DOI:
10.1039/D0RA02936J
(Paper)
RSC Adv., 2020,
10, 20809-20816
The broad-spectrum and ultra-sensitive detection of zeranol and its analogues by an enzyme-linked immunosorbent assay in cattle origin samples
Received
31st March 2020
, Accepted 11th May 2020
First published on 2nd June 2020
Abstract
Zeranol (α-zearalanol) has been used as a growth promoter in livestock since 1969 in some non-EU countries; the residues of zeranol and its five analogues in animal origin foods may endanger human health due to their strong estrogenic and anabolic activities. Therefore, it is urgent to establish simple, rapid, real-time, broad-spectrum and high-sensitivity detection methods for the residues of zeranol and its analogues. In this study, an ultrasensitive indirect-competition enzyme-linked immunosorbent assay (ic-ELISA) was established for the rapid multi-residue detection of zeranol and its five analogues in cattle origin samples, which was based on a broad-spectrum monoclonal antibody (mAb) that specifically bound to zeranol and its analogues with high sensitivity. The half maximal inhibitory concentration (IC50) values for zeranol, β-zearalanol, zearalanone, α-zearalenol, β-zearalenol, and zearalenone were 0.103, 0.080, 0.161, 0.177, 0.254, and 0.194 ng mL−1, respectively, the recovery rates of cattle origin samples spiked with zeranol ranged from 79.2–104.2%, and the coefficient of variation (CV) values were less than 11.4%. Excellent correlation (R2 = 0.9845) was obtained between the results of HPLC-MS/MS and ic-ELISA. In conclusion, the developed ic-ELISA could be employed as an ultrasensitive and broad-spectrum detection method for monitoring trace ZEN residues in cattle origin foods.
1. Introduction
Zeranol (α-zearalanol, α-ZAL) is a nonsteroidal estrogenic agonist and a mycotoxin first derived from Gibberella zeae in 1962.1 Zeranol is similar in configuration to estradiol (E2) which is the major female sex hormone, thus it possesses estrogenic and anabolic activities. Therefore, zeranol has been approved for use as a growth promoter to increase weight gain and feed conversion in livestock in some non-EU countries since 1969.2,3 However, various studies have demonstrated that zeranol could be classified as an endocrine disrupting chemicals and may pose adverse effects to human health, such as reproductive disorders, developmental problems, nephrotoxicity, hepatotoxicity and a higher risk of breast cancer.4–8 In addition, the homologous analogues of zeranol, including β-zearalanol (β-ZAL), zearalanone (ZAN), α-zearalenol (α-ZEL), β-zearalenol (β-ZEL) and zearalenone (ZEA), are also similar in configuration to E2 and also possess these activities (Fig. 1).9 The in vivo metabolism of the six analogues in fungi, plants and animals could lead to more than one compound residue in related products. Moreover, these compounds are tolerant of heat and milling, and are difficult to inactivate and remove during processing or cooking.10 Thus, zeranol and its analogues, named as ZENs in this study, could be classified as estrogenic food contaminants in the human diet. To protect consumers from these contaminants, most international organizations and countries have set regulations for permissible levels of zeranol in cattle origin foods,11,12 while zeranol is banned for use in livestock and must not be detected in cattle origin foods in the EU13 and China.14 However, it is not enough to just detect one ZEN in one sample at one time: a satisfactory detection method should simultaneously detect all ZENs or the total level of ZENs in one sample at one time with sufficient sensitivity and specificity.
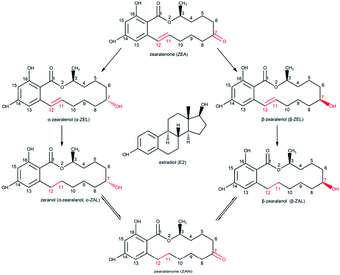 |
| Fig. 1 The chemical structures of ZENs and E2. | |
In recent decades, numerous detection methods for zeranol residues in foods have been established, such as gas chromatography-mass spectrometry (GC-MS),15 liquid chromatography-tandem mass spectrometry (LC-MS/MS),16 high performance liquid chromatography-tandem mass spectrometry (HPLC-MS)17 and ultra-high performance liquid chromatography-tandem mass spectrometry (UHPLC-MS/MS).6 These methods have the advantages of high sensitivity and reliability, but they demand expensive precision instruments, complicated sample preparation, experienced professional operators and highly regimented experimental environments. Recently, immunoassay methods, based on the interaction between antibodies and target specific molecules, have been extensively used, such as the radio immunoassay (RIA),18 chemiluminescence immunoassay (CLIA),19 electrochemical immunosensing (ECIS),20 time-resolved fluorescence immunoassay (TR-FIA),21 and enzyme-linked immunosorbent assay (ELISA).22 Compared with the methods involving precision instruments, immunoassay methods are more cost-efficient and easy-to-use with sufficient sensitivity and specificity, which can satisfy the requirements for rapid detection. Among these methods, ELISA is the most commonly developed immunoassay method for detecting mycotoxins, prohibited additives, and veterinary drugs.
