DOI:
10.1039/D0RA00802H
(Paper)
RSC Adv., 2020,
10, 10502-10509
Photoinduced metal-free α-selenylation of ketones†
Received
27th January 2020
, Accepted 28th February 2020
First published on 11th March 2020
Abstract
Herein, we report an efficient photoinduced α-selenylation of ketones without metal, additives or under photosensitizer-free conditions, providing a green protocol using light energy to synthesize a variety of α-selenoketones. This new methodology proved to be a mild, simple and eco-friendly tool for the efficient synthesis of the desired products.
Introduction
Nowadays, when evaluating a new synthetic approach, the choice of energy source when an organic transformation is involved is important, and in this context, photochemistry has emerged as an environmentally friendly alternative to assisting organic reactions.1 Compared with traditional methods, luminous energy-assisted reactions involve mild reaction conditions, simple operation, and a sustainable approach.2 Among these, photoinduced reactions are of great importance since activation of certain organic molecules occurs without the use of transition metal catalysts, such as ruthenium and iridium complexes or organic dyes such as Eosin Y and Rose Bengal. Photosensitizers usually have high costs and their removal produces harmful waste, which affects the environment and the economy of the reaction.3
Thus, photoinduced protocols are environmentally benign and attractive alternatives for the formation of organoselenium compounds through the construction of the light-promoted C–Se bond.4 Organoselenium compounds are of considerable importance in synthetic5 and pharmaceutical chemistry.6 Particularly, α-selenoketones have attracted the attention of synthetic chemists, due to their versatile synthetic applications in organic synthesis7 and, more recently, their biological activities for disorders such as depression and anxiety.8 Given their value in chemistry and biological systems, intensive efforts have been devoted to the synthesis of α-selenoketones. However, none of them involve the use of light energy to accomplish this transformation. Most of the α-selenofunctionalisation protocols include the following: in situ generation of nucleophilic selenium species9 (Scheme 1a) and previous formation of an electrophilic species from diselenides10 (Scheme 1b). The formation of these species usually requires the presence of metals, reducing agents or halogenated reagents.10c–e,11 In addition, to perform activation in the α position of ketones, acidic or basic media are generally used.10c,d,12 Another way of activating this position is through the use of secondary amines, such as organocatalysts, but these methodologies also require an electrophilic selenium species.10c–e,12 Moreover, these protocols for obtaining α-selenoketones have restricted reaction scopes, and are limited to different selenium-bonded substituents.
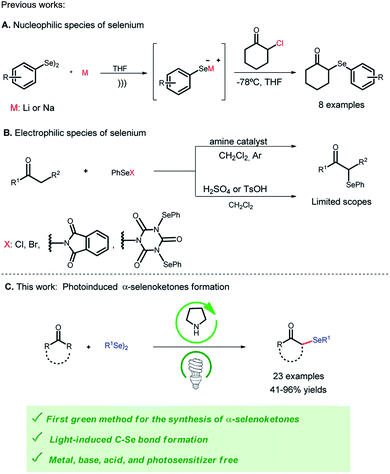 |
| Scheme 1 Previous works and our approach for α-selenylation of ketones. | |
Therefore, in continuation of our studies on the reactions for obtaining organoselenated compounds13 promoted by a photoinduced process,4d herein we demonstrate an efficient, general, simple and eco-friendly protocol for the preparation of α-selenoketones through the reaction between diselenides, ketones and pyrrolidine in catalytic amounts and in the absence of photosensitizers, halogenated reactants, additives and harsh reaction conditions (Scheme 1c).
Results and discussion
We started our investigations using diphenyl diselenide 1a and cyclohexanone 2a as model substrates, in the presence of 10 mol% pyrrolidine in THF, under CFL 27 W irradiation. With these reaction conditions, the desired α-selenoketone 3a was obtained in a conversion of only 14% (Table 1, entry 1). We then probed the effect of pyrrolidine on the reaction (Table 1, entries 1–4). Catalyst loadings, ranging from 10 to 30 mol%, were evaluated. The best result was observed when 20 mol% of pyrrolidine was used, leading to an improvement in the conversion (Table 1, entry 2). Moreover, when the reaction was conducted in the absence of pyrrolidine no product was obtained (Table 1, entry 4). Furthermore, the stoichiometry was also investigated, and an excess of diphenyl diselenide 1a or cyclohexanone 2a, did not affect the reaction (Table 1, entries 5–6). Next, we evaluated the influence of the solvent in the reaction, and different solvents such as DMSO, DCM, and MeCN, were used (Table 1, entries 7–9). MeCN and THF led to the formation of 3a in a similar manner (Table 1, entry 2 vs. 9). However, acetonitrile provided the best result in terms of environmental benefits (Table 1, entry 9). After screening the initial experimental parameters, we evaluated the effect of luminous irradiation (Table 2, entries 1–5). The use of a lower power lamp did not improve the conversion (Table 2, entry 1). Other lamps with different wavelengths were tested and we observed that the reaction in the presence of CFL UVA 26 W provided the best result, with 55% conversion (Table 2, entry 5) showing superiority for this transformation in relation to the others lamps. The presence of light was necessary for this protocol since only traces of the product were observed in the complete absence of luminous energy (Table 2, entry 5). Subsequently, the influence of the reaction time (Table 2, entries 6–10) was examined, and the best results were observed after 6 h (Table 2, entry 9), in which 61% conversion was obtained. Moreover, additives were used in the reaction with the aim of increasing the formation of the product (Table 2, entries 11–12), but neither bismuth oxide nor benzoic acid led to an increase in the yield of α-selenoketone 3a. Finally, the best condition were used under an argon atmosphere, and a decrease in conversion (26%) was observed (Table 2, entry 13). This is a valuable result because it suggests that atmospheric O2 is necessary for the formation of the product. Considering the data from Tables 1 and 2, equivalent amounts of 1a and 2a, 20 mol% pyrrolidine as organocatalyst and MeCN as the solvent, under irradiation of a CFL UVA 26 W (Table 2, entry 9) were chosen for subsequent studies. It is important mention that the diselenides are very stable, and can be recovered in the purification step, and reused in further reactions. For example, under the optimized conditions, for the synthesis of 3a, we could recover 106 mg (0.34 mmol) of the diphenyl diselenide, which is almost all the unreacted diselenide (0.38 mmol).
