DOI:
10.1039/D0RA02779K
(Paper)
RSC Adv., 2020,
10, 25435-25445
Biodegradable reduction and pH dual-sensitive polymer micelles based on poly(2-ethyl-2-oxazoline) for efficient delivery of curcumin
Received
26th March 2020
, Accepted 26th June 2020
First published on 6th July 2020
Abstract
A series of disulfide-linked amphiphilic polymers polyoxaline-SS-poly(lactide) (PEtOx-SS-PLA) were prepared and self-assembled into nano-micelles in water. The anticancer drug curcumin (Cur) was selected as a model drug, the entrapment of Cur in PEtOx-SS-PLA micelles was investigated and the intracellular transport and release of Cur-loaded micelles was studied in C6 cells. The preparation of Cur-loaded polymer micelles showed that micelle size decreased after drug loading, favoring cell phagocytosis. MTT experiments showed that PEtOx-SS-PLA 52 micelles have a small IC50 (2.05 μg mL−1). The release behavior of PEtOx-SS-PLA 52 drug-loaded micelles in C6 cells showed that polymer micelle enhanced the intracellular release of Cur, and increased the inhibition effect of cancer cells. In a word, these reduction and pH-dual sensitive, biodegradable, hydrophilic shell-discarding PEtOx-SS-PLA micelles have great potential for future tumour administration.
1. Introduction
Currently, cancer is one of the main causes of death. Over the past decades, one of the traditional treatments for cancer is chemotherapy with a variety of chemicals. However, these anti-cancer drugs (such as doxorubicin, paclitaxel, etc.) have serious side effects on normal human tissues because of their properties such as hydrophobicity.1 In addition, the tumor sites have a high penetration and retention (EPR) effect, which results in smaller size nanoparticles being enriched in cancer cells and exhibiting some passive targeting properties. Therefore, nanocarrier controlled release technology has become the key for hydrophobic anticancer drugs to be used in clinical treatment.2,3 Polymer micelles have unique advantages in terms of drug specificity, tolerance and therapeutic index as well as reducing toxic side effects.4 Compared with traditional drug delivery vehicles, polymer micelles have many potential advantages such as wider drug-loading range, stronger drug-loading ability, longer drug circulation time, lower systemic toxicity and better anti-tumor effect, and thus have been widely used in cancer treatment.5,6 Generally, nano-micelle often obtained by the self-assembly of amphiphilic polymer which comprising hydrophilic segment and hydrophobic segment. The core of the polymer micelles has the ability to solubilise hydrophobic drugs, which depends on the affinity of the drug and the hydrophobic segment of the polymer.7,8
Poly(lactic acid) (PLA), poly(lactic-co-glycolic acid) (PLGA) and poly(ε-caprolactone) (PCL) are common aliphatic polyesters that have been approved by Food and Drug Administration (FDA).9,10 Among them, PLA has good biodegradability and biocompatibility, and some products have been clinically applied.11 PEG is the golden standard for hydrophilic polymers and has been considered as a benchmark for polymers used for medical applications, drug delivery or biomaterials.12,13 However, some drug delivery systems (DDS) based on PEG were reported with limitations, such as limited stability, undesired impurities, high viscosity and accumulation in some organs, leading to the urgent study of new materials to substitute for PEG.14,15 In recent decades, poly(2-ethyl-2-oxazoline) (PEtOx) has attracted more and more attention as a hydrophilic polymer with many advantages,16,17 such as simple synthetic route,18,19 low toxicity,20,21 high water solubility, high stability,22,23 and good biocompatibility,24,25 which has been approved by FDA as a food additive.23 PEtOx also has pH-sensitive structural features that enable targeted drug delivery in tumour cells at specific pH conditions.
In recent years, nanomedicines, especially nanomicelles have received widespread attention in the treatment of cancer.26,27 How to safely, accurately and efficiently deliver anticancer drug to tumour sites is the main direction of current research.28,29 A disadvantage of general drug carriers is that the loaded drug cannot be completely released in time from drug carriers, thus resulting in resistance of drug and low efficiency. It can be improved by introducing environmental responsiveness to these carriers. Such carriers undergo physical or chemical changes under the intricate intracellular stimulation such as temperature, pH, reducing substances, light, and enzymes to achieve controlled release of encapsulating drug.30–34 In 2017, our group prepared reduction-responsive micelles based on the amphiphilic block copolymer poly(2-ethyl-2-oxazoline)-b-poly(ε-caprolactone) containing disulfide bond in the backbone (PEtOx-SS-PCL).35 For the treatment of gliomas, DOX was loaded and the micelle size could be easily adjusted by changing the molecular weight of hydrophobic segment of polymer. The size of DOX-loaded PEtOx-SS-PCL micelles was very small about 88.4 ± 2.7 nm, and DOX could rapidly release from PEtOx-SS-PCL micelles in a reducing/pH intracellular environment. In vivo antitumor experiments showed that, DOX-loaded PEtOx-SS-PCL 43 micelles have higher antitumor activity than other PEtOx-SS-PCL micelles towards mice bearing orthotopic C6-Luci cells. Compared with PCL, PLA had a shorter alkyl chain between the ester groups, thus resulting in faster degradation rate.36 PLA was introduced as hydrophobic segment in our experiment design, we hope it is a useful supplement for the reported drug delivery system of PEtOx-SS-PCL micelles.
Based on these work, to enlarge the application of nanomicelles based on polyoxazolines, we prepared a pH and reduction dual-responsive diblock polymer micelles for encapsulation and triggered intracellular release of the anti-cancer drug curcumin (Cur) (Scheme 1). Cur is a chemotherapeutic drug with multiple properties. It has a very strong analgesic, antioxidant, anti-inflammatory, anti-sepsis, anti-tumour, anti-rheumatoid and anti-atherosclerotic biotherapeutic effect.37,38 At the same time, Cur has little side effects on the human body.39 Compared with other chemotherapy drug, Cur can kill tumour cells more specifically.40 In current work, we synthesized a series of amphiphilic polymers with poly(2-ethyl-2-oxazoline) as a hydrophilic segment and PLA as hydrophobic segment, here hydrophobic segments with different molecular weights (denoted as PEtOx-SS-PLA 17, PEtOx-SS-PLA 34 and PEtOx-SS-PLA 52), disulfide bond was introduced into the backbone (denoted as PEtOx-SS-PLA). After the polymer form micelle by self-assembly, the micelle size, potential and critical micelle concentration (CMC) was measured, and the changes of micelle particle size under acidic conditions and reducing conditions was monitored. The intracellular environment was simulated to study the drug release of Cur-loaded PEtOx-SS-PLA micelles under acidic conditions (pH 5.0) and/or reducing environment. The cytotoxicity of Cur-loaded micelles was studied by MTT assay. The intracellular uptake behaviour of the Cur-loaded micelles was observed by laser confocal microscopy (CLSM).
