DOI:
10.1039/D0RA00811G
(Paper)
RSC Adv., 2020,
10, 17870-17880
Research on the controllable degradation of N-methylamido and dialkylamino substituted at the 5th position of the benzene ring in chlorsulfuron in acidic soil†‡
Received
27th January 2020
, Accepted 6th April 2020
First published on 7th May 2020
Abstract
Owing to the lengthy residual problems associated with chlorsulfuron, metsulfuron-methyl, and ethametsulfuron, which prevents them from being used in the “annual multi-crop planting system”, the application of these sulfonylurea herbicides (SU) has regrettably been terminated in China since 2014. In this field, we were the first to discover that the 5th position of the benzene ring in chlorsulfuron is a key point for influencing its degradation rate and the amino moiety at this position showed faster degradation rates and maintained their original potent bioactivity. In this study, we further elaborated on N-methylamido and dialkylamino substituents at the same position in chlorsulfuron to obtain 18 novel structures as M and N series. Their half-life degradation (DT50) values were faster, to varying degrees, than chlorsulfuron in acidic soil. It was found that most of the titled structures also retained their potent herbicidal activity and the crop safety of the M series towards corn greatly increased. Based on these data, a comprehensive graph describing the structure/degradation relationship was established first. Relating to the new molecules, their herbicidal activity (A), degradation rates (D), and crop safety (S) relationship were correlated and we used this approach to predict and explore the most preferable molecule, which coincided to the corresponding experimental data. The new concept of controllable degradation will provide us with more insight when searching for new ecological bioactive molecules in the future.
Introduction
Sulfonylurea (SU) herbicides belong to a class of herbicides discovered and commercialized by DuPont in the late eighties. SU have super-activity, ultra-low dosage, very low toxicity, and desirable selectivity, which meet the growing environmental and ecological concerns posed by modern herbicides. SU targets acetolactate synthase (ALS), which exists solely in plants and microbes, thus being very safe for mammals.1,2 The biosynthesis of branched chain amino acids, such as valine, leucine, and isoleucine, gets hindered when SU docks on ALS, which leads to the inability to synthesize proteins and eventually causes the death of the herbs. Regrettably, several SU herbicides are not suitable for use in China due to their lengthy residual problems. The Chinese agricultural production follows the “annual multi-crop planting system”, which is quite different from regions abroad that adopt the “annual one-crop planting system” on the same piece of land. Due to the undesirable prolonged residual problems, the Ministry of Agriculture of China has suspended field application licenses for chlorsulfuron, metsulfuron-methyl, and ethametsulfuron since 2014.3
The pH of the soil is one of the key factors that affects the degradation behavior of SU.4 The DT50 of SU in acidic conditions is faster than that in neutral or alkaline condition.5 Walker et al.6 reported that the DT50 of metsulfuron-methyl is 23 days in soil with pH 5.8 and 73 days in soil with pH 7.3. Thirunarayanan et al.7 reported that the DT50 of chlorsulfuron is 88.5 days at pH 6.2 and 144 days at pH 8.1 at 20 °C. These SU soil degradation experiments confirmed the correlation between the DT50 and pH values. In our previous study, Hua et al.8–10 introduced amino groups on the 5th position of the benzene ring in chlorsulfuron and the modified structures A, B, and C with substituted amino groups (Fig. 1), surprisingly, mostly maintained their original potent bioactivity, yet exhibited a faster degradation rate in pH 5.41 soil. In order to find a suitable DT50 to adapt to crops in different growth periods, we further introduced various N-methylamido and dialkylamino substituents on the 5th position of the benzene ring in chlorsulfuron and synthesized 18 titled compounds coded as M01–M05 and N01–N13 (Fig. 2.). We studied their DT50 in pH 5.52 soil and compared them with chlorsulfuron as a control. Their herbicidal activity and crop safety were also evaluated.
 |
| Fig. 1 The design of titled compounds. | |
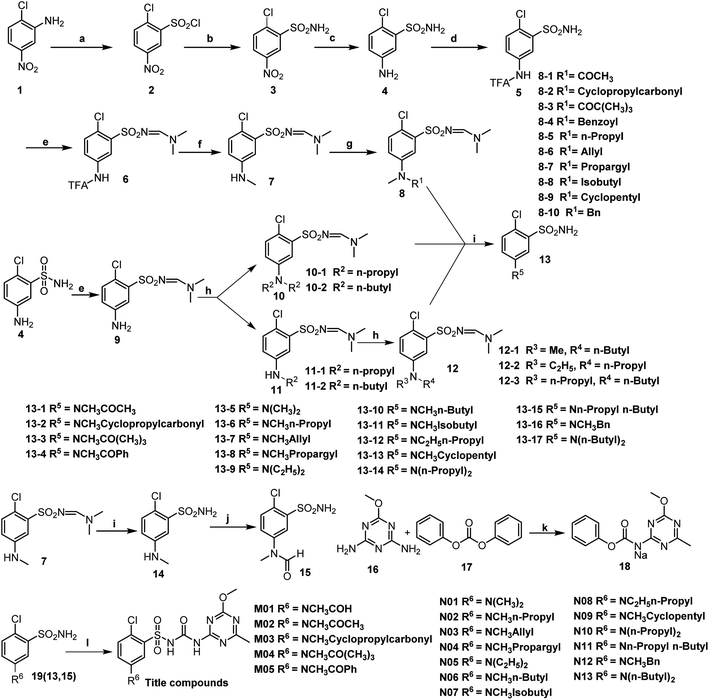 |
| Fig. 2 Synthetic strategy for title compounds. Reagents and conditions: (a) H2O, HCl, NaNO2 → H2O, HCl, CuCl2, NaHSO3, −5 °C; (b) 28% NH3·H2O, THF, 0 °C → RT (room temperature), overnight; (c) Fe, HCl, C2H5OH, H2O, reflux; (d) TFAA (trifluoroacetic anhydride), CH2Cl2, 0 °C; (e) DMF-DMA (N,N-dimethylformamide dimethyl acetal), CH2Cl2; (f) ICH3, K2CO3, DMF, 50 °C; (g) haloalkane or acyl chloride, K2CO3, CH3CN, reflux; (h) haloalkane, K2CO3, CH3CN, reflux; (i) 80% H2NNH2·H2O, C2H5OH; (j) HCOONa, HCOOH, reflux; (k) 60% NaH, THF, 0 °C → RT; (l) 18, DBU (1,8-diazabicyclo[5.4.0]undec-7-ene), CH3CN. | |
When agrochemical chemists are earnestly searching for new herbicidal candidates, their main interests are often concentrated on the design of new structures with outstanding herbicidal activity (A); however, the related degradation rate (D) and crop safety (S) are often neglected at the early research stage. According to the peculiar cultivation pattern in China, we started to evaluate these factors from the very beginning. Due to the growing concerns on the impact of SU on the environment and ecology system, we studied the interrelated relationship among these important factors including herbicidal activity (A), soil degradation rates (D), and crop safety (S).
