DOI:
10.1039/D0RA02631J
(Paper)
RSC Adv., 2020,
10, 17288-17292
SO2F2-Mediated one-pot cascade process for transformation of aldehydes (RCHO) to cyanamides (RNHCN)†
Received
22nd March 2020
, Accepted 21st April 2020
First published on 4th May 2020
Abstract
A simple, mild and practical cascade process for the direct conversion of aldehydes to cyanamides was developed featuring a wide substrate scope and great functional group tolerability. This method allows for transformations of readily available, inexpensive, and abundant aldehydes to highly valuable cyanamides in a pot, atom, and step-economical manner with a green nitrogen source. This protocol will serve as a robust tool for the installation of the cyanamide moiety in various complicated molecules.
As a class of multistep, one-pot processes without the separation of intermediates, the cascade (tandem or domino) reactions have been acknowledged as one of the most powerful tools in modern chemistry with the features of atom-economy, saving power and consumption, better resource management, easy purification and lowest waste generation while still providing a higher yield than the traditional reactions.1 Therefore, designing controllable cascade reactions with excellent molecular efficiencies and high selectivity is a very challenging but rather highly desirable and strategic key element for modern synthetic and sustainable chemistry.2 Cyanamides represent the core motif in biologically active molecules and have been widely used in pharmaceuticals and functionalized materials.3 As a reactive N–C–N building block, cyanamides are more commonly used as a precursor in the synthesis of pharmaceutically important N-containing heterocycles and N-alkyl or N-aryl imides.4 Despite their versatile applications, only a limited number of synthetic routes have been reported for cyanamides in the literature.5 The most frequently adopted method is the direct cyanation of amines using cyanogen halides,6 which is overshadowed by its acute toxicity, unfavorable physical properties and sensitivity to moisture.7 Another straightforward approach is the direct alkylation of cyanamides; however, N,N-dialkylated cyanamides are usually obtained due to the competing alkylation of monoalkylated cyanamides.8 Other approaches include the dehydrosulfurization of thiourea,9 the dehydration of urea, and the conversion from isocyanides, isocyanates, or isothiocyanates.10 These above methods are mutually complementary since they all originate from the corresponding amines with multistep manipulations. In addition, some of the transformations require harsh conditions or hazardous reagents. Recently, several new cyanide sources, including CuCN,11 AIBN,12 TMSCN,13 and imidazolium thiocyanates,14 were employed in the direct N-cyanation of amines to synthesize cyanamides. As an alternative approach, the Tiemann rearrangement of amidoximes attracted chemists' interest in the synthesis of cyanamides.15 In 2014, Chien reported that benzenesulfonyl chlorides (TsCl or o-NsCl) promoted the Tiemann rearrangement of amidoximes to generate the corresponding cyanamides.16 However, it was highly dependent on the electronic effect of the substrates and required rigorous reaction conditions, pre-synthesis of substrates and redundant work-up.
Recently, sulfuryl fluoride (SO2F2),17 an inexpensive (about 1$ per kg), abundant and relatively inert electrophile (stable up to 400 °C when dry) has attracted significant attention to be used for SuFEx click chemistry and other versatile manipulations.18 A perusal of the literature revealed that the protons of phenolic hydroxyls or oximes hydroxyls can activate the exchange of the S–F bonds in SO2F2 for the S–O bonds to afford functional products, and the fluorosulfate functional group (–OSO2F) can be applied in a controllable and targeted manner for varied transformations.19 Most recently, our group reported a mild and robust method for efficiently converting aldoximes into the corresponding nitriles mediated by SO2F2/base in a green manner (Scheme 1, a).20a Subsequently, an efficient method for the activation of the Beckmann rearrangement of ketoximes into amides or lactams utilizing SO2F2 was developed in our lab(Scheme 1, b).20b Coincidentally, we found that SO2F2 could also promote the Tiemann rearrangement of amidoximes which were generated from corresponding nitriles to generate the corresponding cyanamides in good to excellent yields (Scheme 1, c).20c Upon viewing the high value of cyanamide moieties, the easy availability of aldehydes, and our continuous efforts on the utilization of SO2F2 for chemical transformations of oximes (aldoximes, ketoximes, and amidoximes),20 we proposed a one-pot process for direct conversion of aldehydes to cyanamides through a cascade sequence following similar mechanism as our cascade nitrile synthesis process. We envisioned that in common polar solvent acetonitrile (CH3CN), aldehydes 1 would react with NH2OH to provide the aldoxime intermediate A after dehydration, and the aldoxime will further react with SO2F2 to generate the corresponding sulfonyl ester B, and with the assistance of the base, the following β-elimination of the precursor sulfonyl ester B would generate the desired carbon–nitrogen triple bonds of nitriles C. Subsequently, the nitriles are transformed to the amidoxime intermediate D reacting with NH2OH through a nucleophilic addition and dehydration process; then the amidoxime was deprotonated with SO2F2 under the promotion of the base to form the corresponding sulfonyl ester E, and the N–O bond cleavage occurred with concomitant R group migration over to the C–N bond to furnish the N-substituted cyanamides 2 (Scheme 1, d).