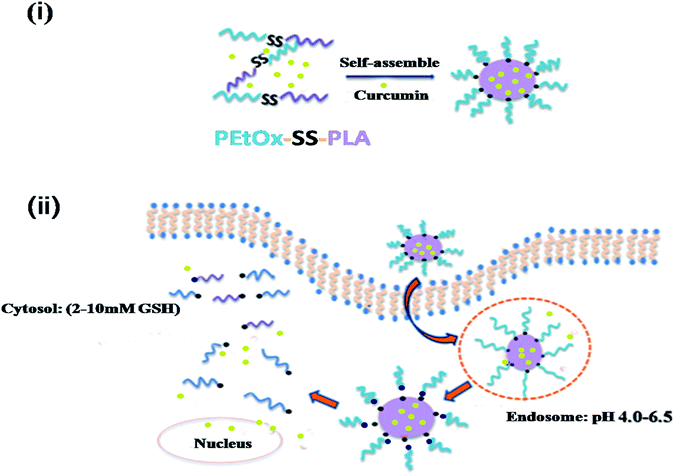 |
| Scheme 1 Schematic illustration for self-assembly and dual-sensitive drug release of Cur-loaded PEtOx-SS-PLA micelles. (i) PEtOx-SS-PLA is self-assembled with Cur into Cur-loaded core–shell micelles in water; (ii) controlled drug release of dual-sensitive Cur-loaded PEtOx-SS-PLA micelles. | |
2. Experimental
2.1. Materials
2-Ethyl-2-oxazoline (EtOx, Alfa, 99%) and dithioethylene glycol (HES, Alfa, 98%) that had been dried over calcium hydride and distilled under reduced pressure. Lactide (LA) had been recrystallized by toluene. Under nitrogen protection, toluene was dried under reflux by adding sodium wire. Chlorobenzene, N,N-dimethylformamide (DMF), acetonitrile, dichloromethane (DCM) were all anhydrous. Curcumin (Cur, 99%) was purchased from Beijing Zhongshuo Pharmaceutical Technology Development Co., Ltd. Methyl p-toluenesulfonate, potassium thiosulphonate (97%), 2,2′-dithiopyridine (98%) were supplied by Sean. Dithiothreitol (DTT, 99%) was bought from Merck. Concentrated hydrochloric acid, glacial acetic acid, potassium hydroxide, ethyl ether, and pyridine were purchased from Sinopharm Chemical Reagent Co., Ltd. and used directly.
Roswell Park Memorial Institute medium (RPMI-1640, Thermo Fisher Scientific Co., Ltd.), fetal bovine serum (FBS, Gibco), 3-(4,5-dimethyl-2-thio-2,5-diphenyl-2H-bromotetrazolium bromide tetrazolium) (MTT, Biosharp). Cell culture flasks were purchased from Corning Costar (Shanghai, China). 4′,6-Diamidino-2-phenylindole (DAPI, Roche). All other chemicals were used directly.
2.2. Characterization
The 1H NMR spectra was tested on a Bruker 400 MHz apparatus using deuterated chloroform (CDCl3) as the solvent. The PL Gel Permeation Chromatography (GPC) 50 instrument equipped with Jordi GPC columns (10 × 104, 2 M) following a differential refractive index detector (PLRI) was used to determine the molecular weight and polydispersity of copolymer. The size and zeta-potential of PEtOx-SS-PLA micelles were measured with a Nano ZS from Malvern Instruments. The fluorescence of Cur was measured by F-4600 FL Spectrophotometer at 298 K. Optical density (OD) values for each well in the assay were measured using a Synergy H4 hybrid multi-well plate reader in MTT experiments. The cellular uptake and intracellular release behaviours of Cur-loaded PEtOx-SS-PLA micelles were observed by fluorescence microscope (Nikon eclipse Ts2R).
2.3. Synthesis of PEtOx-SS-Py and PLA-SH
The synthesis of PEtOx-SS-Py was followed the reference reported by Sun et al. in 2017.6 Yield: 68%. The synthesis method of PLA-SH is similar to the method of PCL-SH reported by our group in 2017, except that PCL was replaced with PLA.35 Yield: 87%.
2.4. Synthesis of PEtOx-SS-PLA
Under protection of nitrogen, PEtOx-SS-Py (0.3 g, 0.06 mmol) was added to DCM (10 mL) and dissolved by stirring at room temperature (r.t.). After dissolution, PLA-SH (0.36 g, 0.05 mmol) was added to the solution, the pH of the system was adjusted to 2.5 using glacial acetic acid, and the reaction system was stirred for 48 h. After completion of the reaction, the mixture was precipitated with ice-ether, and the product was filtered, and washed several times with a small amount of ice-methanol to remove unreacted PEtOx-SS-Py, and dried under vacuum for a whole day to obtain PEtOx-SS-PLA. Yield: 50%.
2.5. Preparation and characterization of micelles
PEtOx-SS-PLA polymer micelles were prepared by dialysis. The specific procedure was as follows: 2 mg of polymer PEtOx-SS-PLA was dissolved in 1 mL of DMSO, and 3 mL of deionized water was slowly added dropwise with stirring at room temperature. After completion of the addition, the solution was loaded into a dialysis bag (SPECTRA/POR, MWCO: 3500) and dialyzed for a whole day with deionized water to obtain a micelle solution. The particle size and distribution of PEtOx-SS-PLA micelles were determined using dynamic light scattering (DLS). The micelles were filtered using a 0.45 μm filter before testing.
The critical micelle solubility (CMC) of the PEtOx-SS-PLA micelles was measured using a fluorescence method taking pyrene as a fluorescent probe. The polymer micelle solutions were prepared with different concentrations, and pyrene solution was added into each sample. The fluorescence intensity of the micelle solution was measured by a fluorescence spectrophotometer (F-4600) using an excitation wavelength of 330 nm and a scanning range of 350–650 nm. The fluorescence values (I1, I3) at the wavelengths of 372 nm and 383 nm were collected, and the ratio of the two fluorescence values (I1/I3) was used as the Y axis and the corresponding concentrations as the X axis. The abscissa of the inflection point of the curve in the figure is the CMC value.
2.6. Degradation of PEtOx-SS-PLA micelles under acidic and reducing conditions
The reduction of PEtOx-SS-PLA 52 micelles and particle size change at pH 5.0 were measured by DLS. First, the oxygen in the PEtOx-SS-PLA 52 micelles (1.5 mL) was removed by nitrogen bubbling. The micelles were respectively putted in sodium acetate buffer (pH 5.0, 10 mM) or PB buffer (pH 7.4, 10 mM). And then 10 mM DTT was added to create a reduced environment. The above mixture was placed in a shaker (200 rpm) at 37 °C. The micelle particle size was measured by DLS at a predetermined time point.
