known fact that mitochondrial dysfunction occurs when active metabolites are produced.4–6 Abundant evidences indicate that the depletion of hepatic glutathione and the covalent attachment of N-acetyl-p-benzoquinone imine to cellular macromolecules promote protein modification and mitochondrial function through ATP depletion, which ultimately leads to a large amount of massive centrilobular necrosis.7–9
Lithocarpus polystachyus Rehd. is mainly distributed in wild mountainous terrain which is rich in plant resources.10,11 It has the popular name “sweet tea” (ST) in folk medicine and serves as a traditional herbal medicine against various diseases.12–14 The tender leaves of L. polystachyus have been used as a traditional Chinese herb and a sweet tonic drink for several hundreds of years in southern China.15 In searching for the sweet components in the leaves, flavonoid with a content up to 7% in the leaves16,17 was determined as a sweet compound, among which trilobatin is also one of the main sweet sources.18 These components exert extensive pharmacological activities, such as anti-diabetes,19–21 memory improvement, anti-aging,22–24 inhibition of lipid peroxidation,24 and anti-cancer.25
Although people in China have been accustomed to using Chinese medicine to prevent liver diseases for several decades,3,26 there have been no pharmacological studies of hepatoprotective effects of ST leaf extract (STL-E) on APAP-induced liver injury in experimental animals to date. Therefore, the current work was designed to investigate the possible anti-inflammatory and anti-apoptotic effects of STL-E in APAP-induced acute liver injury (ALI).
2. Materials
2.1. Plant materials
Tender leaves of L. polystachyus were obtained from Hunan Funong Sweet Tea Co. Ltd, Zhijiang County, Hunan Province and identified by Professor Wei Li, College of Chinese Medicinal Materials, Jilin Agricultural University.
2.2. Reagents
APAP (purity ≥ 99%, HPLC method) was supplied from Sigma Chemicals (St. Louis, MO, USA). Hematoxylin and eosin (H&E) and Hoechst 33258 dye kits were obtained from Beyotime Co. Ltd (Shanghai, China). Immunofluorescence staining and TUNEL kits were purchased from BOSTER Biological Technology (Wuhan, China) and Roche Applied Science (Shanghai, China). The antibodies of rabbit monoclonal anti-mouse PI3K, p-PI3K, Bax, Bcl-2, caspases 3 and 9 and cleaved-caspases 3 and 9, NF-κB, IKKα/β, IκBα and β-actin were recruited from Cell Signaling Technology (Danvers, MA, USA) and BOSTER Biological Technology (Wuhan, China). All other chemicals were the highest grade commercial chemicals.
2.3. Sample preparation
Ultrasound-assisted extraction was used to extract 100 g of powdery leaves with 100% ethanol in a 45 °C water bath three times. Then, the crude extract (STL-E) was obtained by concentrated evaporation and freeze-drying.27 Trilobatin, a major and representative compound in STL-E, was analyzed by using HPLC analysis on a Waters e2695 system with a UV detector. HPLC analysis was performed through a hyper alloy ODS2 column (250 × 4.6 mm, 5 μm) with the following chromatographic conditions: column temperature of 30 °C, flow rate of 1.0 ml min−1, detection at 280 nm, and mobile phase of acetonitrile (A) and water (B). The chromatographic peak of trilobatin was confirmed by its retention time compared to a reference standard containing trilobatin. The peak integrals were quantified using an external standard method. The content of trilobatin in STL-E was determined as 81.6%. The chemical structure of trilobatin and the HPLC chromatogram of STL-E are shown in Fig. 1.
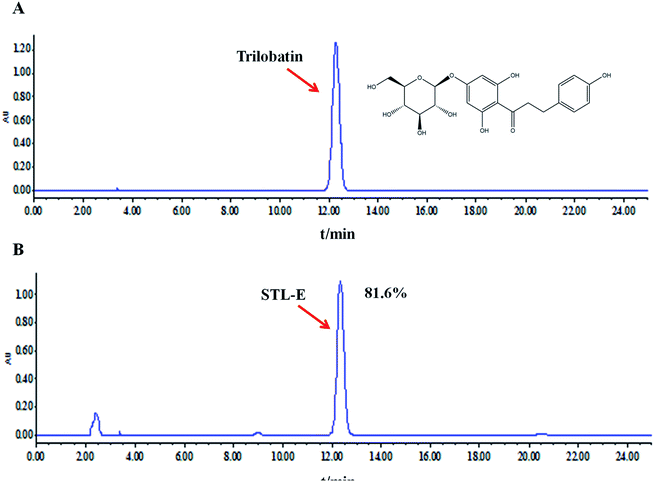 |
| Fig. 1 The chemical structure of trilobatin (A) and HPLC analysis of trilobatin in STL-E (B). | |
2.4. Animals
Male ICR mice (8 weeks old), weighing 22 ± 3 g, were purchased from Changchun Yisi Experimental Animal Holding with a Certificate of Quality No. SCXK (JI)-2018-0002 (Changchun, China). The animals were housed for 1 week with a 12 hour light/dark cycle in a temperature- and humidity-controlled room. The animals were given free access to food and water. All animals were cared for according to the Guiding Principle in the Care and Use of Animals. All experimental procedures were approved by the Ethical Committee for Laboratory Animals at Jilin Agricultural University.
2.5. Animal treatment and experimental design
All experimental animals were randomly divided into four groups (n = 8). (1) Normal group; (2) APAP (250 mg kg−1) group; (3) APAP + STL-E (50 mg kg−1) group; (4) APAP + STL-E (100 mg kg−1) group. The dosages of STL-E and APAP were based on other previous studies.28,29 STL-E was prepared using a suspension of 0.05% (w/v) sodium carboxyl methyl cellulose (CMC-Na). STL-E was intragastrically administered to the treatment group for 7 days, and the normal group and the APAP group were treated with 0.9% physiological saline. After the final administration, APAP and APAP + STL-E treatment mice were intraperitoneally injected with APAP at a dose of 250 mg kg−1 to induce ALI. At the same time, normal mice were given 0.9% physiological saline in the same manner. After fasting 12 hours, all the mice peritoneal injection of APAP, during fasting to allow free water. Next, all mice died of cervical dislocation after a single injection of APAP for 24 h. The liver tissue was quickly dissected, and the separated liver was washed twice with saline, blotted dry with filter paper, weighed, and observed for shape, size, etc. A small piece of liver tissue from the left lobe of the liver was fixed in 10% (w/v) buffered formalin solution for histopathological analysis and the remaining liver tissues were stored at −80 °C.