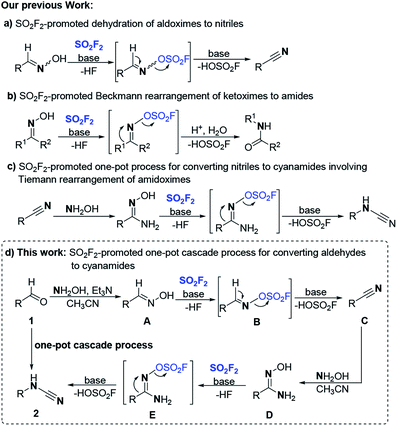 |
| Scheme 1 Our works on transforming of oximes mediated by SO2F2. | |
We conducted our initial study with benzaldehyde 1a as the model substrate to examine the feasibility of the proposed transformation. Accordingly, after screening a large variety of conditions as shown in Table 1. Considering of inorganic bases have significant advantages over their organic counterparts,21 inorganic bases, including K2CO3, Na2CO3, KHCO3, NaHCO3 and Na3PO4, were firstly screened (entries 1–5). Although inorganic bases were more advantageous than organic bases in Qin's oxidation system,19 we were disappointed to find that the use of inorganic bases provided only a trace amount of the desired product 2a. It is worth noting that the use of 1,8-diazabicyclo[5.4.0]undec-7-ene (DBU) and N,N-diisopropylethylamine (DIPEA) provided 40% and 68% isolated yields of the desired product, respectively, while the use of triethylamine (Et3N) assisted the reaction more efficiently to generate the desired product 2a in a great isolated yield of 94% (entries 6–8). It is not surprising to find that fixing Et3N as base 1 and switching base 2 to DBU and DIPEA, or fixing Et3N as base 2 and switching base 1 to DBU and DIPEA caused varying degrees decreased yields of the product 2a (entries 9–12).20 Hence, further studies were carried out by using Et3N as base since it provided the best yield of 2a to 94% isolated yield (entry 8). Although solid NH2OH·HCl is easier to operate and more inexpensive that 50 wt% NH2OH (aqueous solution), the moderate decreased yields of 2a were occurred when using NH2OH·HCl instead of 50 wt% NH2OH as the nitrogen source (entries 13, 14). Subsequently, in order to simplify the operation, the reaction mixture wasn't concentrated and carried out in CH3CN in the fourth step of one-pot process, however, efficiency was sharply decreased (entry 15). Therefore, the conditions of entry 8 were chosen as the standard procedure for the examinations of functional group tolerability and substrate scope.
