DOI:
10.1039/D0RA03211E
(Paper)
RSC Adv., 2020,
10, 16510-16514
PPh3/NaI driven photocatalytic decarboxylative radical cascade alkylarylation reaction of 2-isocyanobiaryls†
Received
18th February 2020
, Accepted 14th April 2020
First published on 25th April 2020
Abstract
The first triphenylphosphine/sodium iodide driven photocatalytic decarboxylative cascade cyclization of 2-isocyano-biaryls with alkyl N-hydroxyphthalimide (NHP) esters was developed. This operationally simple protocol results in multiple carbon–carbon bond formation under transition metal free conditions, affording a novel and environmentally benign entry to producing 6-alkyl phenanthridines with moderate to good yields.
Introduction
Visible-light photocatalysis has been widely recognized as a powerful tool in organic synthesis to construct carbon–carbon or carbon–heteroatom bonds.1 Through reactive intermediates such as radicals and radical ions, unique reactions that are previously inaccessible under thermal conditions can be accessed. Significant advances have been realized in this field by employing metal based catalysts2 or organic dyes3a–d as photoredox catalysts. However, employing these metal based photoredox catalysts has certain disadvantages: such as; they are expensive, potentially toxic and limited availability.3e Recently, visible light driven photoredox catalysis employing inexpensive chemicals such as combination of triphenylphosphine/sodium iodide and CeCl3 emerged as a robust alternative to generate radical entities under mild reaction conditions.4 This approach replaces metal based photocatalysts by inexpensive catalysts, thus overcoming some of the aforementioned problems.
Phenanthridine, a privileged structural core motif found in natural products.5 Many synthetic phenanthridine derivatives show bioactive and pharmaceutical properties, including anti-tumoral, antibacterial, antiviral, cytotoxic, and DNA inhibitory properties.6,7 In addition, phenanthridine derivatives reveal significant optoelectronic properties.8 Therefore, the development of new and efficient methods for the preparation of phenanthridine derivatives has gained significant importance in academic research. Recently, a cascade radical pathway involving radical addition to 2-isocyanobiaryls and subsequently intramolecular homolytic aromatic substitution has been developed,9 which allows the rapid assembly of a phenanthridine framework with high efficiency. In 2012, Chatani's group disclosed Mn(III)-mediated radical cascade reaction of 2-isocyanobiaryls with boronic acid under thermal conditions.10 Subsequently, several groups put their efforts for the construction of 6-substituted phenanthridines through the reaction of 2-isocyanobiaryls with corresponding carbon radical precursors, such as simple alkanes,11 alcohols,11a ethers,12 aldehydes,13 2-bromide ethyl esters,14 aryl sulfonyl chlorides15 and 1,3-dicarbonyl compounds.16 Although these reported methods have their own specific applications, but remains associated with certain disadvantages such as employing metal catalysts, harsh reaction condition and use of stoichiometric amounts of oxidants. Very recently, few groups reported a metal-free approach that utilizes aryl amines,17 carboxylic acids,18 hydrazines19 acyl peroxides,20 as a carbon radical precursor, which appears to be an environmentally benign method for accessing 6-substituted phenanthridines. However, most of acyl peroxides, alkyl amines and hydrazenes are commercially unavailable. Thus, further new methodologies are quite desired for the synthesis of 6-substituted phenanthridines.
Recently decarboxylative functionalization of alkyl N-hydroxyphthalimide (NHP) esters in carbon–carbon bond formation reactions have gained a significant importance, as they convert widely available and inexpensive chemicals into valuable chemicals and reactive intermediates for synthesis.21 Photo decarboxylation of alkyl N-hydroxyphthalimide (NHP) esters has been widely used for organic synthesis,22 as the liberation of phthalimide and volatile CO2 as a by-products indicates a strong driving force for the reaction, forming versatile radical intermediates to build more valuable products. Herein, we report the first PPh3/NaI driven photocatalyzed decarboxylative cascade alkylarylation reaction of 2 isocyanobiaryls in the presence of visible light irradiation at room temperature.