2.7. Encapsulation of curcumin
Curcumin is a commonly used drug-loading model drug. Since it is a fluorescent-sensitive substance, the entire experiment was carried out in the dark. Firstly, tetrahydrofuran was used to prepare a curcumin solution with a concentration of 5 mg mL−1. This solution was added to the polymer PEtOx-SS-PLA solution in DMSO according to the theoretical drug loading (5%, 10% and 20%) and sonicated for 30 min. After the end of the ultrasound, under vigorous stirring (rotation speed greater than 1000 rpm), an appropriate amount of double distilled water was slowly added dropwise to the mixed solution, and the ultrasound was again performed for 1 h after the completion of the addition. The mixed solution was then transferred to a dialysis bag (SPECTRA/POR, MWCO: 3500) for dialysis for 24 h, and the dialyzing medium was 10 mM PB buffer (pH 7.4). The entire experiment was conducted under dark conditions.
To determine the content of curcumin, took a certain amount of drug-loaded micelle solution, freeze-dried for 24 h to obtain a freeze-dried Cur-loaded micelle, and then added 0.5 mL of DMSO and sonicate for 1 h, the purpose of the sonication was to fully dissolve the micelles. And 20 μL of the sample was added to 3 mL of DMSO and shaken evenly. Fluorescence was measured by an F-4600 fluorescence spectrophotometer, and the corresponding drug loading content (DLC) and drug loading efficiency (DLE) were calculated using the standard curve of curcumin.
Calculated as follows:
DLC (wt%) = (weight of Cur loaded in micelles/weight of polymer) × 100% |
DLE (%) = (weight of Cur loaded in micelles/total Cur input) × 100% |
2.8. In vitro release of Cur
The in vitro release of Cur from PEtOx-SS-PLA Cur-loaded micelles was performed at 37 °C with constant shaking (200 rpm). The Cur drug-loaded micelles were placed in a dialysis bag with a molecular weight cut-off of 12
000–14
000 Da and placed in four different media (10 mM PB buffer solution, pH 7.4; PB buffer solution containing 10 mM DTT, pH 7.4; 10 mM acetate buffer solution, pH 5.0 and 10 mM acetate buffer solution containing 10 mM DTT, pH 5.0) at 37 °C. In this experiment, there were four experimental groups, each groups set three parallel samples, and the dual-sensitive PEtOx-SS-PLA 52 Cur-loaded micelles were released in the above four media. First of all, each sample (1 mL Cur-loaded micelle solution) was putted into a dialysis bag (MWCO: 12
000-14
000 Da) and dialyzed against 20 mL of the corresponding medium. At a predetermined time point, 6 mL of the release media outside the dialysis bag was removed and an equal volume of fresh release media was added. The fluorescence of Cur was measured with an F-4600 fluorescence spectrophotometer to determine the concentration of curcumin in the release medium taken at each time point. Each release experiment was performed in parallel three times.
2.9. Biocompatibility experiment
Biocompatibility testing of PEtOx-SS-PLA 17, PEtOx-SS-PLA 34 and PEtOx-SS-PLA 52 micelles was performed using the MTT method. When the monolayer coverage of C6 cells (glioma cells) was 80%, they were digested with an appropriate amount of trypsin and seeded in 96-well plates at a density of 104 cells per well. 100 μL of DMEM/high glucose medium containing 10% fetal bovine serum (FBS), 1% L-glutamic acid, antibiotic penicillin (100 IU mL−1), and streptomycin (100 μg mL−1) were added to each well. Then incubated in a 5% CO2 cell incubator at 37 °C for 24 h. This biocompatibility experiments consisted of 5 experimental groups (micelle concentrations of 1 mg mL−1, 0.8 mg mL−1, 0.6 mg mL−1, 0.3 mg mL−1 and 0.1 mg mL−1, respectively). Normally growing cells without drugs were used as a control group. After C6 cells were incubated for 24 h, the experimental groups were added with the corresponding volume of micelle solution and duplicated in 6 wells. After adding the micelles, the cells were incubated for 24 h. 10 μL of MTT solution was added to each well and the incubation was continued in the incubator for 4 h. The mixed media was removed and 150 μL DMSO solutions were added to each well. The blue-purple precipitate of formazan was fully dissolved by gentle shaking and incubating for 10–15 min. Then, the OD values at 490 nm for each well were measured with a Synergy H4 type multi-functional micro plate reader, and zeroing was performed using a blank medium well as a zero adjustment group. The cell survival rate was calculated as follows:
Cell viability (%) = (OD490 sample/OD490 control) × 100% |
Biocompatibility testing of PEtOx-SS-PLA 52 micelles for a healthy cell line (HA1800) was performed using a similar method.
2.10. Cytotoxicity experiment of Cur-loaded PEtOx-SS-PLA micelles
The cytotoxicity test of Cur-loaded PEtOx-SS-PLA micelles was also performed using the MTT method. The cells used in the experiment were C6 cells. The normal growing cell group was served as the control group and the blank culture group served as the zeroing group. C6 cells were seeded at a cell density of 5000 cells per well in a 96-well plate, and 100 μL of each well was added DMEM/high glucose medium, was incubated in 37 °C cell incubator with 5% CO2 for 24 h. Remove the medium from the 96-well plate, and 80 μL of fresh culture solution was added with 20 μL of Cur-loaded PEtOx-SS-PLA 52 micelles, Cur-loaded PEtOx-PLA 52 and free Cur to the experimental group and make the concentration of Cur in the wells was 0.1, 0.5, 1.0, 5.0, 10, 20 and 40 μg mL−1, respectively. Then incubated the cells in the incubator for another 48 h and the mixed media was removed later. Finally, 10 μL of MTT was added to each well and incubated in the incubator for 4 h. Subsequently, the culture solution was removed and 150 μL of DMSO solution was added to each well, with lightly shaking, the blue-purple precipitate was fully dissolved. The absorbance at 490 nm of each well was measured with a Synergy H4 type multi-functional micro plate reader and blanked with blank media wells as the zero adjustment groups.
2.11. Intracellular release of Cur
The uptake and release behaviour of Cur-loaded PEtOx-SS-PLA 52 polymer micelles in C6 cells were performed on a confocal laser scanning microscope. Cur-loaded PEtOx-PLA polymer micelles and free Cur were used as a control. First, C6 cells were seeded at a concentration of 5 × 104 cells per well in a glass dish and placed in a cell incubator containing at 37 °C with 5% CO2 for 24 h. The medium in the glass dish was aspirated and fresh medium containing Cur-loaded PEtOx-SS-PLA micelles, Cur-loaded PEtOx-PLA micelles and free Cur (the final concentration of Cur was 40 μg mL−1) was added. Then cells were cultured for 2 h and 4 h, respectively. Subsequently, the solution from each well was removed and the cells were washed three times with PBS and fixed in 4% paraformaldehyde solution for 30 min. After paraformaldehyde was aspirated completely, the cells were washed with PBS for three times. Then, DAPI staining solution was added to each well to stain the nuclei and after 15 min, the staining solution was removed, and washed three times with PBS to obtain a CLSM sample.