Table 1 Optimization of reaction conditionsa
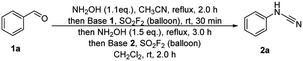
|
Entry |
Base 1 (2.0 equiv.) |
Base 2 (2.0 equiv.) |
Yieldb (2a, %) |
Reaction conditions: benzaldehyde 1a (1.0 mmol), 50 wt% NH2OH (1.2 mmol, 1.2 equiv.), CH3CN (10 mL), reflux, 2.0 h; then Base 1 (2.0 mmol, 2.0 equiv.), and SO2F2 balloon, r.t., 30 min; then 50 wt% NH2OH (1.5 mmol, 1.5 equiv.), reflux, 3.0 h; then the mixture was concentrated, base 2 (2.0 mmol, 2.0 equiv.), CH2Cl2 (10 mL), and SO2F2 balloon, r.t., 2.0 h. Isolated yields. NH2OH.HCl (1.2 mmol, 1.2 equiv.) and Base 1 (1.5 mmol, 1.5 equiv.) were used to replace 50 wt% NH2OH in the first step of one-pot process. NH2OH.HCl (1.5 mmol, 1.5 equiv.) and Base 2 (2.0 mmol, 2.0 equiv.) were used to replace 50 wt% NH2OH in the third step of one-pot process. The reaction mixture wasn't concentrated, and carried out in CH3CN in the fourth step of one-pot process. |
1 |
K2CO3 |
K2CO3 |
5 |
2 |
Na2CO3 |
Na2CO3 |
<1 |
3 |
KHCO3 |
KHCO3 |
<1 |
4 |
NaHCO3 |
NaHCO3 |
<1 |
5 |
Na3PO4 |
Na3PO4 |
7 |
6 |
DBU |
DBU |
40 |
7 |
DIPEA |
DIPEA |
68 |
8 |
Et3N |
Et3N |
94 |
9 |
Et3N |
DBU |
47 |
10 |
Et3N |
DIPEA |
75 |
11 |
DBU |
Et3N |
86 |
12 |
DIPEA |
Et3N |
89 |
13c |
Et3N |
Et3N |
90 |
14d |
Et3N |
Et3N |
78 |
15e |
Et3N |
Et3N |
51 |
Next, we evaluated the substrate scopes, functional group compatibility and limitation of the one-pot cascade process (Table 2). In most cases, the corresponding aldehydes, including aromatic and aliphatic substituted aldehydes, were successfully furnished in moderate to great yields (2a–2y). Both electron-donating (2b–2e) and electron-withdrawing groups (2f–2i) were all well tolerated under the standard reaction conditions. Notably, the satisfactory results showed that the position of the substituents on the aryl rings exhibited insignificant influence on the efficiency (2b, 2g, 2h vs. 2k–2m vs. 2n–2p). Furthermore, multi-functionalized benzylic aldehydes (2q, 2r) and naphthalene aldehydes (2s, 2t) were also successfully converted into their corresponding cyanamide products in moderated to great yields. Excitingly, a set of heterocyclic benzylic aldehydes (2u, 2v) were successfully converted into their corresponding cyanamides in acceptable yields. Besides, the phenylpropiolaldehyde 1w was also converted to give the final product at a 70% isolated yield (2w). For aliphatic moieties, the representative aldehydes (2x–2z) were also successfully transformed into their corresponding cyanamides with moderate yields.
Table 2 Scope of this SO2F2-promoted one-pot cascade process.a,b
Reaction conditions: aldehyde 1 (1.0 mmol), 50 wt% NH2OH (1.2 mmol, 1.2 equiv.), CH3CN (10 mL), reflux, 2.0 h; then Et3N (2.0 mmol, 2.0 equiv.), and SO2F2 balloon, r.t., 30 min; then 50 wt% NH2OH (1.5 mmol, 1.5 equiv.), reflux, 3.0 h; then the mixture was concentrated, Et3N (2.0 mmol, 2.0 equiv.), CH2Cl2 (10 mL), and SO2F2 balloon, r.t., 2.0 h. Isolated yields. Stirring 6.0 h for the fourth step of one-pot process. |
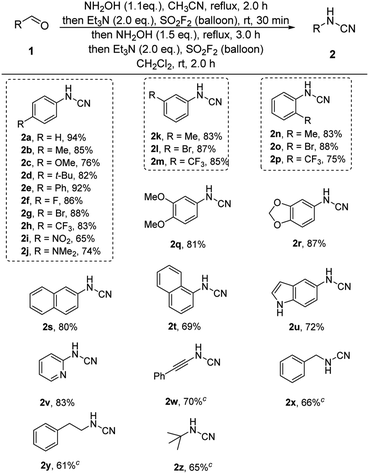 |
In order to further demonstrate the practicality of this novel cascade process, a gram-scale (20 mmol, 2.12 g) reaction was performed under standard conditions (Scheme 2). The desired N-phenylcyanamide 2a was obtained with 85% isolated yield. Since the resulting product N-phenylcyanamide 2a is widely applied as an estimable building block in the direct and efficient synthesis of many bioactive molecules, this protocol is particularly useful. Examples include tetrazolamine 3,22 urea 4,23 amide 5,24 guanidine 6,25 2-aminoquinazolin-4-one 7,26 and aminobenzonitrile 8.27 These representative transformations clearly demonstrate the versatility of cyanamides in organic chemistry.
