DOI:
10.1039/D0RA02990D
(Paper)
RSC Adv., 2020,
10, 19600-19609
A novel substrate directed multicomponent reaction for the syntheses of tetrahydro-spiro[pyrazolo[4,3-f]quinoline]-8,5′-pyrimidines and tetrahydro-pyrazolo[4,3-f]pyrimido[4,5-b]quinolines via selective multiple C–C bond formation under metal-free conditions†
Received
2nd April 2020
, Accepted 8th May 2020
First published on 22nd May 2020
Introduction
In the present scenario, the exploration of novel nitrogen-containing spiro compounds is a demanding and challenging task in synthetic organic chemistry. Among the numerous methods available in the literature for the synthesis of nitrogen-bearing derivatives, multicomponent reactions (MCRs) are the most advantageous as they offer high atom economy, and provide access to highly complex molecules through multiple bond formation efficiencies.1–10 Generally, these techniques avoid the purification and isolation of the intermediates, which minimizes solvent waste and enhances the eco-compatibility of the protocol. Spiro compounds are well-known due to their inherent three-dimensional structure in which the fusion point of the bicyclic system shares a single atom. Moreover, these are an interesting family of nitrogen-containing heterocycles due to their distinct pharmacological applications like anti-cancer,11,12 anti-HIV,13 antifungal,14,15 antibacterial,16 antidiabetic,17 and cytotoxic properties.18
A literature survey reveals pioneering work for the synthesis of isatin based pyrazole-fused spiro compounds.19–21 On the other hand the present work focuses on the synthesis of pyrazole-fused spiro compounds excluding isatin as a basic constituent (Scheme 1). The double bond functionalization at carbon-5 of barbituric acid facilitates the formation of spiro compounds like rigid architectures. Some of them are useful in the development of nucleotide mimics, an inhibitor of matrix metalloproteinases (MMP),22 and as anticancer agents23 (Fig. 1).
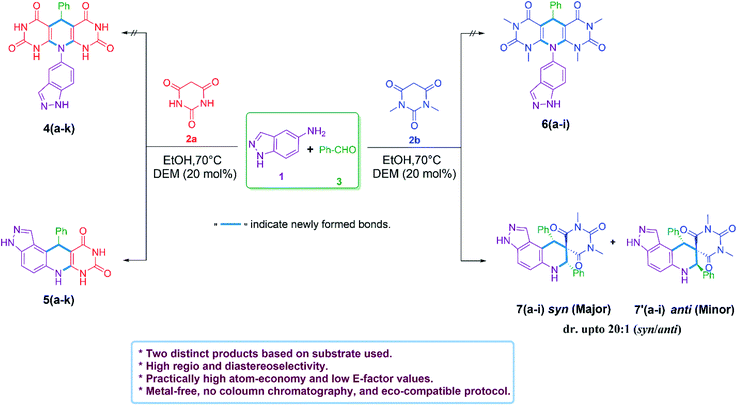 |
| Scheme 1 A substrate oriented multicomponent reaction for the syntheses of tetrahydro-spiro[pyrazolo[4,3-f]quinoline]-8,5′-pyrimidines and tetrahydro-pyrazolo[4,3-f]pyrimido[4,5-b]quinolines. | |
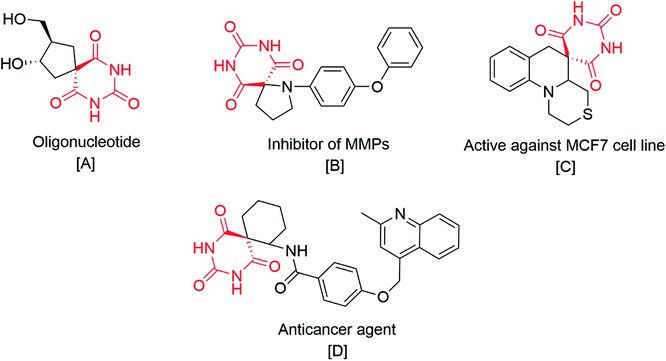 |
| Fig. 1 Barbituric acid based bioactive spiro compounds. | |
The ease of operational procedure and better reaction efficiency make the MCRs more effective over conventional multi-step synthesis thus, in present days more attention has been made on the design of new MCRs for the construction of diverse heterocyclic scaffolds.24–30 In earlier decades much attention was paid to ionic liquids (ILs) as green solvent and catalyst system. However, ILs based on imidazolium cations and fluorinated anions have cons like non-biodegradability, toxicity, less cost-effectiveness, and poor eco-compatibility.31,32 The inclusion of natural deep eutectic mixtures (NADEMs) in organic synthesis has made a great contribution in green and sustainable developments.33–36 Significantly, NADEMs are advantageous due to their cost-effectiveness, less toxicity, biodegradability, and large scope of production by choosing appropriate hydrogen bond donor and acceptor systems.37 In continuation of our research interest for the development of novel nitrogen-containing heterocyclic scaffolds38–42 here, we wish to report a substrate directed novel MCR of 5-aminoindazole (1), barbituric acids (2a or 2b), and aldehyde 3(a–k) or 3(a–i) using betaine-based DEMs in ethanol. Significantly, the MCR of 5-aminoindazole (1), barbituric acid (2a) and aldehyde 3(a–k) selectively produce 11-aryl-3,6,7,11-tetrahydro-8H-pyrazolo[4,3-f]pyrimido[4,5-b]quinoline-8,10(9H)-dione derivatives 5(a–k) instead of 5-aryl-5,10-dihydropyrido[2,3-d:6,5-d′]dipyrimidine-2,4,6,8(1H,3H,7H,9H)-tetraone type derivatives 4(a–k).43 Another interesting finding is the synthesis of high diastereoselective and novel tetrahydro-1′H-spiro[pyrazolo[4,3-f]quinoline-8,5′-pyrimidine]-2′,4′,6′(3′H)-triones 7(a–i) (d.r. up to 20
:
1 (syn
:
anti)) when the same reaction performed by replacing barbituric acid with N,N-dimethyl barbituric acid (Scheme 1).