2.6. Serological determinations
Serum ALT and AST (Nanjing Jiancheng Biotechnology Research Institute, Nanjing) and TNF-α (Minneapolis, MN, USA) activities were determined using commercial kits, according to the manufacturer's instructions (Bio-Rad, Hercules, CA, USA) as specified in the protocol provided under 450 nm conditions.
2.7. Estimation of lipid peroxidation
The oxidation kits of GSH and MDA were purchased from Nanjing Jiancheng Biotechnology Research Institute, analyzed using commercial reagent kits according to the manufacturer's instructions (Bio-Rad, Hercules, CA, USA). The absorbance of each sample was measured at 532 nm.
2.8. Histopathology and immunofluorescence staining
Experimental methods and procedures were as described previously.30 The histopathological examination was performed with H&E staining. Apoptotic cells were measured by TUNEL staining and Hoechst 33258 staining. Antibody for TNF-α (1
:
200) was purchased from Cell Signaling, and IgA (abs120195) was obtained from (BOSTER, Wuhan, China) for immunofluorescence staining. Sections were of approximately 5 μm in thickness and the liver histology of mice was studied under a light microscope (Leica DM2500, Shanghai). At the same time, the degree of hepatocyte inflammation and necrosis was assessed by the liver injury score method.31 Partial quantified data were analysised using with Image-Pro plus 6.0 (Media Cybernetics, Rockville, MD, USA).
2.9. Western blotting analysis
Western blotting analysis was performed as previously described in our laboratory.32 The antibody dilution ratios of PI3K, p-PI3K, Akt, p-Akt, caspase 3, cleaved-caspase 3, caspase 9, cleaved-caspase 9, Bax, Bcl-2, β-actin, IKKα, IKKβ, p-IKKα/β, IκBα, p-IκBα, IκBα, NF-κB and p-NF-κB were (1
:
2000) with Ultra Signal Hypersensitive ECL Western Blotting Substrate (Pierce Chemical Co., Rockford, IL, USA).
2.10. Statistical analysis
The data are expressed as the mean ± SD. Statistical analyses were performed by using one-way ANOVA followed by the two-tailed test. Statistical significance was defined as p < 0.05 or p < 0.01.
3. Results
3.1. Effects of STL-E on body weights and organ indices in mice
As shown in Table 1, the body weight of mice was significantly reduced 24 h after injection of 250 mg per kg APAP, compared with the normal group (p < 0.05). However, the change was significantly reversed (p < 0.01, p < 0.05) in the two treatment groups of STL-E (50 and 100 mg kg−1).
Table 1 Effects of STL-E on body weight and organ index in micea
Group |
Dosage (mg kg−1) |
Body weight (g) |
Liver index (mg g−1) |
Initial |
Final |
Values represent the mean ± SD, n = 8. *p < 0.05 vs. normal group; #p < 0.05, ##p < 0.01 vs. APAP group. |
Normal |
— |
28.51 ± 1.50 |
32.98 ± 1.75 |
5.64 ± 0.34 |
APAP |
250 |
28.70 ± 1.62 |
27.65 ± 1.45* |
6.29 ± 0.24* |
APAP + ST-E |
50 |
28.32 ± 1.64 |
31.42 ± 1.85# |
5.72 ± 0.63# |
APAP + ST-E |
100 |
28.16 ± 1.38 |
31.85 ± 1.56# |
5.69 ± 0.32## |
3.2. Effects of STL-E on liver enzymes and oxidative stress
As shown in Fig. 2, serum levels of ALT and AST in the APAP group significantly increased compared with those in the normal group (p < 0.01), indicating severe liver injury. In the mice treated with STL-E, the levels of ALT and AST were significantly reduced as compared with the APAP group. The pretreatment of STL-E alleviated the APAP-induced elevation of the two transaminases (Fig. 2A and B).
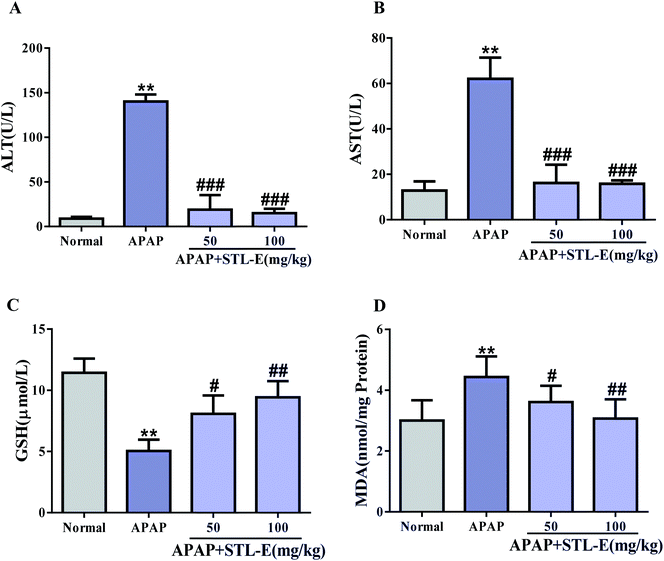 |
| Fig. 2 Pretreatment with STL-E protecting against APAP-induced ALI. Serum ALT and AST activities (A and B). The hepatic levels of GSH and MDA (C and D). All data are expressed as mean ± SD, n = 8. *p < 0.05, **p < 0.01 vs. normal group; #p < 0.05, ##p < 0.01 vs. APAP group. | |
The activity of GSH was measured by a commercial reagent kit. As shown in Fig. 2C, APAP treatment caused a significant decrease in GSH levels in liver homogenate, compared with normal mice. However, STL-E supplementation inhibited the depletion of hepatic GSH content resulting from APAP injection (Fig. 2C) (p < 0.01). Oxidative damage was assessed by determining the production level of MDA. As the final product of lipid peroxidation, MDA was considerably enhanced in the liver of APAP-treated mice. However, STL-E pretreatment significantly inhibited such increase (Fig. 2D) (p < 0.01). In conclusion, STL-E could further protect against APAP-induced hepatotoxicity by regulating the level of oxidative markers to reduce oxidative stress.