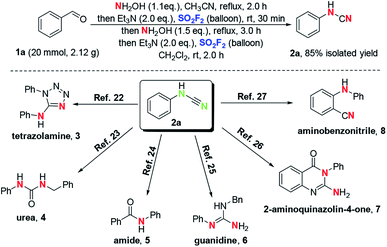 |
| Scheme 2 The gram-scale preparation and further transformations of N-phenylcyanamide 2a. | |
Conclusions
In conclusion, we disclosed a new one-pot cascade process which allowed transformation of a broad range of inexpensive, easily accessible and abundant aldehydes into cyanamides with green nitrogen source. This reported SO2F2-modiated reaction proceeded with the features of mild condition, high efficiency, wide scope, and excellent functional group compatibility. Moreover, gram-scale reaction was performed to demonstrate the applicability of cyanamides, which could be efficiently converted to various structures.
Conflicts of interest
There are no conflicts to declare.
Notes and references
-
(a) P. Anastas and N. Eghbali, Chem. Soc. Rev., 2010, 39, 301 RSC;
(b) K. C. Nicolaou and J. S. Chen, Chem. Soc. Rev., 2009, 38, 2993 RSC;
(c) K. C. Nicolaou, T. Montagnona and S. A. Snyder, Chem. Commun., 2003, 551 RSC;
(d) P. J. Parsons, C. S. Penkett and A. J. Shell, Chem. Rev., 1996, 96, 195 CrossRef CAS PubMed.
-
(a) Y. Hayashi, Chem. Sci., 2016, 7, 866 RSC;
(b) I. Wheeldon, S. D. Minteer, S. Banta, S. C. Barton, P. Atanassov and M. Sigman, Nat. Chem., 2016, 8, 299 CrossRef CAS PubMed;
(c) A. Behr, A. J. Vorholt, K. A. Ostrowski and T. Seidensticker, Green Chem., 2014, 16, 982 RSC;
(d) K. Sanderson, Nature, 2011, 469, 18 CrossRef CAS PubMed;
(e) C. Grondal, M. Jeanty and D. Enders, Nat. Chem., 2010, 2, 167 CrossRef CAS.
-
(a) A. Ricci, Amino Group Chemistry From Synthesis to the Life Sciences, Wiley-VCH, Weinheim, 2008 Search PubMed;
(b) A. Kleemann, A. Engel, B. Kutscher and D. Reichert, Pharmaceutical sustance: Synthesis Patents, Applications, Georg Thieme, Stuttgart, 4th edn, 2001 Search PubMed;
(c) J. P. Falgueyret, R. M. Oballa, O. Okamoto, G. Wesolowski, Y. Aubin, R. M. Rydzewski, P. Prasit, D. Riendeau, S. B. Rodan and N. D. Percival, J. Med. Chem., 2001, 44, 94 CrossRef CAS;
(d) A. F. Kluge, B. R. Lagu, P. Maiti, M. Jaleel, M. Webb, J. Malhotra, A. Mallat and P. A. Srinivas, Bioorg. Med. Chem. Lett., 2018, 28, 2655 CrossRef CAS PubMed.