Table 1 Optimization of the reaction conditionsa
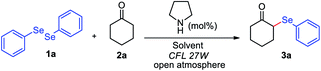
|
Entry |
Pyrrolidine (mol%) |
Solvent |
Conv.b(%) |
The reaction was performed in the presence of 1a (0.5 mmol), 2a (0.5 mmol) and pyrrolidine in the solvent (1 mL) for 20 h. Determined by GC, based on the amount of cyclohexanone 2a. 1.2 equivalents of 1a. 1.2 equivalents of 2a. |
1 |
10 |
THF |
14 |
2 |
20 |
THF |
45 |
3 |
30 |
THF |
10 |
4 |
— |
THF |
— |
5c |
20 |
THF |
42 |
6d |
20 |
THF |
40 |
7 |
20 |
DMSO |
5 |
8 |
20 |
DCM |
5 |
9 |
20 |
MeCN |
45 |
Table 2 Optimization of the reaction conditionsa
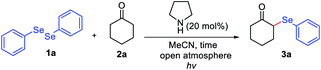
|
Entry |
Lamp |
Time (h) |
Conv.b(%) |
The reaction was performed in the presence of 1a (0.5 mmol), 2a (0.5 mmol) and pyrrolidine (20 mol%) in MeCN (1 mL). Determined by GC, based on the amount of cyclohexanone 2a. 20 mol% benzoic acid was added. 20 mol% of Bi2O3 was added. Under an argon atmosphere. |
1 |
CFL 20 W |
20 |
24 |
2 |
Blue 26 W |
20 |
20 |
3 |
Green 26 W |
20 |
10 |
4 |
CFL UVA 26 W |
20 |
55 |
5 |
— |
20 |
Traces |
6 |
CFL UVA 26 W |
1 |
12 |
7 |
CFL UVA 26 W |
2 |
37 |
8 |
CFL UVA 26 W |
4 |
48 |
9 |
CFL UVA 26 W |
6 |
61 |
10 |
CFL UVA 26 W |
8 |
50 |
11c |
CFL UVA 26 W |
6 |
60 |
12d |
CFL UVA 26 W |
6 |
55 |
13e |
CFL UVA 26 W |
6 |
26 |
Once the best experimental conditions for the synthesis of 3a were established (Table 2, entry 9), we evaluated the generality of the method concerning different diorganyldiselenides 1 and ketones 2 (Scheme 2). We first evaluated the influence of the introduction of different substituents in ortho, meta, and para positions of diaryldiselenide, as well as one dialkyldiselenide 1, while keeping cyclohexanone constant. The reaction worked well, affording the respective α-selenoketones 3a–k in good to excellent yields. To analyze the electronic effects on the reaction yield, a range of diaryldiselenides containing electron-donating groups (EDGs) and electron-withdrawing groups corresponding α-selenoketones 3b–h in excellent yields. Furthermore, introduction of various substituents in different positions of the diaryldiselenide did not affect the reaction, and the corresponding products were isolated in 67–96% yield (3b–h). No influence was observed due to the steric hindrance of ortho-substituted aryl diselenides as compared to the respective para derivatives (3b–c vs. 3e–f). The methodology was extended to sterically hindered and bulkier substrates. The selenylated products 3i–j were obtained in good yields, showing the versatility of this reaction. In addition to diaryldiselenides, dibutyldiselenide was used, and the alkylated ketone 3k was obtained after 9 h in 80% yield. This significant result reinforces the versatility of the developed methodology. Encouraged by the results of this metal-free approach for α-selenation of ketones using luminous energy and looking to expand this protocol and broaden the scope in relation to the substrate, different cyclic and acyclic ketones were reacted with diselenides. In general, the reaction furnished the respective α-selenoketones 3l–w in moderate to good yields.
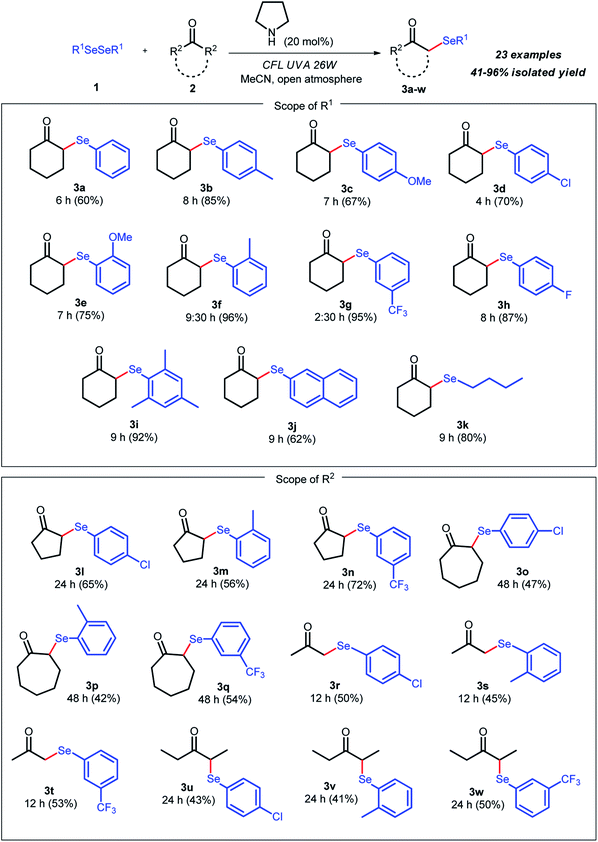 |
| Scheme 2 Scope of the photoinduced metal-free α-selenylation of ketones. | |
Different cyclic ketones, such as 5, 6, or 7-membered rings, were used, and the corresponding products were isolated in 42–72% yield (3a, 3l, and 3q). In this case, an influence related to ring size was observed. The 5-membered ketone leaded to the α-selenylated products in better yields and in shorter reaction times compared to the 7-membered ketone (3l–n vs. 3o–q). In addition, the protocol was extended to acyclic ketones, and products 3r–w were obtained in 41–53% yield. It was observed that propanone led to the desired products in similar reaction yields to 3-pentanone, however, in shorter times. The higher reaction times can be explained due to the slow formation of the intermediate enamine. It is important to point out that neither condensation adducts nor double α-phenylselenenylated products were detected in these reactions.
To evaluate the mechanism involved in the α-selenylation of ketones, some control experiments were carried out (Scheme 3). At first, 2,2,6,6-tetramethylpiperidin-1-yloxyl (TEMPO) as a radical inhibitor was added, and following our optimal experimental conditions, only traces of 3a were obtained (<10%), suggesting a possible involvement of a radical process in this reaction. An interesting observation, during the optimization of the reaction conditions (Table 2, entry 13), was that the reaction under an argon atmosphere did not work well, which imply that an oxidation step can be involved. In this way, two more experiments were performed using stronger oxidant conditions, and under an O2 atmosphere or by adding K2S2O8, a dramatical decrease in the reaction yield was observed. These lower yields observed in a more oxidizing system could be explained by the higher capture rate of selenium radicals under these conditions.14 This outcome confirms that an open atmosphere plays a crucial role, and the aerobic O2 acts as a mild oxidant, furnishing the best results.