3.3. Effects of STL-E on pathological changes of liver tissues in mice
As shown in Fig. 3A, hepatocytes were evenly distributed, with complete cytoskeleton and intact morphology in the normal group. On the contrary, the liver injected with APAP showed cellular tissue disorder and cytoskeleton damage, and was accompanied by inflammatory infiltration and necrosis. Interestingly, the mice pretreated with STL-E had significant improvement of liver histopathology in comparison to the APAP-exposed group. Histopathological change and necrosis score also clearly showed that STL-E pretreatment could alleviate APAP-induced liver lesions (p < 0.01) (Fig. 3D). The liver morphology of APAP-exposed mice pretreated with high dose of STL-E (100 mg kg−1) was similar to that of normal mice. Therefore, STL-E pretreatment could effectively lessen inflammation and necrotic cells, increase normal cells, and enhance the liver pathological change resulting from APAP exposure.
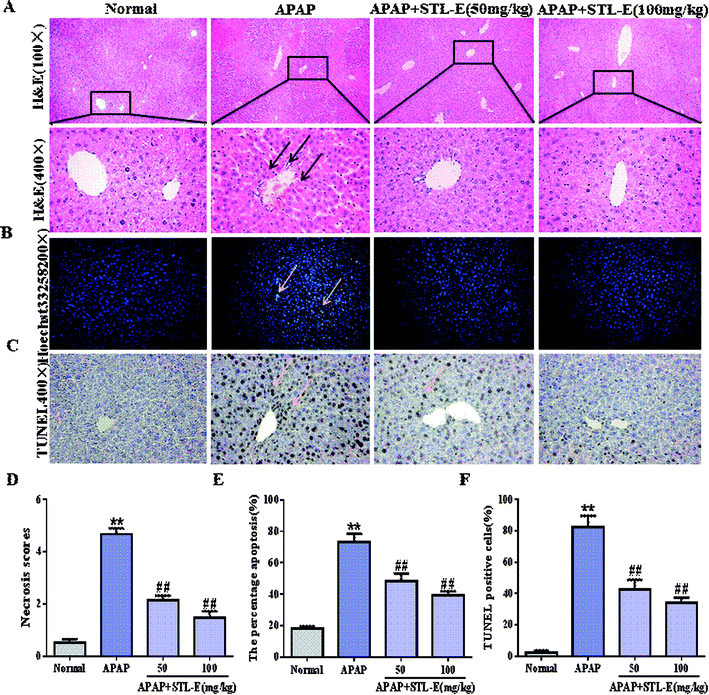 |
| Fig. 3 Histological examination of morphological changes in liver tissues. (A) Liver tissues were stained with H&E (100×, 400×). In the H&E staining, yellow arrows indicate hepatocyte necrosis and inflammatory cell infiltration. (D) The scores representing the approximate extent of necrotisis around the central veins. 0 = no damage, 1 = 0–10%, 2 = 11–25%, 3 = 26–45%, 4 = 46–75%, 5 = >75%. (B and C) Liver tissues were stained with Hoechst 33258 (200×) and TUNEL (400×); yellow arrows indicate apoptotic cells. (E and F) Percentage of Hoechst 33258 positive cells and TUNEL positive cells were evaluated by an image analyzer. All data are expressed as mean ± SD, n = 8. *p < 0.05, **p < 0.01 vs. normal group; #p < 0.05, ##p < 0.01 vs. APAP group. | |
3.4. Inhibitory effects of STL-E on apoptotic molecular expression in mice
To verify the inhibition of STL-E on APAP-induced hepatocyte apoptosis, Hoechst 33258 and TUNEL staining were adopted to analyze changes in nuclear morphology. As shown in Fig. 3B and C, the nucleus was intact, evenly distributed, neatly arranged, with clear edges and uniform chromatin staining in the normal group. In the APAP group, nuclear contraction, chromatin condensation, and high fluorescence intensity after APAP injection indicated serious apoptosis. STL-E pretreatment could significantly inhibit nuclear morphological change and attenuate fluorescence intensity. As shown in Fig. 3E and F, the result of TUNEL staining showed that APAP exposure resulted in an increase of positive hepatocyte numbers, while the APAP + STL-E group tended to show alleviation of this condition, indicating that STL-E effectively reduced APAP-induced nuclear apoptosis to a certain extent.
Since PI3K/Akt may be involved in the apoptotic mechanism of regulating apoptotic cytokine transcription, the apoptotic degrees of hepatocytes in all experimental groups were quantitatively analyzed by measuring the expression levels of the PI3K/Akt signaling pathway-related proteins, downstream pro-apoptotic factor Bax, and anti-apoptotic factor Bcl-2. The expressions of p-PI3K and p-Akt were reduced significantly after APAP exposure (p < 0.05 or p < 0.01). However, one week of STL-E pretreatment dramatically reversed this situation. In addition, APAP treatment markedly increased the protein expression levels of Bax, cleaved-caspase 3 and cleaved-caspase 9, and reduced Bcl-2 protein expression levels in liver tissues.33 Fortunately, one week of STL-E supplementation could lessen the occurrence of apoptosis by improving the expressions of caspase family proteins. All the above results verified that STL-E exerted a repressive effect on APAP-induced liver cell apoptosis (Fig. 4A and C).