Results and discussion
Our preliminary investigation was started with the multicomponent reaction of 5-aminoindazole (1), barbituric acid (2a), and benzaldehyde (3a) in equimolar proportion under different catalytic conditions. We made first attempt to carry out title MCR using water as a solvent, unfortunately in this experiment sticky reaction mass formation is observed. To overcome this, we replace water by ethanol as solvent and performed several reaction optimizations experiments, found the ideal reaction temperature and catalyst for the successful transformation. The product isolation and characterizations showed the formation of tetrahydro-8H-pyrazolo[4,3-f]pyrimido[4,5-b]quinoline (5a) instead of expected 10-(1H-indazol-5-yl)-5-aryl-5,10-dihydropyrido[2,3-d:6,5-d′]dipyrimidine-2,4,6,8(1H,3H,7H,9H)-tetraone (4a). The basic skeleton was further confirmed by X-ray single-crystal analysis of compound (5g) (see Table 2). To explore the substrate scope, we performed multicomponent reaction of 5-aminoindazole (1), N,N-dimethyl barbituric acid (2b), and benzaldehyde (3a). This time isolated product was tetrahydro-2′H-spiro[pyrazolo[4,3-f]quinoline-8,5′-pyrimidine]-2′,4′,6′(3′H)-triones (7a) instead of 7,9-dimethyl-11-phenyl-3,6,7,11-tetrahydro-8H-pyrazolo[4,3-f]pyrimido[4,5-b]quinoline-8,10(9H)-dione (5a). The same was braced by X-ray single-crystal analysis of representative compounds (7b and 7h) (see Table 3). As our investigation commenced with sustainable development, we performed the title multicomponent reaction under catalyst-free reaction condition. This require prolong heating for the successful reaction transformation and comparative reaction yields for compounds 5a and 7a observed. To make the rapid reaction transformation, we further tested our model reaction using L-proline (Table 1, entry-4), acetic acid (Table 1, entry-5), formic acid (Table 1, entry-6), p-TSA (Table 1, entry-8), and newly developed betaine based DEMs (Table 1, entries: 9–12) as catalysts. We also check the feasibility of the model reaction under basic condition (Table 1, entry-7). The obtained results are summarized in Table 1. Reaction optimization studies revealed that title multicomponent reaction proceeds efficiently using acid based deep eutectic mixture to produce 11-phenyl-3,6,7,11-tetrahydro-8H-pyrazolo[4,3-f]pyrimido[4,5-b]quinoline-8,10(9H)-dione 5a and 7,9-diphenyl-6,7,9,9a-tetrahydro-2′H-spiro[pyrazolo[4,3-f]quinoline-8,5′-pyrimidine]-2′,4′,6′(1′H,3′H)-trione 7a (see Table 1).
Table 1 Optimization of the reaction conditions
Entry |
Catalyst |
Solvent |
Relative molar ratio of 1, 2a/2b, and 3a |
Temp. (°C) |
Time (min) for 5a/7a |
Yield of 5aa (%) |
Yield of 7ab (%) |
E-Factor (without catalyst) |
E-Factor (including catalyst) |
(1 : 2a : 3a) |
(1 : 2b : 3a) |
5a |
7a |
5a |
7a |
Reaction conditions: 1 mmol of 1, 1 mmol of 2a, 1 mmol of 3a and respective amount of catalyst. Reaction conditions: 1 mmol of 1, 1 mmol of 2b, 2 mmol of 3a and respective amount of catalyst. |
1 |
— |
Water |
(1 : 1 : 1) |
(1 : 1 : 2) |
26–28 |
120 |
NR |
NR |
— |
— |
— |
— |
2 |
— |
EtOH |
(1 : 1 : 1) |
(1 : 1 : 2) |
26–28 |
120 |
NR |
NR |
— |
— |
— |
— |
3 |
— |
EtOH |
(1 : 1 : 1) |
(1 : 1 : 2) |
70 |
180 |
58 |
60 |
0.46 |
0.42 |
0.46 |
0.42 |
4 |
L-Proline (20 mol%) |
EtOH |
(1 : 1 : 1) |
(1 : 1 : 2) |
Reflux |
150 |
62 |
70 |
0.74 |
0.56 |
0.85 |
0.63 |
5 |
Acetic acid (20 mol%) |
EtOH |
(1 : 1 : 1) |
(1 : 1 : 2) |
70 |
100 |
60 |
65 |
0.93 |
0.67 |
0.99 |
0.71 |
6 |
Formic acid (20 mol%) |
EtOH |
(1 : 1 : 1) |
(1 : 1 : 2) |
70 |
100 |
56 |
63 |
1.03 |
0.73 |
1.08 |
0.75 |
7 |
Et3N (20 mol%) |
EtOH |
(1 : 1 : 1) |
(1 : 1 : 2) |
Reflux |
150 |
59 |
60 |
0.93 |
0.86 |
1.03 |
0.92 |
8 |
p-TSA (20 mol%) |
EtOH |
(1 : 1 : 1) |
(1 : 1 : 2) |
70 |
100 |
63 |
67 |
0.83 |
0.61 |
1.0 |
0.72 |
9 |
Betaine-oxalic acid (B1) (20 mol%) |
EtOH |
(1 : 1 : 1) |
(1 : 1 : 2) |
70 |
90 |
80 |
86 |
0.41 |
0.25 |
0.56 |
0.35 |
10 |
Betaine-succinic acid (B2) (20 mol%) |
EtOH |
(1 : 1 : 1) |
(1 : 1 : 2) |
70 |
90 |
75 |
77 |
0.52 |
0.43 |
0.72 |
0.56 |
11 |
Betaine-citric acid (B3) (20 mol%) |
EtOH |
(1 : 1 : 1) |
(1 : 1 : 2) |
70 |
90 |
64 |
68 |
0.74 |
0.61 |
1.03 |
0.81 |
12 |
Betaine-tartaric acid (B4) (20 mol%) |
EtOH |
(1 : 1 : 1) |
(1 : 1 : 2) |
70 |
90 |
60 |
66 |
0.93 |
0.67 |
1.2 |
0.85 |
As we established the reaction conditions, subsequently explored the substrate scope for the 11-aryl-3,6,7,11-tetrahydro-8H-pyrazolo[4,3-f]pyrimido[4,5-b]quinoline-8,10(9H)-dione 5(a–k) and 7,9-diaryl-6,7,9,9a-tetrahydro-2′H-spiro[pyrazolo[4,3-f]quinoline-8,5′-pyrimidine]-2′,4′,6′(1′H,3′H)-triones 7(a–i). The results achieved under optimized reaction conditions are summarized in Tables 2 and 3. This protocol endures both electron releasing as well as electron withdrawing functionalities present in the aldehyde and provide the targeted derivatives within stipulated time. To our delight all synthesized 7,9-diaryl-6,7,9,9a-tetrahydro-2′H-spiro[pyrazolo[4,3-f]quinoline-8,5′-pyrimidine]-2′,4′,6′(1′H,3′H)-triones 7(a–i) were showed high diastereoselectivity (d.r. up to 20
:
1 (syn
:
anti)) (Table 3).
Table 2 Substrate scope for tetrahydro-8H-pyrazolo[4,3-f]pyrimido[4,5-b]quinoline-8,10(9H)-dione 5(a–k)a,b,c
Table 3 Substrate scope for tetrahydro-2′H-spiro[pyrazolo[4,3-f]quinoline-8,5′-pyrimidine]-2′,4′,6′(3′H)-triones 7(a–i)a,b,c,d
Green chemistry metric evaluation
To validate the present work from sustainability concern, here we evaluated it using green metric toolkit like E-factor, Atom Economy (AE), Reaction Mass Efficiency (RME), Optimum Efficiency (OE), and Atom Efficiency (AEf).44–51 The calculated value of E-factor ranging from 0.24 to 0.6 for 5(a–k) series of compounds and that for 7(a–i) series of compounds ranging from 0.17 to 0.56. This highlight the product formation with minimum wastes and show eco-compatibility of the present protocol. The excellent values for Atom Economy (AE) (up to 91.92% and 96.67% for 5(a–k) and 7(a–i) series of compounds respectively) shows how protocol atom economic is. Notably, excellent values of Reaction Mass Efficiency (RME) Optimum Efficiency (OE), and Atom Efficiency (AEf) support the same (see Fig. 2). For calculation of representative entry (5a) see the ESI (S117–S119†).