Materials and methods
Materials and instruments
The reagents used in the organic reactions were of analytical grade and the reagents used in high-performance liquid chromatograph (HPLC) were of chromatographic grade. An X-4 binocular microscope melting point apparatus (Beijing Tech Instrument Co., Beijing, China), 400 MHz Bruker AV 400 nuclear magnetic resonance spectrometer (NMR) (Bruker Co., Switzerland), Agilent 6520 Q-TOF LC/MS high-resolution mass spectrometer (HRMS) (Agilent Co., America), Rigaku Saturn 724 CCD diffractometer (Rigaku Corporation, Japan), TU-1810 ultraviolet-visible spectrophotometer (Persee General Analysis Co., Beijing, China), SHZ-88 thermostatic oscillator (Jintan Medical Instrument Factory, Jiangsu, China), Thermo Scientific Legend Mach 1.6 R centrifuge (Thermo Fisher Scientific Inc, US), SPX-150B-Z biochemical incubator (Boxun Industrial Co., Shanghai, China), and Shimadzu HPLC (series LC-20AT), equipped with a binary pump (Shimadzu, LC-20AT), an UV/VIS detector (Shimadzu, SPD-20A), auto sampler (Shimadzu, SIL-20A), Shimadzu shim-pack VP-ODS column (5 mm, 250 mm × 4.6 mm, C18 reversed phase chromatography) connected to a Shimadzu shim-pack GVP-ODS (10 mm × 4.6 mm) pre-column, column oven (Shimadzu, CTO-20AC), and computer (model Dell) for carrying out the experimental data analysis were used.
All of the degradation experiments abided by legal guidelines and formulated instructions from the Institute for the Control of Agrochemicals, Ministry of Agriculture (ICAMA, MOA), China.
General procedure for the synthesis of the intermediate compounds
2-Chloro-5-nitrobenzenesulfonyl chloride 2 was synthesized according to the reported methods.11,12 2-chloro-5-nitrobenzenesulfonamide 3 was prepared by referring to a previously reported procedure,13 5-amino-2-chlorobenzenesulfonamide 4 was synthesized according to the method reported in a literature,14 N-(4-chloro-3-sulfamoylphenyl)-2,2,2-trifluoroacetamide 5 and N-((2-chloro-5-(methylamino)phenyl)sulfonyl)-N,N-dimethylformimidamide 7 were synthesized according to the methods given in ref. 15. N-(4-chloro-3-(N-((dimethylamino)methylene)sulfamoyl)phenyl)-2,2,2-trifluoroacetamide 6, (Z)-N-((5-amino-2-chlorophenyl)sulfonyl)-N,N-dimethylformimidamide 9, compound 13, 2-chloro-5-(methylamino)benzenesulfonamide 14, and N-(4-chloro-3-sulfamoylphenyl)-N-methylformamide 15 were synthesized referring a previously reported methods in literature.16–18
The synthetic procedure for sodium (4-methoxy-6-methyl-1,3,5-triazin-2-yl) (phenoxy-carbonyl)-amide 18
4-Methoxy-6-methyl-1,3,5-triazin-2-amine 16 (1.0 g, 7.08 mmol) and diphenyl carbonate 17 (3.0 g, 14.17 mmol) were added to dry 20 mL THF at 0 °C in an ice bath, and then 60% NaH (0.34 g, 8.56 mmol) was slowly added to the reaction system. Gas was generated at the same time. The ice bath was removed after 1 h and the reaction system turned gray and turbid. The reaction was monitored by TLC until its completion. Suction filtration was implemented quickly and the filter cake was washed with a small amount of dichloromethane. After drying, the important intermediate 18 was obtained.
The synthetic procedure for target compounds M01–M05 and N01–N13
Taking N-(4-chloro-3-(N-((4-methoxy-6-methyl-1,3,5-triazin-2-yl)carbamoyl)sulfamoyl)phenyl)-N-methylformamide M01 as an example: 15 (0.2 g, 0.80 mmol) and 18 (0.23 g, 0.80 mmol) were added to 5 mL of acetonitrile at room temperature and then DBU (0.15 g, 0.97 mmol) was slowly added to the reaction system; it became translucent immediately. The reaction was monitored by TLC until its completion. An additional 30 mL of H2O was added to the reaction system and it was clear and transparent at this time. The pH value of the reaction system was adjusted to 3–5 with 1 M HCl under stirring conditions and a large amount of white solid formed. Suction filtration was carried out, and the filter cake was washed with a small amount of water. After drying, the titled compound M01 was obtained.
N01 and N05 were reported in our previous research.8,9
X-ray diffraction
The crystals of M03 and N03 were obtained by self-evaporation in ethyl acetate and methyl tert-butyl ether (v/v = 5
:
1) and dichloromethane and methyl tert-butyl ether (v/v = 5
:
1), respectively. They were colorless and analyzed via X-ray diffraction (ESI 1 and 2‡). We elucidated the configurations by direct methods using the SHELXS-97 program.19
Biological assay
Pot trials for herbicidal activities against Brassica campestris, Amaranthus tricolor, Echinochloa crusgalli, and Digitaria sanguinalis were tested in our greenhouse according to the procedures in the literature.20,21
Crop safety
Chlorsulfuron is well known as a low-toxicity and broad-spectrum wheat herbicide. However, it was prohibited in northern China due to its lengthy residual problem, which prevents corn from being planted, in the same field, after the wheat harvest. Therefore, corn was also screened along with wheat.
Culture method
The seeds were planted (0.6 cm depth) in a 7.0 cm-diameter disposable paper cup (250 mL) containing artificial mixed soil: loam, vermiculite, and fertilizer soil (v/v/v = 1
:
1
:
1). Before the plant emerged, the cups were covered with a plastic film to keep them moist. The plants were grown in a greenhouse (25 ± 2 °C). The fresh weight of the up-ground plants were measured at certain days after treatment.
Treatment
The dose (active ingredient) for each compound was 30 and 60 (a. i.) g ha−1 for the crops. The purified compounds were dissolved in 100 μL of N,N-dimethylformamide with the addition of a little Tween 80 (1.0 g) in distilled water (1000 mL), and then they were sprayed using a laboratory belt sprayer delivering at 750 L ha−1 spray-volume. The same amount of distilled water was sprayed as a control.
Pre-emergency treatment. The compounds were sprayed immediately after seeding the plant. Three replicates of each treatment were performed. The fresh weight of the up-ground plants were measured 16 (corn) or 22 (wheat) days after treatment.
Post-emergency treatment. The compounds were sprayed after the third (corn) or forth (wheat) true leave expanding. Three replicates of each treatment were performed. The fresh weight of the up-ground plants were measured 23 (corn) or 28 (wheat) days after treatment.The variance data analysis was completed by Duncan multiple comparison using Spss 22.0, a statistical analysis software for the analysis of variance, regression, and correlation etc.
Soil degradation experimental strategies
Soil selection. The soil was derived from fresh farmland (0–25 cm) in Shaoguan City, Guangdong Province and standardized according to the Chinese National Standard GB/T 31270.1-2014.22 The texture, pH value, cation exchange capacity (CEC), organic matter concentration, and mechanical composition of the test soil are listed in Table 1, which was determined by the Tianjin Eco-Environmental Monitoring Center.