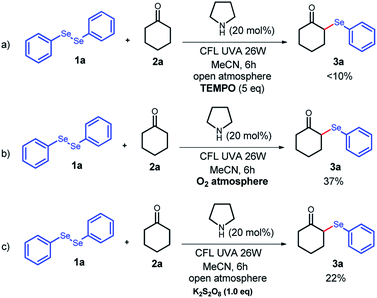 |
| Scheme 3 Control experiments. | |
On the basis of previous reports and the above results, we proposed a plausible mechanism for this transformation (Scheme 4). Enamine 4b is formed in situ from the condensation of pyrrolidine 4a with cyclohexanone 2a. The next step comprises the reversible homolytic cleavage of the Se–Se bond. The selenium radical specie adds to the enamine 4b, leading to the formation of α-selenylated intermediate 4c. The reaction is not efficiently promoted when conducted under an inert atmosphere (Table 2, entry 13), indicating the need for a mild oxidizer such as aerobic O2. Thus, species 4c can be oxidized by atmospheric O2,15 generating the radical anion (O2˙−) and the iminium ion 4d that undergo hydrolysis, furnishing the desired product 3a and regenerating the organocatalyst 4a.
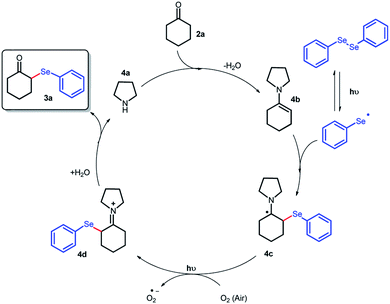 |
| Scheme 4 Proposed radical mechanism induced by light. | |
Conclusions
In summary, we developed an alternative method for the synthesis of α-selenoketones using an environmentally friendly, photo-induced process for the first time. A range of α-selenylated products were obtained from substituted diorganoyldiselenides and cyclic and acyclic ketones in moderate to excellent yields, proving the generality of this methodology. Compared with traditional methods, our methodology is a mild, metal-free, simple, and practical tool for the synthesis of a range of α-selenoketones, and it has potential applications in the synthesis of synthetic intermediates in organic chemistry, as well as bioactive compounds.
Experimental
General information
The solvents were used without further purification. Pyrrolidine was previously distilled. Other commercially available materials were used as received. The photoreactions were monitored using Shimadzu GC-MSQP2010 mass spectrometer and thin layer chromatography. The TLC was carried out on Merck silica gel (60 F254) and flash chromatography was performed in silica gel (230–400 mesh). The NMR spectra were recorded on 400 MHz and 500 MHz spectrometer Varian Inova 400, Varian Inova 500 and Bruker Avance 400. Chemical shifts (δ) are expressed in ppm downfield from tetramethylsilane (TMS) as internal standard. Hydrogen coupling patterns are described as singlet (s), doublet (d), double doublet (dd), double triplet (dt), triplet (t), double quartet (dq), quartet (q), multiplet (m). Coupling constants (J) are reported in Hertz. Low-resolution mass spectra were obtained with a Shimadzu GC-MSQP2010 mass spectrometer. GC analysis were conducted on a RESTEC HP-5MS capillary column (30 m, 0.25 mm id, 0.25 μm film thickness) using the products dissolved in ethyl acetate. High-resolution mass spectra were obtained in a Micromass Q-Tof micro mass spectrometer.
General procedure for the synthesis of the α-selenoketones (3)
To a reaction flask was added diselenide (1.0 equiv.) and MeCN (1 mL). Then, ketone (0.5 mmol) and the organocatalyst pyrrolidine (20 mol%) was added. After, the system was arranged in a photoreactor containing a CFL UVA 26 W. The reaction was kept stirring at room temperature by the indicated time (the reaction progress was monitored by GC-MS), then pyrrolidine and solvent were removed in reduced pressure. The product was purified on column chromatography using hexane and diethyl ether as eluent.
2-(Phenylselanyl)cyclohexanone (3a)10b
Yield: 77.1 mg (60%). Light yellow oil. 1H-NMR (400 MHz, CDCl3): δ 7.56–7.51 (m, 2H), 7.31–7.23 (m, 3H), 3.91 (td, J = 4.7, 1.2 Hz, 1H), 3.04–2.92 (m, 1H), 2.34–2.27 (m, 1H), 2.25–2.13 (m, 2H), 2.01–1.91 (m, 1H), 1.92–1.67 (m, 3H); 13C-NMR (100 MHz, CDCl3): δ 207.8, 134.5, 129.1, 128.5, 128.0, 51.5, 38.4, 33.9, 26.8, 22.8.
2-(p-Tolylselanyl)cyclohexanone (3b)9
Yield: 120 mg (85%). Light yellow oil. 1H-NMR (400 MHz, CDCl3): δ 7.45–7.41 (m, 2H), 7.11–7.07 (m, 2H), 3.85 (td, J = 5.1, 1.7 Hz, 1H), 3.04–2.94 (m, 1H), 2.38–2.25 (m, 4H), 2.24–2.14 (m, 2H), 2.03–1.91 (m, 1H), 1.91–1.64 (m, 3H); 13C-NMR (100 MHz, CDCl3): δ 207.8, 138.3, 135.0, 129.9, 124.6, 51.6, 38.3, 33.7, 26.8, 22.7, 21.1.
2-((4-Methoxyphenyl)selanyl)cyclohexanone (3c)9
Yield: 94.8 mg (67%). Light yellow oil. 1H-NMR (400 MHz, CDCl3): 7.49–7.44 (m, 2H), 6.83–6.79 (m, 2H), 3.82–3.76 (m, 4H), 3.02–2.93 (m, 1H), 2.34–2.24 (m, 1H), 2.21–2.11 (m, 2H), 2.01–1.90 (m, 1H), 1.89–1.63 (m, 3H); 13C-NMR (100 MHz, CDCl3): 207.7, 159.9, 137.1, 118.3, 114.8, 55.1, 51.9, 38.2, 33.5, 26.7, 22.5.