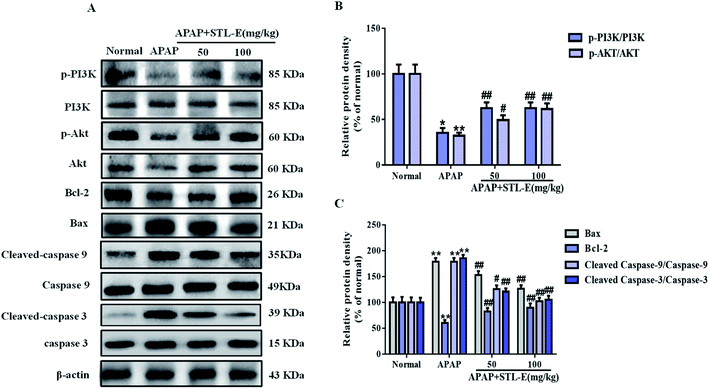 |
| Fig. 4 Effects of STL-E against APAP-induced liver toxicity by regulating the apoptosis pathway. (A) The protein expressions of p-PI3K, PI3K, p-Akt, Akt, Bax and Bcl-2 were measured by western blotting with specific primary antibodies, and GAPDH protein level was used as a loading normal. (B and C) Quantification of relative protein expression was performed by densitometric analysis. All data are expressed as mean ± SD, n = 8. *p < 0.05, **p < 0.01 vs. normal group; #p < 0.05, ##p < 0.01 vs. APAP group. | |
3.5. STL-E inhibited inflammation response after APAP treatment
Currently, inflammation is still often considered to be the cause of liver tissue damage. In this study, the expression of TNF-α in liver tissue was verified by immunofluorescence staining. APAP exposure enhanced the expression of TNF-α, compared to the normal group. As shown in Fig. 5A and B, the expression level of TNF-α induced by APAP was mitigated by STL-E pretreatment (p < 0.05, p < 0.01). As shown in Fig. 5C, serum TNF-α level in mice with elevated APAP treatment was higher than that in the normal group (p < 0.05 or p < 0.01). However, the TNF-α level was remarkably decreased with STL-E treatment in mice (p < 0.05 or p < 0.01).
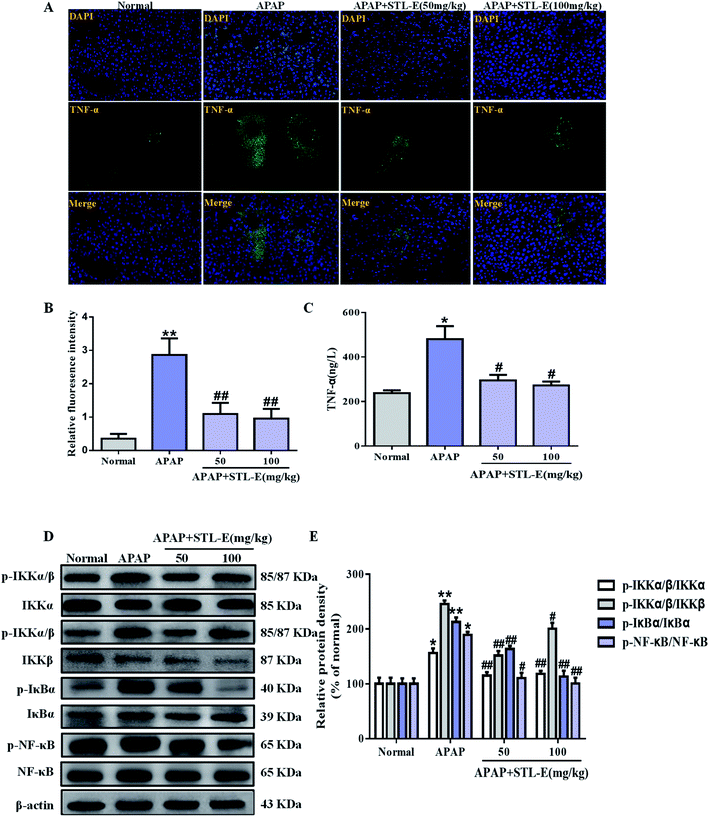 |
| Fig. 5 Effects of STL-E on APAP-induced liver toxicity by regulating the inflammation response. (A) The expression level of NF-κB (green) in tissue section isolated from different groups was evaluated with immunofluorescence. Representative immunofluorescence images were taken at 400×; 4′,6-diamidino-2-phenylindole (DAPI) (blue) acted as a nuclear counterstain. (B) Column chart showing relative fluorescence intensity. (C) Serum TNF-α activity. (D) The protein expressions of phosphorylated and total IKKα, IKKβ and NF-κB were measured by western blotting with specific primary antibodies, and GAPDH protein level was used as a loading normal. (E) Quantification of relative protein expression was performed by densitometric analysis. Data are expressed as mean ± SD, n = 8. *p < 0.05, **p < 0.01 vs. normal group; #p < 0.05, ##p < 0.01 vs. APAP group. | |
The NF-κB signaling pathway plays an important role in inflammation, immunity, cell proliferation, and apoptosis, especially in an animal model of APAP-induced hepatotoxicity. So, the protein level was analyzed in this study. APAP exposure increased the phosphorylated protein expression of NF-κB and its upstream regulatory factors, IKKα/β and IκBα (p < 0.01). As expected, STL-E supplementation considerably inhibited NF-κB phosphorylation, IKKα/β and IκBα phosphorylation (p < 0.05, p < 0.01), which was essentially protective against APAP-induced liver damage (Fig. 5D and E). The result showed that STL-E inhibited APAP-induced hepatotoxicity by restraining the inflammatory response.