3. Results and discussion
3.1. Synthesis of polymer PEtOx-SS-PLA
Polymer PEtOx-SS-PLA was obtained through the exchange reaction of poly(2-ethyl-2-oxazoline) (PEtOx-SS-Py) with a thiol-terminated PLA (PLA-SH). The synthesis of PEtOx-SS-PLA is similar with polymer PEtOx-SS-PCL.35 The 1H NMR spectrum of the polymer PEtOx-SS-PLA 52 was shown in Fig. 1. The 1H NMR spectrum showed the integral area ratio of the methyl peak at δ 1.56 on PEtOx to the methyne peak at δ 5.16 on PLA is 3
:
1, which proved that the polymer PEtOx-SS-PLA was successfully synthesized.
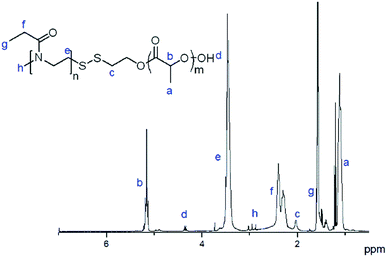 |
| Fig. 1 1H NMR spectra (400 MHz, CDCl3) of PEtOx-SS-PLA 52. | |
The GPC chromatogram of PEtOx-SS-PLA 52 showed a single peak with polydispersity (PDI) of 1.32, confirming the last step of the exchange reaction was successful and the synthesized amphiphilic block polymer PEtOx-SS-PLA 52 was narrowly distributed (Fig. 2). We obtained three polymers with different relative molecular weight by changing the degree of polymerization (DP) of PLA. The molar ratio of lactide to initiator was set at 52
:
1, 34
:
1 and 17
:
1, respectively. The relative molecular weight and PDI of polymer PEtOx-SS-PLA were determined by GPC and listed in Table 1. Polymer PEtOx-PLA 52 was synthesized as the control of polymer PEtOx-SS-PLA 52 by using hydroxyl terminated PEtOx (PEtOx-OH) as initiator to open the ring of LA.
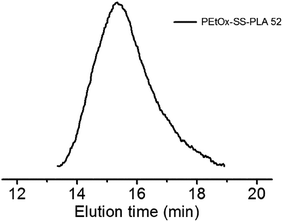 |
| Fig. 2 GPC of PEtOx-SS-PLA 52 (polystyrene standard, eluant: DMF, temperature: 50 °C, RI detection). | |
Table 1 Characteristics of block copolymers PEtOx-SS-PLA and PEtOx-PLA
Block copolymers |
Theoretical Mn |
Mna (1H NMR) |
Mnb (GPC) |
PDIb (GPC) |
Determined by 1H NMR (400 MHz, CDCl3). Determined by GPC (DMF was the eluent, 1.0 mL min−1, 50 °C, polystyrene standards). |
PEtOx-SS-PLA 17 |
7000 |
7500 |
19 600 |
1.18 |
PEtOx-SS-PLA 34 |
10 000 |
10 000 |
28 500 |
1.34 |
PEtOx-SS-PLA 52 |
15 000 |
12 500 |
36 200 |
1.32 |
PEtOx-PLA 52 |
15 000 |
12 500 |
35 200 |
1.19 |
3.2. Preparation and characterization of polymeric PEtOx-SS-PLA micelles
The amphiphilic block polymer PEtOx-SS-PLA can self-assembly into micelles in aqueous solution. PEtOx is a hydrophilic shell and PLA is a hydrophobic core, the core and the shell are connected by a breakable disulfide bond. The responding PEtOx-SS-PLA micelles can be used to encapsulate Cur, a generally used anti-cancer drug. The dynamic light scattering (DLS) was used to determine the particle size of PEtOx-SS-PLA micelles (Fig. 3). It can be seen from Fig. 3 that the micelle size gradually decreased from 216.5 nm to 140.7 nm when DP of PLA increased from 17 to 52. The distribution of micelles was narrow with PDI value in a range of 0.128 to 0.230, indicating that the PEtOx-SS-PLA micelles were uniformly distributed (Table 2). CMC is an important property of micelles, and it is the minimum concentration of micelle formation. From the CMC values of polymer PEtOx-SS-PLA micelles with different DP of PLA, we can see that CMC of the micelles decrease with increased DP of hydrophobic segment, which is due to the increased hydrophobility with the increased DP of PLA, this is also in accordance with drug delivery systems based on block polymer poly(L-lactide)-poly(2-ethyl-2-oxazoline)-poly(L-lactide) (PLLA-PEOz-PLLA) and poly (2-ethyl-2-oxazoline)-b-poly (ε-caprolactone) (PEtOz-SS-PCL).35,40 The CMCs of PEtOx-SS-PLA micelles ranged from 1.20 to 8.28 mg L−1 (Table 2). The smaller CMC value is more conducive for drug-loaded micelles to get longer circulation in the human body fluids.
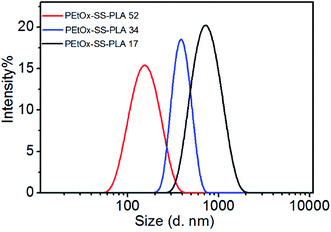 |
| Fig. 3 The size distribution of PEtOx-SS-PLA micelles determined by DLS. | |
Table 2 Characteristics of PEtOx-SS-PLA micelles
Block copolymers |
Micelles sizea (nm) |
PDIa |
Zetaa (mv) |
CMCb (mg L−1) |
Measured by DLS at 25 °C. Measured by fluorescence using pyrene as a hydrophobic probe (pyrene final concentration is 0.6 μM). |
PEtOx-SS-PLA 17 |
216.5 |
0.230 |
−19.6 |
8.28 |
PEtOx-SS-PLA 34 |
196.6 |
0.226 |
−29.5 |
3.70 |
PEtOx-SS-PLA 52 |
140.7 |
0.128 |
−25.4 |
1.20 |
PEtOx-PLA 52 |
130.9 |
0.104 |
−26.9 |
10.93 |
3.3. Degradation of micelles under reducing conditions and acidic conditions
The degradation of polymer micelles is extraordinary important for drug carrier in clinical application. In our work, the degradation of PEtOx-SS-PLA micelles was investigated by DLS measurement at 37 °C. Here PEtOx-SS-PLA 52 micelle with the smallest size was used as an example. The size of PEtOx-SS-PLA 52 micelles was measured by DLS under different conditions: acetate buffer solution (pH 5.0) containing 10 mM DTT and acetate buffer solution (pH 5.0), PB (pH 7.4) containing 10 mM DTT and PB (pH 7.4), respectively. As shown in Fig. 4, the micelles swelled after 2 h at pH 5.0 and then splitted into two peaks after 7 h, which indicated that PEtOx has an acid-sensitive capability and the hydrophilic shell of micelles swelled causing micelle destruction in the acidic environment. With the presence of 10 mM DTT, the average particle sizes of PEtOx-SS-PLA 52 micelles were changed from 140.3 nm to more than 1000 nm after 5 h, which illustrated that the micelles were destroyed and some aggregates appeared (Fig. 5). As the disulfide bond between the hydrophilic shell and the hydrophobic core of the polymer broke under the reduction condition, the hydrophilic shells fell off, causing the micelles become unstable, the micelles swelled and the particle size became larger. It was noteworthy that the micelles rapidly swelled under the condition of pH 5.0 and 10 mM DTT (Fig. 6). After 2 h, the micelles split into two peaks and were destroyed. On the contrary, in the absence of DTT at pH 7.4, the particle size of the micelles hardly changed. The micelle degradation experiments demonstrated that PEtOx-SS-PLA micelles were both pH-sensitive and reduction-responsive.
