DOI:
10.1039/D0RA03417G
(Paper)
RSC Adv., 2020,
10, 21174-21179
All-in-one NMR spectroscopy of small organic molecules: complete chemical shift assignment from a single NMR experiment†
Received
16th April 2020
, Accepted 29th May 2020
First published on 3rd June 2020
Abstract
A new class of NOAH NMR experiments (NOAH-AST and NOAH-ASTPS), with the abbreviations, A: 1,1-ADEQUATE, S: sensitivity improved version of multiplicity-edited (ME)-HSQC, T: TOCSY, and TPS: pure shift TOCSY, are reported to obtain complete chemical shift assignments of small organic molecules from a single NMR experiment. While NOAH-AST provides 13C–13C, 1H–13C, and 1H–1H connectivities for molecules with well resolved chemical shifts, NOAH-ASTPS experiments discern 1H–1H connectivities even in complex organic molecules such as steroids at ultra-high resolution. These methods are very flexible and allow to record data through non-uniform-sampling, which reduces the experimental time to a great extent. In order to make these methods friendly to non-NMR experts (especially organic chemists and natural product scientists), python scripts have been developed and they help researchers in using these methods.
Introduction
In nuclear magnetic resonance (NMR) spectroscopy, chemical shift assignment is the primary and foremost task. Typically, in most cases, a set of 2D experiments are recorded to obtain this chemical shift information. Recently, it has been shown that, a whole set NMR data can be acquired in a single experiment by considering (i) NOAH (NMR by ordered acquisition using 1H detection,1 including multiple-receivers)2 or (ii) MFA (multiple-FID acquisition)3 schemes. Both these methods use only one recycle delay block for all the experimental modules of the different pulse schemes combined. Therefore, experimental times are significantly reduced compared to the time taken to record the combined individual experiments, separately. In the case of NOAH pulse schemes, the unutilized magnetization of the ith experimental module is utilized in the (i+1)th experimental module. Whereas, in the MFA experiments, both the ith and (i+1)th experiments share common indirect evolution times. They both have their own inherent flexibilities in concatenating a set of experimental modules in a single experiment and several of such combinations have been proposed. ASAP versions have also been reported to minimize the relaxation artefacts in the NOAH schemes.4 Very recently our group has also reported a NOAH-based PROSMASH–HSQC2 scheme5 for monitoring the small molecule–protein interactions in a single experiment, wherein very high chemical shift resolution for the small molecule signals is achieved with the aid of real-time homonuclear broadband BIRD decoupling.6
Often, rigid ring systems are a part of many organic natural products such as steroids, terpenoids, macrocyclic antibiotics, and alkaloids. For such spin-systems, recording a 1,1-ADEQUATE7–9 experiment alone provides the complete backbone 13C–13C connectivity. The 1,1-ADEQUATE experiment filters the double-quantum 13C–13C magnetization, i.e., at the natural abundance levels of carbon, it utilizes only one active spin-pair (13C–13C) out of 10
000 spin-pairs of carbons. Hence, the similar proportion of 1H magnetization is only used for that experiment; therefore, the remaining magnetization which is not a part of the double-quantum 13C–13C spin-pair is discarded. Now, in the present work, in order to use that remaining magnetization, we have proposed a novel method, and named it as NOAH-AST. In general, along with the 1,1-ADEQUATE experiment, there is an immense need to record the multiplicity-edited (ME)-HSQC10 experiment, as it discriminates the multiplicity of CH, CH2, and CH3 carbons and the respective attached protons. Further, to verify 1H–1H spin network, we need to record either a COSY or a TOCSY11 experiment. Therefore, by combining 1,1-ADEQUATE (A), sensitivity improved version of ME-HSQC (S), and TOCSY (T) in a single experiment (AST) in a NOAH fashion, NOAH-AST delivers the complete chemical shift details of various classes of organic molecules; needless to say that sufficient concentrations are required to be able to work at natural abundance of 13C.