4. Discussion
Multiple liver necrosis secondary to APAP-induced ALI is a rapidly progressive clinical syndrome which often causes multiple organ failure. As a traditional Chinese medicine, the leaves of L. polystachyus have served as a sweet tonic for centuries.34 Current pharmacological researches suggest that L. polystachyus has a preventive effect against hypertension and has significant protective activity for type 2 and type 1 diabetes.17,28 Recently, the favorable effects of trilobatin on anti-oxidation and anti-inflammation were explained by researchers.35 Oxidative stress is a major target of drug toxicity.36 The levels of MDA and GSH respectively indicated the degree of oxidative damage and lipid peroxidation.37 The results of this study proved that APAP overdose led to oxidative stress in liver tissue by reducing GSH levels and increasing MDA levels, which was consistent with many previous reports.38,39 Intriguingly, STL-E remarkably improved this situation. This result indicated that STL-E protected the liver from oxidative stress by inhibiting ROS production and lipid/protein peroxidation (Fig. 6).
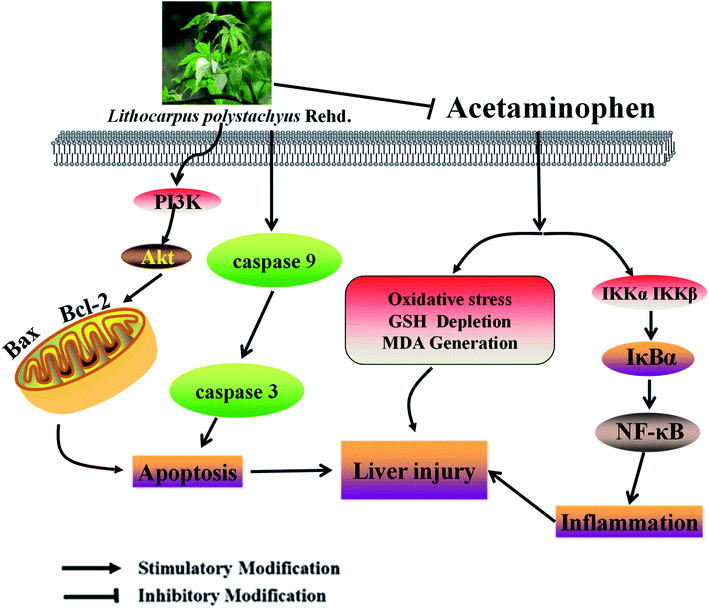 |
| Fig. 6 A schematic diagram of molecular mechanisms underlying ameliorative effects of STL-E against APAP-induced hepatotoxicity. | |
More and more evidence suggests that APAP-induced ALI is associated with hepatocyte necrosis, inflammation and apoptosis.40 The expression of inflammatory pathway NF-κB was assessed by western blotting analysis to further verify that STL-E could inhibit APAP-induced inflammation of liver tissue. The NF-κB pathway is generally regarded as a typical pro-inflammatory signal transduction pathway, which is based primarily on the activation of pro-inflammatory cytokines such as TNF-α. NF-κB is located in the cytosol bound to the effector of IκBα. Once activated, NF-κB might cause various biological events, such as cytokine transcription and inflammatory responses.41 Therefore, maintaining the stability of NF-κB/IκBα or inhibiting the release of NF-κB and IκBα in hepatocytes might promote the lessening of APAP-induced liver injury. The study by Liu et al. indicated that saikosaponin D protected mice from APAP-induced hepatotoxicity mainly by downregulating NF-κB and Stat3-mediated inflammatory signals,42 and Liang et al. also reported that urantide had a protective effect on the acutely inflamed injury of partial liver by preventing the release of pro-inflammatory cytokines and the activation of the NF-κB pathway.43 In this study, it was found that STL-E could effectively inhibit the occurrence of such a situation. Western blotting analysis showed that STL-E lessened the generation of NF-κB signals by inhibiting the activation of IKKα, IKKβ and IκBα. Accordingly, it could be concluded that STL-E inhibited NF-κB bioactivity from IκBα, then decreased and eventually prevented TNF-α release due to the APAP-induced inflammatory response of liver-injured mice.
Increasing evidence has demonstrated that APAP induces translocation of Bcl-2 family proteins44 and the expression of increasing positive cells for TUNEL assay.45 Thus, the effect of STL-E on APAP-induced apoptotic signals was examined and the protein expression of PI3K/Akt signaling pathway and the downstream protein were evaluated by western blotting analysis. The activation of such pathway plays a vital role in essential cellular functions such as survival, proliferation, migration and differentiation which underlie the biology of human cancer. Activated Akt stimulated or inhibited its downstream target proteins such as Bcl-2 and Bax to further regulate cell proliferation and apoptosis.46–48 The result demonstrated that APAP downregulated Bcl-2 and upregulated Bax, cleaved-caspase 9 and cleaved-caspase 3 in hepatocytes, and related protein expression in the PI3K/Akt signaling pathway was suppressed. The activity was close to normal level after STL-E pretreatment. This result was also consistent with those of previous reports.49,50
5. Conclusions
In brief, the findings of the present work have confirmed that STL-E exerts mitigation effects of oxidative stress damage and the inhibition of inflammation and apoptosis of APAP-induced ALI in mice. STL-E treatment mechanism involves the activation of the PI3K/Akt-mediated apoptosis signaling pathway and the decline of the NF-κB signaling pathway. Therefore, STL-E treatment can be used as a potential therapeutic strategy to prevent ALI from APAP overdose. In addition, due to the extremely high level of trilobatin in STL-E, it is proposed that trilobatin may also have a protective effect against APAP-induced liver injury, which is worth further exploration in the future.
Conflicts of interest
The authors declare no conflicts of interest.
Abbreviations
APAP | Acetaminophen |
Akt | Protein kinase B |
PI3K | Phosphatidylinositol-3-kinase |
Bax | Bcl-2 associated X protein |
Bcl-2 | B-cell-lymphoma-2 |
NF-κB | Nuclear factor-kappa B |
IκB | Inhibitor of nuclear factor kappa-B |
IKK (IκBk) | IκB kinase |
TNF-α | Tumor necrosis factor-α |
ALT | Alanine aminotransferase |
AST | Aspartate aminotransferase |
MDA | Malondialdehyde |
GSH | Glutathione |
Acknowledgements
This work was supported by the National Natural Science Foundation of China (no. 81860691) and “13th five year plan” scientific research planning project of Jilin Provincial Department of Education (no. JJKH20190946KJ).