Table 1 Analysis data of soils
Soils |
Soil texture |
pH |
CEC (cmol+ kg−1) |
Organic matter (g kg−1) |
Soil separate (mm)/mechanical composition (g kg−1) |
Acidic soils |
Sandy clay |
5.52 |
8.9 |
47.3 |
1–2 |
0.5–1 |
0.025–0.5 |
0.05–0.02 |
0.02–0.002 |
<0.002 |
0.25–0.05 |
2.0–0.05 |
0.05–0.002 |
0.857 |
18.0 |
12.3 |
28.0 |
118 |
352 |
471 |
502 |
146 |
Establishment of the standard curve. The standard curves used for quantitative conversion were established with an injection volume of 10 μL at 20 °C (ESI 3‡). The samples were analyzed via HPLC according to the Chinese National Standard GB/T16631-2008.23
Measurement of the recovery rate. According to the Chinese National Standard GB/T 31270.1-2014 (ref. 22) and the Chinese Agricultural Industry Standard NY/T788-2004,24 the concentration of the test compounds in 20 g of soil in a 100 mL conical flask were 5 mg kg−1, 2 mg kg−1, and 0.5 mg kg−1 (adjusted with an acetonitrile solution), respectively. As required, each concentration was repeated 5 times. The regulation of 60% water holding capacity (4.5 mL) after the acetonitrile evaporated completely (about 30 min) was implemented and the soil samples were mixed well. A suitable mixture of solvents was selected for extraction (Table 2.) and decanted into a 100 mL conical flask. The soil samples were sealed with parafilm and shaken with a thermostatic oscillator for 3 h at 200 rpm min−1. The sample was centrifuged at 6500 r/min for 2 min with a Thermo Scientific centrifuge. The supernatant was combined and concentrated in vacuum at 20 °C. Subsequently, the concentrate, an additional 30 mL of HPLC grade water and dichloromethane (30 mL × 2) for extraction were poured into a 250 mL separating funnel. The organic phase was combined and dried using anhydrous sodium sulfate and filtered. The organic solution was concentrated in vacuum at 25 °C and the residual sample was dissolved in 10 mL of chromatographic grade acetonitrile. The acetonitrile solution was filtered through a Millipore filter (organic, nylon-66, 0.22 μm) and 10 μL was injected for HPLC analysis. The average value of the recovery rate and coefficient of variation (R2) were calculated (Table 2).
Table 2 Verification of the recovery rates in various concentrations (in acidic soil, pH 5.52)a
Compd |
HPLC analysis condition |
Extraction solvent (v/v) |
Additive concentration (mg kg−1) |
Average recovery ratio (%) |
Coefficient of variation R2 (%) |
Wavelength |
Flow rate |
Mobile phase (v/v) |
H2O (pH 3.0) was acidified with H3PO4. |
M01 |
225 nm |
0.8 mL min−1 |
CH3OH : H2O (pH 3.0) = 53/47 |
CH3COCH3 : CH2Cl2 : phosphoric acid solution (pH 2.0) = 40/5/5 |
5 |
79.64 |
3.98 |
2 |
82.65 |
1.55 |
0.5 |
87.11 |
1.97 |
M02 |
225 nm |
0.8 mL min−1 |
CH3OH : H2O (pH 3.0) = 53/47 |
CH3COCH3 : CH2Cl2 : phosphoric acid solution (pH 2.0) = 40/5/5 |
5 |
83.39 |
1.47 |
2 |
84.58 |
2.29 |
0.5 |
95.83 |
1.10 |
M03 |
225 nm |
0.8 mL min−1 |
CH3OH : H2O (pH 3.0) = 60/40 |
CH3COCH3 : CH2Cl2 : phosphoric acid solution (pH 2.0) = 40/5/5 |
5 |
81.13 |
2.57 |
2 |
76.66 |
2.37 |
0.5 |
75.89 |
2.47 |
M04 |
225 nm |
0.8 mL min−1 |
CH3OH : H2O (pH 3.0) = 65/35 |
CH3COCH3 : CH2Cl2 : Phosphoric acid solution (pH 2.0) = 40/5/5 |
5 |
81.80 |
3.25 |
2 |
79.78 |
1.25 |
0.5 |
78.59 |
0.90 |
M05 |
225 nm |
0.8 mL min−1 |
CH3OH : H2O (pH 3.0) = 63/43 |
CH3COCH3 : CH2Cl2 : phosphoric acid solution (pH 2.0) = 40/5/5 |
5 |
81.00 |
1.56 |
2 |
78.41 |
3.85 |
0.5 |
73.62 |
2.93 |
N01 |
235 nm |
0.8 mL min−1 |
CH3OH : H2O (pH 3.0) = 70/30 |
CH3COCH3 : THF : CH2Cl2 : phosphoric acid solution (pH 2.0) = 30/10/10/10 |
5 |
88.92 |
2.23 |
2 |
85.45 |
2.87 |
0.5 |
75.15 |
1.66 |
N02 |
230 nm |
0.9 mL min−1 |
CH3OH : H2O (pH 3.0) = 75/25 |
CH3COCH3 : THF : CH2Cl2 : phosphoric acid solution (pH 2.0) = 30/10/10/10 |
5 |
79.52 |
0.84 |
2 |
73.57 |
2.41 |
0.5 |
72.58 |
0.49 |
N03 |
225 nm |
0.7 mL min−1 |
CH3OH : H2O (pH 3.0) = 72/28 |
CH3COCH3 : THF : CH2Cl2 : phosphoric acid solution (pH 2.0) = 30/10/10/10 |
5 |
88.15 |
3.09 |
2 |
85.01 |
0.80 |
0.5 |
80.00 |
0.87 |
N04 |
230 nm |
0.7 mL min−1 |
CH3OH : H2O (pH 3.0) = 68/32 |
CH3COCH3 : THF : CH2Cl2 : phosphoric acid solution (pH 2.0) = 30/10/10/10 |
5 |
84.53 |
0.40 |
2 |
73.51 |
2.89 |
0.5 |
83.89 |
1.86 |
N05 |
230 nm |
1.0 mL min−1 |
CH3OH : H2O (pH 3.0) = 75/25 |
CH3COCH3 : THF : CH2Cl2 : phosphoric acid solution (pH 2.0) = 30/10/10/10 |
5 |
95.28 |
1.15 |
2 |
92.11 |
2.38 |
0.5 |
85.50 |
2.92 |
N06 |
230 nm |
1.0 mL min−1 |
CH3OH : H2O (pH 3.0) = 75/25 |
CH3COCH3 : THF : CH2Cl2 : phosphoric acid solution (pH 2.0) = 36/12/12/12 |
5 |
75.33 |
3.30 |
2 |
76.97 |
1.92 |
0.5 |
75.13 |
1.10 |
N07 |
225 nm |
0.9 mL min−1 |
CH3OH : H2O (pH 3.0) = 78/22 |
CH3COCH3 : THF : CH2Cl2 : phosphoric acid solution (pH 1.5) = 36/15/15/12 |
5 |
77.05 |
2.48 |
2 |
79.71 |
2.04 |
0.5 |
79.79 |
0.61 |
N08 |
230 nm |
0.9 mL min−1 |
CH3OH : H2O (pH 3.0) = 75/25 |
CH3COCH3 : THF : CH2Cl2 : H3PO4 aqueous solution (pH 2.0) = 30/10/10/10 |
5 |
74.10 |
2.96 |
2 |
78.17 |
2.25 |
0.5 |
74.32 |
2.69 |
N09 |
225 nm |
0.9 mL min−1 |
CH3OH : H2O (pH 3.0) = 76/24 |
CH3COCH3 : THF : CH2Cl2 : phosphoric acid solution (pH 2.0) = 30/10/10/10 |