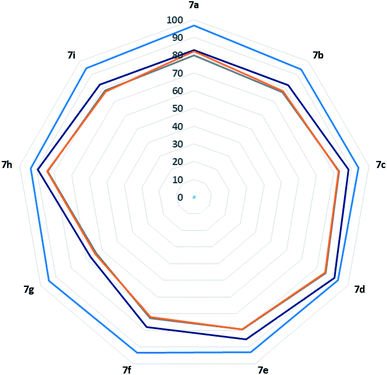 |
| Fig. 2 Radar plot of evaluated green chemistry metrics for compounds 7(a–i). | |
To test the recyclability and efficiency of the catalyst, here we carried out several catalyst recycling experiments on the model reaction of 5-aminoindazole (1), barbituric acids (2a and 2b), with benzaldehyde (3a) for the syntheses of, 6,7,11-tetrahydro-8H-pyrazolo[4,3-f]pyrimido[4,5-b]quinoline-8,10(9H)-dione (5a) and 1′,3′-dimethyl-7,9-diphenyl-3,6,7,9-tetrahydro-2′H-spiro[pyrazolo[4,3-f]quinoline-8,5′-pyrimidine]-2′,4′,6′(1′H,3′H)-trione (7a) accordingly. To our delight, developed catalyst recycled and work efficiently up to four subsequent cycles (Fig. 3). At the end of the reaction, excess solvent was recovered by rotary evaporator operating at 60–70 °C temperature and under reduced pressure. Later on, 5 mL of distilled water was added to the crude reaction mass so as to make the catalyst soluble and complete precipitation of the final product (5a and 7a). Afterward, resultant mixture is filtered and product washed thoroughly twice with 5 mL of distilled water. The filtrate containing soluble catalyst was charged into rotary evaporator to distilled off excess water under reduced pressure and temperature (70–80 °C). The recycled catalyst was allowed to dry under vacuum for 4–5 h at 60 °C temperature for next use. As an auxiliary proof, we have attached 1H-NMR and 13C-NMR spectra of fresh and recycled catalyst in ESI (see the ESI S11–S14†).
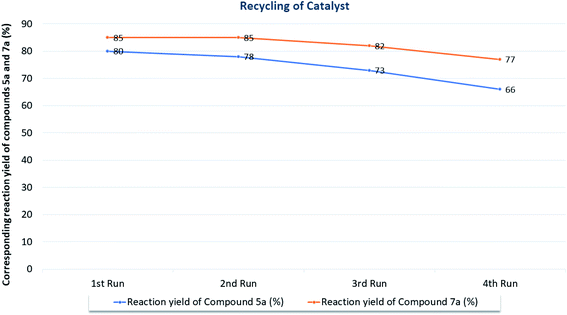 |
| Fig. 3 Efficiency of catalyst. | |
On the basis of our experimental observations, we proposed possible synthetic routes for the formations of two distinct products viz. 11-aryl-3,6,7,11-tetrahydro-8H-pyrazolo[4,3-f]pyrimido[4,5-b]quinoline-8,10(9H)-diones 5(a–k) and tetrahydro-1′H-spiro[pyrazolo[4,3-f]quinoline-8,5′-pyrimidine]-2′,4′,6′(3′H)-triones 7(a–i) by multicomponent reaction of 5-aminoindazole (1) and barbituric acids (2a and 2b) with arylaldehyde 3(a–k) under identical reaction conditions. Initially barbituric acid (2a or 2b) reacts with aldehyde (3(a–k) or 3(a–i)) and produce Knoevenagel adduct (K). This undergoes Michael addition type reaction with 5-aminoindazole to produce an intermediate (L), which endures intramolecular ring closing step and produce 11-aryl-3,6,7,11-tetrahydro-8H-pyrazolo[4,3-f]pyrimido[4,5-b]quinoline-8,10(9H)-diones 5(a–k). On other hand, intermediate (L) formed by the reaction of N,N-dimethyl barbituric acid (2b) and aldehyde reacts with second equivalent of aldehyde in the presence of DEM and produce benzylidene type derivatives (M), which undergoes intramolecular ring closing step via C–C bond formation and furnish high diastereoselective (d.r. up to 20
:
1 (syn
:
anti)) tetrahydro-1′H-spiro[pyrazolo[4,3-f]quinoline]-8,5′-pyrimidine]-2′,4′,6′(3′H)-triones 7(a–i) (see Scheme 2).
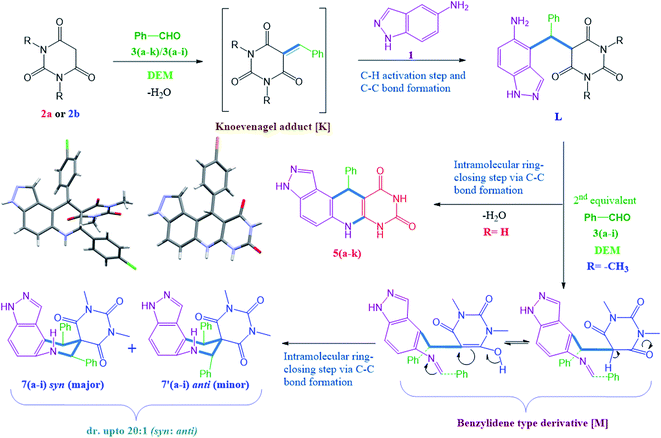 |
| Scheme 2 Plausible reaction mechanism. | |
Conclusion
In conclusion, we successfully explore a substrate directed, eco-compatible, and multicomponent reaction of 5-aminoindazole, aldehyde, and barbituric acids. The key feature of the present protocol is formation of two distinct products viz. 11-aryl-3,6,7,11-tetrahydro-8H-pyrazolo[4,3-f]pyrimido[4,5-b]quinoline-8,10(9H)-diones and tetrahydro-1′H-spiro[pyrazolo[4,3-f]quinoline-8,5′-pyrimidine]-2′,4′,6′(3′H)-triones under the identical reaction conditions. Significantly, the spiro compounds show high diastereoselectivity (dr. up to 20
:
1 (syn
:
anti)).
Experimental section
General methods
All reactions were performed in air, unless specified otherwise. All necessary chemicals utilized in this study were purchased from commercially available sources and used for performing reactions without further purifications. 1H-NMR and 13C-NMR spectral analysis were made using BRUKER AVANCE II 400 NMR Spectrometer using CDCl3 and DMSO-d6 as a solvent with shifts referenced to TMS. The chemical shift values are expressed in parts per million and coupling constants (J) are provided in hertz. The LC-MS analysis of all compounds collected on MS-Agilent 6120 quadrupole. Melting points were determined by the open capillary tube method and are uncorrected.
General procedure for synthesis of 11-aryl-3,6,7,11-tetrahydro-8H-pyrazolo[4,3-f]pyrimido[4,5-b]quinoline-8,10(9H)-dione 5(a–k)
In an oven dried round bottom flask (50 mL capacity) with a magnetic stirrer bar, 5-aminoindazole (1, 1.0 mmol), barbituric acid (2a, 1.0 mmol), aldehyde 3(a–k) (1.0 mmol), DEM (20 mol%) and ethanol (5 mL) were stirred at 70 °C for the stipulated reaction time. The reaction progress was monitored on TLC plate using ethyl acetate: n-hexane (50
:
50, v/v) as eluents. At the end of reaction, solvent was recovered. The crude reaction mass was diluted with 5 mL of distilled water and allowed to stir at room temperature for the fully precipitation of the product and to separate out the water-soluble catalyst. The precipitates of the products were washed thoroughly twice with distilled water (2× 5 mL). All derived compounds were fully characterized by 1H-NMR, 13C-NMR, LC-MS, HPLC, and X-ray single-crystal analysis (for representative compound 5g).