2-((4-Chlorophenyl)selanyl)cyclohexanone (3d)9
Yield: 100 mg (70%). Light yellow solid. 1H-NMR (500 MHz, CDCl3): δ 7.48–7.44 (m, 2H), 7.26–7.23 (m, 2H), 3.89 (td, J = 5.4, 1.5 Hz, 1H), 3.01–2.92 (m, 1H), 2.35–2.29 (m, 1H), 2.26–2.14 (m, 2H), 2.00–1.92 (m, 1H), 1.87–1.77 (m, 2H), 1.75–1.67 (m, 1H); 13C-NMR (125 MHz, CDCl3): δ 207.4, 136.0, 134.5, 129.3, 126.6, 51.7, 38.4, 33.8, 26.7, 22.9.
2-((2-Methoxyphenyl)selanyl)cyclohexanone (3e)
Yield: 106.2 mg (75%). Light yellow oil. 1H-NMR (400 MHz, CDCl3): δ 7.51 (dd, J = 6, 1.7 Hz, 1H), 7.29–7.24 (m, 1H), 6.92–6.83 (m, 2H), 4.05 (td, J = 4.9, 1.6 Hz, 1H), 3.86 (s, 3H), 3.13–3.03 (m, 1H), 2.32–2.18 (m, 3H), 2.05–1.95 (m, 1H), 1.95–1.84 (m, 1H), 1.83–1.68 (m, 2H); 13C-NMR (100 MHz, CDCl3): δ 208.2, 158.3, 134.3, 129.2, 121.4, 117.7, 110.6, 55.8, 48.5, 38.1, 33.6, 27.0, 22.7. MS (relative intensity) m/z: 284 (16.2), 186 (10.8), 108 (100). HRMS (ESI+): exact mass calculated for [M + Na]+ (C13H16O2SeNa) requires m/z 307.0213, found: m/z 307.0211.
2-(o-Tolylselanyl)cyclohexanone (3f)
Yield: 128.3 mg (96%). Light yellow oil. 1H-NMR (400 MHz, CDCl3): δ 7.54 (dd, J = 7.3, 1.2 Hz, 1H), 7.23–7.17 (m, 2H), 7.13–7.07 (m, 1H), 3.88 (td, J = 5.0, 1.7 Hz, 1H), 3.05–2.95 (m, 1H), 2.44 (s, 3H), 2.35–2.26 (m, 1H), 2.25–2.17 (m, 2H), 2.03–1.95 (m, 1H), 1.94–1.84 (m, 1H), 1.81–1.65 (m, 2H). 13C-NMR (100 MHz, CDCl3): δ 207.8, 140.8, 134.9, 130.0, 129.6, 128.2, 126.6, 50.4, 38.4, 33.8, 26.8, 22.7, 22.7. MS (relative intensity) m/z: 268 (54.6), 170 (59), 91 (100.0). HRMS (ESI+): exact mass calculated for [M + Na]+ (C13H16OSeNa) requires m/z 291.0264, found: m/z 291.0261.
2-((3(Trifluoromethyl)phenyl)selanyl)cyclohexanone (3g)
Yield: 154.5 mg (95%). Yellow oil. 1H-NMR (500 MHz, CDCl3): δ 7.80 (s, 1H), 7.71 (d, J = 7.7 Hz, 1H), 7.54 (d, J = 8.0 Hz, 1H), 7.40 (t, J = 7.7 Hz, 1H), 3.97 (t, J = 5.5 Hz, 1H), 2.99–2.91 (m, 1H), 2.38–2.31 (m, 1H), 2.29–2.15 (m, 2H), 2.01–1.92 (m, 1H), 1.90–1.78 (m, 2H), 1.77–1.69 (m, 1H). 13C-NMR (125 MHz, CDCl3): δ 207.3, 137.5 (q, J = 1.1 Hz), 131.3 (q, J = 32.4 Hz), 130.8 (q, J = 3.8 Hz), 129.6, 129.4, 124.7 (q, J = 3.8 Hz), 123.5 (q, J = 272.8 Hz), 51.7, 38.6, 34.0, 26.8, 23.1. MS (relative intensity) m/z: 322 (22.18), 225 (35.6), 69 (36), 41 (100.0). HRMS (ESI+): exact mass calculated for [M + Na]+ (C13H13F3OSeNa) requires m/z 344.9981, found: m/z 344.9981.
2-((4-Fluorophenyl)selanyl)cyclohexanone (3h)9
Yield: 115.1 mg (87%). Light yellow oil. 1H-NMR (400 MHz, CDCl3): δ 7.54–7.49 (m, 2H), 7.01–6.94 (m, 2H), 3.84 (td, J = 5.3, 1.7 Hz, 1H), 3.01–2.93 (m, 1H), 2.34–2.27 (m, 1H), 2.24–2.13 (m, 2H), 2.01–1.92 (m, 1H), 1.89–1.75 (m, 2H), 1.75–1.66 (m, 1H). 13C-NMR (100 MHz, CDCl3): δ 207.5, 162.9 (d, J = 248.6 Hz), 137.1 (d, J = 8.0 Hz), 122.9 (d, J = 3.5 Hz), 116.3 (d, J = 21.5 Hz), 51.9, 38.3, 33.7, 26.7, 22.8.
2-(Mesitylselanyl)cyclohexanone (3i)
Yield: 137.2 mg (92%). Light yellow oil. 1H-NMR (400 MHz, CDCl3): δ 6.92 (q, J = 0.5 Hz, 2H), 3.58 (td, J = 4.3, 1.1 Hz, 1H), 3.09–2.98 (m, 1H), 2.48 (s, 6H), 2.34–2.06 (m, 6H), 2.04–1.95 (m, 1H), 1.93–1.83 (m, 1H), 1.76–1.641 (m, 2H); 13C-NMR (100 MHz, CDCl3): δ 207.7, 143.4, 138.8, 128.6, 126.8, 50.6, 38.0, 33.5, 26.5, 24.3, 22.4, 20.9. MS (relative intensity) m/z: 296 (35), 198 (16.2), 119.1 (100.0). HRMS (ESI+): exact mass calculated for [M + Na]+ (C15H20OSeNa) requires m/z 319.0577, found: m/z 319.0575.