Previously, NOAH version of HMBC–HSQC–COSY/TOCSY1 and HMBC–H2OBC12 experiments have been reported to achieve similar purposes; however, the 1,1-ADEQUATE module in the NOAH-AST experiment provides several significant benefits when compared to the HMBC/H2OBC pulse modules: (i) in the case of HMBC there is no specificity/directionality in showing the chemical shift correlations, sometimes it shows correlations up to 4–5 bonds, whereas 1,1-ADEQUATE shows correlations only for the adjacent carbons; therefore, attributing chemical shifts for different carbon atoms is easier than in the HMBC. (ii) Interestingly, H2OBC experiment is useful to obtain specific correlations between two-bond separated C–H pairs, but it is not useful in monitoring the correlations of carbonyls with the other spins; however, such information can easily be obtained from the 1,1-ADEQUATE. (iii) Notwithstanding the benefits of HMBC, in terms of its capability in showing the correlations with quaternary carbons, it suffers from significant resolution issues along the proton dimension as each carbon correlates with several protons. In such cases, it is rather difficult to monitor the precise chemical shift correlations, especially for molecules which exhibit complex proton NMR spectra. In this regard, 1,1-ADEQUATE outperforms both the H2OBC and HMBC, since, the H2OBC does not show correlations with quaternary carbons (iv).
Steroidal molecules often exhibit reasonable 13C chemical shift resolution; thus, 1,1-ADEQUATE is a good choice over the H2OBC experiments, as the carbonyl chemical shift can be used as a starting point while analyzing the data. (v) Further, as 1,1-ADEQUATE shows relatively fewer chemical shift correlations along the 1H dimension when compared with the HMBC, it enables 1H chemical shift deconvolution which would be at least at one of the 1H sites of diastereotopic protons.
One particular concern with regard to 1,1-ADEQUATE is its low sensitivity, which may demand higher sample concentrations, in general. However, in the light of the above mentioned benefits, the low sensitivity of 1,1 ADEQUATE can be tolerated. Moreover, at the current times, the availability of high sensitivity cryogenic probes, reduces the severity of the sensitivity issue of the 1,1-ADEQUATE experiment, in practical terms. In fact, in 2015, applications of 1,1-ADEQUATE experiment have been demonstrated only with 0.7 mg of cryptospirolepine (molecular weight ∼504 g mol−1).13 This reveals that by having access to such probes, low intrinsic sensitivity of 1,1-ADEQUATE would no longer be an issue of concern, and structural studies of any synthetic organic/natural product molecules available at very small amounts can be carried out. Hence, the present NOAH-AST experiments will also take the sensitivity benefits of 1.7 mm probes in the similar way.
Often, the chemical shift resolution in the TOCSY is impaired due to the severe overlap of 1H–1H scalar multiplets; this can be circumvented by invoking ideas of homodecoupling in the TOCSY block of the NOAH-AST. Therefore, NOAH-ASTPS experiments have also been proposed; wherein TPS represents the PSYCHE pure shift TOCSY.14
Results and discussion
NOAH-AST NMR of artemisinin
Fig. 1 shows the NOAH-AST spectra recorded on the anti-malarial natural product drug, artemisinin (Fig. 1a). From the 1D projections which are added on the two-dimensional spectra, it is clear that 1H and 13C chemical shift resolution is sufficient to record the simple version of NOAH-AST experiment. The resultant 1,1-ADEQUATE, ME-HSQC, and TOCSY spectra are respectively shown in Fig. 1b, c, and d. Complete 13C assignments can be obtained from the 1,1-ADEQUATE experiment while using the carbonyl chemical shift as a starting point. For better understanding of the analysis, strip plots are shown (Fig. 1e) with appropriate labelling. Initially, the analysis has been started by considering the strip which has carbonyl carbon and it has been assigned to H9, wherein the self-correlation, i.e., H9–C9 is the negative peak and the remaining correlations are positive peaks. At this 1H chemical shift (∼3.2 ppm), two other correlations to carbons are seen; the upfield carbon chemical shift is for C15, and the other peak is for C8a. For the subsequent analysis, C8a is used as a starting point and we performed the analysis in a similar fashion. However, in order to have an unambiguous walk in the analysis process, we have to identify the multiplicity of carbons (multiplicity, –CH, CH2, and CH3), which can be obtained from the ME-HSQC module of NOAH-AST module. For example, the chemical shift of H4 proton is used to identify the C4, C5, and C3 carbon chemical shifts (Fig. 1f), and subsequently the ME-HSQC (Fig. 1g) has enabled attributing the multiplicity pattern to –CH2. This can clearly be seen from the 1D internal projections provided. Finally, the TOCSY spectrum that has been obtained from the NOAH-AST is used to establish the 1H spin system, e.g., breaks in the TOCSY correlations provide insights about the presence of quaternary carbons and hetero-atoms. A similar analysis has been performed on the alkaloid, strychnine (see ESI, Fig. S1†).