General procedure for synthesis of tetrahydro-1′H-spiro[pyrazolo[4,3-f]quinoline-8,5′-pyrimidine]-2′,4′,6′(3′H)-triones 7(a–i)
In an oven dried round bottom flask (50 mL capacity) with a magnetic stirrer bar, 5-aminoindazole (1, 1.0 mmol), N,N-dimethyl barbituric acid (2b, 1.0 mmol), aldehyde 3(a–i) (2.0 mmol), DEM (20 mol%) and ethanol (5 mL) were stirred at 70 °C for the stipulated reaction time. The reaction progress was monitored on TLC using ethyl acetate: n-hexane (30
:
70, v/v) as eluents. At the end of reaction, solvent was recovered. The crude reaction mass was diluted with 5 mL of distilled water and allowed to stir at room temperature for fully precipitation of the product and to separate out the water-soluble catalyst. The precipitates of the products were washed thoroughly twice with distilled water (2× 5 mL). All derived compounds were fully characterized by 1H-NMR, 13C-NMR, LC-MS, HPLC, and X-ray single-crystal analysis (for representative compounds 7b and 7h).
General procedure for the preparation of betaine based deep eutectic mixtures
All betaine based deep eutectic mixtures were developed by mixing 1 equiv. of betaine (a hydrogen bond acceptor) with 1 equiv. of acid (a hydrogen bond donor), a little amount of deionized water was added and resultant mixture stirred at ambient temperature for 3 h. This resultant DEM was washed thoroughly with absolute ethanol/isopropyl alcohol and allowed to dry at 60 °C temperature under vacuum for 4–5 h.
Spectroscopic and analytical characterization of all 11-aryl-3,6,7,11-tetrahydro-8H-pyrazolo[4,3-f]pyrimido[4,5-b]quinoline-8,10(9H)-dione 5(a–k).
11-Phenyl-3,6,7,11-tetrahydro-8H-pyrazolo[4,3-f]pyrimido[4,5-b]quinoline-8,10(9H)-dione (5a). Light pink solid, % purity (HPLC) = 88.1%, mp 282–284 °C; 1H-NMR (400 MHz, DMSO-d6) (δ, ppm): 12.98 (s, 1H, NH), 10.54 (s, 1H, NH), 10.34 (s, 1H, NH), 8.88 (s, 1H, ArH), 8.02 (s, 1H, ArH), 7.30–7.39 (m, 3H, ArH), 7.13–7.19 (m, 3H, ArH), 7.05 (t, J = 7.2 Hz, 1H, ArH), 5.41 (s, 1H, CH); 13C-NMR (100 MHz, DMSO-d6) (δ, ppm): 162.77, 150.25, 147.13, 145.66, 137.23, 131.76, 128.45, 128.23, 127.92, 127.49, 125.92, 121.48, 117.07, 113.94, 109.43, 85.26, 64.88, 38.08, 15.13; MS(MM-ES + APCI) 329.9 [M + H]+.
11-(4-Chlorophenyl)-3,6,7,11-tetrahydro-8H-pyrazolo[4,3-f]pyrimido[4,5-b]quinoline-8,10(9H)-dione (5b). Off white solid, mp 280–282 °C; 1H-NMR (400 MHz, DMSO-d6) (δ, ppm): 13.05 (s, 1H, NH), 10.67 (s, 1H, NH), 10.45 (s, 1H, NH), 7.52 (s, 1H, ArH), 7.45 (d, J = 9.2 Hz, 2H, ArH), 7.30–7.39 (m, 2H, ArH), 7.22 (d, J = 7.6 Hz, 2H, ArH), 7.07 (d, J = 9.2 Hz, 1H, ArH), 6.91 (s, 1H, CH), 4.91 (s, 1H, CH); 13C-NMR (100 MHz, DMSO-d6) (δ, ppm): 169.98, 165.22, 162.76, 153.25, 146.01, 139.90, 137.25, 137.23, 132.07, 131.24, 130.48, 130.27, 130.20, 129.35, 128.70, 128.66, 128.59, 128.22, 127.87, 127.79, 121.36, 117.13, 116.54, 113.68, 113.29, 109.70, 109.57, 84.89; MS(MM-ES + APCI) 365.8 [M + H]+.
11-(4-Nitrophenyl)-3,6,7,11-tetrahydro-8H-pyrazolo[4,3-f]pyrimido[4,5-b]quinoline-8,10(9H)-dione (5c). Pale yellow solid, mp 268–270 °C; 13C-NMR (100 MHz, DMSO-d6) (δ, ppm): 169.69, 167.73, 162.74, 154.26, 153.20, 150.12, 145.78, 145.72, 137.24, 131.63, 129.57, 128.80, 128.33, 123.92, 123.30, 121.36, 117.27, 112.31, 110.19, 110.12, 84.35; MS(MM-ES + APCI) 376.8 [M + H]+.
11-(4-Methoxyphenyl)-3,6,7,11-tetrahydro-8H-pyrazolo[4,3-f]pyrimido[4,5-b]quinoline-8,10(9H)-dione (5d). Off white solid, % purity (HPLC) = 98.7%, mp 278–280 °C; 1H-NMR (400 MHz, DMSO-d6) (δ, ppm): 12.97 (s, 1H, NH), 10.54 (s, 1H, NH), 10.29 (s, 1H, NH), 8.82 (s, 1H, ArH), 7.34 (d, J = 8.8 Hz, 1H, ArH), 7.22 (d, J = 8.8 Hz, 2H, ArH), 7.13 (d, J = 8.8 Hz, 1H, ArH), 6.72 (d, J = 8.8 Hz, 2H, ArH), 5.36 (s, 1H, CH), 3.63 (s, 1H, OCH3); 13C-NMR (100 MHz, DMSO-d6) (δ, ppm): 162.78, 157.41, 150.19, 145.41, 139.47, 137.24, 131.78, 128.41, 128.10, 121.46, 117.05, 114.26, 113.28, 109.30, 85.46, 54.86, 37.17; MS(MM-ES + APCI) 359.8 [M + H]+.
11-(p-Tolyl)-3,6,7,11-tetrahydro-8H-pyrazolo[4,3-f]pyrimido[4,5-b]quinoline-8,10(9H)-dione (5e). Off white solid, % purity (HPLC) = 98.5%, mp > 300 °C; 1H-NMR (400 MHz, DMSO-d6) (δ, ppm): 12.97 (s, 1H, NH), 10.54 (s, 1H, NH), 10.29 (s, 1H, NH), 8.82 (s, 1H, ArH), 8.01 (s, 1H, ArH), 7.34 (d, J = 8.8 Hz, 1H, ArH), 7.19 (d, J = 8.4 Hz, 2H, ArH), 7.13 (d, J = 8.8 Hz, 1H, ArH), 6.96 (d, J = 8.0 Hz, 2H, ArH), 5.36 (s, 1H, CH), 2.15 (s, 1H, CH3); 13C-NMR (100 MHz, DMSO-d6) (δ, ppm): 162.75, 150.15, 145.45, 144.25, 134.87, 131.79, 128.46, 128.12, 127.37, 117.07, 114.11, 110.66, 109.37, 109.32, 108.0, 90.66, 85.34, 84.77, 20.49; MS(MM-ES + APCI) 345.0 [M + H]+.