2-(Naphthalen-2-ylselanyl)cyclohexanone (3j)
Yield: 90.9 mg (62%). Light yellow oil. 1H-NMR (400 MHz, CDCl3): δ 8.44–8.40 (m, 1H), 7.86–7.81 (m, 3H), 7.59–7.47 (m, 2H), 7.38 (dd, J = 8.2, 7.1 Hz, 1H), 3.92 (td, J = 5.4, 1.5 Hz, 1H), 3.03–2.93 (m, 1H), 2.35–2.27 (m, 1H), 2.24–2.07 (m, 2H), 2.01–1.60 (m, 4H); 13C-NMR (100 MHz, CDCl3): δ 207.6, 135.3, 134.7, 134.0, 129.6, 128.6, 127.7, 127.7, 126.9, 126.2, 125.7, 51.4, 38.8, 34.0, 26.8, 23.0. MS (relative intensity) m/z: 304 (55.1), 207 (37.18), 128 (100), 97 (11.8). HRMS (ESI+): exact mass calculated for [M + Na]+ (C16H16OSeNa) requires m/z 327.0264, found: m/z 327.0262.
2-(Butylselanyl)cyclohexanone (3k)
Yield: 128.3 mg (80%). Light yellow oil. 1H-NMR (400 MHz, CDCl3): δ 3.54 (t, J = 3.2 Hz, 1H), 3.22–3.12 (m, 1H), 2.67–2.51 (m, 2H), 2.27–2.20 (m, 1H), 2.20–2.11 (m, 2H), 2.06–1.97 (m, 1H), 1.74–1.58 (m, 5H), 1.43–1.33 (m, 2H), 0.90 (t, J = 7.4 Hz, 3H). NMR (100 MHz, CDCl3): δ 208.2, 45.3, 36.5, 32.9, 32.0, 26.4, 25.2, 22.9, 21.7, 13.5. MS (relative intensity) m/z: 234 (4.6), 98 (100), 55 (14.7). HRMS (ESI+): exact mass calculated for [M + Na]+ (C10H18OSeNa) requires m/z 257.0421, found: m/z 257.0421.
2-((4-Chlorophenyl)selanyl)cyclopentanone (3l)
Yield: 89 mg (65%). Light yellow oil. 1H-NMR (400 MHz, CDCl3): 7.56–7.49 (m, 2H), 7.29–7.23 (m, 2H), 3.75–3.69 (m, 1H), 2.40–2.26 (m, 2H), 2.26–2.14 (m, 1H), 2.13–1.90 (m, 3H). 13C-NMR (100 MHz, CDCl3): 214.1, 136.6, 134.9, 129.3, 125.9, 46.5, 36.1, 30.5, 20.9. MS (relative intensity) m/z: 274 (11.9), 55 (100), 83 (4.8). HRMS (ESI+): exact mass calculated for [M + Na]+ (C11H11ClOSeNa) requires m/z 296.9561, found: 296.9557.
2-(o-Tolylselanyl)cyclopentanone (3m)
Yield: 71.1 mg (56%). Light yellow oil. 1H-NMR (400 MHz, CDCl3): δ 7.61 (d, J = 7.5 Hz, 1H), 7.25–7.20 (m, 2H), 7.15–7.08 (m, 1H), 3.81–3.76 (m, 1H), 2.49–2.44 (m, 4H), 2.34–2.05 (m, 3H), 2.02–1.91 (m, 2H). 13C-NMR (100 MHz, CDCl3): δ 214.2, 141.26, 135.6, 130.0, 129.1, 128.6, 126.5, 45.5, 36.1, 30.5, 22.8, 20.9. MS (relative intensity) m/z: 254 (10.5), 170 (12.8), 91 (100). HRMS (ESI+): exact mass calculated for [M + Na]+ (C12H14OSeNa) requires m/z 277.0108, found: 277.0104.
2-((3-(Trifluoromethyl)phenyl)selanyl)cyclopentanone (3n)
Yield: 110.8 mg (72%). Light yellow oil. 1H-NMR (400 MHz, CDCl3): δ 7.80 (s, 1H), 7.71 (d, J = 7.7 Hz, 1H), 7.54 (d, J = 8.0 Hz, 1H), 7.41 (t, J = 7.8 Hz, 1H), 3.83–3.78 (m, 1H), 2.43–2.32 (m, 2H), 2.28–2.18 (m, 1H), 2.12–1.95 (m, 3H). 13C-NMR (100 MHz, CDCl3): 213.9, 138.0 (q, J = 1.2 Hz), 131.3 (q, J = 32.6 Hz), 131.3 (q, J = 3.9 Hz), 129.4, 129.1, 125.0 (q, J = 3.8 Hz), 123.5 (q, J = 272.7 Hz), 46.4, 36.1, 30.6, 21.0. MS (relative intensity) m/z: 308 (21.6), 252 (10.4), 83 (11.3), 55 (100). HRMS (ESI+): exact mass calculated for [M + Na]+ (C12H11F3OSeNa) requires m/z 330.9825, found: m/z 330.9834.
2-((4-Chlorophenyl)selanyl)cycloheptanone (3o)
Yield: 71 mg (47%). Light yellow oil. 1H-NMR (400 MHz, CDCl3): δ 7.51–7.45 (m, 2H), 7.28–7.22 (m, 2H), 3.77 (dd, J = 11.2, 5.6 Hz, 1H), 2.77–2.71 (m, 1H), 2.45–2.35 (m, 1H), 2.33–2.23 (m, 1H), 1.98–1.89 (m, 2H), 1.88–1.80 (m, 1H), 1.68–1.56 (m, 1H), 1.52–1.36 (m, 2H), 1.34–1.20 (m, 1H). 13C-NMR (100 MHz, CDCl3): δ 209.0, 136.2, 134.7, 129.3, 126.5, 52.5, 39.8, 30.3, 30.2, 27.9, 25.6. MS (relative intensity) m/z: 302 (9.6), 192 (4.8), 83 (14.2), 55 (100). HRMS (ESI+): exact mass calculated for [M + Na]+ (C13H15ClOSeNa) requires m/z 324.9874, found: m/z 324.9868.
2-(o-Tolylselanyl)cycloheptanone (3p)
Yield: 59.2 mg (42%). Light yellow oil. 1H-NMR (400 MHz, CDCl3): δ 7.57 (d, J = 7.7 Hz, 1H), 7.24–7.18 (m, 2H), 7.14–7.07 (m, 1H), 3.78 (dd, J = 11.1, 5.7 Hz, 1H), 2.86–2.78 (m, 1H), 2.44 (s, 3H), 2.43–2.35 (m, 1H), 2.33–2.24 (m, 1H), 1.98–1.89 (m, 2H), 1.87–1.79 (m, 1H), 1.71–1.60 (m, 1H), 1.53–1.39 (m, 2H), 1.35–1.21 (m, 1H). 13C-NMR (100 MHz, CDCl3): δ 209.0, 141.0, 135.5, 130.0, 129.5, 128.5, 126.5, 51.4, 39.7, 30.4, 30.3, 27.9, 25.7, 22.8. MS (relative intensity) m/z: 282 (19.8), 172 (16.2), 91 (45.8), 55 (100). HRMS (ESI+): exact mass calculated for [M + Na]+ (C14H18OSeNa) requires m/z 305.0421, found: 305.0419.