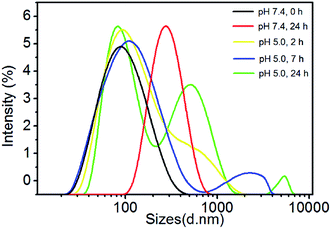 |
| Fig. 4 Change of PEtOx-SS-PLA 52 micelles in response to pH 5.0 at 37 °C. | |
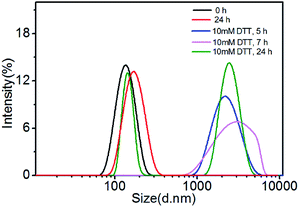 |
| Fig. 5 Change of PEtOx-SS-PLA 52 micelles in response to 10 mM DTT at 37 °C. | |
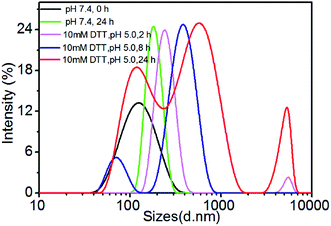 |
| Fig. 6 Change of PEtOx-SS-PLA 52 micelles in response to pH 5.0 and 10 mM DTT at 37 °C. | |
3.4. Loading and in vitro release of Cur
Cur was chosen as a model drug in current work. Dialysis method was used to prepare Cur-loaded micelles of PEtOx-SS-PLA 52 with theoretical drug loadings content of 5%, 10%, and 20%, respectively. The drug loading efficiency of PEtOx-SS-PLA 52 micelles were listed in Table 3. We found that the DLE value of PEtOx-SS-PLA micelles was decreased when their DLC increased. The size of drug loaded micelle was also measured by DLS. It was found that the loading content of Cur increased from 3.40% to 10.66%, the size of PEtOx-SS-PLA 52 micelles sharply decreased from 184.0 nm to 112.0 nm (Table 3). This further demonstrated that there exist the interaction between the drug molecule and the micelle. In addition, the decreased micelle size after drug loading for PEtOx-SS-PLA micelle was beneficial because it was easily phagocytosed by cancer cells, facilitating the transportation of Cur into the cancer cells.
Table 3 DLC and DLE of Cur-loaded PEtOx-SS-PLA micelles
Sample |
Theoretical DLC wt% |
DLCc wt% |
bDLE% |
Micelle sizea nm |
PDI |
Measured by DLS at 25 °C. DLC (%) = (weight of loaded drug/weight of drug in feed) × 100%. DLE was determined by fluorescence measurements. |
PEtOx-SS-PLA 52 |
5 |
3.40 |
68.00 |
184.0 |
0.264 |
10 |
5.88 |
58.80 |
170.5 |
0.225 |
20 |
10.66 |
53.28 |
112.0 |
0.123 |
PEtOx-PLA 52 |
5 |
3.10 |
61.25 |
214.3 |
0.258 |
10 |
5.45 |
54.50 |
164.0 |
0.168 |
20 |
9.99 |
49.90 |
115.8 |
0.124 |
In addition, PEtOx-SS-PLA 52 drug-loaded micelles had excellent redissolving ability. The PEtOx-SS-PLA 52 drug-loaded micelles were dried in a freeze dryer then re-dissolved and the particle size was measured. We found that the particle size distribution was very good, and there was no much change in the particle size.
In vitro release of Cur was also investigated for PEtOx-SS-PLA 52 Cur-loaded micelle. Cur's release curve was shown in Fig. 7. It can be seen that under pH 7.4, the drug-loaded micelles of PEtOx-SS-PLA 52 was stable and released only 39% of loaded drug after 24 h. In the presence of 10 mM DTT, Cur-loaded micelles of PEtOx-SS-PLA 52 released about 52% Cur after 4 h and 92% Cur was released after 24 h. At pH 5.0, the Cur-loaded PEtOx-SS-PLA 52 micelles gave 88% Cur release after 24 h. The fastest drug release was obtained with 56% of Cur was released in 4 h and 92% Cur was released after 12 h at pH 5.0 in the presence of 10 mM DTT. This phenomenon fully demonstrated that the Cur-loaded PEtOx-SS-PLA micelles could rapidly release the loaded Cur under reducing conditions and acidic environment. The results of drug release were consistent with the previous results of degradation of the PEtOx-SS-PLA micelles under reducing condition of acidic condition (pH 5.0) and 10 mM DTT (Fig. 6).
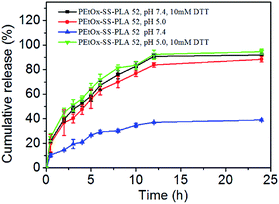 |
| Fig. 7 Cur released from PEtOx-SS-PLA 52 micelles at 37 °C. | |
3.5. Biocompatibility experiment of PEtOx-SS-PLA micelles
In biocompatibility experiment, C6 cells were incubated with different concentrations of PEtOx-SS-PLA micelles for a whole day, and the cell viability was measured by MTT assay. The concentrations of polymer micelles in experimental group were set at 1.0 mg mL−1, 0.8 mg mL−1, 0.6 mg mL−1, 0.3 mg mL−1, and 0.1 mg mL−1, respectively. The results of the cell biocompatibility test showed that even in the highest concentration group (1.0 mg mL−1), the cell survival rate reached more than 90% (Fig. 8). It was demonstrated that PEtOx-SS-PLA micelles did not show inhibition of cell growth. The cell survival rate of other experimental groups was also high, indicating that PEtOx-SS-PLA micelles are not cytotoxic and have good biocompatibility. This is because for polymer PEtOx-SS-PLA, both the hydrophilic segment of polyoxazoline and hydrophobic segment of PLA, have good biocompatibility and have been approved by FDA for using as a drug carrier, tissue engineering and other biomedical fields. In addition, biocompatibility experiment of PEtOx-SS-PLA 52 micelles was also performed on a healthy cell line (HA1800), the cell survival rate was close to or more than 90% (Fig. 9). This experiment was used as a control and the results revealed that PEtOx-SS-PLA 52 micelles have no cytotoxic effect on the healthy cells and are safe drug carrier in future application.