5 |
80.53 |
7.16 |
2 |
73.28 |
2.38 |
0.5 |
74.06 |
0.37 |
N10 |
230 nm |
1.0 mL min−1 |
CH3OH : H2O (pH 3.0) = 78/22 |
CH3COCH3 : THF : CH2Cl2 : phosphoric acid solution (pH 1.5) = 36/12/12/5 |
5 |
75.01 |
2.88 |
2 |
74.30 |
2.90 |
0.5 |
77.05 |
2.40 |
N11 |
230 nm |
1.0 mL min−1 |
CH3OH : H2O (pH 3.0) = 80/20 |
CH3COCH3 : THF : CH2Cl2 : phosphoric acid solution (pH 1.5) = 36/12/12/5 |
5 |
72.75 |
2.14 |
2 |
77.47 |
1.01 |
0.5 |
72.61 |
2.43 |
N12 |
230 nm |
0.9 mL min−1 |
CH3OH : H2O (pH 3.0) = 75/25 |
CH3COCH3 : THF : CH2Cl2 : phosphoric acid solution (pH 2.0) = 30/10/10/10 |
5 |
77.35 |
1.24 |
2 |
84.32 |
2.94 |
0.5 |
86.93 |
2.10 |
N13 |
230 nm |
1.0 mL min−1 |
CH3OH : H2O (pH 3.0) = 80/20 |
CH3COCH3 : THF : CH2Cl2 : phosphoric acid solution (pH 2.0) = 30/10/10/10 |
5 |
75.17 |
2.22 |
2 |
72.08 |
0.82 |
0.5 |
77.26 |
2.35 |
Chlorsulfuron |
235 nm |
0.8 mL min−1 |
CH3OH : H2O (pH 3.0) = 60/40 |
CH3COCH3 : CH2Cl2 : phosphoric acid solution (pH 2.0) = 40/5/5 |
5 |
79.41 |
2.32 |
2 |
79.34 |
2.26 |
0.5 |
83.54 |
3.46 |
Cultivation of samples and management. The concentration of each test compound in 20 g of soil in a 100 mL conical flask was 5 mg kg−1. The regulation of 60% water holding capacity after the acetonitrile evaporated completely was implemented and the soil samples were mixed well. The soil samples were sealed with parafilm placed in a biochemical incubator to maintain the temperature (25 ± 1 °C) and humidity (80%) without light. A total of 18 soil samples were divided into six groups for every test compound, in triplicate for each group. In the process of cultivation, the moisture content of the soil samples was adjusted regularly to maintain the original water-holding state. The samples were taken periodically for HPLC analysis at six different intervals. A degradation curve consistent with the first-order kinetic equation Ct = C0 × e−kt (Ct: sample concentration (mg kg−1) at t days; C0: original sample concentration; k: the degradation rate constant; t: time (days))25–29 was established with the correlation coefficient (R2). Then, DT50 were calculated according to the formula: DT50 = ln
2/k.According to the ICAMA guidelines the following data listed in Table 2 should be undertaken first of all to ensure every step is accurate and reliable and secondly to carry out this multi-step micro-scale operation (each additive concentration was repeated 5 times). It is of prime importance that all of the results reached the set accuracy (90% ± 20%), so that the further degradation data using this procedure can be acknowledged.
An approach to predict a preferential herbicide molecule
Based on our own data, we adopted a Python (a cross-platform computer programming language that can be used for scientific computing, statistics, artificial intelligence, education, and software development, etc.) approach applying the Kriging method30 to establish a special 3D graph from which we seek a new preferential molecule, which can evaluate herbicidal activity (A), soil degradation rates (D), and crop safety (S) coordinately.
Results and discussion
Chemistry
The titled compounds (N01 and N05 were not included) and compound 18 were characterized by melting points, 1H-NMR, 13C-NMR, and HRMS (ESI 4‡). Due to the poor nucleophilicity of triazine heterocyclic amnio (compound 16), highly toxic and corrosive reagents were often used to synthesize the target compounds.31–35 The complicated process also led to difficultly in purification.8,9,36 An improved method was described using diphenyl carbonate that has low-toxicity as the carbonylation reagent to obtain the important intermediate 18. The approach can be carried out at room temperature and gave higher yields within one-step.
Crystal structure analysis
The crystal structures of M03 (CCDC number. 1975827) and N03 (CCDC number. 1975828) are shown in Fig. 3.
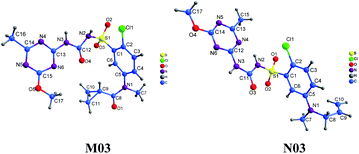 |
| Fig. 3 The crystal structures of M03 and N03. | |
The crystal structure of M03. The bond lengths of N(1)–C(5), N(2)–C(12), N(3)–C(12) are 1.431(4) nm, 1.403(4) nm, and 1.382(4) nm, respectively, which are shorter than general C–N bonds, such as the bond length of N(1)–C(7) is 1.470(4) nm. The shorter bonds may be caused by the transfer of π electrons. The sum angle of O(4)–C(12)–N(2) 123.0(3)°, O(4)–C(12)–N(3) 126.5(3)° and N(2)–C(12)–N(3) 110.5(3)° is 360°, indicating the planar sp2 hybridization state of C(12). The bond angles of O(2)–S(1)–C(1), O(3)–S(1)–C(1), and N(2)–S(1)–C(1) are 110.34(15)°, 107.49(15)°, and 105.05(15)°, respectively, which indicate that state sp3 hybridization state of the S(1). The torsion angle of O(4)–C(12)–N(2)–H(2) is −120.973(360)°, while that of O(4)–C(12)–N(3)–H(3) is 178.013(327)°. The benzene ring and the triazine ring are non-planar because their dihedral angle is 80.915(330)°. The dihedral angle between the benzene ring and the cyclopropyl is 66.957(285)°, and the dihedral angle between the triazine ring and the cyclopropyl is 78.062(308)°. The configuration of M03 is ring-shaped; the distance between C(17) and C(11) is only 3.7515(51) nm.