2-((3-(Trifluoromethyl)phenyl)selanyl)cycloheptanone (3q)
Yield: 90.7 mg (54%). Light yellow oil. 1H-NMR (400 MHz, CDCl3): δ 7.81 (s, 1H), 7.72 (d, J = 7.8 Hz, 1H), 7.55 (d, J = 7.8 Hz, 1H), 7.40 (t, J = 7.8 Hz, 1H), 3.87 (dd, J = 11.2, 5.6 Hz, 1H), 2.83–2.75 (m, 1H), 2.46–2.39 (m, 1H), 2.36–2.26 (m, 1H), 1.99–1.90 (m, 2H), 1.89–1.82 (m, 1H), 1.70–1.60 (m, 1H), 1.55–1.40 (m, 2H), 1.36–1.26 (m, 1H). 13C-NMR (100 MHz, CDCl3): δ 208.9, 137.6 (q, J = 1.2 Hz), 131.3 (q, J = 32.3 Hz), 130.9 (q, J = 3.8 Hz), 129.4, 128.7, 124.9 (q, J = 3.7 Hz), 123.6 (q, J = 272.5 Hz), 52.4, 39.8, 30.3, 30.2, 28.0, 25.6. MS (relative intensity) m/z: 336 (14.4), 145 (10.7), 83 (42.5), 55 (100). HRMS (ESI+): exact mass calculated for [M + H]+ (C15H15F3OSe) requires m/z 337.0318, found: m/z 337.0313.
1-((4-Chlorophenyl)selanyl)propan-2-one (3r)16
Yield: 62 mg (50%). Light yellow oil. 1H-NMR (400 MHz, CDCl3): δ 7.45–7.41 (m, 2H), 7.26–7.22 (m, 2H), 3.57 (s, 2H), 2.27 (s, 3H). 13C-NMR (100 MHz, CDCl3): δ 202.9, 134.4, 134.0, 129.3, 126.6, 36.8, 27.8.
1-(o-Tolylselanyl)propan-2-one (3s)16
Yield: 51.3 mg (45%). Light yellow oil. 1H NMR (400 MHz, CDCl3): δ 7.49 (d, J = 7.5 Hz, 1H), 7.22–7.14 (m, 2H), 7.16–7.10 (m, 1H), 3.58 (s, 2H), 2.45 (s, 3H), 2.28 (s, 3H). 13C NMR (100 MHz, CDCl3): δ 203.4, 139.9, 133.1, 130.2, 129.6, 128.0, 126.8, 35.8, 28.0, 22.3.
2-((3-(Trifluoromethyl)phenyl)selanyl)propan-2-one (3t)16
Yield: 74.7 mg (53%). Light yellow oil. 1H-NMR (400 MHz, CDCl3): δ 7.77 (s, 1H), 7.69 (d, J = 7.8 Hz, 1H), 7.54 (d, J = 7.8 Hz, 1H), 7.41 (t, J = 7.7 Hz, 1H), 3.66 (s, 2H), 2.31 (s, 3H). 13C-NMR (100 MHz, CDCl3): δ 202.9, 136.0 (q, J = 1.0 Hz), 131.2 (q, J = 32.5 Hz), 129.6, 129.4 (q, J = 3.8 Hz), 129.1, 124.6 (q, J = 3.7 Hz), 123.5 (d, J = 272.7 Hz), 36.7, 28.0.
2-((4-Chlorophenyl)selanyl)pentan-3-one (3u)
Yield: 59 mg (43%). Light yellow oil. 1H-NMR (400 MHz, CDCl3): δ 7.44–7.40 (m, 2H), 7.28–7.24 (m, 2H), 3.79 (q, J = 7.0 Hz, 1H), 2.79 (dq, J = 17.4, 7.3 Hz, 1H), 2.51 (dq, J = 17.4, 7.3 Hz, 1H), 1.46 (d, J = 7.0 Hz, 3H), 1.09 (t, J = 7.3 Hz, 3H). 13C-NMR (100 MHz, CDCl3): δ 207.2, 137.3, 135.3, 129.3, 124.8, 45.0, 32.9, 16.3, 8.4. MS (relative intensity) m/z: 276 (12), 219 (16.7), 139 (100), 57 (24.3). HRMS (ESI+): exact mass calculated for [M + Na]+ (C11H13ClOSeNa) requires m/z 298.9718, found: m/z 298.9715.
2-(o-Tolylselanyl)pentan-3-one (3v)
Yield: 52 mg (41%). Light yellow oil. 1H-NMR (400 MHz, CDCl3): δ 7.52 (d, J = 7.5 Hz, 1H), 7.27–7.21 (m, 2H), 7.15–7.08 (m, 1H), 3.83 (q, J = 7.0 Hz, 1H), 2.75 (dq, J = 17.4, 7.3 Hz, 1H), 2.54–2.41 (m, 4H), 1.51 (d, J = 7.0 Hz, 3H), 1.05 (t, J = 7.3 Hz, 3H). 13C-NMR (100 MHz, CDCl3): δ 208.0, 141.6, 136.0, 130.2, 128.9, 128.8, 126.5, 44.8, 32.9, 22.9, 16.5, 8.3. MS (relative intensity) m/z: 256 (4.2), 119 (100), 91 (13.2). HRMS (ESI+): exact mass calculated for [M + Na]+ (C12H16OSeNa) requires m/z 279.0264, found: m/z 279.0262.
2-((3-(Trifluoromethyl)phenyl)selanyl)pentan-3-one (3w)
Yield: 75.9 mg (49%). Light yellow oil. 1H-NMR (400 MHz, CDCl3): 7.77 (s, 1H), 7.67 (d, J = 7.7 Hz, 1H), 7.60 (d, J = 7.8 Hz, 1H), 7.42 (t, J = 7.8 Hz, 1H), 3.87 (q, J = 7.0 Hz, 1H), 2.80 (dq, J = 17.5, 7.3 Hz, 1H), 2.54 (dq, J = 17.5, 7.3 Hz, 1H), 1.50 (d, J = 7.0 Hz, 3H), 1.09 (t, J = 7.3 Hz, 3H). 13C-NMR (100 MHz, CDCl3): 207.1, 138.8 (q, J = 1.1 Hz), 132.2 (q, J = 3.9 Hz), 131.3 (q, J = 32.3 Hz), 129.4, 127.9, 125.4 (q, J = 3.6 Hz), 123.5 (q, J = 272.8 Hz), 45.2, 32.8, 16.5, 8.3. MS (relative intensity) m/z: 310 (7.6), 253 (11.6), 145 (7.8), 57 (100). HRMS (ESI+): exact mass calculated for [M + Na]+ (C12H13F3OSeNa) requires m/z 332.9981, found: m/z 332.9983.