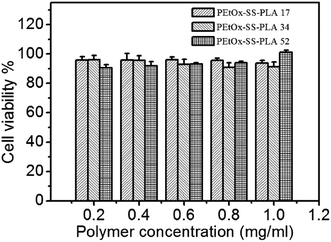 |
| Fig. 8 Cytotoxicity of PEtOx-SS-PLA micelles against C6 cells. The cells were incubated with micelles for 24 h. Data are presented as the average ± standard deviation (n = 6). | |
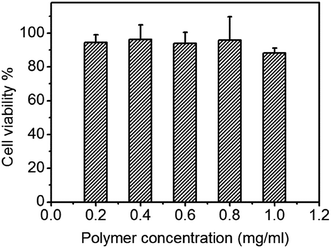 |
| Fig. 9 Cytotoxicity of PEtOx-SS-PLA 52 micelles against HA1800 cells. The cells were incubated with micelles for 24 h. Data are presented as the average ± standard deviation (n = 6). | |
3.6. Inhibitory effect of Cur-loaded PEtOx-SS-PLA micelles on C6 cells
The inhibitory effect of Cur-loaded PEtOx-SS-PLA 52 micelles on C6 cells was also measured by MTT assay. The Cur-loaded PEtOx-PLA 52 micelles and free Cur were used as control. The final concentration of Cur added to the cells in the experimental group was 0.1, 0.5, 1.0, 5.0, 10, 20 and 40 μg mL−1, respectively, and the cells were incubated for 48 h and MTT was used to determine the cell viability. As shown in Fig. 10, the results of experimental groups showed that the cell viability of Cur-loaded PEtOx-SS-PLA 52 micelles was lower than Cur-loaded PEtOx-PLA 52 micelles at the same dosing concentration after 48 h of incubation. The IC50 of Cur-loaded PEtOx-SS-PLA 52 micelles and PEtOx-PLA 52 micelles were 2.05 μg mL−1 and 5.62 μg mL−1, respectively (Table 4). These results indicate that the PEtOx-SS-PLA 52 drug-loaded micelles were advantageous for endocytosis of C6 cells because they had a smaller particle size. In addition, the presence of disulfide bond in polymer PEtOx-SS-PLA 52 micelles increased the release of Cur and further inhibit the growth of C6 cells, resulting in significant inhibition on C6 cells.
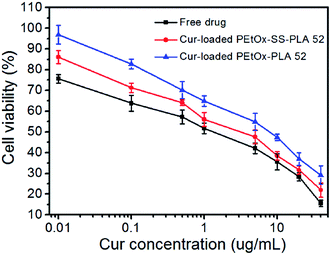 |
| Fig. 10 Anti-tumor activity of Cur-loaded PEtOx-SS-PLA 52 micelles, Cur-loaded PEtOx-PLA 52 micelles and free Cur as a function of Cur dosages. The cells (C6) were incubated with Cur-loaded micelles or free Cur for 48 h. Data are presented as the average ± standard deviation (n = 6). | |
Table 4 The IC50 value of drug-loaded micelles and free drug
Drug loaded copolymers |
IC50 (μg mL−1) |
Free drug |
0.79 |
PEtOx-SS-PLA 52 |
2.05 |
PEtOx-PLA 52 |
5.62 |
3.7. Cellular uptake and intracellular release
The transmission and release behaviour of Cur-loaded PEtOx-SS-PLA 52 micelles in C6 cells was observed by CLSM. In this experiment, there were three groups, Cur-loaded PEtOx-SS-PLA 52 micelles, Cur-loaded PEtOx-PLA 52 micelles and free Cur, with three replicates in each group.
The results were shown in Fig. 11, when Cur-loaded PEtOx-SS-PLA micelles were co-cultured with C6 cells for 2 h, some Cur's yellow fluorescence appeared in the cytoplasm. In contrast, in the control group, the Cur-loaded PEtOx-PLA micelles incubated with the cells for 2 h exhibited only weak Cur yellow fluorescence. This phenomenon indicated that the amount of Cur released by PEtOx-SS-PLA drug-loaded micelles was much higher than PEtOx-PLA drug-loaded micelles. The fluorescence intensity of nuclei and cytoplasm was significantly increased after 4 h incubation of C6 cells with Cur-loaded PEtOx-SS-PLA micelles. As for Cur-loaded PEtOx-PLA, Cur fluorescence was only could be seen in some of the cell nucleus and the fluorescence intensity was weaker than that of the PEtOx-SS-PLA micelles. These experimental results showed that Cur-loaded PEtOx-SS-PLA micelles could release more Cur into the nucleus of C6 cells than Cur-loaded PEtOx-PLA micelles. The PEtOx-SS-PLA micelles are responsive to the acidic and reducing environment within the cell, causing the micelles to disintegrate and thus accelerate the release of Cur within the cell.
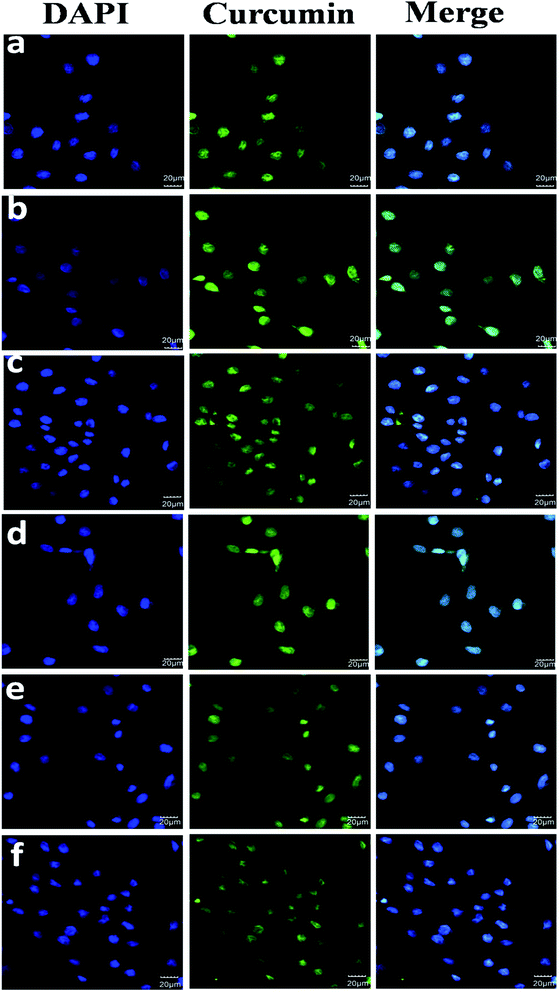 |
| Fig. 11 CLSM images of C6 cells incubated with free Cur, Cur-loaded PEtOx-SS-PLA and PEtOx-PLA micelles (dosage: 25 μg Cur equiv. mL−1). For each panel, the images from left to right show cell nuclei stained by DAPI (blue), Cur fluorescence in cells (green), and overlays of the two images. (a) Free Cur, 2 h incubation; (b) free Cur, 4 h incubation; (c) Cur-loaded PEtOx-SS-PLA micelles, 2 h incubation; (d) Cur-loaded PEtOx-SS-PLA micelles, 4 h incubation; (e) Cur-loaded PEtOx-PLA, 2 h incubation; (f) Cur-loaded PEtOx-PLA, 4 h incubation. | |
4. Conclusions
In this paper, we successfully synthesized three block polymers, PEtOx-SS-PLA, with different lengths of hydrophobic segments of PLA. The micelles were obtained by self-assembly of the polymers PEtOx-SS-PLA with size range from 140.7 nm to 216.5 nm, and narrow distribution in the range of 0.128–0.230. PEtOx-SS-PLA micelles had small CMCs and were stable under normal physiological conditions. At the same time, the micelles had both reduction and acid sensitivity. The particle size became smaller after loading with Cur, ranged from 184.0 nm to 112.0 nm, which made the drug-loaded PEtOx-SS-PLA micelles easily endocytosed and could quickly enter the cells, facilitating the transport and release of encapsulated drugs. In vitro release experiments demonstrated that the pH/reduction dual-sensitive shell-discarded PEtOx-SS-PLA Cur-loaded micelles could rapidly release Cur under reducing conditions and acidic conditions, indicating that PEtOx-SS-PLA micelles had pH/reduction double sensitivity. The results of cell experiments showed that PEtOx-SS-PLA 52 had a better effect on inhibiting the growth of C6 cells due to its smaller particle size. PEtOx-SS-PLA 52 drug-loaded micelles could rapidly abandoned the hydrophilic shell, dissociated the micelles, and quickly released the loaded drug Cur. Therefore, pH/reduction dual sensitive, biodegradable, hydrophilic shell-discarding PEtOx-SS-PLA micelles have great potential for future tumour administration.