Results and discussions
Inspired by the recent work of Shang and Fu (Fig. 1) who developed photocatalytic decarboxylative alkylations mediated by triphenylphosphine/sodium iodide,4d we wondered how these activated carboxylic acids (1) would behave under similar reaction conditions (Fig. 1) in presence of 2-isocyanobiaryls (2). At first, we chose 1a and 2a as model reactants to optimize the reaction conditions for the desired decarboxylative radical cascade alkylarylation using inexpensive PPh3/NaI as a photocatalyst. To our delight, when a solution of 1.5 equiv. of 1a with 1.0 equiv. of 2a in the presence of PPh3 (20 mol%) and NaI (100 mol%) in acetonitrile was illuminated with a blue LED (455 nm) at 25 °C for 24 h, product 3a was formed in 84% yield (Fig. 2, entry 1). Replacement of NaI by KI also proceeded smoothly to give product 3a in 80% yield (Fig. 2, entry 2). The product formation 3a was slightly decreased upon employing other iodide salts (Fig. 2, entries 3 and 4). Solvents such as dimethylsulfoxide (DMSO) and dimethylformamide (DMF) in place of MeCN afforded 3a in 65% and 60% yield respectively (Fig. 2, entries 5 and 6). While solvents such as acetone, chloroform (CHCl3) and EtOAc had a deleterious effect on the reaction outcome (Fig. 2, entries 7–9). When triphenyl phosphine was replaced by other phosphines such as PCy3 and P(o-tol)3, caused a drastic reduction in the yield of the reaction (Fig. 2, entries 10 and 11). Substitution of PPh3 by other nitrogen donors such as DMAP, Et3N observed trace amount of product (Fig. 2, entries 12 and 13). Additionally, control experiments confirmed that a catalytic amount of PPh3 and continuous blue light irradiation were necessary for the reaction to occur. Not even traces amount of 3a were observed in the absence of light or the PPh3 (Fig. 2, entries 15 and 17).
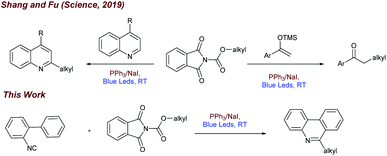 |
| Fig. 1 Decarboxylative alkylations driven by PPh3/NaI photoredox catalysis. | |
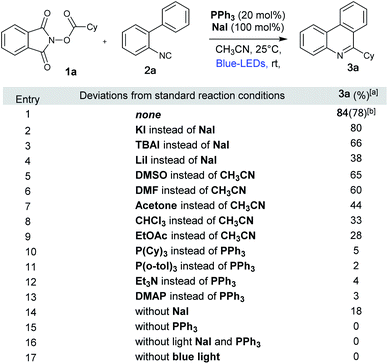 |
| Fig. 2 Optimization of the reaction conditions. 1a (0.3 mmol), 2a (0.2 mmol), PPh3 (20 mol%), NaI (100 mol%), CH3CN (3 mL) at 25 °C, 455 nm LED for 24 h. [a]NMR yields using benzyl alcohol as internal standard. [b]Isolated yield. | |
With the optimized reaction conditions in our hand, we survey the scope of the reaction. As shown in Fig. 3, a broad range of alkyl NHP esters (1), reacted with 2-isocyanobiphenyl (2a) providing the corresponding 6-alkyl phenanthridines in good to moderate yields. Different types of secondary cyclic (1a–1b) and acyclic (1c–1f) alkyl NHP esters participate in this reaction to provide good yields (3a–3f, 67–78%). We were pleased to find that different functional groups such as amide (1g) and ketone (1h) can be tolerated in our reaction conditions to produce the corresponding 6-substituted phenanthridines (3g and 3h) in 65% and 68% yield. Tertiary alkyl NHP esters were smoothly converted to 6-alkyl phenanthridines in good yields (3i–3k, 70–77%). Primary alkyl NHP-esters containing variety of functional groups readily participated in this reaction and gave corresponding 6-alkyl phenanthridines in good to moderate yields. Primary alkyl NHP-esters derived from isovaleric acid, 4-phenyl buteric acid and 3-bromo-phenyl proponoic acid gave the corresponding 6-substituted phenanthridines (3l–3n) in moderate yields (60–63%). Terminal alkynes (1o) and alkenes (1p) are tolerated under these reaction conditions gave the corresponding products 3o and 3p in 58% and 56% yield. Also, primary alkyl NHP-esters (1q and 1r) derived from 2-methoxy aceticacid and 4-oxopentanoic acid were successfully converted to 6-alkyl phenanthridines 3q and 3r in moderate yields (57% and 56%).