Conflicts of interest
There are no conflicts to declare.
Acknowledgements
We are grateful to CAPES (Finance Code 001), CNPq, INCT-CMN and FAPERGS for financial and technical support.
Notes and references
-
(a) N. A. Romero and D. A. Nicewicz, Organic photoredox catalysis, Chem. Rev., 2016, 116, 10075 CrossRef CAS PubMed;
(b) X. Yang, Y. Zheng, L. Zheng, L. Wu, C. Tung and B. Chen, Visible light-catalytic dehydrogenation of benzylic alcohols to carbonyl compounds by using an eosin Y and nickel–thiolate complex dual catalyst system, Green Chem., 2019, 21, 1401 RSC.
-
(a) A. Albini and M. Fagnoni, 1908: Giacomo Ciamician and the Concept of Green Chemistry, ChemSusChem, 2008, 1, 63 CrossRef CAS PubMed;
(b) M. Kozlowski and T. Yoon, Editorial for the special issue on photocatalysis, J. Org. Chem., 2016, 81, 6895 CrossRef CAS PubMed.
-
(a) V. Rathore and S. Kumar, Visible-light-induced metal and reagent-free oxidative coupling of sp2 C–H bonds with organo-dichalcogenides: synthesis of 3-organochalcogenyl indoles, Green Chem., 2019, 21, 2670 RSC;
(b) E. Barragan, P. A. Noonikara, C. H. Yang, H. Wang and A. Bugarin, Metal-free cross-coupling of π-conjugated triazenes with unactivated arenes via photoactivation, Org. Chem. Front., 2019, 6, 152 RSC.
-
(a) A. Nomoto, Y. Higuchi, Y. Kobiki and A. Ogawa, Synthesis of selenium compounds by free radical addition based on visible-light-activated Se–Se bond cleavage, Mini-Rev. Med. Chem., 2013, 13, 814 CrossRef CAS PubMed;
(b) Y. Kobiki, S. I. Kawaguchi and A. Ogawa, Highly regioselective hydroselenation of inactivated terminal alkynes using diselenide–Ph2P(O)H mixed systems under visible-light irradiation, Tetrahedron Lett., 2013, 54, 5453 CrossRef CAS;
(c) D. Yang, G. Li, C. Xing, W. Cui, K. Li and W. Wei, Metal-and photocatalyst-free visible-light-promoted regioselective selenylation of coumarin derivatives via oxidation-induced C–H functionalization, Org. Chem. Front., 2018, 5, 2974 RSC;
(d) A. C. Weber, F. L. Coelho, R. F. Affeldt and P. H. Schneider, Visible-Light Promoted Stereoselective Arylselanyl Functionalization of Alkynes, Eur. J. Org. Chem., 2018, 47, 6738 CrossRef.
-
(a) F. L. Coelho, E. S. Gil, P. F. B. Gonçalves, L. F. Campo and P. H. Schneider, Intramolecular Hydroamination of Selenoalkynes to 2-Selenylindoles in the Absence of Catalyst, Chem. - Eur. J., 2019, 25, 1 CrossRef PubMed;
(b) Q. Zhang, Y. Ban, P. Yuan, S. Peng, J. Fang, L. Wu and Q. Liu, Visible-light-mediated aerobic selenation of (hetero)arenes with diselenides, Green Chem., 2017, 19, 5559 RSC;
(c) S. K. Tripathi, S. Sharma, H. B. Singh and R. J. Butcher, 2-Phenoxyethanol derived diselenide and related compounds; synthesis of a seven-membered seleninate ester, Org. Biomol. Chem., 2011, 9, 581 RSC;
(d) E. J. Lenardão, C. Santi and L. Sancineto, New Frontiers in Organoselenium Compounds, Springer, Cham, Switzerland, 2018 CrossRef.
-
(a) D. Bartolini, L. Sancineto, A. F. de Bem, Q. D. Tew, C. Santi, R. Radi, P. Toquato and F. Galli, Selenocompounds in cancer therapy: an overview, Adv. Cancer Res., 2017, 136, 259 CrossRef CAS PubMed;
(b) M. Ibrahim, B. H. M. Mussulini, L. Moro, D. B. Rosemberg, D. L. Oliveira, J. Rocha, R. S. Schwab, A. M. Assis, D. Souza, E. P. Rico and P. H. Schneider, Anxiolytic effects of diphenyl diselenide on adult zebrafish in a novelty paradigma, Prog. Neuro-Psychopharmacol. Biol. Psychiatry, 2014, 54, 187 CrossRef CAS PubMed;
(c) F. N. Victoria, D. M. Martinez, M. Castro, A. M. Casaril, D. Alves, E. J. Lenardão, H. D. Salles, P. H. Schneider and L. Savegnago, Antioxidant properties of (R)-Se-aryl thiazolidine-4-carboselenoate, Chem.-Biol. Interact., 2013, 205, 100 CrossRef CAS PubMed;
(d) G. K. Azad and R. S. Tomar, Ebselen: a promising antioxidant drug: mechanisms of action and targets of biological pathways, Mol. Biol. Rep., 2014, 41, 4865 CrossRef CAS PubMed;
(e) F. Martini, S. G. Rosa, I. P. Klann, B. C. W. Fulco, F. B. Carvalho, L. F. Rahmeier, M. C. Fernandes and C. W. Nogueira, A multifunctional compound ebselen reverses memory impairment, apoptosis and oxidative stress in a mouse model of sporadic Alzheimer's disease, J. Psychiatr. Res., 2019, 109, 107 CrossRef PubMed;
(f) N. F. Pavin, F. Donato, F. W. Cibin, C. R. Jesse, P. H. Schneider, H. D. de Salles, L. A. Soares, D. Alves and L. Savegnago, Antinociceptive and anti-hypernociceptive effects of Se-phenyl thiazolidine-4-carboselenoate in mice, Eur. J. Pharmacol., 2011, 668, 169 CrossRef CAS PubMed.