In immunoassays, the antibody is the first critical factor and will directly affect the performance of the developed ELISA method. To detect a class of compounds such as ZENs, the antibody should have broad specificity, similar sensitivity and uniform affinity to all the analytes. Hence, the aim of this study was to prepare a broad-specific monoclonal antibody (mAb) for ZENs with similar sensitivity and uniform affinity, and then develop an ELISA method for multi-residue detection of zeranol and its analogues in cattle origin samples. The developed ELISA should detect the total level of ZENs and have the advantages of simple sample preparation, insusceptibility to matrix effects, and sufficient sensitivity and specificity.
2. Materials and methods
2.1. Chemicals, materials, and apparatus
α-Zearalanol (α-ZAL, zeranol), β-zearalanol (β-ZAL), zearalanone (ZAN), α-zearalenol (α-ZEL), β-zearalenol (β-ZEL), zearalenone (ZEA), aflatoxin B1 (AFB1), deoxynivalenol (DON), fumonisin B1 (FB1), ochratoxin A (OTA), patulin (PAT), T-2 toxin, O-(carboxymethyl) hydroxylamine hemihydrochloride (CMO), 1-(3-(dimethylamino)propyl)-3-ethylcarbodiimide hydrochloride (EDC), N-hydroxysuccinimide (NHS), Freund's complete adjuvant (FCA), Freund's incomplete adjuvant (FIA), a mouse monoclonal antibody isotyping kit and β-glucuronidase/sulfatase were purchased from Sigma-Aldrich (St. Louis, USA). Trenbolone acetate (TBA), melengestrol acetate (MLGA) and progesterone (P4) were purchased from Dr Ehrenstorfer GmbH (Augsburg, Germany). Bovine serum albumin (BSA) and ovalbumin (OVA) were purchased from BDH (VWR International Ltd., USA). RPMI-1640 medium was purchased from Solarbio (Beijing, China). Fetal bovine serum (FBS), cell culture plastics and a goat anti-mouse IgG antibody (whole molecule) were obtained from Thermo Fisher Scientific (Waltham, USA). Ninety-six-well high adsorption ELISA plates were purchased from GSBIO (Wuxi, China). The other reagents and solvents were of analytical grade or higher.
Eight-week-old female BALB/c mice were obtained from the Laboratory Animal Centre (Zhengzhou University, China). Animal immunizations were performed at the Henan Key Laboratory of Animal Immunology (Zhengzhou, China). All animals were raised and treated according to the principles of Institutional Animal Care and Use Committees of the Henan Academy of Agricultural Sciences.
Murine myeloma SP2/0 cells were provided by Dr Howard, Institute for Animal Health, UK, and maintained in RPMI-1640 medium supplemented with 10% (v/v) FBS.
A microplate reader 550 was purchased from Bio-Rad (Richmond, USA). An ultrapure water purification system was purchased from Millipore (Bedford, USA).
2.2. Production of monoclonal antibodies
The synthesis of hapten–protein conjugates was performed according to previous studies with modifications, as described in Fig. 2.23 Five milligrams of ZAN and 10 mg of CMO were dissolved in 5 mL of pyridine, the mixture was stirred at 100 °C for 6 h and dried under vacuum, and the residue was re-dissolved in 5 mL of distilled water (the pH was adjusted to 8 with 2 M sodium bicarbonate buffer). Unmodified ZAN was extracted from the aqueous phase with 5 mL of methylbenzene three times. Then, the aqueous phase was extracted with 5 mL of ethyl acetate three times. Subsequently, the ethyl acetate phase was dried under vacuum, and the residue was the oxime ZAN-7-CMO. ZAN-7-CMO was dissolved in 1 mL of N,N-dimethylformamide and transferred into a 10 mL glass bottle with 5 mg of EDC and 3 mg of NHS. The mixture ZAN-7-CMO/EDC/NHS was stirred gently at 4 °C for 12 h. Next, 13 mg of BSA or 9 mg of OVA was dissolved in 2 mL of 0.01 M phosphate-buffered saline (PBS, pH 7.4) and the mixture ZAN-7-CMO/EDC/NHS was added dropwise under slight stirring. The reaction mixture ZAN-7-CMO/EDC/NHS/protein was stirred gently at 4 °C for 12 h. Finally, the conjugates ZAN–BSA and ZAN–OVA were dialyzed in 0.01 M PBS at 4 °C for 72 h and stored at −20 °C.