11-(4-Fluorophenyl)-3,6,7,11-tetrahydro-8H-pyrazolo[4,3-f]pyrimido[4,5-b]quinoline-8,10(9H)-dione (5f). Off white solid, % purity (HPLC) = 99.5%, mp > 300 °C; 1H-NMR (400 MHz, DMSO-d6) (δ, ppm): 13.0 (s, 1H, NH), 10.57 (s, 1H, NH), 10.33 (s, 1H, NH), 8.88 (s, 1H, ArH), 8.01 (s, 1H, ArH), 7.33–7.38 (m, 3H, ArH), 7.15 (d, J = 8.8 Hz, 1H, ArH), 6.99 (t, J = 8.8 Hz, 2H, ArH), 5.45 (s, 1H, CH); 13C-NMR (100 MHz, DMSO-d6) (δ, ppm): 162.77, 161.69, 159.29, 150.16, 145.53, 143.33, 143.29, 137.25, 131.73, 131.71, 129.27, 129.19, 128.19, 121.39, 117.12, 114.66, 114.45, 113.66, 109.59, 85.16, 37.35; MS(MM-ES + APCI) 349.8 [M + H]+.
11-(4-Bromophenyl)-3,6,7,11-tetrahydro-8H-pyrazolo[4,3-f]pyrimido[4,5-b]quinoline-8,10(9H)-dione (5g). Off white solid, mp 290–292 °C; 1H-NMR (400 MHz, DMSO-d6) (δ, ppm): 13.05 (s, 1H, NH), 10.66 (s, 1H, NH), 10.45 (s, 1H, NH), 7.57 (d, J = 8.4 Hz, 2H, ArH), 7.44 (d, J = 8.8 Hz, 1H, ArH) 7.24–7.34 (m, 2H, ArH), 7.08 (d, J = 8.8 Hz, 1H, ArH), 6.92 (s, 1H, ArH), 4.90 (s, 1H, CH); 13C-NMR (100 MHz, DMSO-d6) (δ, ppm): 169.98, 165.21, 162.79, 153.27, 146.42, 140.32, 137.25, 131.64, 131.28, 131.24, 130.80, 130.55, 130.41, 130.29, 129.86, 129.77, 121.36, 120.61, 119.02, 116.59, 113.61, 110.06, 84.85, 54.91, 42.62; MS(MM-ES + APCI) 408.8 [M + H]+.
11-(3-Methoxyphenyl)-3,6,7,11-tetrahydro-8H-pyrazolo[4,3-f]pyrimido[4,5-b]quinoline-8,10(9H)-dione (5h). White solid, % purity (HPLC) = 99.6%, mp > 300 °C; 1H-NMR (400 MHz, DMSO-d6) (δ, ppm): 12.98 (s, 1H, NH), 10.56 (s, 1H, NH), 10.32 (s, 1H, NH), 8.85 (s, 1H, ArH), 7.36 (d, J = 8.8 Hz, 1H, ArH), 7.06–7.15 (m, 2H, ArH), 6.91 (s, 1H, ArH), 6.85 (d, J = 7.6 Hz, 1H, ArH), 6.64 (dd, J = 6.4 Hz, J = 1.6 Hz, 1H, ArH), 5.39 (s, 1H, CH), 3.66 (s, 3H, OCH3); 13C-NMR (100 MHz, DMSO-d6) (δ, ppm): 162.77, 158.90, 148.59, 138.51, 128.95, 121.49, 119.81, 119.67, 119.53, 117.12, 117.05, 113.90, 113.86, 113.65, 110.66, 109.46, 109.44, 109.41, 109.35, 107.37, 73.14, 54.82; MS(MM-ES + APCI) 360.0 [M + H]+.
11-([1,1′-Biphenyl]-4-yl)-3,6,7,11-tetrahydro-8H-pyrazolo[4,3-f]pyrimido[4,5-b]quinoline-8,10(9H)-dione (5i). Off white solid, mp > 300 °C; 1H-NMR (400 MHz, DMSO-d6) (δ, ppm): 12.97 (s, 1H, NH), 10.54 (s, 1H, NH), 10.34 (s, 1H, NH), 8.88 (s, 1H, ArH), 8.06 (d, J = 16.8 Hz, 1H, ArH), 7.26–7.79 (m, 10H, ArH), 5.44 (s, 1H, CH); 13C-NMR (100 MHz, DMSO-d6) (δ, ppm): 162.81, 162.78, 150.22, 150.20, 146.34, 145.63, 140.06, 138.01, 137.26, 131.81, 129.29, 128.99, 128.92, 128.76, 128.24, 128.04, 127.96, 127.09, 126.69, 126.51, 126.40, 126.08, 121.52, 121.50, 117.13, 117.10, 113.81, 113.78, 109.50, 85.17; MS(MM-ES + APCI) 407.0 [M + H]+.
11-(3-Chlorophenyl)-3,6,7,11-tetrahydro-8H-pyrazolo[4,3-f]pyrimido[4,5-b]quinoline-8,10(9H)-dione (5j). Off white solid, % purity (HPLC) = 99.5%, mp > 300 °C; 1H-NMR (400 MHz, DMSO-d6) (δ, ppm): 13.02 (s, 1H, NH), 10.59 (s, 1H, NH), 10.36 (s, 1H, NH), 8.91 (s, 1H, ArH), 8.05 (s, 1H, ArH), 7.38 (d, J = 8.4 Hz, 2H, ArH), 7.12–7.28 (m, 4H, ArH), 5.46 (s, 1H, CH); 13C-NMR (100 MHz, DMSO-d6) (δ, ppm): 162.75, 157.90, 150.14, 150.13, 149.39, 145.67, 143.05, 137.25, 136.75, 132.60, 131.76, 129.85, 128.30, 128.24, 127.42, 127.23, 126.53, 126.26, 125.98, 117.17, 113.05, 110.22, 109.82, 109.81, 109.81, 109.61, 107.35, 84.77, 79.21; MS(MM-ES + APCI) 365.8 [M + H]+.
11-(2-Chlorophenyl)-3,6,7,11-tetrahydro-8H-pyrazolo[4,3-f]pyrimido[4,5-b]quinoline-8,10(9H)-dione (5k). Off white solid, % purity (HPLC) = 99.0%, mp > 300 °C; 1H-NMR (400 MHz, DMSO-d6) (δ, ppm): 13.04 (s, 1H, NH), 10.49 (s, 1H, NH), 10.30 (s, 1H, NH), 8.93 (s, 1H, ArH), 7.88 (s, 1H, ArH), 7.37 (d, J = 8.4 Hz, 1H, ArH), 7.30 (d, J = 6.8 Hz, 1H, ArH), 7.09–7.19 (m, 3H, ArH), 5.82 (s, 1H, CH); 13C-NMR (100 MHz, DMSO-d6) (δ, ppm): 162.57, 150.16, 145.61, 144.39, 137.15, 131.39, 131.29, 131.24, 128.98, 128.52, 127.68, 127.28, 121.59, 117.30, 112.70, 109.91, 84.77; MS(MM-ES + APCI) 365.8 [M + H]+.