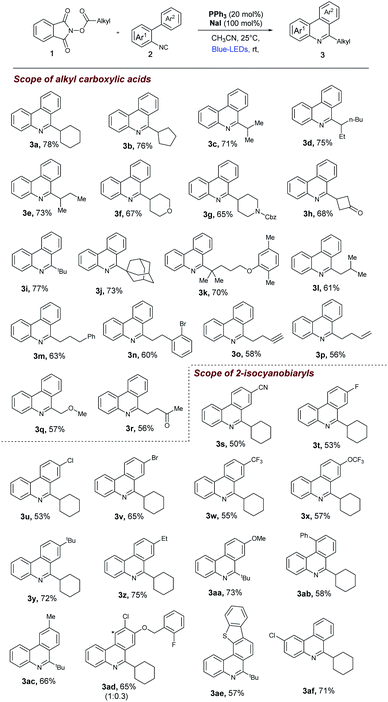 |
| Fig. 3 Decarboxylative cascade alkylarylation reaction of 2-isocyanobiaryls with alkyl NHP-esters. Reaction conditions as given in Fig. 2 (entry 1). Isolated yields, average of at least two independent runs. *regioisomer. | |
Next, the scope of 2-isocyanobiaryls was examined. As shown in Fig. 3, first, the electronic variation at the para position of the Ar2 ring was studied with NHP-esters (1a and 1i). Electron withdrawing groups such as cyano, fluoro, chloro, bromo, trifluoro methyl, trifluoromethoxy groups were all well tolerated, giving 6-substituted phenanthridines (3s–3x) in 50–65% yields. Electron donating groups such as tbutyl, ethyl, methoxy gave the corresponding 6-alkyl substituted phenanthridines (3y–3aa) in 72–75% yields. Next ortho- and meta-substituted 2-isocyano biphenyls were tested in our reaction conditions. In case of ortho-phenyl substituted 2-isocyano biphenyl gave the corresponding substituted phenanthridines (3ab) in 58% yield. In case of meta-methyl substituted 2-isocyano biphenyl gave the corresponding 6-alkyl phenanthridine (3ac) as a single regio-isomer in 66% yield. In case of meta–para substituted 2-isocyano biphenyl gave the 6-alkyl phenanthridines (3ad) as mixture of regio-isomers 65% yield. Dibenzothiofuran and benzyloxy groups can be tolerated in our reaction conditions. In case of 2-isocyano biphenyl containing dibenzothiofuran with alkyl NHP-ester (1i) gave the 6-alkyl phenantharidine (3ae) in 57% yield. Finally, we tested one example of 2-isocyanobiphenyl (2a) by introducing substitution on Ar1 ring was studied with NHP-esters (1a) in our reaction conditions provided 6-alkyl phenantharidine (3af) in 71% yield. Further, we have found that Katritzky's N-cyclohexylpyridinium salt (4) and Togni's reagent (5) can be activated by PPh3/NaI system to deliver 3a (75% yield) and 6-trifluoromethyl substituted phenanthridine (3ag) in good yield (78% yield, see ESI†).
The efficiency of our photocatalytic decarboxylative radical cascade cyclization leads us to conduct some preliminary mechanistic studies (Fig. 4). As anticipated ON/OFF experiment indicated that our reaction required continuous blue light irradiation to proceed (see ESI†). We currently believe that this decarboxylative cascade cyclization reaction could proceed via the generation of alkyl radicals. In a radical clock experiment using alkyl NHP-ester (1s) derived from 2-cyclopropylacetic acid under our reaction conditions, the ring-opened product 3p was isolated (Fig. 4, upper). Moreover, secondary chiral alkyl NHP-ester (1t) derived from enantiopure (S)-2-methylbutanoic acid provided the racemic 6-alkyl substituted phenanthridines product 3e (Fig. 4, center).
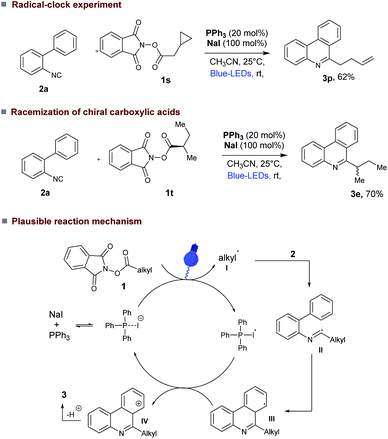 |
| Fig. 4 Preliminary mechanistic investigations; (Upper) radical clock experiment; (Center) racemization of chiral alkyl NHP-ester; (Bottom) plausible reaction mechanism. | |
Based on these experimental observations and the report of Rui Shang and Yao Fu et al.4d we propose that the decarboxylative cascade cyclization reaction proceeds via formation of charge transfer complex (CTC)23 (see ESI† for UV-visible absorption spectra) between PPh3, NaI and NHP-ester (1a) (Fig. 4).4d After photo fragmentation of this CTC complex generates key alkyl radical I and PPh3-I˙. Subsequently, the generated alkyl radical is added to 2-isocyanobiaryl 2, which produce imine radical II that can form intermediate III through an intramolecular radical cyclization. Further oxidation of III by PPh3-I˙ (Ered = 0.69 vs. SCE)4d produces the corresponding carbocation IV and NaI, PPh3. The carbocation IV loses a proton under reaction conditions to provide the desired product 3.