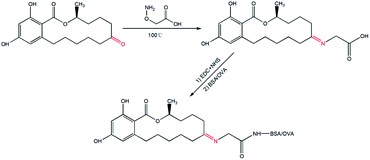 |
| Fig. 2 The synthetic scheme used to produce the hapten–protein conjugates. | |
Each BALB/c mouse was subcutaneously immunized with 50 μg of ZAN–BSA. The first injection was performed with 100 μL of the immunogen which was emulsified with 100 μL of FCA, and the other three booster injections were carried out at intervals of 3 weeks with 100 μL of immunogen emulsified in 100 μL of FIA. Antisera were collected on the 14th day after the fourth immunization to investigate the sensitivity and specificity of the anti-zeranol antibody. The titre of the anti-zeranol antibody and the recognition property of the ZENs were tested by indirect ELISA (i-ELISA) and indirect competitive ELISA (ic-ELISA), respectively. The mouse that could produce antibodies with the highest anti-zeranol activity was selected for cell fusion.24 Ten days later, the cell fusion supernatants were screened by ic-ELISA. Positive hybridoma cells were subcloned to obtain monoclonal cells by limiting dilution and then screened by ic-ELISA. Monoclonal cells with the highest titre and best specificity were used to produce ascitic fluids in BALB/c mice after pretreatment with paraffin, and anti-zeranol mAbs were obtained by purifying the ascites by the ammonium sulfate method.
2.3. Protocol of i-ELISA and ic-ELISA
ELISA plates were added to 100 μL per well of ZAN–OVA in 0.05 M carbonate–bicarbonate buffer (CBS, pH 9.6), and incubated at 37 °C for 2 h; after washing with 0.01 M PBS with tween-20 (0.05%, v/v) (PBST, pH 7.4), the plates were blocked by 270 μL per well of skimmed milk (5%, w/v) in PBST and incubated at 37 °C for 2 h; after washing, the prepared plates were stored at 4 °C.
For i-ELISA, firstly, 100 μL per well of antiserum in 5% skimmed milk was added to the prepared plate, followed by incubation at 37 °C for 15 min; after washing, 100 μL per well of HRP-IgG in 5% skimmed milk (1
:
1000, v/v) was added, followed by incubation at 37 °C for 30 min; then, after washing and drying, 100 μL per well of the TMB substrate solution was added, followed by incubation at 37 °C for 10 min; finally, the reaction was terminated by adding 100 μL per well of 2 M sulfuric acid. The optical densities (OD) were determined at 450 nm by the microplate reader. For ic-ELISA, the detection procedures were similar to the above, except that 100 μL per well of standard solution was added simultaneously in the first step.
2.4. Optimization and establishment of the ic-ELISA method
The optimal ic-ELISA working conditions were determined as the optimal working concentration of the coating antigen with the optimal dilution of mAb. To determine the optimal ic-ELISA conditions, different concentrations of ZAN–OVA (0.1875 to 3 μg mL−1) with a series of dilutions of anti-zeranol mAb (1
:
1000 to 1
:
64
000, v/v) were screened by checkerboard titration.
Under the optimal working conditions, the assay buffer was investigated using ic-ELISA. In this study, a standard solution of zeranol ranging from 0.0098 to 2.5 ng mL−1 was prepared in PBS buffers containing different amounts of methanol, then the standard curve was obtained by importing the measured data into GraphPad Prism software to fit to a four-parameter equation.
The developed ic-ELISA was used to investigate the specificity of anti-zeranol mAb against the analogues of zeranol, other mycotoxins and hormonal growth promoters, including β-ZAL, α-ZEL, β-ZEL, ZAN, ZEA, AFB1, FB1, DON, OTA, PAT, T-2 toxin, TBA, MLGA and P4. The specificity of the anti-zeranol mAb against the analytes was evaluated by the value of the half maximal inhibitory concentration (IC50), which was the concentration that could inhibit the specific molecular recognition between the coating antigen and antibody by half, acquired from a four-parameter logistic fit of the standard points. The values of cross-reactivity (CR) were calculated according to the following equation:
2.5. Preparation of the cattle origin sample
Milk, skim milk powder and bovine muscle were purchased from formal markets. Bovine liver and kidney were collected from controlled slaughterhouses. Bovine urine samples were collected from the cattle farm affiliated to the Henan Academy of Agricultural Sciences. All the samples were confirmed as negative (no ZENs detected) by HPLC.