References
- C. L. Fu, Y. Liu, J. Leng, J. Zhang, Y. F. He, C. Chen, Z. Wang and W. Li, Platycodin D protects acetaminophen-induced hepatotoxicity by inhibiting hepatocyte MAPK pathway and apoptosis in C57BL/6J mice, Biomed. Pharmacother., 2018, 107, 867–877 CrossRef CAS PubMed.
- H. Liang, Y. Feng, R. Cui, M. Qiu, J. Zhang and C. Liu, Simvastatin protects against acetaminophen-induced liver injury in mice, Biomed. Pharmacother., 2018, 98, 916–924 CrossRef CAS PubMed.
- K. Wu, J. Fan, X. Huang, X. Wu and C. Guo, Hepatoprotective effects exerted by Poria Cocos polysaccharides against acetaminophen-induced liver injury in mice, Int. J. Biol. Macromol., 2018, 114, 137–142 CrossRef CAS PubMed.
- A. O. Abdel-Zaher, R. H. Abdel-Hady, M. M. Mahmoud and M. M. Farrag, The potential protective role of alpha-lipoic acid against acetaminophen-induced hepatic and renal damage, Toxicology, 2008, 243(3), 261–270 CrossRef CAS PubMed.
- P. Zhao, T. F. Kalhorn and J. T. Slattery, Selective mitochondrial glutathione depletion by ethanol enhances acetaminophen toxicity in rat liver, Hepatology, 2002, 36(2), 326–335 CrossRef CAS PubMed.
- S. Arakawa, T. Maejima, K. Fujimoto, T. Yamaguchi, M. Yagi, T. Sugiura, R. Atsumi and Y. Yamazoe, Resistance to acetaminophen-induced hepatotoxicity in glutathione S-transferase Mu 1-null mice, J. Toxicol. Sci., 2012, 37(3), 595–605 CrossRef CAS PubMed.
- H. Zaher, J. T. Buters, J. M. Ward, M. K. Bruno, A. M. Lucas, S. T. Stern, S. D. Cohen and F. J. Gonzalez, Protection against acetaminophen toxicity in CYP1A2 and CYP2E1 double-null mice, Toxicol. Appl. Pharmacol., 1998, 152(1), 193–199 CrossRef CAS PubMed.
- S. S. Kalsi, P. I. Dargan, W. S. Waring and D. M. Wood, A review of the evidence concerning hepatic glutathione depletion and susceptibility to hepatotoxicity after paracetamol overdose, Open Access Emerg. Med., 2011, 3, 87–96 Search PubMed.
- A. Canbay, C. Jochum, L. P. Bechmann, S. Festag, R. K. Gieseler, Z. Yuksel, P. Lutkes, F. H. Saner, A. Paul and G. Gerken, Acute liver failure in a metropolitan area in Germany: a retrospective study (2002 - 2008), Z. Gastroenterol., 2009, 47(9), 807–813 CrossRef CAS PubMed.
- X. Li, Y. Zhao, S. Huang, W. Song, X. Zeng, S. Z. Hou and X. P. Lai, New dihydrochalcone and propenamide from Lithocarpus polystachyus, Nat. Prod. Commun., 2014, 9(5), 653–654 CrossRef CAS PubMed.
- R. N. Ning, H. M. Wang, Y. Shen, Z. H. Chen, R. J. Zhang, Y. Leng and W. M. Zhao, Lithocarpic acids O-S, five homo-cycloartane derivatives from the cupules of Lithocarpus polystachyus, Bioorg. Med. Chem. Lett., 2014, 24(23), 5395–5398 CrossRef CAS PubMed.
- W. T. Chang, W. C. Huang and C. J. Liou, Evaluation of the anti-inflammatory effects of phloretin and phlorizin in lipopolysaccharide-stimulated mouse macrophages, Food Chem., 2012, 134(2), 972–979 CrossRef CAS PubMed.
- C. J. Zhou, S. Huang, J. Q. Liu, S. Q. Qiu, F. Y. Xie, H. P. Song, Y. S. Li, S. Z. Hou and X. P. Lai, Sweet tea leaves extract improves leptin resistance in diet-induced obese rats, J. Ethnopharmacol., 2013, 145(1), 386–392 CrossRef CAS PubMed.
- F. B. Zhu, J. Y. Wang, Y. L. Zhang, Y. G. Hu, Z. S. Yue, L. R. Zeng, W. J. Zheng, Q. Hou, S. G. Yan and R. F. Quan, Mechanisms underlying the antiapoptotic and anti-inflammatory effects of monotropein in hydrogen peroxide-treated osteoblasts, Mol. Med. Rep., 2016, 14(6), 5377–5384 CrossRef CAS PubMed.
- G. Y. Koh, K. McCutcheon, F. Zhang, D. Liu, C. A. Cartwright, R. Martin, P. Yang and Z. Liu, Improvement of obesity phenotype by Chinese sweet leaf tea (Rubus suavissimus) components in high-fat diet-induced obese rats, J. Agric. Food Chem., 2011, 59(1), 98–104 CrossRef CAS PubMed.
- X. Li, Y. Zhao, S. Hou, S. Huang, W. Yang, X. Lai and X. Zeng, Identification of the bioactive components of orally administered Lithocarpus polystachyus Rehd and their metabolites in rats by liquid chromatography coupled to LTQ Orbitrap mass spectrometry, J. Chromatogr. B: Anal. Technol. Biomed. Life Sci., 2014, 962, 37–43 CrossRef CAS PubMed.
- S. Z. Hou, S. J. Xu, D. X. Jiang, S. X. Chen, L. L. Wang, S. Huang and X. P. Lai, Effect of the flavonoid fraction of Lithocarpus polystachyus Rehd. on spontaneously hypertensive and normotensive rats, J. Ethnopharmacol., 2012, 143(2), 441–447 CrossRef CAS PubMed.