The crystal structure of N03. The bond lengths of N(1)–C(5), N(2)–C(11), N(3)–C(11) are 1.375(3) nm, 1.369(3) nm, and 1.382(3) nm, respectively, which are shorter than general C–N bonds; for example, the bond lengths of N(1)–C(7) and N(1)–C(8) are 1.470(4) nm and 1.447(3) nm, respectively. The shorter bond lengths are possibly caused by the transfer of π electrons. The sum angle of O(3)–C(11)–N(2) 123.9(2)°, O(3)–C(11)–N(3) 120.9(2)° and N(2)–C(11)–N(3) 115.2(2)° is 360°, indicating the planar sp2 hybridization state of C(12). The bond angles of O(1)–S(1)–C(1), O(2)–S(1)–C(1), N(2)–S(1)–C(1) are 110.24(12)°, 108.00(11)°, and 105.54(11)°, respectively, which indicates that the sp3 hybridization state of S(1). The torsion angle of O(3)–C(11)–N(2)–H(2) was −170.029(1974)°, while that of O(3)–C(11)–N(3)–H(3) was −1.858(1796)°. The benzene ring and the triazine ring are non-planar because their dihedral angle is 89.744(192)°. The dihedral angle between the benzene ring and the planar (C8, C9, C10) is 89.213(253)°; the dihedral angle between the benzene ring and the planar (C7, C8, N1) is 5.880(226)°.Formally it seems that the configuration of M03 is circular and N03 is linear.
Biological assay
The herbicidal activities of the as-synthesized compounds against representative herbs such as dicotyledons (Brassica campestris and Amaranthus tricolor) and monocotyledons (Echinochloa crusgalli and Digitaria sanguinalis) were screened through pot experiments at 15 and 150 (a. i.) g ha−1 with chlorsulfuron as the positive control. The results are shown in Table 3. The titled compounds basically maintained the super-herbicidal activity of their parent structure. M01, N03, N04, and N05 also exhibited excellent activity against Digitaria sanguinalis (pre-emergence treatment at 150 (a. i.) g ha−1) of 93.8%, 60.8%, 67.6%, and 71.6%, respectively and that of chlorsulfuron was only 30.1%. Various dialkylamino substituents decreased their herbicidal activity, e.g., N13 exhibited its herbicidal activity against Brassica campestris, Amaranthus tricolor and Echinochloa crusgalli (pre-emergence and post-emergence treatment at 15 (a. i.) g ha −1) as only 34.0 and 0%, 66.8% and 0%, and 0% and 55.2%, and that of chlorsulfuron was 93.7% and 87.5%, 97.4% and 99.7%, and 72.6% and 71.8%. It was noticed that the herbicidal spectra of individual compounds had also changed, e.g., N10 and N11 exhibited their activity against Amaranthus tricolor (post-emergence treatment at 15 and 150 (a. i.) g ha−1) as 0% and 9% and 0% and 0% and that of chlorsulfuron was 99.7% and 99.8%. Overall, most titled compounds still retained potent herbicidal activity against both dicotyledons and monocotyledons, though several structures had some limited variation.
Table 3 The herbicidal activity of the titled compounds
Compd |
Concentration (a. i.) g ha −1 |
Herbicidal activity/% |
Brassica campestris |
Amaranthus tricolor |
Echinochloa crusgalli |
Digitaria sanguinalis |
Pre |
Post |
Pre |
Post |
Pre |
Post |
Pre |
Post |
Chlorsulfuron |
15 |
93.7 |
87.5 |
97.4 |
99.7 |
72.6 |
71.8 |
8.1 |
44.8 |
150 |
97.9 |
92.3 |
98.8 |
99.8 |
89.3 |
89.5 |
30.1 |
48.3 |
M01 |
15 |
92.9 |
88.6 |
82.7 |
67.7 |
77.2 |
88.5 |
38.2 |
40.1 |
150 |
98.9 |
100 |
97.1 |
76.3 |
92.8 |
94.2 |
93.8 |
100 |
M02 |
15 |
44.1 |
18.0 |
55.2 |
0.6 |
6.4 |
60.7 |
0 |
0 |
150 |
86.7 |
79.7 |
62.5 |
67.8 |
56.2 |
79.4 |
0 |
0 |
M03 |
15 |
21.2 |
0 |
11.9 |
0 |
4.6 |
47.7 |
0 |
34.2 |
150 |
77.1 |
3.9 |
61.7 |
14.4 |
40.4 |
100 |
2.1 |
66.0 |
M04 |
15 |
0 |
0 |
23.5 |
0 |
13.1 |
70.7 |
0 |
26.1 |
150 |
83.1 |
35.2 |
70.4 |
0 |
56.1 |
100 |
0 |
36.0 |
M05 |
15 |
64.2 |
31.5 |
48.7 |
0 |
18.5 |
95.2 |
34.0 |
0 |
150 |
97.5 |
81.6 |
99.6 |
0 |
89.2 |
95.7 |
0 |
33.8 |
N01 |
15 |
74.6 |
85.8 |
69.6 |
97.0 |
77.9 |
85.8 |
74.3 |
15.0 |
150 |
88.5 |
92.1 |
92.4 |
100 |
87.8 |
88.0 |
93.2 |
36.2 |
N02 |
15 |
87.9 |
92.9 |
82.9 |
91.0 |
73.5 |
89.4 |
24.3 |
16.3 |
150 |
91.5 |
98.1 |
92.4 |
100 |
81.2 |
89.7 |
75.7 |
43.6 |
N03 |
15 |
79.5 |
67.5 |
69.6 |
96.0 |
71.3 |
72.3 |
48.6 |
13.8 |
150 |
95.8 |
73.1 |
92.4 |
99.5 |
76.8 |
78.6 |
60.8 |
35.2 |
N04 |
15 |
77.6 |
88.9 |
81.0 |
98.0 |
75.9 |
86.2 |
45.9 |
46.4 |
150 |
93.4 |
90.4 |
88.6 |
99.0 |
81.8 |
86.6 |
67.6 |
56.0 |
N05 |
15 |
81.9 |
94.7 |
77.2 |
100 |
72.4 |
83.2 |
58.1 |
11.4 |
150 |
84.3 |
96.3 |
90.5 |
100 |
83.4 |
84.8 |
71.6 |
21.8 |
N06 |
15 |
76.3 |
0 |
72.5 |
43.3 |
48.3 |
48.0 |
8.3 |
17.8 |
150 |
95.8 |
80.6 |
98.1 |
54.4 |
87.4 |
65.2 |
26.1 |
29.1 |
N07 |
15 |
65.6 |
93.5 |
79.1 |
80.0 |
64.6 |
87.2 |
25.7 |
11.0 |
150 |
90.3 |
98.8 |
92.4 |
92.0 |
82.3 |
89.8 |
68.9 |
38.1 |
N08 |
15 |
65.8 |
88.0 |
90.5 |
34.1 |
79.1 |
44.1 |
32.4 |
0 |
150 |
99.4 |
90.1 |
93.4 |
44.1 |
89.7 |
51.9 |
38.7 |
18.3 |
N09 |
15 |
57.7 |
87.3 |
44.9 |
95.0 |
56.9 |
81.4 |
51.4 |
15.4 |
150 |
87.9 |
91 |
79.1 |
97.0 |
79.0 |
85.2 |
64.9 |
26.7 |
N10 |
15 |
64.3 |
75.3 |
84.8 |
0 |
10.3 |
55.9 |
0 |
0 |
150 |
94.6 |
93.8 |
98.1 |
9.0 |
86.2 |
68.0 |
9.8 |
18.6 |
N11 |
15 |
41.0 |
34.6 |
92.1 |
0 |
0 |
15.4 |
0 |
0 |
150 |
94.6 |
88.9 |
97.2 |
0 |
81.0 |
67.8 |
0 |
4.7 |
N12 |
15 |
65.8 |
43.2 |
97.2 |
24.9 |
74.1 |
40.8 |
37.8 |
0 |
150 |
95.8 |
88.9 |
99.1 |
47.9 |
89.1 |
55.4 |
52.8 |
20.7 |
N13 |
15 |
34.4 |
0 |
66.8 |
0 |
0 |
55.2 |
17.6 |
7.1 |
150 |
73.6 |
50.9 |
97.6 |
50.9 |
74.9 |
57.0 |
38.0 |
20.1 |
Crops safety
The crop safety of M01–M05 to corn and wheat was screened through pot experiments at 30 and 60 (a. i.) g ha −1 with chlorsulfuron as a control. The experimental data are shown in Tables 4 and 5 and indicated that the compounds retain their safety to wheat, while the inhibition rate to corn greatly reduced e.g. the inhibition rate of M01 to corn decreased from 41.7% and 76.5% to 0 and 20.1% through pre-emergence treatment and from 12.1% and 33.4% to 3.4% and 12.0% through post-emergence treatment at 30 and 60 (a. i.) g ha −1, respectively. Obviously, M01 was the preferable molecule.