-
(a) J. L. La Mattina, J. Heterocycl. Chem., 1983, 20, 533 CrossRef CAS;
(b) R. W. Stephens, L. A. Domeier, M. G. Todd and V. A. Nelson, Tetrahedron Lett., 1992, 33, 733 CrossRef CAS;
(c) T. Cai, M. Xian and P. G. Wang, Bioorg. Med. Chem. Lett., 2002, 12, 1507 CrossRef CAS;
(d) V. Kumar, M. P. Kaushik and A. Mazumdar, Eur. J. Org. Chem., 2008, 1910 CrossRef.
-
(a) M. R. R. Prabhath, L. Williams, S. V. Bhat and P. Sharma, Molecules, 2017, 22, 615 CrossRef PubMed;
(b) M. H. Larraufie, G. Maestri, M. Malacria, C. Ollivier, L. Fensterbank and E. Lacote, Synthesis, 2012, 44, 1279 CrossRef CAS;
(c) D. D. Nekrasov, Russ. J. Org. Chem., 2004, 40, 1387 CrossRef CAS.
-
(a) G. Kaupp, J. Schmeyers and J. Boy, Chem.–Eur. J., 1998, 4, 2467 CrossRef CAS;
(b) J. Van Barun, Ber. Dtsch. Chem. Ges., Beil., 1900, 33, 1468 Search PubMed.
- W. E. Luttrell, J. Chem. Health Saf., 2009, 16, 29 CrossRef CAS.
-
(a) J. N. Ayres, M. W. Ashford, Y. Stöckl, V. Prudhomme, K. B. Ling, J. A. Platts and L. C. Morrill, Org. Lett., 2017, 19, 3835 CrossRef CAS PubMed;
(b) R. J. Crutchley, Coord. Chem. Rev., 2001, 219, 125 CrossRef.
-
(a) A. M. Van Leusen and J. C. Jagt, Tetrahedron Lett., 1970, 12, 967 CrossRef;
(b) R. C. Wheland and E. L. J. Martin, J. Org. Chem., 1975, 40, 3101 CrossRef CAS;
(c) W. A. Davis and M. P. J. Cava, J. Org. Chem., 1983, 48, 2774 CrossRef CAS;
(d) T. V. Hughes, S. D. Hammond and M. P. J. Cava, J. Org. Chem., 1998, 63, 401 CrossRef CAS;
(e) Y. Q. Wu, D. C. Limburg, D. E. Wikinson and G. S. Hamiton, Org. Lett., 2000, 2, 795 CrossRef CAS PubMed.
-
(a) K. Škoch, I. Cíarová and P. Štěpnicka, Chem.–Eur. J., 2018, 24, 13788 CrossRef PubMed;
(b) C. Y. Chen, F. F. Wong, J. J. Huang, S. K. Lin and M. Y. Yeh, Tetrahedron Lett., 2008, 49, 6505 CrossRef CAS.
- F. Teng, J. T. Yu, Y. Jiang, H. Yang and J. Cheng, Chem. Commun., 2014, 50, 8412 RSC.
- F. Teng, J. T. Yu, Z. Zhou, H. Chu and J. Cheng, J. Org. Chem., 2015, 80, 2822 CrossRef CAS PubMed.
- C. Zhu, J. B. Xia and C. Chen, Org. Lett., 2014, 16, 247 CrossRef CAS PubMed.
- G. Talavera, J. Peña and M. Alcarazo, J. Am. Chem. Soc., 2015, 137, 8704 CrossRef CAS PubMed.
-
(a) F. Tiemann, Ber. Dtsch. Chem. Ges., Beil., 1891, 24, 4162 CrossRef;
(b) S. A. Bakunov, A. V. Rukavishnikov and A. V. Tkachev, Synthesis, 2000, 1148 CrossRef CAS.
- C. C. Lin, T. H. Hsieh, P. Y. Liao, Z. Y. Liao, C. W. Chang, Y. C. Shih, W. H. Yeh and T. C. Chien, Org. Lett., 2014, 16, 892 CrossRef CAS PubMed.