Spectroscopic and analytical characterization of 7,9-diaryl-3,6,7,9-tetrahydro-1′H-spiro[pyrazolo[4,3-f]quinoline-8,5′-pyrimidine]-2′,4′,6′(3′H)-triones (7a–i).
1′,3′-Dimethyl-7,9-diphenyl-3,6,7,9-tetrahydro-2′H-spiro[pyrazolo[4,3-f]quinoline-8,5′-pyrimidine]-2′,4′,6′(1′H,3′H)-trione (7a). White solid, % purity (HPLC) = 98.7%, mp 252–254 °C; 1H-NMR (400 MHz, CDCl3) (δ, ppm): 7.46–7.52 (m, 1H, ArH), 7.29–7.40 (m, 7H, ArH), 7.23–7.27 (m, 2H, ArH), 7.05–7.14 (m, 2H, ArH), 6.81 (d, J = 7.6 Hz, 1H, ArH), 5.42 (s, 1H, CH), 4.95 (s, 1H, CH), 2.99 (s, 3H, CH3), 2.90 (s, 3H, CH3); 13C-NMR (100 MHz, CDCl3) (δ, ppm): 170.20, 165.18, 149.91, 137.72, 136.24, 130.0, 129.83, 129.64, 128.96, 128.82, 128.67, 128.55, 128.36, 128.20, 126.77, 125.80, 120.63, 120.62, 120.58, 64.71, 59.86, 59.85, 50.37; MS(MM-ES + APCI) 465.8 [M + H]+.
7,9-Bis(4-chlorophenyl)-1′,3′-dimethyl-3,6,7,9-tetrahydro-2′H-spiro[pyrazolo[4,3-f]quinoline-8,5′-pyrimidine]-2′,4′,6′(1′H,3′H)-trione (7b). White solid, % purity (HPLC) = 99.3%, mp 246–248 °C; 1H-NMR (400 MHz, CDCl3) (δ, ppm): 7.48 (d, J = 9.2 Hz, 1H, ArH), 7.27–7.36 (m, 4H, ArH), 7.09–7.20 (m, 4H, ArH), 5.39 (s, 1H, CH), 4.92 (s, 1H, CH), 3.04 (s, 3H, CH3), 2.93 (s, 3H, CH3); 13C-NMR (100 MHz, CDCl3) (δ, ppm): 169.74, 164.70, 149.53, 135.84, 135.76, 134.63, 134.41, 131.24, 131.20, 129.29, 129.05, 128.17, 121.83, 63.83, 59.59, 49.37; MS(MM-ES + APCI) 535.8 [M + H]+.
1′,3′-Dimethyl-7,9-bis(4-nitrophenyl)-3,6,7,9-tetrahydro-2′H-spiro[pyrazolo[4,3-f]quinoline-8,5′-pyrimidine]-2′,4′,6′(1′H,3′H)-trione (7c). Pale yellow solid, % purity (HPLC) = 99.6%, mp 264–266 °C; 1H-NMR (400 MHz, CDCl3) (δ, ppm): 8.24 (d, J = 8.8 Hz, 3H, ArH), 8.0 (d, J = 8.8 Hz, 1H, ArH), 7.41–7.56 (m, 4H, ArH), 6.48 (s, 1H, ArH), 5.64 (s, 1H, CH), 5.08 (s, 1H, CH), 3.05 (s, 3H, CH3), 2.92 (s, 3H, CH3); 13C-NMR (100 MHz, CDCl3) (δ, ppm): 169.27, 164.13, 149.11, 148.65, 147.81, 145.20, 142.94, 131.34, 130.70, 128.13, 128.04, 124.25, 124.17, 123.90, 123.83, 123.62, 123.46, 119.57, 64.14, 59.75, 49.62, 49.55, 28.77, 27.99; MS(MM-ES + APCI) 555.8 [M + H]+.
7,9-Bis(4-methoxyphenyl)-1′,3′-dimethyl-3,6,7,9-tetrahydro-2′H-spiro[pyrazolo[4,3-f]quinoline-8,5′-pyrimidine]-2′,4′,6′(1′H,3′H)-trione (7d). Off white solid, % purity (HPLC) = 96.2%, mp 244–246 °C; 1H-NMR (400 MHz, CDCl3) (δ, ppm): 7.58 (d, J = 4.0 Hz, 1H, ArH), 7.16–7.25 (m, 4H, ArH), 6.73–6.83 (m, 4H, ArH), 5.34 (s, 1H, CH), 4.95 (s, 1H, CH), 3.81 (s, 6H, 2-OCH3), 3.02 (s, 3H, CH3), 2.95 (s, 3H, CH3); 13C-NMR (100 MHz, CDCl3) (δ, ppm): 170.15, 170.12, 160.43, 149.91, 131.16, 130.66, 128.03, 127.93, 127.84, 127.75, 114.50, 114.31, 114.17, 113.91, 63.85, 63.78, 63.72, 59.75, 55.31, 55.26, 49.47, 28.49, 27.81; MS(MM-ES + APCI) 525.8 [M + H]+.
1′,3′-Dimethyl-7,9-di-p-tolyl-3,6,7,9-tetrahydro-2′H-spiro[pyrazolo[4,3-f]quinoline-8,5′-pyrimidine]-2′,4′,6′(1′H,3′H)-trione (7e). Off white solid, % purity (HPLC) = 98.7%, mp 178–180 °C; 1H-NMR (400 MHz, CDCl3) (δ, ppm): 7.38 (d, J = 8.8 Hz, 1H, ArH), 7.06–7.25 (m, 8H, ArH), 6.92 (d, J = 8.0 Hz, 1H, ArH), 6.67 (d, J = 8.4 Hz, 1H, ArH), 5.35 (s, 1H, CH), 4.91 (s, 1H, CH), 3.0 (s, 3H, CH3), 2.92 (s, 3H, CH3), 2.33 (s, 6H, 2CH3); 13C-NMR (100 MHz, CDCl3) (δ, ppm): 170.31, 165.30, 150.09, 150.07, 139.52, 138.40, 138.12, 136.01, 134.45, 133.18, 132.67, 129.92, 129.62, 129.51, 129.44, 129.32, 126.63, 121.18, 64.36, 59.72, 59.69, 50.10, 38.27, 28.40, 27.70, 21.17, 21.15; MS(MM-ES + APCI) 493.8 [M + H]+.
7,9-Bis(4-fluorophenyl)-1′,3′-dimethyl-3,6,7,9-tetrahydro-2′H-spiro[pyrazolo[4,3-f]quinoline-8,5′-pyrimidine]-2′,4′,6′(1′H,3′H)-trione (7f). White solid, % purity (HPLC) = 99.4%, mp 262–264 °C; 1H-NMR (400 MHz, CDCl3) (δ, ppm): 7.22–7.37 (m, 4H, ArH), 7.01–7.13 (m, 4H, ArH), 6.81–6.86 (m, 2H, ArH), 6.53 (s, 1H, ArH), 5.42 (s, 1H, CH), 4.93 (s, 1H, CH), 3.03 (s, 3H, CH3), 2.93 (s, 3H, CH3); 13C-NMR (100 MHz, CDCl3) (δ, ppm): 170.04, 164.97, 164.41, 163.75, 161.92, 161.28, 149.68, 133.46, 133.42, 132.08, 132.04, 131.72, 131.64, 131.54, 131.46, 128.67, 128.58, 120.35, 116.17, 115.96, 115.92, 115.71, 115.62, 115.41, 63.93, 60.04, 49.44, 28.50, 27.78; MS(MM-ES + APCI) 501.8 [M + H]+.