Milk, skim milk powder and urine. Five microliters of each liquid samples were centrifuged at 12
000 rpm for 20 min at 4 °C to form sediment particulate matter or a lipid layer. 2.5 g of skim milk powder in 5 mL of distilled water was processed similarly to the above. 1 mL of the supernatants or middle layers were taken and de-conjugated by incubation at 37 °C for 3 h with 50 μL of β-glucuronidase/sulfatase. Finally, the samples were diluted with the methanol-PBS assay buffer before analysis with ic-ELISA.
Muscle, liver and kidney. After removing the fat and fascia tissue, bovine muscle, liver and kidney samples were finely minced, then 2 g of the sample and 4 mL of distilled water containing 16 μL of β-glucuronidase/sulfatase were added together into a 50 mL polypropylene centrifuge tube. After incubating at 37 °C for 3 h, 16 mL of methanol was added. After being shaken vigorously for 10 min, the sample mixture was centrifuged at 5000 rpm for 10 min. Subsequently, the supernatant was collected and diluted with 0.1 M PBS before analysis with ic-ELISA.In the livestock breeding process approximately 35% of the absorbed zeranol was excreted via the urinary system, and if animals were fed with ZEN-contaminated feedstuff, about 33% of the ZENs (the sum of ZEA, the metabolites and the respective isomers) could be excreted as above. Therefore, in this study, bovine urine was selected as one sample to evaluate the developed immunoassay, aiming to monitor ZEN contamination.
2.6. Validation of the ic-ELISA method
The ic-ELISA was validated on the basis of several parameters, including the limit of detection (LOD), the limit of quantification (LOQ), accuracy, precision and reliability. The values of LOD and LOQ were determined with 20 blank negative samples which were prepared based on the results of the matrix effects analysis. LOD was the concentration corresponding to the sum of the mean value of 20 blank samples plus three times the standard deviation (SD) and LOQ was the concentration corresponding to the sum of the mean value of 20 blank samples plus ten times the SD.
The accuracy and precision were evaluated by the recovery rate (RV) and the coefficient of variation (CV), respectively. Six blank samples were prepared and spiked with concentrations corresponding to 1, 2 and 5 times the LOQ values in triplicate, and the RVs were calculated according to the following equation:
The reliability was determined from the correlation between the ic-ELISA and HPLC-MS/MS results. Four negative milk samples were spiked with different concentrations of zeranol and then equally divided into two groups. One group was analysed by ic-ELISA, and the other group was analysed by HPLC-MS/MS.
3. Results and discussion
3.1. Antigen selection
Zeranol, as well as the five other ZENs, is a typical hapten; haptens are very small molecules (MW < 1 kDa) that can elicit an immune response and stimulate the production of antibodies only when conjugated to carrier proteins. The structures of ZENs are almost identical, except for differences at positions 7 and 11 on the ring. Suitable carrier proteins and the most preferable synthesis pathways were the most influential factors affecting hapten conjugation, and these factors relate to the production and sensitivity of the antibody. The two most common carrier proteins, BSA and OVA, were employed for hapten conjugation in this study. To select a preferable conjugation method, the functional groups of ZENs and the carrier proteins must be identified.
In preliminary tests of this work, five synthesis pathways were employed which involved two positions on the ring of the ZENs. In the first pathway, position 16 of α-ZAL was modified to produce α-ZAL-16-carboxypropyl ether; then, the ether was coupled with proteins in a mixed anhydride reaction. The second pathway was similar to the first; the position-7 hydroxyl group of α-ZAL was modified by insertion of an alkyl chain, resulting in α-ZAL-7-hemisuccinate, which was then conjugated to proteins by the active ester method. The principles of the third through fifth pathways were similar, position 7 of α-ZAL, ZAN and ZEA was modified by inserting a carboxyl group and then coupled with the carrier proteins BSA and OVA by the active ester method.
According to the results of the preliminary tests, the antiserum of antigen ZAN–BSA (ZAN-7-CMO–BSA, the fourth pathway) had the highest specificity towards zeranol, and the subsequent results shown in Table 2 indicate that the mAb obtained from antigen ZAN–BSA exhibited excellent broad-spectrum specificity for all ZENs.