Table 4 The crop safety of target compounds M01–M05 to corn
Compd |
Concentration (a. i.) g ha −1 |
Corn (Xindan 66) |
Pre. (16 days after treatment) |
Post. (23 days after treatment) |
Fresh weight g per 6 strains |
Analysis of variancea |
Inhibition/% |
Fresh weight g per 6 strains |
Analysis of variancea |
Inhibition rate/% |
5% |
1% |
5% |
1% |
Among the averages, there was no significant difference if marked with the same letter and there was a significant difference among those marked with different letters. |
|
0 |
11.730 |
abcd |
ABC |
— |
12.010 |
abcdefg |
ABCDE |
— |
Chlorsulfuron |
30 |
6.843 |
e |
DEF |
41.7 |
10.560 |
cdefgh |
ABCDE |
12.1 |
60 |
2.755 |
f |
G |
76.5 |
8.002 |
h |
E |
33.4 |
M01 |
30 |
13.233 |
a |
A |
0 |
11.600 |
abcdefgh |
ABCDE |
3.4 |
60 |
9.373 |
cd |
BCDE |
20.1 |
10.567 |
cdefgh |
ABCDE |
12.0 |
M02 |
30 |
10.451 |
bcd |
ABC |
10.9 |
12.295 |
abcdefg |
ABCDE |
0 |
60 |
10.310 |
bcd |
ABC |
12.1 |
13.384 |
abcd |
ABCD |
0 |
M03 |
30 |
11.347 |
abcd |
ABC |
3.3 |
12.203 |
abcdefg |
ABCDE |
0 |
60 |
10.723 |
abcd |
ABC |
8.6 |
9.596 |
efgh |
BCDE |
20.1 |
M04 |
30 |
11.056 |
abcd |
ABC |
5.7 |
12.163 |
abcdefg |
ABCDE |
0 |
60 |
10.957 |
abcd |
ABC |
6.6 |
14.143 |
abc |
ABC |
0 |
M05 |
30 |
11.544 |
abcd |
ABC |
1.6 |
13.383 |
abcd |
ABCD |
0 |
60 |
11.473 |
abcd |
ABC |
2.2 |
8.893 |
gh |
DE |
26.0 |
Table 5 The crops safety of target compounds M01–M05 to wheat
Compd |
Concentration (a. i.) g ha −1 |
Wheat (Jimai 22) |
Pre. (22 days after treatment) |
Post. (28 days after treatment) |
Fresh weight g per cup |
Analysis of variancea |
Inhibition/% |
Fresh weight g per cup |
Analysis of variancea |
Inhibition rate/% |
5% |
1% |
5% |
1% |
Among the averages, there was no significant difference if there was one marked with the same letter, and there was a significant difference among those with different marked letters. |
|
0 |
2.853 |
defghi |
ABCDE |
— |
3.258 |
abcd |
AB |
— |
Chlorsulfuron |
30 |
3.120 |
abcdefgh |
ABCDE |
0 |
3.290 |
abcd |
AB |
0 |
60 |
2.963 |
bcdefghi |
ABCDE |
0 |
3.252 |
abcd |
AB |
0.2 |
M01 |
30 |
3.343 |
abcdef |
ABCD |
0 |
3.108 |
abcd |
ABC |
4.6 |
60 |
2.923 |
cdefghi |
ABCDE |
0 |
2.729 |
cde |
BC |
16.3 |
M02 |
30 |
3.333 |
abcdef |
ABCD |
0 |
3.296 |
abcd |
AB |
0 |
60 |
2.620 |
ghi |
CDE |
8.2 |
3.146 |
abcd |
ABC |
3.5 |
M03 |
30 |
2.902 |
cdefghi |
ABCDE |
0 |
3.084 |
abcd |
ABC |
5.3 |
60 |
3.150 |
abcdefgh |
ABCDE |
0 |
2.976 |
bcd |
ABC |
8.7 |
M04 |
30 |
2.850 |
defghi |
ABCDE |
0.1 |
3.484 |
abc |
AB |
0 |
60 |
2.733 |
fghi |
BCDE |
4.2 |
3.603 |
ab |
AB |
0 |
M05 |
30 |
2.777 |
efghi |
BCDE |
2.7 |
3.743 |
a |
A |
0 |
60 |
2.760 |
efghi |
BCDE |
3.3 |
3.246 |
abcd |
AB |
0.4 |
Chlorsulfuron |
30 |
3.120 |
abcdefgh |
ABCDE |
0 |
3.290 |
abcd |
AB |
0 |
60 |
2.963 |
bcdefghi |
ABCDE |
0 |
3.252 |
abcd |
AB |
0.2 |
Soil degradation
The degradation curves of the first-order kinetic equations of the test compounds were established according to the 6 times of extraction (ESI 5‡). Then, DT50 of the test compounds were calculated (Table 6). The degradation data indicated that the DT50 of M01–M05 was 6.66–9.76 days and the range of the DT50 of N01–N13 was 3.57–10.66 days, while the DT50 of chlorsulfuron was 13.08 days. Overall, the DT50 of the test compounds was 3.57–10.66 days. Their degradation rate was faster to varying degrees than chlorsulfuron in acidic soil.