-
(a) J. Dong, L. Krasnova, M. G. Finn and K. B. Sharpless, Angew. Chem., Int. Ed., 2014, 53, 9430 CrossRef CAS PubMed;
(b) Sulfuryl fluoride (SO2F2) has been produced annually at more than 3 million kilograms per year since 2000, with a price as low as $1 per kg;
(c) SO2F2 is commercially available from chemical vendors worldwide, http://synquestlabs.com/product/id/51619.html.
- For examples of SO2F2 in SuFEx click chemistry, see:
(a) W. Chen, J. Dong, L. Plate, D. E. Mortenson, G. J. Brighty, S. Li, Y. Liu, A. Galmozzi, P. S. Lee, J. J. Hulce, B. F. Cravatt, E. Saez, E. T. Powers, I. A. Wilson, K. B. Sharpless and J. W. Kelly, J. Am. Chem. Soc., 2016, 138, 7353 CrossRef CAS PubMed;
(b) B. Gao, L. Zhang, Q. Zheng, F. Zhou, L. M. Klivansky, J. Lu, Y. Liu, J. Dong, P. Wu and K. B. Sharpless, Nat. Chem., 2017, 9, 1083 CrossRef CAS PubMed;
(c) H. Wang, F. Zhou, G. Ren, Q. Zheng, H. Chen, B. Gao, L. Klivansky, Y. Liu, B. Wu, Q. Xu, J. Lu, K. B. Sharpless and P. Wu, Angew. Chem., Int. Ed., 2017, 56, 11203 CrossRef CAS PubMed;
(d) Q. Zhao, X. Ouyang, X. Wan, K. S. Gajiwala, J. C. Kath, L. H. Jones, A. L. Burlingame and J. Taunton, J. Am. Chem. Soc., 2017, 139, 680 CrossRef CAS PubMed;
(e) Z. Liu, J. Li, S. Li, G. Li, K. B. Sharpless and P. Wu, J. Am. Chem. Soc., 2018, 140, 2919 CrossRef CAS PubMed;
(f) D. E. Mortenson, G. J. Brighty, L. Plate, G. Bare, W. Chen, S. Li, H. Wang, B. F. Cravatt, S. Forli, E. T. Powers, K. B. Sharpless, I. A. Wilson and J. W. Kelly, J. Am. Chem. Soc., 2018, 140, 200 CrossRef CAS PubMed;
(g) N. Wang, B. Yang, C. Fu, H. Zhu, F. Zheng, T. Kobayashi, J. Liu, S. Li, C. Ma, P. G. Wang, Q. Wang and L. Wang, J. Am. Chem. Soc., 2018, 140, 4995 CrossRef CAS PubMed.
- For some selected examples of using SO2F2 in chemical transformations, see:
(a) P. S. Hanley, T. P. Clark, A. L. Krasovskiy, M. S. Ober, J. P. O'Brien and T. S. Staton, ACS Catal., 2016, 6, 3515 CrossRef CAS;
(b) S. D. Schimler, M. A. Cismesia, P. S. Hanley, R. D. J. Froese, M. J. Jansma, D. C. Bland and M. S. Sanford, J. Am. Chem. Soc., 2017, 139, 1452 CrossRef CAS PubMed;
(c) M. Epifanov, P. J. Foth, F. Gu, C. Barrillon, S. S. Kanani, C. S. Higman, J. E. Hein and G. M. Sammis, J. Am. Chem. Soc., 2018, 140, 16464 CrossRef CAS PubMed;
(d) W. Y. Fang, G. F. Zha and H. L. Qin, Org. Lett., 2019, 21, 8657 CrossRef CAS PubMed;
(e) Y. Jiang, N. S. Alharbi, B. Sun and H. L. Qin, RSC Adv., 2019, 9, 29784 RSC;
(f) R. Lekkala, R. Lekkala, B. Moku, K. P. Rakesh and H. L. Qin, Org. Chem. Front., 2019, 6, 3490 RSC;