3.2. Optimization and specificity of the ic-ELISA method
Immunoassay methods mainly rely on the effective combination of an antibody with an antigen, the sensitivity of the mAb is a decisive factor. In summary, a mAb for zeranol and other ZENs with broad specificity and high sensitivity is necessary to meet the requirements for the immunoassay. Fortunately, in this study, we obtained a more sensitive and broad-spectrum anti-zeranol mAb than other recently reported mAbs for ZENs.19,21,22,25 Therefore an ultrasensitive ic-ELISA can be established for the determination of zeranol and its analogues in one sample at one time.
The working concentrations of the coating antigen and mAb are decisive factors concerning the specificity and sensitivity of the ic-ELISA. For IC50, different concentration combinations of the coating antigen and mAb yield different results. In this assay, IC50 values were used to determine the optimal working concentrations of the coating antigen and mAbs by checkerboard titration. As shown in Table 1, the optimal working conditions were determined as 0.25 μg mL−1 of ZAN–OVA and a 1
:
2 × 104 mAb dilution, and remained constant after that.
Table 1 Optimization of ic-ELISA via checkerboard titration
Concentration of coating antigen (μg mL−1) |
3 |
1.5 |
0.75 |
0.375 |
0.25 |
0.1875 |
Dilution of mAb (1 : X) |
2.7 × 104 |
2.5 × 104 |
2.4 × 104 |
2.2 × 104 |
2 × 104 |
1.6 × 104 |
IC50 (ng mL−1) |
0.134 |
0.124 |
0.101 |
0.096 |
0.086 |
0.091 |
Methanol is one of the most commonly used organic solvent for dissolving the standards or extracting and purifying the biological samples. However, the activity of the antibody could be affected by the amount of organic solvents in the sample. In order to minimize or eliminate the nonspecific interferences, the standard solution of zeranol was diluted with buffers containing different amount of methanol in PBS, and then analysed by ic-ELISA. As shown in Fig. 3a and b, with an increase of the methanol content in PBS, the B0 values and the IC50 values of α-ZAL were both increased. It is probably because the methanol might increase the non-specific protein adsorption but decrease the affinity of the anti-zeranol mAb for free zeranol. Specifically, when the methanol content in PBS was lower than 6.7%, the activity of the antibody and the sensitivity of ic-ELISA were not changed. Therefore, in this assay, 5% of methanol was selected.
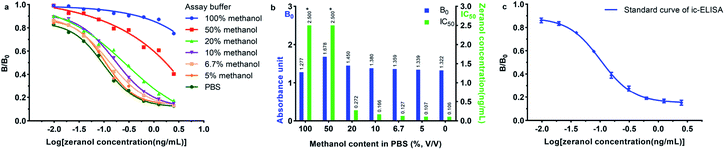 |
| Fig. 3 The influence of the methanol content in PBS (v/v) used as the assay buffer in ic-ELISA against zeranol (a and b) and the standard curve of ic-ELISA for zeranol in 5% methanol (c); * the IC50 value was >2.5 ng mL−1. | |
Under the optimum conditions, 5% of methanol in PBS buffer was used as the solvent of the standard solution and the extraction reagent of the real sample. As shown in Fig. 3c, the standard curve was obtained within 0.0098 to 2.5 ng mL−1, and the B and B0 represented the OD value (450 nm) of zeranol at different concentrations and at 0 ng mL−1. The four-parameter equation was
, where bottom = 0.1509, top = 0.8829, log
IC50 = −0.9885, hill slope = −1.560, and R2 = 0.9970. As shown in Table 2, the IC50 value for zeranol was 0.103 ng mL−1, and those for β-ZAL, ZAN, α-ZEL, β-ZEL and ZEA were 0.080 ng mL−1, 0.161 ng mL−1, 0.177 ng mL−1, 0.254 ng mL−1 and 0.194 ng mL−1, respectively, with the CRs ranging from 40.6% to 128.8%. The results indicated that the mAb obtained in this work was very effectively inhibited by zeranol, ZEA and the analogues, without any cross reactions (the CRs were all less than 0.1%) with other mycotoxins or other growth promoters.