Table 6 Kinetic parameters for acidic soil (pH 5.52) degradation
Compd |
First-order kinetic equations |
Correlation coefficient (R2) |
DT50/days |
M02 |
Ct = 4.2429 e−0.104t |
0.9853 |
6.66 |
M05 |
Ct = 3.8798 e−0.093t |
0.9872 |
7.45 |
M01 |
Ct = 4.1745 e−0.089t |
0.9763 |
7.79 |
M04 |
Ct = 4.2067 e−0.086t |
0.9855 |
8.06 |
M03 |
Ct = 3.7700 e−0.071t |
0.9981 |
9.76 |
N01 |
Ct = 4.3050 e−0.194t |
0.9870 |
3.57 |
N06 |
Ct = 3.6110 e−0.156t |
0.9910 |
4.44 |
N02 |
Ct = 4.1393 e−0.137t |
0.9921 |
5.06 |
N04 |
Ct = 4.0699 e−0.132t |
0.9966 |
5.25 |
N03 |
Ct = 4.4109 e−0.120t |
0.9887 |
5.78 |
N08 |
Ct = 4.0214 e−0.113t |
0.9936 |
6.13 |
N05 |
Ct = 4.6677 e−0.095t |
0.9915 |
7.30 |
N07 |
Ct = 3.8053 e−0.095t |
0.9907 |
7.30 |
N11 |
Ct = 3.8549 e−0.093t |
0.9725 |
7.45 |
N09 |
Ct = 3.9869 e−0.082t |
0.9985 |
8.45 |
N12 |
Ct = 3.8541 e−0.077t |
0.9940 |
9.00 |
N10 |
Ct = 3.5662 e−0.071t |
0.9956 |
9.76 |
N13 |
Ct = 3.4493 e−0.065t |
0.9800 |
10.66 |
Chlorsulfuron |
Ct = 3.7001 e−0.053t |
0.9874 |
13.08 |
Structure/degradation relationship graph
On the basis of Table 6 and referring to the initial information from each degradation curve obtained in our laboratory (referring to ESI 5‡ for detail), 19 titled compounds (M01–M05 and N01–N13, plus chlorsulfuron as the control) had their degradation process in an acidic soil tracked (pH 5.52). At least 6 points for each structure were recorded in detail. In summing of all these data, a collective structure/degradation relationship graph was first depicted as following (Fig. 4). This is a new relationship graph established from our numerous experimental data from which some valuable information could be obtained.
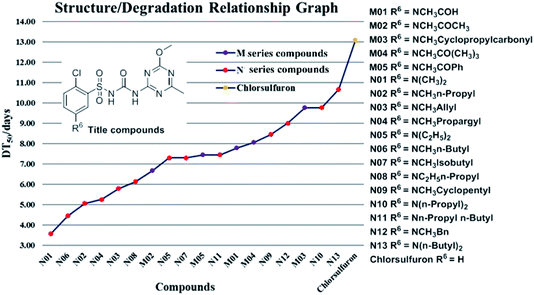 |
| Fig. 4 Structure/degradation relationship graph in pH 5.52 soil. | |
From the information above a preliminary conclusion could be summarized as follows:
(1) Design and syntheses of the M and N series of 18 titled new molecules were designed and modified from the structure of chlorsulfuron. At the 5th position of the benzene ring of chlorsulfuron, any delicate alteration on the amino-moiety would significantly change each soil degradation rate.
(2) The DT50 of M01–M05 accelerated to 6.66–9.76 days and that of N01–N13 accelerated to 3.57–10.66 days compared with chlorsulfuron (13.08 days in pH 5.52 soil). It was interesting to note that most new structures have faster degradation rates and retained the potent herbicidal properties of chlorsulfuron.
(3) Dialkylamino-analogs (N01, N05, N09, and N13) altered their degradation rates sequentially following the alkyl sequence (CH3)2, (C2H5)2, (C3H7)2 or (C4H9)2, though all of them degraded faster than chlorsulfuron (Fig. 4).
(4) If the nitrogen atoms contained the same number of carbon atoms, DT50 was longer when the carbon atoms on both sides of the nitrogen atom were distributed symmetrically (e.g. DT50 of N02 and N05, N12 and N13 are 5.06 and 7.45, 9.00 and 10.66 days, respectively).
(5) M01 and N01 (Table 3) exhibited better herbicidal activity against Digitaria sanguinalis in pre-emergence treatment, while in the rotation crop planting system, M01, M03, and M05 were much safer to corn than chlorsulfuron (Table 4).
Preferential herbicide molecule
We selected 5 new molecules M01–M05 and calculated herbicidal activity (A) toward Brassica napus (pre-emergence, at 15 (a. i.) g ha −1), a degradation rate in acidic soil expressed by DT50 (D), and crop (corn) safety (S) expressed by the crop inhibition rate. In Fig. 5, the blue curvature denoted the preponderant domain, while the deeper blue color was more weighted to “hit” on an “ideal” candidate. The red part denoted a relationship further from the “ideal” molecule. The calculation results indicated that M01 situated at the peak at the lowest part of the blue curvature, which possesses simultaneously a high herbicidal bio-activity (60–95%) (A), DT50 (D) within 8 days (much less than 13.08 days for chlorsulfuron), and crop safety (S) toward corn close to zero, which means no inhibition towards corn seedlings. Referring to the original experimental data, the “ideal” molecule within the calculation was cross-checked to be structure M01. This was a preliminary attempt to predict a preferential (ideal) molecule in which the 3 important correlated factors (A, D, S) were all taken into account at the same time. This result encourages us to carry out more studies based on a larger sample size in the future.
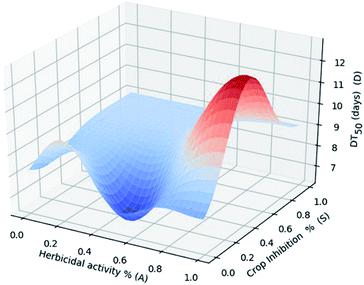 |
| Fig. 5 The relationship among bio-activity (A), degradation rates (D) and crop safety (S). | |
Conclusions
Based on our former research, the design and modification of new structures from classical chlorsulfuron, with the hope of improving its ecological properties, was undertaken. With the introduction of various N-methylamido and dialkylamino groups into the chlorsulfuron structure, two series of 18 new structures M01–M05 and N01–N13 were synthesized using improved methods. Their structural characterization, biological evaluation, crop safety, and degradation rates were successfully studied. It was found that most of the titled structures retained their herbicidal activity but their degradation rates became faster, while a few structures presented some unexpected results, within which new ecological merits could be observed. As a result, a systematic structure/degradation relationship of the new sulfonylureas was established for the first time. An activity(A)/degradation(D)/safety(S) relationship was also suggested, with which an approach to predict a preferential herbicide molecule with improved ecological consideration was explored. This is a preliminary exploration in this new field. These new molecules, though structurally derived from chlorsulfuron, definitely exhibited different degradation behavior in our laboratory, yet their complicated toxicological assessment, metabolic pathway, and environmental impacts are awaiting clarification if their further development requires. With increasing consideration and growing concern over our environmental and ecological prospects, the present study could give us more insight to reach our original goal, i.e. to develop new tailored SU herbicides with controllable degradation to meet the needs of individual crops.
Conflicts of interest
There are no conflicts to declare.
Acknowledgements
This work was supported by “the Fundamental Research Funds for the Central Universities”, Nankai University (No. 63181206), the State Key Laboratory of Elemento-Organic Chemistry (Nankai University), and Syngenta PhD Scholarship.
References
- T. B. Ray, Plant Physiol., 1984, 75, 827–831 CrossRef CAS PubMed.
- T. Lonhienne, A. Nouwens, C. M. Williams, J. A. Fraser, Y. T. Lee, N. P. West and L. W. Guddat, Angew. Chem., 2016, 55, 4247–4251 CrossRef CAS PubMed.