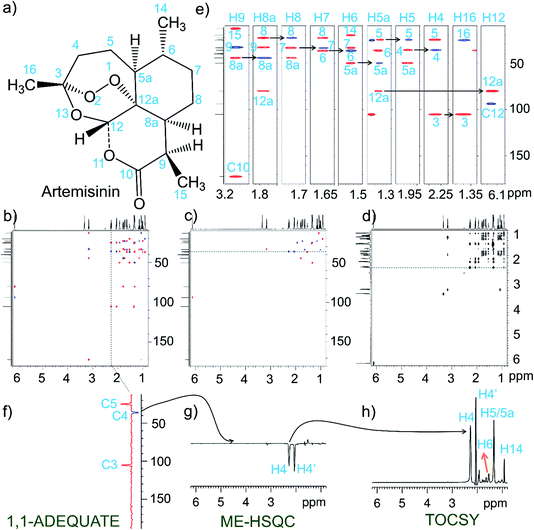 |
| Fig. 1 1,1-ADEQUATE (b, e and f), ME-HSQC (c and g), and TOCSY (d and h) spectra obtained from a single NOAH-AST recorded on artemisinin (a) molecule (∼140 mM in DMSO-D6), using an 800 MHz magnet equipped with cryoprobe. | |
NOAH-ASTPS NMR of estradiol
Next, NOAH-ASTPS experiments are demonstrated using the estradiol molecule (Fig. 2). As has been seen in the previous example, here too, whole 13C chemical shift assignments are achieved from the 1,1-ADEQUATE data set (Fig. 2b, g and f). This molecule has only one methyl group (C18) on one of the quaternary carbons (C13), which is used as a starting point for the analysis. Next, the strip at H17 is considered, as it has a hydroxyl group and C12 is bonded with C13. This facilitated identification of C16, and then proceeding in a similar fashion the 13C and 1H analysis (Fig. 2f) could be completed. However, identification of both the diastereotopic protons from the 1,1-ADEQUATE experiment is not often possible as is the case for estradiol too. At least in this kind of steroid molecule, the presence of functional groups and aromatic rings facilitates discrimination of two diastereotopic protons with different chemical shifts. Thus, the well-separated proton chemical shifts belonging to one of the diastereotopic chemical sites can be used for the spectral interpretation and it eventually provides the carbon chemical shift assignments. The remaining proton chemical shifts which have not been analyzed from the 1,1-ADEQUATE experiment can be obtained subsequently from the ME-HSQC module with multiplicity editing (Fig. 2c and h). Understanding the complete proton network connectivity is not possible from the conventional TOCSY experiment alone in steroid molecules (Fig. 2d). For example, the 1D-internal trace obtained for the H9 proton shows severe overlap in scalar-coupled multiplets that hampers the analysis (Fig. 2i). This necessitates recording the homodecoupled PSYCHE–TOCSY which results in 1H singlets that simplify proton connectivity analyses (Fig. 2e and j). However, in order to obtain such very high resolution along the indirect dimensions of pure shift TOCSY, the NOAH-ASTPS experiment had to be recorded for almost 7 hours on 50 mg of estradiol (with more indirect increments). In such cases, invoking the ideas of non-uniform-sampling (NUS)15 certainly helps in decreasing the experimental time to a significant extent. The NUS based sampling has been implemented for NOAH-ASTPS experiments, and 25% NUS sampling has taken only ∼1 hour 45 min of instrument time, yet the spectral quality of NUS–NOAH-ASTPS is comparable to that of the NOAH-ASTPS spectra recorded in the conventional manner for about ∼7 hours (see ESI, Fig. S2†). In the present case, while recording NOAH-ASTPS experiments, due to the low sensitivity of TPS module, a large number of scans are required; this is also true for the 1,1-ADEQUATE module. Since both these modules require practically a similar number of scans to get desirable signal to ratio (SNR), combining them in a single NOAH module is worthwhile.