Table 2 Cross-reactivity of mAb with ZENs, other mycotoxins and growth promoters
ZENs |
IC50 (ng mL−1) |
CR (%) |
Other mycotoxins |
IC50 (ng mL−1) |
CR (%) |
Other growth promoters |
IC50 (ng mL−1) |
CR (%) |
α-ZAL |
0.103 |
100.0 |
AFB1 |
>1000 |
<0.1 |
TBA |
>1000 |
<0.1 |
β-ZAL |
0.080 |
128.8 |
DON |
>1000 |
<0.1 |
MLGA |
>1000 |
<0.1 |
ZAN |
0.161 |
64.0 |
FB1 |
>1000 |
<0.1 |
P4 |
>1000 |
<0.1 |
α-ZEL |
0.177 |
58.2 |
OTA |
>1000 |
<0.1 |
|
|
|
β-ZEL |
0.254 |
40.6 |
PAT |
>1000 |
<0.1 |
|
|
|
ZEA |
0.194 |
53.1 |
T-2 |
>1000 |
<0.1 |
|
|
|
3.3. Matrix effects
The matrix effect is one of the challenges in immunoassay methods, which can cause an inaccurate result or even a false-positive result. In some studies, sample pre-treatment procedures such as solid phase extraction and liquid–liquid extraction were often tedious and time consuming. However, for immunoassay methods, the preparation procedures of samples should be simplified, and a dilution method is more suitable. To evaluate the matrix effects, the standard curves obtained from the prepared samples were compared with the curve generated from PBS, as shown in Fig. 4 and 5. As shown in Fig. 4 and 5, all the B/B0 values and IC50 values in milk, skim milk powder, urine and liver samples were larger than that in PBS. The results indicated that the matrix effect existed in all cattle origin samples for this study, but it could be eliminated through the above sample pre-treatment procedures. As shown in Fig. 4a and 5a, take the standard curves and IC50 values in the milk sample as an example, the results indicated that after diluting 6-fold or more, there were no statistically significant differences in the IC50 values between the milk sample and PBS. For the milk powder sample and urine sample, they needed to be diluted 4-fold and 8-fold respectively (Fig. 4b, c and 5a). For the liver extract sample, after diluting 20-fold, the matrix effect could be removed, to ensure uniform experimental conditions, 4% methanol (v/v) was added to PBS (Fig. 4d and 5b). Meanwhile, similar results were obtained for muscle samples and kidney samples. Thus, the ic-ELISA method can be used for the semiquantitative or quantitative detection of ZENs in milk, skim milk powder and urine after diluting the samples. As for the edible bovine tissues, such as muscle, liver and kidney, the pre-treatment procedures were similar to the above with some slight modifications. Firstly, the samples were extracted by methanol. Then, the extracted examples were analysed by the ic-ELISA method at 20-fold dilution. In practical applications, to ensure the concentrations of analytes are within the detection range by ic-ELISA, the sample dilution factor was required to be >1.
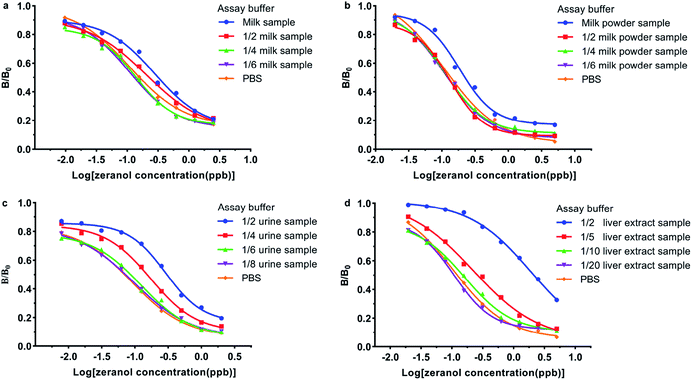 |
| Fig. 4 The evaluation and elimination of the matrix effects in a (a) milk sample, (b) skim milk powder sample, (c) urine sample, and (d) liver extract sample. | |
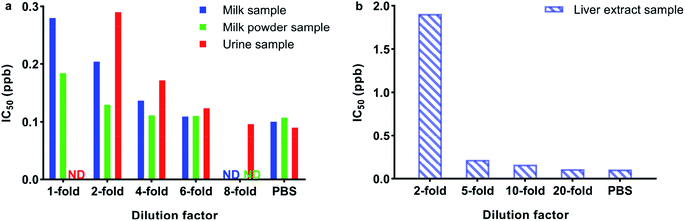 |
| Fig. 5 IC50 values for (a) milk, skim milk powder, urine and (b) liver following different dilutions with PBS; ND: not detected. | |
3.4. Validation of the ic-ELISA method
Zeranol was selected as the analyte to validate the ic-ELISA method. As shown in Table 3, the LOD values and LOQ values for zeranol in the cattle origin samples, including milk, skim milk powder, bovine urine, bovine liver, bovine muscle and bovine kidney, ranged from 0.016–0.027 ppb and 0.027–0.066 ppb, respectively. The RVs for the spiked samples were 79.2–104.2%, and the CVs were less than 11.4%. In addition, there was a good correlation (R2 = 0.9845) between the ic-ELISA and HPLC-MS/MS results (Fig. 6), indicating that the proposed ic-ELISA was reliable for real applications. These results demonstrated that the developed ic-ELISA could meet the requirements of real-time monitoring zeranol and its analogues with broad specificity and high sensitivity in cattle origin samples.