Conflicts of interest
There are no conflicts to declare.
Acknowledgements
This work is financially supported by the National Natural Science Foundation of China (No. 51802127 and 81871805), Graduate Scientific Research Innovation Program of Jiangsu Province and Priority Academic Program Development of Jiangsu Higher Education Institutions.
References
- S.-T. Yu, T.-M. Chen, S.-Y. Tseng and Y.-H. Chen, Tryptanthrin inhibits MDR1 and reverses doxorubicin resistance in breast cancer cells, Biochem. Biophys. Res. Commun., 2007, 358(1), 79–84 CrossRef CAS PubMed.
- Y. Yamada and H. Harashima, Mitochondrial drug delivery systems for macromolecule and their therapeutic application to mitochondrial diseases, Adv. Drug Delivery Rev., 2008, 60(13–14), 1439–1462 CrossRef CAS PubMed.
- N. S. Santos-Magalhaes and V. C. Furtado Mosqueira, Nanotechnology applied to the treatment of malaria, Adv. Drug Delivery Rev., 2010, 62(4–5), 560–575 CrossRef CAS PubMed.
- U. Kedar, P. Phutane, S. Shidhaye and V. Kadam, Advances in polymeric micelles for drug delivery and tumor targeting, Nanomed. Nanotechnol. Biol. Med., 2010, 6(6), 714–729 CrossRef CAS PubMed.
- L. Chen, F. Chen, M. Zhao, X. Zhu, C. Ke, J. Yu, Z. Yan, F. Zhang, Y. Sun, D. Chen, C. Jiang, X. Zhao, Y. Gao, S. Guo and W. Li, A Redox-Sensitive Micelle-Like Nanoparticle Self-Assembled from Amphiphilic Adriamycin-Human Serum Albumin Conjugates for Tumor Targeted Therapy, BioMed Res. Int., 2015, 987404 Search PubMed.
- H. Sun, B. Guo, R. Cheng, F. Meng, H. Liu and Z. Zhong, Biodegradable micelles with sheddable poly(ethylene glycol) shells for triggered intracellular release of doxorubicin, Biomaterials, 2009, 30(31), 6358–6366 CrossRef CAS PubMed.
- I. Bala, S. Hariharan and M. Kumar, PLGA nanoparticles in drug delivery: the state of the art, Crit. Rev. Ther. Drug Carrier Syst., 2004, 21(5), 387–422 CrossRef CAS PubMed.
- Y. Wu, M. Li and H. Gao, Polymeric micelle composed of PLA and chitosan as a drug carrier, J. Polym. Res., 2009, 16(1), 11–18 CrossRef CAS.
- R. James, S. G. Kumbar, C. T. Laurencin, G. Balian and A. B. Chhabra, Tendon tissue engineering: adipose-derived stem cell and GDF-5 mediated regeneration using electrospun matrix systems, Biomed. Mater., 2011, 6(2), 025011 CrossRef CAS.
- G. Adamus, P. Rizzarelli, M. S. Montaudo, M. Kowalczuk and G. Montaudo, Matrix-assisted laser desorption/ionization time-of-flight mass spectrometry with size-exclusion chromatographic fractionation for structural characterization of synthetic aliphatic copolyesters, Rapid Commun. Mass Spectrom., 2006, 20(5), 804–814 CrossRef CAS PubMed.
- C. Giovino, I. Ayensu, J. Tetteh and J. S. Boateng, Development and characterisation of chitosan films impregnated with insulin loaded PEG-b-PLA nanoparticles (NPs): a potential approach for buccal delivery of macromolecules, Int. J. Pharm., 2012, 428(1-2), 143–151 CrossRef CAS PubMed.
- T. Tagami, K. Nakamura, T. Shimizu, N. Yamazaki, T. Ishida and H. Kiwada, CpG motifs in pDNA-sequences increase anti-PEG IgM production induced by PEG-coated pDNA-lipoplexes, J. Controlled Release, 2010, 142(2), 160–166 CrossRef CAS PubMed.
- K. Knop, R. Hoogenboom, D. Fischer and U. S. Schubert, Poly(ethylene glycol) in Drug Delivery: Pros and Cons as Well as Potential Alternatives, Angew. Chem. Int. Ed., 2010, 49(36), 6288–6308 CrossRef CAS PubMed.
- G. Pasut and F. M. Veronese, Polymer-drug conjugation, recent achievements and general strategies, Prog. Polym. Sci., 2007, 32(8–9), 933–961 CrossRef CAS.
- H. Koide, T. Asai, K. Hatanaka, S. Akai, T. Ishii, E. Kenjo, T. Ishida, H. Kiwada, H. Tsukada and N. Oku, T cell-independent B cell response is responsible for ABC phenomenon induced by repeated injection of PEGylated liposomes, Int. J. Pharm., 2010, 392(1–2), 218–223 CrossRef CAS PubMed.
- T. Lorson, M. M. Luebtow, E. Wegener, M. S. Haider, S. Borova, D. Nahm, R. Jordan, M. Sokolski-Papkov, A. V. Kabanov and R. Luxenhofer, Poly(2-oxazoline)s based biomaterials: a comprehensive and critical update, Biomaterials, 2018, 178, 204–280 CrossRef CAS PubMed.
- R. Hoogenboom, Poly(2-oxazoline)s: A Polymer Class with Numerous Potential Applications, Angew. Chem. Int. Ed., 2009, 48(43), 7978–7994 CrossRef CAS.
- K.-Y. Peng, S.-W. Wang and R.-S. Lee, Amphiphilic diblock copolymers based on poly(2-ethyl-2-oxazoline) and poly(4-substituted-epsilon-caprolactone): synthesis, characterization, and cellular uptake, J. Polym. Sci., Part A: Polym. Chem., 2013, 51(13), 2769–2781 CrossRef CAS.