- Y. Sun, Z. Chen, J. Li, J. Li, H. Lv, J. Yang, W. Li, D. Xie, Z. Xiong, P. Zhang and Y. Wang, Diterpenoid UDP-Glycosyltransferases from Chinese Sweet Tea and Ashitaba Complete the Biosynthesis of Rubusoside, Mol. Plant, 2018, 11(10), 1308–1311 CrossRef CAS PubMed.
- H. Y. Gaisano, C. G. Ostenson, L. Sheu, M. B. Wheeler and S. Efendic, Abnormal expression of pancreatic islet exocytotic soluble N-ethylmaleimide-sensitive factor attachment protein receptors in Goto-Kakizaki rats is partially restored by phlorizin treatment and accentuated by high glucose treatment, Endocrinology, 2002, 143(11), 4218–4226 CrossRef CAS PubMed.
- R. J. McCrimmon, M. L. Evans, R. J. Jacob, X. Fan, Y. Zhu, G. I. Shulman and R. S. Sherwin, AICAR and phlorizin reverse the hypoglycemia-specific defect in glucagon secretion in the diabetic BB rat, Am. J. Physiol.: Endocrinol. Metab., 2002, 283(5), E1076–E1083 CrossRef CAS PubMed.
- H. Zhao, S. Yakar, O. Gavrilova, H. Sun, Y. Zhang, H. Kim, J. Setser, W. Jou and D. LeRoith, Phloridzin improves hyperglycemia but not hepatic insulin resistance in a transgenic mouse model of type 2 diabetes, Diabetes, 2004, 53(11), 2901–2909 CrossRef CAS PubMed.
- J. L. Hall, R. T. Reilly, K. L. Cottrill, W. S. Stone and P. E. Gold, Phlorizin enhancement of memory in rats and mice, Pharmacol., Biochem. Behav., 1992, 41(2), 295–299 CrossRef CAS.
- L. Xiang, K. Sun, J. Lu, Y. Weng, A. Taoka, Y. Sakagami and J. Qi, Anti-aging effects of phloridzin, an apple polyphenol, on yeast via the SOD and Sir2 genes, Biosci., Biotechnol., Biochem., 2011, 75(5), 854–858 CrossRef CAS PubMed.
- T. Ridgway, J. O'Reilly, G. West, G. Tucker and H. Wiseman, Antioxidant action of novel derivatives of the apple-derived flavonoid phloridzin compared to oestrogen: relevance to potential cardioprotective action, Biochem. Soc. Trans., 1997, 25(1), 106S CrossRef CAS PubMed.
- K. Ugocsai, A. Varga, P. Molnar, S. Antus and J. Molnar, Effects of selected flavonoids and carotenoids on drug accumulation and apoptosis induction in multidrug-resistant colon cancer cells expressing MDR1/LRP, In Vivo, 2005, 19(2), 433–438 CAS.
- K. J. Lee, H. J. You, S. J. Park, Y. S. Kim, Y. C. Chung, T. C. Jeong and H. G. Jeong, Hepatoprotective effects of Platycodon grandiflorum on acetaminophen-induced liver damage in mice, Cancer Lett., 2001, 174(1), 73–81 CrossRef CAS PubMed.
- Y. Z. Li, S. Ren, X. T. Yan, H. P. Li, W. Li, B. Zheng, Z. Wang and Y. Y. Liu, Improvement of Cisplatin-induced renal dysfunction by Schisandra chinensis stems via anti-inflammation and anti-apoptosis effects, J. Ethnopharmacol., 2018, 217, 228–237 CrossRef CAS PubMed.
- J. Zhang, J. Y. Zeng and M. Tang, Effects of different liquid extracts from polypanicle on MDA/CAT activity in diabetic mice, Hubei Agricultural Sciences, 2012, 51(02), 354–357 Search PubMed.
- J. Leng, Z. Wang, C. L. Fu, J. Zhang, S. Ren, J. N. Hu, S. Jiang, Y. P. Wang, C. Chen and W. Li, Phytother. Res., 2018, 32, 2235–2246 CrossRef CAS PubMed.
- W. Zhang, J. Hou, X. Yan, J. Leng, R. Li, J. Zhang, J. Xing, C. Chen, Z. Wang and W. Li, Platycodon grandiflorum Saponins Ameliorate Cisplatin-Induced Acute Nephrotoxicity through the NF-kappaB-Mediated Inflammation and PI3K/Akt/Apoptosis Signaling Pathways, Nutrients, 2018, 10(9), 1328 CrossRef PubMed.
- X. J. Mi, J. G. Hou, S. Jiang, Z. Liu, S. Tang, X. X. Liu, Y. P. Wang, C. Chen, Z. Wang and W. Li, Maltol Mitigates Thioacetamide-induced Liver Fibrosis through TGF-beta1-mediated Activation of PI3K/Akt Signaling Pathway, J. Agric. Food Chem., 2019, 67(5), 1392–1401 CrossRef CAS PubMed.
- R. Y. Li, W. Z. Zhang, X. T. Yan, J. G. Hou, Z. Wang, C. B. Ding, W. C. Liu, Y. N. Zheng, C. Chen, Y. R. Li and W. Li, Arginyl-fructosyl-glucose, a Major Maillard Reaction Product of Red Ginseng, Attenuates Cisplatin-Induced Acute Kidney Injury by Regulating Nuclear Factor kappaB and Phosphatidylinositol 3-Kinase/Protein Kinase B Signaling Pathways, J. Agric. Food Chem., 2019, 67(20), 5754–5763 CAS.
- X. T. Yan, Y. S. Sun, S. Ren, L. C. Zhao, W. C. Liu, C. Chen, Z. Wang and W. Li, Dietary alpha-Mangostin Provides Protective Effects against Acetaminophen-Induced Hepatotoxicity in Mice via Akt/mTOR-Mediated Inhibition of Autophagy and Apoptosis, Int. J. Mol. Sci., 2018, 19(5), 1335 CrossRef PubMed.