7,9-Bis(4-bromophenyl)-1′,3′-dimethyl-3,6,7,9-tetrahydro-2′H-spiro[pyrazolo[4,3-f]quinoline-8,5′-pyrimidine]-2′,4′,6′(1′H,3′H)-trione (7g). White solid, % purity (HPLC) = 98.8%, mp 208–210 °C; 1H-NMR (400 MHz, CDCl3) (δ, ppm): 7.47–7.50 (m, 3H, ArH), 7.40 (d, J = 8.8 Hz, 1H, ArH), 7.20–7.26 (m, 2H, ArH), 7.05–7.13 (m, 3H, ArH), 6.69 (d, J = 8.4 Hz, 1H, ArH), 6.56 (s, 1H, ArH), 5.39 (s, 1H, CH), 4.90 (s, 1H, CH), 3.04 (s, 3H, CH3), 2.93 (s, 3H, CH3); 13C-NMR (100 MHz, CDCl3) (δ, ppm): 169.80, 160.73, 149.56, 138.28, 136.54, 135.04, 132.23, 131.94, 131.92, 131.60, 131.53, 128.44, 123.84, 122.71, 120.96, 111.84, 110.32, 63.99, 59.54, 49.51, 28.60, 27.86; MS(MM-ES + APCI) 623.6 [M + H]+.
7,9-Bis(3-methoxyphenyl)-1′,3′-dimethyl-3,6,7,9-tetrahydro-2′H-spiro[pyrazolo[4,3-f]quinoline-8,5′-pyrimidine]-2′,4′,6′(1′H,3′H)-trione (7h). White solid, % purity (HPLC) = 99.1%, mp 234–236 °C; 13C-NMR (100 MHz, CDCl3) (δ, ppm): 170.25, 170.12, 165.35, 165.14, 160.07, 159.75, 159.58, 150.04, 150.01, 139.19, 139.14, 138.07, 137.76, 130.01, 129.57, 122.38, 122.19, 120.72, 120.60, 118.98, 118.96, 116.0, 115.02, 114.78, 113.17, 112.68, 112.35, 112.33, 109.87, 64.53, 59.58, 59.47, 55.35, 55.28, 55.12, 50.59, 50.29, 28.47, 28.44, 27.78, 27.73; MS(MM-ES + APCI) 525.8 [M + H]+.
7,9-Di([1,1′-biphenyl]-4-yl)-1′,3′-dimethyl-3,6,7,9-tetrahydro-2′H-spiro[pyrazolo[4,3-f]quinoline-8,5′-pyrimidine]-2′,4′,6′(1′H,3′H)-trione (7i). Off white solid, % purity (HPLC) = 98.3%, mp 258–260 °C; 1H-NMR (400 MHz, CDCl3) (δ, ppm): 7.53–7.65 (m, 7H, ArH), 7.28–7.51 (m, 11H, ArH), 7.08 (d, J = 6.0 Hz, 1H, ArH), 6.88 (d, J = 8.4 Hz, 1H, ArH), 5.47 (s, 1H, CH), 5.02 (s, 1H, CH), 3.04 (s, 3H, CH3), 2.95 (s, 3H, CH3); 13C-NMR (100 MHz, CDCl3) (δ, ppm): 170.18, 165.19, 149.86, 142.55, 141.10, 140.10, 139.87, 136.59, 135.06, 134.95, 130.46, 130.22, 128.90, 128.81, 127.86, 127.56, 127.28, 127.22, 127.10, 127.06, 126.96, 121.23, 64.40, 64.33, 59.77, 50.10, 50.01, 49.94, 28.53, 27.82; MS(MM-ES + APCI) 617.8 [M + H]+.
Conflicts of interest
There are no conflicts to declare.
Acknowledgements
Authors are grateful to Department of Chemistry, Sardar Patel University, DST-SERB, New Delhi, India, for providing research grant (File No. EEQ/2016/000376, dated 07/02/2017), UGC-CPEPA, New Delhi, India, phase-II, for assistance in general and for the NMR facility in particular, sponsored under award letter no. F. No. 1-14/2002-2016 (NS/PE) dated 28/04/2016, UGC-CAS, phase-II for assistance in general, sponsored under award letter no. F-540/5/CAS-II/2018 (SAP-I) dated 25/01/2018, and analytical scientist Mr Manoj Mangukiya, Aether Industries Ltd. Surat, for analytical testing. This work is dedicated to my beloved parents.
References
- A. Domling, W. Wang and K. Wang, Chem. Rev., 2012, 112, 3083 CrossRef CAS PubMed.
- B. H. Rotstein, S. Zaretsky, V. Rai and A. K. Yudin, Chem. Rev., 2014, 114, 8323 CrossRef CAS PubMed.
- J. Yu, F. Shi and L.-Z. Gong, Acc. Chem. Res., 2011, 44, 1156–1171 CrossRef CAS PubMed.
- T. Shi, L. Kaneko, M. Sandino, R. Busse, M. Zhang, D. Mason, J. Machulis, A. J. Ambrose, D. D. Zhang and E. Chapman, ACS Sustainable Chem. Eng., 2019, 7, 1524 CrossRef CAS PubMed.
- P. Liu, J.-W. Hao, L.-P. Mo and Z.-H. Zhang, RSC Adv., 2015, 5, 48675–48704 RSC.
- J. D. Sunderhaus and S. F. Martin, Chem.–Eur. J., 2009, 15, 1300 CrossRef CAS PubMed.
- J. L. Wang, X. Y. Chen, Q. Wu and X. F. Lin, Adv. Synth. Catal., 2014, 356, 999 CrossRef CAS.
- X.-X. Sun, C. Li, Y.-Y. He, Z.-Q. Zhu, G.-J. Mei and F. Shi, Adv. Synth. Catal., 2017, 359, 2660 CrossRef CAS.
- D. M. Patel, M. G. Sharma, R. M. Vala, I. Lagunes, A. Puerta, J. M. Padron, D. P. Rajani and H. M. Patel, Bioorg. Chem., 2019, 86, 137–150 CrossRef CAS PubMed.
- L.-Y. Zeng, B. Xi, K. Huang, J. Bi, L. Wei, C. Cai and S. Liu, ACS Comb. Sci., 2019, 21(10), 656–665 CrossRef CAS PubMed.
- B. Yu, Z. Yu, P. P. Qi, D. Q. Yu and H. M. Liu, Eur. J. Med. Chem., 2015, 95, 35–40 CrossRef CAS PubMed.
- K. Saravana Mani, W. Kaminsky and S. P. Rajendran, New J. Chem., 2018, 42, 301–310 RSC.
- P. Saraswat, G. Jeyabalan, M. Z. Hassan, M. U. Rahman and N. K. Nyola, Synth. Commun., 2016, 46, 1643 CrossRef CAS.
- A. Barakat, S. M. Soliman, A. Mohammed Al-Majid, M. Ali, M. Shahidul Islam, Y. A. M. M. Elshaier and H. A. Ghabbour, J. Mol. Struct., 2018, 1152, 101–114 CrossRef CAS.