Conclusions
In summary, we have developed a general strategy for the catalytic, radical decarboxylative cascade alkyl arylation of alkyl NHP-esters using an inexpensive PPh3/NaI system as a photocatalyst. This operationally simple protocol allows an efficient synthesis of 6-substituted phenanthridines in moderate to good yields under metal free conditions. Furthermore we showed that in our photocatalytic conditions, different carbon radical precursors can be activated to deliver 6-substituted phenanthridines.
Conflicts of interest
There are no conflicts to declare.
Acknowledgements
V. R. Y. acknowledges SERB, New Delhi, for the financial support of Ramanujan Fellowship (SB/S2/RJN-138/2018). V. R. Y. thanks to Dr Ch. Raji Reddy for his kind support. V. R. Y. thanks to Prof. Burkhard König for providing the blue LED set up. V. R. Y thank to Dr Prathama S. Mainkar and Dr S. Chandrasekhar (Director, CSIR-IICT) for their kind support and encouragement. CSIR-IICT Communication No. for this manuscript is IICT/Pubs./2020/025.
Notes and references
- For selected reviews on photoredox catalysis, see:
(a) L. Marzo, S. K. Pagire, O. Reiser and B. König, Angew. Chem., Int. Ed., 2018, 57, 10034 (Angew. Chem., 2018, 130, 10188) CrossRef PubMed;
(b) M. Kärkäs, J. Porco Jr and C. Stephenson, Chem. Rev., 2016, 116, 9683 CrossRef PubMed;
(c) N. A. Romero and D. A. Nicewicz, Chem. Rev., 2016, 116, 10075 CrossRef PubMed;
(d) K. Skubi, T. Blum and T. Yoon, Chem. Rev., 2016, 116, 10035 CrossRef PubMed;
(e) M. H. Shaw, J. Twilton and D. W. C. MacMillan, J. Org. Chem., 2016, 81, 6898 CrossRef PubMed;
(f) J. Twilton, C. C. Le, P. Zhang, M. H. Shaw, R. W. Evans and D. W. C. MacMillan, Nat. Rev. Chem., 2017, 1, 52 CrossRef;
(g) Y. Xi, H. Yi and A. Lei, Org. Biomol. Chem., 2013, 11, 2387 RSC.
- For selected examples of Ir and Ru based photocatalysts see:
(a) Z. Zuo, D. Ahneman, L. Chu, J. Terrett, A. Doyle and D. W. C. MacMillan, Science, 2014, 345, 437 CrossRef CAS PubMed;
(b) A. Musacchio, L. Nguyen, G. Beard and R. Knowles, J. Am. Chem. Soc., 2014, 136, 12217 CrossRef CAS PubMed;
(c) J. Jeffrey, J. Terrett and D. W. C. MacMillan, Science, 2015, 349, 1532 CrossRef CAS;
(d) J. Chu and T. Rovis, Nature, 2016, 539, 272 CrossRef PubMed;
(e) For other metals like Cu, Zn, Ni, Cr, Co and Fe based photocatalytsts see recent review; B. M. Hockin, C. Li, N. Robertson and E. Zysman-Colman, Catal. Sci. Technol., 2019, 9, 889 RSC.
-
(a) D. A. Nicewicz and T. M. Nguyen, ACS Catal., 2014, 4, 355 CrossRef CAS;
(b) N. A. Romero and D. A. Nicewicz, Chem. Rev., 2016, 116, 10075 CrossRef CAS PubMed;
(c) D. P. Hari and B. König, Chem. Commun., 2014, 50, 6688 RSC;
(d) M. K. Bogdos, E. Pinard and J. A. Murphy, Beilstein J. Org. Chem., 2018, 14, 2035 CrossRef CAS PubMed;
(e) M. Neumann, S. Füldner, B. König and K. Zeitler, Angew. Chem., Int. Ed., 2011, 50, 951 (Angew. Chem., 2011, 123, 981) CrossRef CAS PubMed.