Table 3 LODs, LOQs, RVs, and CVs for zeranol in cattle origin samples
Sample |
LOD (ppb) |
LOQ (ppb) |
Spiked (ppb) |
Mean RV (%) |
CV (%) |
Milk |
0.022 |
0.043 |
0.043 |
87.5 |
5.7 |
0.086 |
92.6 |
6.8 |
0.215 |
94.3 |
7.1 |
Skim milk powder |
0.016 |
0.034 |
0.034 |
85.1 |
4.5 |
0.068 |
95.2 |
4.7 |
0.171 |
89.0 |
9.3 |
Urine |
0.017 |
0.027 |
0.024 |
80.2 |
7.2 |
0.054 |
90.3 |
1.8 |
0.137 |
104.2 |
8.6 |
Liver |
0.023 |
0.052 |
0.052 |
88.8 |
6.7 |
0.104 |
85.4 |
10.1 |
0.261 |
79.2 |
10.5 |
Muscle |
0.026 |
0.062 |
0.062 |
84.2 |
7.6 |
0.124 |
86.5 |
9.7 |
0.310 |
87.2 |
10.2 |
Kidney |
0.027 |
0.066 |
0.066 |
84.6 |
8.9 |
0.131 |
87.6 |
11.4 |
0.328 |
90.7 |
7.9 |
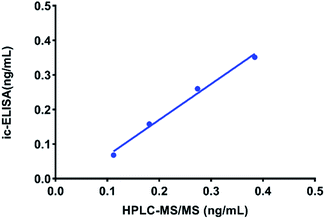 |
| Fig. 6 Correlation between the analysis of zeranol in milk via ic-ELISA and HPLC-MS/MS methods. | |
3.5. Evaluation of ELISA and other immunoassay methods
In recent years, ELISA is the most emphatically developed and gradually commercialized immunoassay method for rapid detection of various analytes. There are indeed some developed methods for detecting zeranol, but none of them meet the requirements of detecting all ZENs with broad specificity, similar sensitivity and uniform affinity. Jiang et al.25 developed a micro-plate CLIEA that could just analyse zeranol and ZEA in bovine tissues, and both the methods developed by Yuan et al.22 and Zhu et al.19 have similar limitations. Even the commercialized ELISA kit did not perform as hoped, the Randox Food Diagnostics Zeranol ELISA test kit (ZR2421) could detect just half of six ZENs at one time and the sensitivity was lower than that of our developed ELISA method. Compared with these studies, the developed mAb in this study shows higher sensitivity and better cross-reactivity values, which successfully meet the requirements of broad-spectrum detection and more accurate results for detecting total levels of ZENs in one sample at one time.
4. Conclusions
In summary, we prepared a broad-spectrum mAb against zeranol and its five analogues, and then developed an ic-ELISA method for the determination of zeranol and its analogues in cattle origin samples. After simple sample preparation, the ic-ELISA method could be used for the semiquantitative and quantitative detection of ZENs in bovine muscle, liver, kidney, urine, milk, and skim milk powder. The obtained detection limits were much lower than the permissible levels of zeranol and other ZENs, and excellent correlation (R2 = 0.9845) was achieved between ic-ELISA and HPLC-MS/MS, which indicated that ic-ELISA could reliably detect ZEN residues in various bovine samples. Therefore, the as-prepared method has been successfully applied in real samples and shows great potential to be used in the field of food safety.
Ethical statement
All animals were raised and treated according to the principles of the Institutional Animal Care and Use Committees of the Henan Academy of Agricultural Sciences and approved by the Animal Ethics Committee of Henan Academy of Agricultural Sciences (approval number SYXK 2014-0007).
Conflicts of interest
There are no conflicts to declare.
Acknowledgements
This work was supported by the China Postdoctoral Science Foundation Funded Project (2018M632778) and the Scientific and Technological Research Project of Henan Province (192102310513).
References
- M. Stob, R. S. Baldwin, J. Tuite, F. N. Andrews and K. G. Gillette, Nature, 1962, 196, 1318 CrossRef CAS PubMed.