(g) J. Liu, S. M. Wang, N. S. Alharbi and H. L. Qin, Beilstein J. Org. Chem., 2019, 15, 1907 CrossRef CAS PubMed;
(h) S. M. Wang, N. S. Alharbi and H. L. Qin, Synthesis, 2019, 51, 3901 CrossRef CAS;
(i) C. Zhao, G. F. Zha, W. Y. Fang, N. S. Alharbi and H. L. Qin, Tetrahedron, 2019, 75, 4648 CrossRef CAS;
(j) W. Y. Fang, G. F. Zha, C. Zhao and H. L. Qin, Chem. Commun., 2019, 55, 6273 RSC;
(k) J. Leng and H. L. Qin, Org. Biomol. Chem., 2019, 17, 5001 RSC;
(l) R. Lekkala, R. Lekkala, B. Moku, K. P. Rakesh and H. L. Qin, Beilstein J. Org. Chem., 2019, 15, 976 CrossRef CAS PubMed;
(m) Y. Jiang, B. Sun, W. Y. Fang and H. L. Qin, Eur. J. Org. Chem., 2019, 3190 CrossRef CAS;
(n) W. Y. Fang and H. L. Qin, J. Org. Chem., 2019, 84, 5803 CrossRef CAS PubMed;
(o) G. F. Zha, W. Y. Fang, J. Leng and H. L. Qin, Adv. Synth. Catal., 2019, 361, 2262 CrossRef CAS;
(p) X. Zhang, K. P. Rakesh and H. L. Qin, Chem. Commun., 2019, 55, 2845 RSC;
(q) R. Lekkala, R. Lekkala, B. Mokua and H. L. Qin, Org. Chem. Front., 2019, 6, 796 RSC;
(r) G. F. Zha, W. Y. Fang, Y. G. Li, J. Leng, X. Chen and H. L. Qin, J. Am. Chem. Soc., 2018, 140, 17666 CrossRef CAS PubMed;
(s) C. Zhao, W. Y. Fang, K. P. Rakesh and H. L. Qin, Org. Chem. Front., 2018, 5, 1835 RSC.
-
(a) Y. Y. Zhao, G. Y. Mei, H. B. Wang, G. F. Zhang and C. R. Ding, Synlett, 2019, 30, 1484 CrossRef CAS;
(b) G. F. Zhang, Y. Y. Zhao, L. D. Xuan and C. R. Ding, Eur. J. Org. Chem., 2019, 4911 CrossRef CAS;
(c) G. F. Zhang, Y. Y. Zhao and C. R. Ding, Org. Biomol. Chem., 2019, 17, 7684 RSC.
- R. A. Sheldon, Chem. Soc. Rev., 2012, 41, 1437 RSC.
- S. N. M. Boddapati, N. Polam, B. R. Mutchu and H. B. Bollikolla, New J. Chem., 2018, 42, 918 RSC.
- H. Basavaprabhu and V. V. Sureshbabu, Org. Biomol. Chem., 2012, 10, 2528 RSC.
- J. Du, K. Luo and X. L. Zhang, RSC Adv., 2014, 4, 54539 RSC.
- B. Rao and X. M. Zeng, Org. Lett., 2014, 16, 314 CrossRef CAS PubMed.
- Z. Y. Liao, W. H. Yeh, P. Y. Liao, Y. T. Liu, Y. C. Chen, Y. H. Chen, T. H. Hsieh, C. C. Lin, M. H. Lu, Y. S. Chen, M. C. Hsu, T. K. Li and T. C. Chien, Org. Biomol. Chem., 2018, 16, 4482 RSC.
- C. J. Zeng, C. J. Chen, C. W. Chang, H. T. Chen and T. C. Chien, Aust. J. Chem., 2014, 67, 1134 CrossRef CAS.
Footnote |
† Electronic supplementary information (ESI) available. See DOI: 10.1039/d0ra02631j |
|
This journal is © The Royal Society of Chemistry 2020 |
Click here to see how this site uses Cookies. View our privacy policy here.