- Ministry of Agriculture of the People's Republic of China, Bulletin of the Ministry of Agriculture of the People's Republic of China, 2014, p. 53, Announcement No. 2032 Search PubMed.
- C. Marucchini, C. Vischetti and M. Businelli, Agrochimical, 1991, 35, 69–77 CAS.
- A. K. Sarmah, R. S. Kookana, M. J. Duffy, A. M. Alston and B. D. Harch, Pest Manage. Sci., 2000, 56, 463–471 CrossRef CAS.
- A. Walker, E. G. Cotterill and S. J. Welch, Weed Res., 1989, 29, 281–287 CrossRef CAS.
- K. Thirunarayanan, R. L. Zimdahl and D. E. Smika, Weed Sci., 1985, 33, 558–563 CrossRef CAS.
- X. W. Hua, M. G. Chen, S. Zhou, D. K. Zhang, M. Liu, S. Zhou, J. B. Liu, K. Lei, H. B. Song, Y. H. Li, Y. C. Gu and Z. M. Li, RSC Adv., 2016, 6, 23038–23047 RSC.
- X. Hua, S. Zhou, M. Chen, W. Wei, M. Liu, K. Lei, Y. Li, S. Zhou, B. Wang and Z. Li, Chin. J. Chem., 2016, 34, 1135–1142 CrossRef CAS.
- S. Zhou, X. W. Hua, W. Wei, M. G. Chen, Y. C. Gu, S. Zhou, H. B. Song and Z. M. Li, Chin. Chem. Lett., 2018, 29, 945–948 CrossRef CAS.
- N. T. Pokhodylo, V. S. Matiychuk and M. D. Obushak, Synthesis, 2009, 14, 2321–2323 CrossRef.
- J. W. Leahy, C. A. Buhr, H. W. Johnson, B. G. Kim, T. Baik, J. Cannoy, T. P. Forsyth, J. W. Jeong, M. S. Lee, S. Ma, K. Noson, L. Wang, M. Williams, J. M. Nuss, E. Brooks, P. Foster, L. Goon, N. Heald, C. Holst, C. Jaeger, S. Lam, J. Lougheed, L. Nguyen, A. Plonowski, J. Song, T. Stout, X. Wu, M. F. Yakes, P. Yu, W. Zhang, P. Lamb and O. Raeber, J. Med. Chem., 2012, 55, 5467–5482 CrossRef CAS PubMed.
- Y. Zheng, J. C. Mao, J. Chen, G. W. Rong, D. F. Liu, H. Yan, Y. J. Chi and X. F. Xu, RSC Adv., 2015, 5, 50113–50117 RSC.
- L. Shi, L. P. Zhou, G. K. Dai, N. Y. Wang and D. L. An, Talanta, 2013, 115, 386–393 CrossRef CAS PubMed.
- P. J. Klein, M. Chomet, A. Metaxas, J. A. M. Christiaans, E. Kooijman, R. C. Schuit, A. A. Lammertsma, B. N. M. Berckel and A. D. Windhorst, Eur. J. Med. Chem., 2016, 118, 143–160 CrossRef CAS PubMed.
- V. Dudutienė, A. Zubrienė, A. Smirnov, J. Gylytė, D. Timm, E. Manakova, S. Gražulis and D. Matulis, Bioorg. Med. Chem., 2013, 21, 2093–2106 CrossRef PubMed.
- L. Pan, Y. Jiang, Z. Liu, X. H. Liu, Z. Liu, G. Wang, Z. M. Li and D. Wang, Eur. J. Med. Chem., 2012, 50, 18–26 CrossRef CAS PubMed.
- G. Brahmachari and S. Laskar, Tetrahedron Lett., 2010, 51, 2319–2322 CrossRef CAS.
- G. M. Sheldrick, SHELXTL, Version 5.0, University of Göttingen, Göttingen, Germany, 2001 Search PubMed.
- B. L. Wang, R. G. Duggleby, Z. M. Li, J. G. Wang, Y. H. Li, S. H. Wang and H. B. Song, Pest Manage. Sci., 2005, 61, 407–412 CrossRef CAS PubMed.
- S. R. Teaney, L. Armstrong, K. Bentley, D. Cotterman, D. Leep, P. H. Liang, C. Powley, J. Summers, S. Cranwell, F. Lichtner and R. Stichbury, Brighton Crop Prot. Conf.--Weeds, 1995, 1, 49 Search PubMed.
- The Institute for the Control of Agrochemicals under the Ministry of Agriculture, Chinese National Standard GB/T 31270.1-2014. Test guidelines on environmental safety assessment for chemical pesticides-Part 1: Transformation in soils, Oct 10, 2014 Search PubMed.
- National Chemical Standardization Technical Committee, Chinese National Standard GB/T 16631-2008. General rules for high performance liquid chromatography, Jun 18, 2008 Search PubMed.
- Ministry of Agriculture and Pesticide Testing Center, Chinese Agricultural Industry Standard NY/T 788-2004. Guideline on pesticide residue trials, Apr 16, 2004 Search PubMed.
- K. Thirunarayanan, R. L. Zimdahl and D. E. Smika, Weed Sci., 1985, 33, 558–563 CrossRef CAS.
- G. Levitt, H. L. Ploeg, R. C. J. Weigel and D. J. Fitzgerald, J. Agric. Food Chem., 1981, 29, 416–418 CrossRef CAS.
- F. K. Oppong and G. R. Sagar, Weed Res., 1992, 32, 167–173 CrossRef CAS.
- J. W. Hamaker and C. A. I. Goring, ACS Symp. Ser. Am. Chem. Soc., 1976, 9, 219–243 Search PubMed.
- S. Zhou, X. W. Hua, W. Wei, Y. C. Gu, X. Q. Liu, J. H. Chen, M. G. Chen, Y. T. Xie, S. Zhou, X. D. Meng, Y. Zhang, Y. H. Li, B. L. Wang, H. B. Song and Z. M. Li, J. Agric. Food Chem., 2017, 65, 7661–7668 CrossRef CAS PubMed.
- J. Cortes, IEEE Trans. Autom. Control, 2009, 54, 2816–2827 Search PubMed.
- W. Meyer and W. Forty, US Pat. 4419121, 1983.
- G. Levitt, US Pat. 4169719, 1979.
- G. Levitt, US Pat. 4225337, 1980.
- E. G. Lepone, EP Pat. 74282, 1983.
- G. Levitt, US Pat. 4818277, 1989.
- K. Muth, W. Aumueller, R. Weyer and H. Weber, US Pat. 3420882, 1969.
Footnotes |
† This paper is dedicated to Professor Youyou Tu, the 2015 Nobel Prize Laureate of Physiology or Medicine on the occasion of her 90th birthday. |
‡ Electronic supplementary information (ESI) available. CCDC 1975827 and 1975828. For ESI and crystallographic data in CIF or other electronic format see DOI: 10.1039/d0ra00811g |
|
This journal is © The Royal Society of Chemistry 2020 |
Click here to see how this site uses Cookies. View our privacy policy here.