- R. A. Cacho, Y. H. Chooi, H. Zhou and Y. Tang, ACS Chem. Biol., 2013, 8, 2322–2330 CrossRef CAS PubMed.
- M. De Candia, C. Altamura, N. Denora, S. Cellamare, M. Nuzzolese, D. De Vito, L. G. Voskressensky, A. V. Varlamov and C. D. Altomare, Chem. Heterocycl. Compd., 2017, 53, 357–363 CrossRef CAS.
- Y. Zheng, C. M. Tice and S. B. Singh, Bioorg. Med. Chem. Lett., 2014, 24, 36733682 Search PubMed.
- X. Cheng, P. Gao, L. Sun, Y. Tian, P. Zhan and X. Liu, Expert Opin. Ther. Pat., 2017, 27, 1277–1286 CrossRef CAS PubMed.
- H. Chen and D. Q. Shi, Tetrahedron, 2011, 67, 5686–5692 CrossRef CAS.
- Y. Zou, Y. Hu, H. Liu and D. Q. Shi, ACS Comb. Sci., 2012, 14, 38–43 CrossRef CAS PubMed.
- H. Chen and D. Q. Shi, J. Comb. Chem., 2010, 12(4), 571–576 CrossRef CAS PubMed.
- S.-H. Kim, A. T. Pudzianowski, K. J. Leavitt, J. Barbosa, P. A. McDonnell, W. J. Metzler, B. M. Rankin, R. Liu, W. Vaccaro and W. Pitts, Bioorg. Med. Chem. Lett., 2005, 15, 1101–1106 CrossRef CAS PubMed.
- R. J. Fessenden, J. G. Larsen, M. D. Coon and J. S. Fessenden, J. Med. Chem., 1964, 7, 695–698 CrossRef CAS PubMed.
- C. Shen, A. Wang, J. Xu, Z. An, K. Y. Loh, P. Zhang and X. Liu, Chem, 2019, 5, 1059 CAS.
- J. Xu, L. Qiao, B. Ying, X. Zhu, C. Shen and P. Zhang, Org. Chem. Front., 2017, 4, 1116 RSC.
- J. Xu, H. Yang, H. Cai, H. Bao, W. Li and P. Zhang, Org. Lett., 2019, 21, 4698–4702 CrossRef CAS PubMed.
- J. Xu, K. Du, J. Shen, C. Shen, K. Chai and P. Zhang, ChemCatChem, 2018, 10, 3675 CrossRef CAS.
- X. S. Wang, M. M. Zhang, H. Jiang, Q. Li, D. Q. Shi and S. J. Tu, Chem. Lett., 2007, 36, 450–451 CrossRef CAS.
- D. Bhuyan, R. Sarma and D. Prajapati, Tetrahedron Lett., 2012, 53, 6460–6463 CrossRef CAS.
- M. M. Sarmah, S. Borthakur, D. Bhuyan and D. Prajapati, RSC Adv., 2015, 5, 68839 RSC.
- S. Stolte, M. Matzke, J. Arning, A. Böschen, W. Pitner, U. Welz-Biermann, B. Jastorff and J. Ranke, Green Chem., 2007, 9, 1170–1179 RSC.
- M. Matzke, S. Stolte, K. Thiele, T. Juffernholz, J. Arning, J. Ranke, U. Welz-Biermann and B. Jastorff, Green Chem., 2007, 9, 1198–1207 RSC.
- E. Massolo, S. Palmieri, M. Benaglia, V. Capriati and F. M. Perna, Green Chem., 2016, 18, 792–797 RSC.
- L. Cicco, N. Ríos-Lombardía, M. J. Rodríguez-Á lvarez, F. Morís, F. M. Perna, V. Capriati, J. García-Álvarez and J. Gonzalez-Sabín, Green Chem., 2018, 20, 3468–3475 RSC.
- F. M. Perna, P. Vitale and V. Capriati, Current Opinion in Green and Sustainable Chemistry, 2020, 21, 27–33 CrossRef.
- D. M. Patel and H. M. Patel, ACS Sustainable Chem. Eng., 2019, 7(22), 18667–18676 CrossRef CAS.
- A. Paiva, R. Craveiro, I. Aroso, M. Martins, R. L. Reis and A. R. C. Duarte, ACS Sustainable Chem. Eng., 2014, 2, 1063–1071 CrossRef CAS.
- D. M. Patel, R. M. Vala, M. G. Sharma, D. P. Rajani and H. M. Patel, ChemistrySelect, 2019, 4, 1031–1041 CrossRef CAS.
- M. G. Sharma, D. P. Rajani and H. M. Patel, R. Soc. Open Sci., 2017, 4, 170006 CrossRef CAS PubMed.
- M. G. Sharma, R. M. Vala, D. M. Patel, I. Lagunes, M. X. Fernandes, J. M. Padron, V. Ramkumar, R. L. Gardas and H. M. Patel, ChemistrySelect, 2018, 3, 12163–12168 CrossRef CAS.
- R. M. Vala, D. M. Patel, M. G. Sharma and H. M. Patel, RSC Adv., 2019, 9, 28886 RSC.
- M. G. Sharma, J. Pandya, D. M. Patel, R. M. Vala, V. Ramkumar, R. Subramanian, V. K. Gupta, R. L. Gardas, A. Dhanasekaran and H. M. Patel, Polycyclic Aromat. Compd., 2019, 39, 1–11 CrossRef.
- K. Nikoofar, H. Heidari and Y. Shahedi, Cellulose, 2018, 25, 5697–5709 CrossRef CAS.
- P. T. Anastas and J. C. Warner, Green Chemistry Theory and Practice, Oxford University Press, Oxford, 1998 Search PubMed.
- R. A. Sheldon, The E factor: fifteen years on, Green Chem., 2007, 9, 1273 RSC.
- Green Chemistry Metrics: Measuring and Monitoring Sustainable Processes, ed. A. Lapkin and D. Constable, Wiley-Blackwell, 2008 Search PubMed.
- R. A. Sheldon, ACS Sustainable Chem. Eng., 2018, 6, 32–48 CrossRef CAS.
- S. Abou-Shehada, P. Mampuys, B. U. W. Maes, J. Clarkand and H. L. Summerton, Green Chem., 2017, 19, 249–258 RSC.
- J. J. Song, J. T. Reeves, D. R. Fandrick, Z. Tan, N. K. Yee and C. H. Senanayake, Green Chem. Lett. Rev., 2008, 1, 141 CrossRef CAS.
- B. M. Trost and C. J. Li, in Handbook of Green Chemistry, Wiley-VCH Verlag GmbH & Co. KGaA, Weinheim, 2012, p. 7 Search PubMed.
- C. R. McElroy, A. Constantinou, L. C. Jones, L. Summerton and J. H. Clark, Green Chem., 2015, 17, 3111–3121 RSC.
Footnote |
† Electronic supplementary information (ESI) available: This include all spectroscopic and analytical data, X-ray single-crystal reports, copy of 1H-NMR and 13C-NMR of all representative entries, LC-MS, HPLC reports, and green chemistry metrics calculation for representative entry. CCDC 1990292, 1978229 and 1968978. For ESI and crystallographic data in CIF or other electronic format see DOI: 10.1039/d0ra02990d |
|
This journal is © The Royal Society of Chemistry 2020 |
Click here to see how this site uses Cookies. View our privacy policy here.