- L. Tauhardt, K. Kempe and U. S. Schubert, Toward the design of LPEI containing block copolymers: improved synthesis protocol, selective hydrolysis, and detailed characterization, J. Polym. Sci., Part A: Polym. Chem., 2012, 50(21), 4516–4523 CrossRef CAS.
- R. Luxenhofer, S. Huber, J. Hytry, J. Tong, A. V. Kabanov and R. Jordan, Chiral and Water-Soluble Poly(2-oxazoline)s, J. Polym. Sci., Part A: Polym. Chem., 2013, 51(3), 732–738 CrossRef CAS.
- L. T. T. Trinh, H. M. L. Lambermont-Thijs, U. S. Schubert, R. Hoogenboom and A.-L. Kjoniksen, Thermoresponsive Poly(2-oxazoline) Block Copolymers Exhibiting Two Cloud Points: Complex Multistep Assembly Behavior, Macromolecules, 2012, 45(10), 4337–4345 CrossRef CAS.
- R. Luxenhofer, A. Schulz, C. Roques, S. Li, T. K. Bronich, E. V. Batrakova, R. Jordan and A. V. Kabanov, Doubly amphiphilic poly(2-oxazoline)s as high-capacity delivery systems for hydrophobic drugs, Biomaterials, 2010, 31(18), 4972–4979 CrossRef CAS PubMed.
- X. Wang, X. Li, Y. Li, Y. Zhou, C. Fan, W. Li, S. Ma, Y. Fan, Y. Huang, N. Li and Y. Liu, Synthesis, characterization and biocompatibility of poly(2-ethyl-2-oxazoline)-poly(D,L-lactide)-poly(2-ethyl-2-oxazoline) hydrogels, Acta Biomater., 2011, 7(12), 4149–4159 CrossRef CAS PubMed.
- M. C. Woodle, C. M. Engbers and S. Zalipsky, New Amphipatic Polymer Lipid Conjugates Forming Long-Circulating Reticuloendothelial System-Evading Liposomes, Bioconjugate Chem., 1994, 5(6), 493–496 CrossRef CAS PubMed.
- S. Zalipsky, C. B. Hansen, J. M. Oaks and T. M. Allen, Evaluation of blood clearance rates and biodistribution of poly(2-oxazoline)-grafted liposomes, J. Pharm. Sci., 1996, 85(2), 133–137 CrossRef CAS PubMed.
- H. Cabral, K. Miyata, K. Osada and K. Kataoka, Block Copolymer Micelles in Nanomedicine Applications, Chem. Rev., 2018, 118(14), 6844–6892 CrossRef CAS PubMed.
- G. P. Yu, Q. Ning, Z. C. Mo and S. S. Tang, Intelligent polymeric micelles for multidrug co-delivery and cancer therapy, Artif. Cells, Nanomed., Biotechnol., 2019, 47(1), 1476–1487 CrossRef CAS PubMed.
- S. Krol, R. Macrez, F. Docagne, G. Defer, S. Laurent, M. Rahman, M. J. Hajipour, P. G. Kehoe and M. Mahmoudi, Therapeutic Benefits from Nanoparticles: The Potential Significance of Nanoscience in Diseases with Compromise to the Blood Brain Barrier, Chem. Rev., 2013, 113(3), 1877–1903 CrossRef CAS.
- M. von Mehren, G. P. Adams and L. M. Weiner, Monoclonal antibody therapy for cancer, Annu. Rev. Med., 2003, 54, 343–369 CrossRef CAS.
- A. Kumari, S. K. Yadav and S. C. Yadav, Biodegradable polymeric nanoparticles based drug delivery systems, Colloids Surf. B Biointerfaces, 2010, 75(1), 1–18 CrossRef CAS.
- E. S. Lee, Z. Gao, D. Kim, K. Park, I. C. Kwon and Y. H. Bae, Super pH-sensitive multifunctional polymeric micelle for tumor pH(e) specific TAT exposure and multidrug resistance, J. Controlled Release, 2008, 129(3), 228–236 CrossRef CAS PubMed.
- H. Kim, S.-M. Jeong and J.-W. Park, Electrical Switching
between Vesicles and Micelles via Redox-Responsive Self-Assembly of Amphiphilic Rod-Coils, J. Am. Chem. Soc., 2011, 133(14), 5206–5209 CrossRef CAS PubMed.
- W. Shao, K. Miao, H. Liu, C. Ye, J. Du and Y. Zhao, Acid and reduction dually cleavable amphiphilic comb-like copolymer micelles for controlled drug delivery, Polym. Chem., 2013, 4(11), 3398–3410 RSC.
- S. Cerritelli, D. Velluto and J. A. Hubbell, PEG-SS-PPS: reduction-sensitive disulfide block copolymer vesicles for intracellular drug delivery, Biomacromolecules, 2007, 8(6), 1966–1972 CrossRef CAS PubMed.
- Y. Li, B. Li, L. Bu, Z. Wang, Y. Xie, B. Du, D. Zhu, Y. Zhu, J. Liang, R. Yu and H. Liu, Reduction-responsive PEtOz-SS-PCL micelle with tailored size to overcome blood-brain barrier and enhance doxorubicin antiglioma effect, Drug Delivery, 2017, 24(1), 1782–1790 CrossRef CAS PubMed.
- H. C. Nam and W. H. Park, Aliphatic Polyester-Based Biodegradable Microbeads for Sustainable Cosmetics, ACS Biomater. Sci. Eng., 2020, 6(4), 2440–2449 CrossRef CAS.
- Y. Zhong, W. Yu, H. Feng, Z. Fan and J. Li, Curcumin suppresses tumor necrosis factor-alpha-induced matrix metalloproteinase-2 expression and activity in rat vascular smooth muscle cells via the NF-kappa B pathway, Exp. Ther. Med., 2014, 7(6), 1653–1658 CrossRef CAS PubMed.
- A. Guri, I. Guelseren and M. Corredig, Utilization of solid lipid nanoparticles for enhanced delivery of curcumin in cocultures of HT29-MTX and Caco-2 cells, Food Funct., 2013, 4(9), 1410–1419 RSC.
- Y. Zhang, A. R. Khan, M. Fu, Y. Zhai, J. Ji, L. Bobrovskaya and G. Zhai, Advances in curcumin-loaded nanopreparations: improving bioavailability and overcoming inherent drawbacks, J. Drug Targeting, 2019, 27(9), 917–931 CrossRef CAS PubMed.
- J. Ravindran, S. Prasad and B. B. Aggarwal, Curcumin and Cancer Cells: How Many Ways Can Curry Kill Tumor Cells Selectively?, AAPS J., 2009, 11(3), 495–510 CrossRef CAS PubMed.
Footnote |
† Hena Zhang and Xiaojun Liu contributed equally on this manuscript. |
|
This journal is © The Royal Society of Chemistry 2020 |
Click here to see how this site uses Cookies. View our privacy policy here.