- Y. Sun, W. Li and Z. Liu, Preparative isolation, quantification and antioxidant activity of dihydrochalcones from Sweet Tea (Lithocarpus polystachyus Rehd.), J. Chromatogr. B: Anal. Technol. Biomed. Life Sci., 2015, 1002, 372–378 CrossRef CAS PubMed.
- J. Gao, S. Liu, F. Xu, Y. Liu, C. Lv, Y. Deng, J. Shi and Q. Gong, Trilobatin Protects Against Oxidative Injury in Neuronal PC12 Cells Through Regulating Mitochondrial ROS Homeostasis Mediated by AMPK/Nrf2/Sirt3 Signaling Pathway, Front. Mol. Neurosci., 2018, 11, 267 CrossRef PubMed.
- E. Song, J. Fu, X. Xia, C. Su and Y. Song, Bazhen decoction protects against acetaminophen induced acute liver injury by inhibiting oxidative stress, inflammation and apoptosis in mice, PLoS One, 2014, 9(9), e107405 CrossRef PubMed.
- T. Kamiyama, C. Sato, J. Liu, K. Tajiri, H. Miyakawa and F. Marumo, Role of lipid peroxidation in acetaminophen-induced hepatotoxicity: comparison with carbon tetrachloride, Toxicol. Lett., 1993, 66(1), 7–12 CrossRef CAS PubMed.
- C.-J. Zhou, Sweet tea leaves extract improves leptin resistance in diet-induced obese rats, J. Ethnopharmacol., 2013, 145(1), 386–392 CrossRef CAS PubMed.
- S.-z. Hou, The hypoglycemic activity of Lithocarpus polystachyus Rehd. leaves in the experimental hyperglycemic rats, J. Ethnopharmacol., 2011, 138(1), 142–149 CrossRef CAS PubMed.
- A. D. Patterson, Y. M. Shah, T. Matsubara, K. W. Krausz and F. J. Gonzalez, Peroxisome proliferator-activated receptor alpha induction of uncoupling protein 2 protects against acetaminophen-induced liver toxicity, Hepatology, 2012, 56(1), 281–290 CrossRef CAS PubMed.
- S. Ghosh and J. F. Dass, Study of pathway cross-talk interactions with NF-kappaB leading to its activation via ubiquitination or phosphorylation: A brief review, Gene, 2016, 584(1), 97–109 CrossRef CAS PubMed.
- A. Liu, N. Tanaka, L. Sun, B. Guo, J. H. Kim, K. W. Krausz, Z. Fang, C. Jiang, J. Yang and F. J. Gonzalez, Saikosaponin d protects against acetaminophen-induced hepatotoxicity by inhibiting NF-kappaB and STAT3 signaling, Chem.-Biol. Interact., 2014, 223, 80–86 CrossRef CAS PubMed.
- D. Y. Liang, L. M. Liu, C. G. Ye, L. Zhao, F. P. Yu, D. Y. Gao, Y. Y. Wang, Z. W. Yang and Y. Y. Wang, Inhibition of UII/UTR system relieves acute inflammation of liver through preventing activation of NF-kappaB pathway in ALF mice, PLoS One, 2014, 8(6), e64895 CrossRef PubMed.
- F. Tomasello, A. Messina, L. Lartigue, L. Schembri, C. Medina, S. Reina, D. Thoraval, M. Crouzet, F. Ichas, V. De Pinto and F. De Giorgi, Outer membrane VDAC1 controls permeability transition of the inner mitochondrial membrane in cellulo during stress-induced apoptosis, Cell Res., 2009, 19(12), 1363–1376 CrossRef CAS PubMed.
- M. Christina, H. L. Angelika, P. Bernd and P. Martina, Simultaneous detection of a cell surface antigen and apoptosis by microwave-sensitized TUNEL assay on paraffin sections, J. Immunol. Methods, 2006, 316(1–2), 163–166 CrossRef CAS PubMed.
- S. W. Hong, H. S. Lee, K. H. Jung, H. Lee and S. S. Hong, Protective effect of fucoidan against acetaminophen-induced liver injury, Arch. Pharmacal Res., 2012, 35(6), 1099–1105 CrossRef CAS PubMed.
- R. Grespan, R. P. Aguiar, F. N. Giubilei, R. R. Fuso, M. J. Damiao, E. L. Silva, J. G. Mikcha, L. Hernandes, C. Bersani Amado and R. K. Cuman, Hepatoprotective Effect of Pretreatment with Thymus vulgaris Essential Oil in Experimental Model of Acetaminophen-Induced Injury, Evid. Based Complementary Altern. Med., 2014, 2014, 954136 Search PubMed.
- C. Lin, L. Wang, H. Wang, S. Fang, Q. Zhang, L. Yang, H. Guo, P. Lin, J. Zhang and X. Wang, Lithocarpus polystachyus REHD leaf aqueous extract inhibits human breast cancer growth in vitro and in vivo, Nutr. Cancer, 2014, 66(4), 613–624 CrossRef PubMed.
- X. H. Zhou, M. Y. Gong and H. M. Yang, Effects of scutellaria stem-leaf total flavonoids on cardiocyte apoptosis induced by hypoxia/reoxygenation, Chin. J. Integr. Tradit. West. Med., 2011, 31(6), 803–806 Search PubMed.
- S. Ren, J. Leng, X. Y. Xu, S. Jiang, Y. P. Wang, X. T. Yan, Z. Liu, C. Chen, Z. Wang and W. Li, Ginsenoside Rb1, A Major Saponin from Panax ginseng, Exerts Protective Effects Against Acetaminophen-Induced Hepatotoxicity in Mice, Am. J. Chin. Med., 2019, 1–17 Search PubMed.
|
This journal is © The Royal Society of Chemistry 2020 |
Click here to see how this site uses Cookies. View our privacy policy here.