- T. W. Perry, M. Stob, D. A. Huber and R. C. Peterson, J. Anim. Sci., 1970, 31, 789–793 CrossRef CAS.
- G. D. Sharp and I. A. Dyer, J. Anim. Sci., 1971, 33, 865–871 CrossRef CAS.
- J. R. Roy, S. Chakraborty and T. R. Chakraborty, Med. Sci. Monit., 2009, 15, 137–145 Search PubMed.
- M. Metzler, E. Pfeiffer and A. A. Hildebrand, World Mycotoxin J., 2010, 3, 385–401 CrossRef CAS.
- H. Belhassen, I. Jiménez-Díaz, J. P. Arrebola, R. Ghali, H. Ghorbel, N. Olea and A. Hedili, Chemosphere, 2015, 128, 1–6 CrossRef CAS PubMed.
- K. Tachampa, M. Takeda, S. Khamdang, R. Noshiro-Kofuji, M. Tsuda, S. Jariyawat, T. Fukutomi, S. Sophasan, N. Anzai and H. Endou, J. Pharmacol. Sci., 2008, 106, 435–443 CrossRef CAS PubMed.
- S. Liu and Y. C. Lin, Breast J., 2004, 10, 514–521 CrossRef CAS PubMed.
- W. M. Haschek and K. A. Voss, in Haschek and Rousseaux's Handbook of Toxicologic Pathology, 3rd edn, 2013, pp. 1187–1258 Search PubMed.
- B. Kabak, J. Sci. Food Agric., 2009, 89, 549–554 CrossRef CAS.
- Australian Pesticides and Veterinary Medicines Authority, Agricultural and Veterinary Chemicals Code (MRL Standard) Instrument 2019, 2019 Search PubMed.
- Government of Canada, List of Maximum Residue Limits (MRLs) for Veterinary Drugs in Foods, 2018 Search PubMed.
- European Commission, Off. J. Eur. Communities, 1996, 125, 3–9 Search PubMed.
- Ministry of Agriculture of the People's Republic of China, Announcement No. 235, 2002 of the Ministry of Agriculture of the People's Republic of China regarding to the maximum residue limits of veterinary drugs in animal foods, 2002 Search PubMed.
- L. C. Dickson, R. Costain, D. McKenzie, A. C. E. Fesser and J. D. MacNeil, J. Agric. Food Chem., 2009, 57, 6536–6542 CrossRef CAS PubMed.
- X. Sun, Q. Tang, X. Du, C. Xi, B. Tang, G. Wang and H. Zhao, Food Anal. Methods, 2017, 10, 3239–3246 CrossRef.
- B. Socas-Rodríguez, J. González-Sálamo, J. Hernández-Borges and M. Á. R. Delgado, Electrophoresis, 2016, 37, 1359–1366 CrossRef PubMed.
- S. N. Dixon, J. Vet. Pharmacol. Ther., 1980, 3, 177–181 CrossRef CAS.
- J. Zhu, X. Tao, S. Ding, J. Shen, Z. Wang, Y. Wang, F. Xu, X. Wu, T. Hu, A. Zhu and H. Jiang, Anal. Lett., 2012, 45, 2538–2548 CrossRef CAS.
- M. Regiart, M. A. Seia, G. A. Messina, F. A. Bertolino and J. Raba, Microchim. Acta, 2015, 182, 531–538 CrossRef CAS.
- M. Tuomola, K. M. Cooper, S. Lahdenperä, G. A. Baxter, C. T. Elliott, D. G. Kennedy and T. Lövgren, Analyst, 2002, 127, 83–86 RSC.
- Y. Liu, C. Zhang, X. Yu, Z. Zhang, X. Zhang, R. Liu, X. Liu and Z. Gong, J. Zhejiang Univ., Sci., B, 2007, 8, 900–905 CrossRef CAS PubMed.
- D. Thouvenot and R. F. Morfin, Appl. Environ. Microbiol., 1983, 45, 16–23 CrossRef CAS PubMed.
- G. Köhler and C. Milstein, Nature, 1975, 256, 495–497 CrossRef PubMed.
- H. Jiang, W. Wang, J. Zhu, X. Tao, J. Li, X. Xia, K. Wen, F. Xu, Z. Wang, M. Chen, X. Li, X. Wu, S. Wang and S. Ding, Luminescence, 2014, 29, 393–400 CrossRef CAS PubMed.
|
This journal is © The Royal Society of Chemistry 2020 |