-
(a) G. Pandey, S. K. Tiwari, A. Budakoti and P. K. Sahani, Transition-metal-free photoredox intermolecular α-arylation of ketones, Org. Chem. Front., 2018, 5, 2610 RSC;
(b) H. J. Reich, J. H. Renga and I. L. Reiche, Organoselenium chemistry: Conversion of ketones to enones by selenoxide syn elimination, J. Am. Chem. Soc., 1975, 97, 5434 CrossRef CAS.
-
(a) F. S. S. Sousa, P. T. Birmann, R. Baldinotti, M. G. Fronza, R. Balaguez, D. Alves, C. A. Brüning and L. Savegnago, α-(phenylselanyl)acetophenone mitigates reserpine-induced pain–depression dyad: Behavioral, biochemical and molecular docking evidences, Brain Res. Bull., 2018, 142, 129 CrossRef CAS PubMed;
(b) F. S. S. Sousa, P. T. Birmann, S. R. Bampi, M. G. Fronza, R. Balaguez, D. Alves, M. R. Leite, C. W. Nogueira, C. A. Brüning and L. Savegnago, Lipopolysaccharide-induced depressive-like, anxiogenic-like and hyperalgesic behavior is attenuated by acute administration of α-(phenylselanyl) acetophenone in mice, Neuropharmacology, 2019, 146, 128 CrossRef PubMed.
- R. G. Mcleod, B. Johnston and M. Pinto, A generalized exo-anomeric effect: Substituent and solvent effects on the conformational equilibria of 2-(arylseleno)cyclohexanones, Isr. J. Chem., 2000, 40, 307 CrossRef CAS.
-
(a) M. Iwaoka, e-EROS Encycl. Reagents Org. Synth., 2005, 1, 16 Search PubMed;
(b) M. H. Yang, H. S. Wang, Y. F. Zheng and C. J. Zhu, A simple protocol for the synthesis of α-substituted ketones, Chin. J. Org. Chem., 2006, 9, 1268 Search PubMed;
(c) J. Cossy and L. Furet, N-(Phenylseleno)Phthalimide: A Useful Reagent For The α-Selenylation Of Ketones And Aldehydes, Tetrahedron Lett., 1993, 34, 7755 CrossRef CAS;
(d) N. Miyoshi, T. Yamamoto, N. Kambe, S. Murai and N. Sonoda, A convenient method for the synthesis of α-phenylselenenyl carbonyl compounds, Tetrahedron Lett., 1982, 23, 4813 CrossRef CAS;
(e) B. Movassagh and A. Takallou, N,N,N-Triphenylselenylisocyanuric Acid (TPSCA): A New Versatile Reagent for α-Phenylselenenylation of Aldehydes and Ketones, Synlett, 2015, 26, 2247 CrossRef CAS.
- H. J. Reich, M. L. Cohen and P. S. Peter, Reagents for synthesis of organoselenium compounds: diphenyl diselenide and benzeneselenenyl chloride, Curr. Org. Synth., 1980, 59, 141 CAS.
-
(a) N. Nazari and B. Movassagh, α-Phenylselenenylation of aldehydes and ketones with diphenyl diselenide mediated by KF/Al2O3, Tetrahedron Lett., 2009, 50, 1453 CrossRef;
(b) F. N. Victoria, C. S. Radatz, M. Sachini, R. G. Jacob, G. Perin, W. P. da Silva and E. J. Lenardão, KF/Al2O3 and PEG-400 as a recyclable medium for the selective α-selenation of aldehydes and ketones: preparation of potential antimicrobial agents, Tetrahedron Lett., 2009, 50, 6761 CrossRef CAS;
(c) Y. Nishiyama, Y. Koguma, T. Tanaka and R. Umeda, Cesium carbonate-catalyzed α-phenylchalcogenation of carbonyl compounds with diphenyl dichalcogenide, Molecules, 2009, 14, 3367 CrossRef CAS PubMed.
-
(a) D. S. Rampon and P. H. Schneider, Palladium-catalyzed direct arylation of selenophene, J. Org. Chem., 2014, 79, 5987 CrossRef CAS PubMed;
(b) C. S. Radatz, D. Alves and P. H. Schneider, Direct synthesis of 2-aryl-1, 3-benzoselenazoles by reaction of bis(2-aminophenyl) diselenides with aryl aldehydes using sodium metabisulfite, Tetrahedron, 2013, 28, 1316 CrossRef;
(c) T. E. Frizon, D. S. Rampon, H. Gallardo, A. A. Merlo, P. H. Schneider, O. E. D. Rodrigues and A. L. Braga, Selenides and diselenides containing oxadiazoles: a new class of functionalised materials, Liq. Cryst., 2012, 39, 769 CrossRef CAS;
(d) D. S. Rampon, F. S. Rodembusch, R. Lourega, P. F. B. Gonçalves, A. A. Merlo and P. H. Schneider, An evaluation of the chalcogen atom effect on the mesomorphic and electronic properties in a new homologous series of chalcogeno esters, J. Braz. Chem. Soc., 2010, 21, 2100 CrossRef CAS;
(e) C. S. Radatz, D. S. Rampon, R. A. Balaguez, D. Alves and P. H. Schneider, Synthesis of 2-Substituted 1,3-Benzoselenazoles from Carboxylic Acids Promoted by Tributylphosphine, Eur. J. Org. Chem., 2014, 31, 6945 CrossRef.
- Q. Teng, Y. Yao, W. Wei, H. Tang, J. Li and Y. Pan, Direct C–H sulfenylation of quinoxalinones with thiols under visible-light-induced photocatalyst-free conditions, Green Chem., 2019, 21, 6241 RSC.
- S. Mitra, M. Ghosh, S. Mishra and A. Hajra, Metal-free thiocyanation of imidazoheterocycles through visible light photoredox catalysis, J. Org. Chem., 2015, 80, 8275 CrossRef CAS PubMed.
- G. Yan, J. A. Borah, L. Wang, Z. Pan, S. Chen, X. Shen and X. Wu, α-Arylchalcogenation of acetone with diaryl dichalcogenide via metal-free oxidative C(sp3)–H bond functionalization, Tetrahedron Lett., 2015, 56, 4305 CrossRef CAS.
Footnote |
† Electronic supplementary information (ESI) available. See DOI: 10.1039/d0ra00802h |
|
This journal is © The Royal Society of Chemistry 2020 |
Click here to see how this site uses Cookies. View our privacy policy here.