-
(a) A. Hu, J.-J. Guo, H. Pan, H. Tang, Z. Gao and Z. Zuo, J. Am. Chem. Soc., 2018, 140, 1612 CrossRef CAS PubMed;
(b) A. Hu, J.-J. Guo, H. Pan and Z. Zuo, Science, 2018, 361, 668 CrossRef CAS PubMed;
(c) A. Hu, Y. Chen, J.-J. Guo, N. Yu, Q. An and Z. Zuo, J. Am. Chem. Soc., 2018, 140, 13580 CrossRef CAS PubMed;
(d) M.-C. Fu, R. Shang, B. Zhao, B. Wang and Y. Fu, Science, 2019, 363, 1429 CrossRef CAS PubMed;
(e) A. Hossain, A. Vidyasagar, C. Eichinger, C. Lankes, J. Phan, J. Rehbein and O. Reiser, Angew. Chem., Int. Ed., 2018, 57, 8288 (Angew. Chem., 2018, 130, 8420) CrossRef CAS;
(f) V. R. Yatham, P. Bellotti and B. König, Chem. Commun., 2019, 55, 3489 RSC;
(g) J. Schwarz and B. König, Chem. Commun., 2019, 55, 486 RSC;
(h) K. Zhang, L. Chang, Q. An, X. Wang and Z. Zuo, J. Am. Chem. Soc., 2019, 141, 10556 CrossRef CAS;
(i) Y. Li, K. Zhou, Z. Wen, S. Cao, X. Shen, M. Lei and L. Gong, J. Am. Chem. Soc., 2019, 141, 10556 CrossRef PubMed;
(j) H. Yin, P. J. Carroll, J. M. Anna and E. J. Schelter, J. Am. Chem. Soc., 2015, 137, 9234 CrossRef CAS;
(k) Y. Qiao and E. J. Schelter, Acc. Chem. Res., 2018, 51, 2926 CrossRef CAS;
(l) While this manuscript was under preparation, R. Shang, Y. Fu et al. reported a related methodology using PPh3/NaI as a photoredox catalyst: Y.-T. Wang, M.-C. Fu, B. Zhao, R. Shang and Y. Fu, Chem. Commun., 2020, 56, 2495 RSC.
-
(a) M. Suffnes and G. A. Cordell, The Alkaloids, Academic, New York, 1985, vol. 25, p. 178 Search PubMed;
(b) T. Nakanishi, M. Suzuki, A. Saimoto and T. J. Kabasawa, Nat. Prod., 1999, 62, 864 CrossRef CAS PubMed;
(c) T. Ishikawa, Med. Res. Rev., 2001, 21, 61 CrossRef CAS;
(d) J. Hu, X. Shi, J. Chen, X. Mao, L. Zhu, L. Yu and J. Shi, Food Chem., 2014, 148, 437 CrossRef CAS PubMed.
-
(a) S. Simeon, J. L. Rios and A. Villar, Pharmazie, 1989, 44, 593 CAS;
(b) S. D. Phillips and R. N. Castle, J. Heterocycl. Chem., 1981, 18, 223 CrossRef CAS.
-
(a) O. B. Abdel-Halim, T. Morikawa, S. Ando, H. Matsuda and M. Yoshikawa, J. Nat. Prod., 2004, 67, 1119 CrossRef CAS PubMed;
(b) L. Sripada, J. A. Teske and A. Deiters, Org. Biomol. Chem., 2008, 6, 263 RSC.
-
(a) J. Zhang and J. R. Lakowicz, J. Phys. Chem. B, 2005, 109, 8701 CrossRef CAS PubMed;
(b) N. Stevens, N. O'Connor, H. Vishwasrao, D. Samaroo, E. R. Kandel, D. L. Akins, C. M. Drain and N. J. Turro, J. Am. Chem. Soc., 2008, 130, 7182 CrossRef CAS.
- Early examples of radical chemistry with isonitriles see;
(a) D. P. Curran and H. Liu, J. Am. Chem. Soc., 1992, 114, 5863 CrossRef CAS;
(b) D. P. Curran, S.-B. Ko and H. Josien, Angew. Chem., Int. Ed. Engl., 1995, 34, 2683 (Angew. Chem., 2012, 107, 2948) CrossRef CAS;
(c) D. Nanni, P. Pareschi, C. Rizzoli, P. Sgarabotto and T. Tundo, Tetrahedron, 1995, 51, 9045 CrossRef CAS;
(d) B. Janza and A. Studer, Org. Lett., 2006, 8, 1875 CrossRef CAS PubMedFor a recent review on radical addition to biphenyl isonitrile see;
(e) B. Zhang and A. Studer, Chem. Soc. Rev., 2015, 44, 3505 RSC.
- M. Tobisu, K. Koh, T. Furukawa and N. Chatani, Angew. Chem., Int. Ed., 2012, 51, 11363 (Angew. Chem., 2012, 124, 11525) CrossRef CAS PubMed.
-
(a) Z. J. Li, F. H. Fan, J. Yang and Z. Q. Liu, Org. Lett., 2014, 16, 3396 CrossRef CAS PubMed;
(b) W. X. Sha, J. T. Yu, Y. Jiang, H. T. Yang and J. Cheng, Chem. Commun., 2014, 50, 9179 RSC;
(c) Z. Q. Zhu, T. T. Wang, P. Bai and Z. Z. Huang, Org. Biomol. Chem., 2014, 12, 5839 RSC.
-
(a) J. J. Cao, T. H. Zhu, S. Y. Wang, Z. Y. Gu, X. Wang and X. S. J. Ji, Chem. Commun., 2014, 50, 6439 RSC;
(b) L. Wang, W. Sha, Q. Dai, X. Feng, W. Wu, H. Peng, B. Chen and J. Cheng, Org. Lett., 2014, 16, 2088 CrossRef CAS PubMed.
- D. Leifert, C. G. Daniliuc and A. Studer, Org. Lett., 2013, 15, 6286 CrossRef CAS.
- H. Jiang, Y. Cheng, R. Wang, M. Zheng, Y. Zhang and S. Yu, Angew. Chem., Int. Ed., 2013, 52, 13289 (Angew. Chem., 2013, 125, 13531) CrossRef CAS.
- L. Gu, C. Jin, J. Liu, H. Ding and B. Fan, Chem. Commun., 2014, 50, 4643 RSC.
- J. J. Cao, X. Wang, S. Y. Wang and S. J. Ji, Chem. Commun., 2014, 50, 12892 RSC.
- Z. H. Xia, J. B. Huang, Y. M. He, J. J. Zhao, J. Lei and Q. Zhu, Org. Lett., 2014, 16, 2546 CrossRef CAS PubMed.
- L. S. Lu, G. Yaling Gong and Z. Demin, J. Org. Chem., 2015, 80, 9336 CrossRef PubMed.
- T. B. Xiao, L. Y. Li, G. L. Lin, Q. L. Wang, P. Zhang, Z. W. Mao and L. Zhou, Green Chem., 2014, 16, 2418 RSC.
- P. Changduo, Z. Honglin, H. Jie, C. Yixiang and Z. Chengjian, Chem. Commun., 2015, 51, 3786 RSC.
- For a recent review on applications of NHP-esters in organic synthesis see: S. Murarka, Adv. Synth. Catal., 2018, 360, 1735 CrossRef CAS.
- For applications of NHP-esters in photo induced reactions see:
(a) K. Okada, K. Okamoto, N. Morita, K. Okubo and M. Oda, J. Am. Chem. Soc., 1991, 113, 9401 CrossRef CAS;
(b) G. L. Lackner, K. W. Quasdorf and L. E. Overman, J. Am. Chem. Soc., 2013, 135, 15342 CrossRef CAS PubMed;
(c) C. Hu and Y. Chen, Org. Chem. Front., 2015, 2, 1352 RSC;
(d) L. Candish, M. Teders and F. Glorius, J. Am. Chem. Soc., 2017, 139, 7440 CrossRef CAS PubMed;
(e) A. Fawcett, J. Pradeilles, Y. Wang, T. Mutsuga, E. L. Myers and V. K. Aggarwal, Science, 2017, 357, 283 CrossRef CAS PubMed.
-
(a) Y. Cheng, C. Mück-Lichtenfeld and A. Studer, J. Am. Chem. Soc., 2018, 140, 6221 CrossRef CAS PubMed;
(b) L. Candish, M. Teders and F. Glorius, J. Am. Chem. Soc., 2017, 139, 7440 CrossRef CAS PubMed.
Footnotes |
† Electronic supplementary information (ESI) available. See DOI: 10.1039/d0ra03211e |
‡ Equally contributed to this work. |
|
This journal is © The Royal Society of Chemistry 2020 |
Click here to see how this site uses Cookies. View our privacy policy here.