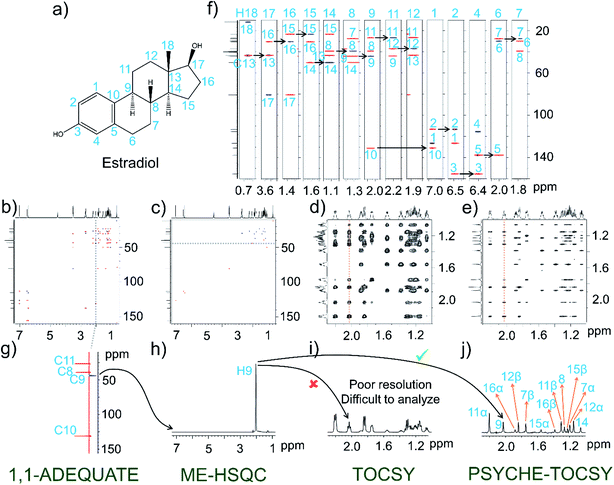 |
| Fig. 2 1,1-ADEQUATE (b, f and g), ME-HSQC (c and h), and PSYCHE–TOCSY (e and j) spectra obtained from a single NOAH-ASTPS recorded on estradiol (a) molecule (∼370 mM in DMSO-D6), using an 800 MHz magnetic field strength. On the other hand, TOCSY (d and i) spectra is recorded from the NOAH-AST experiment. | |
NOAH-AST NMR of mixture of D-glucose and D-xylose
Often, chemical constituents are present in a wide concentration range in the sample (leading to dynamic range problems in NMR). In such situations, NOAH-AST family of experiments have particular advantages. This arises from the fact that all the three experiments are recorded using only one relaxation delay time; if the three experiments were to be recorded independently, each one would require the delay time at the beginning of every scan. In the NOAH experiments, the number of scans required will be dictated by the least sensitive of the experiments; in the present case, 1,1 ADEQUATE. Table 1 shows typical time requirements for the three experiments being done in NOAH style versus being done independently with different scans, as dictated by the sensitivity of the experiments. The first row assumes that 16 scans are required for 1,1 ADEQUATE and 4 scans each are enough for ME-HSQC and TOCSY. The second row assumes 16 scans for all of them, as in the NOAH-AST experiment. It is clearly seen from the last column that NOAH-AST has better performance in terms of time with respect to independent measurements as in row 1, and additionally it would have better signal-to-noise ratio for the ME-HSQC and TOCSY experiments. Thus, even constituents present in smaller concentration would get detected at least in the more sensitive of the three experiments.
Table 1 Typical time requirements to obtain the 1,1-ADEQUATE, ME-HSQC, and TOCSY spectra in independent and NOAH-AST (mixture of D-glucose and D-xylose) fashion while using different number of scans (which are given in the parenthesis)
Experiment type |
1,1-ADEQUATE |
ME-HSQC |
TOCSY |
Total experimental time |
Independent |
1 h 30 min (16 scans) |
25 min (4 scans) |
25 min (4 scans) |
2 h 20 min |
Independent |
1 h 30 min (16 scans) |
1 h 20 min (16 scans) |
1 h 20 min (16 scans) |
4 h 10 min |
NOAH-AST |
1 h 40 min (16 scans) |
|
|
1 h 40 min |
This application is demonstrated using a mixture of D-glucose (10 mg, ∼110 mM) and D-xylose (0.5 mg, ∼6 mM) in 0.5 ml of D2O (Fig. 3). In the resultant 1,1-ADEQUATE (Fig. 3b and c) spectrum of NOAH-AST, only the resonances belonging to D-glucose are observed, since the concentration of D-xylose anomers is significantly less to detect. However, signals of D-xylose are seen in the ME-HSQC (Fig. 3d) and TOCSY spectra (Fig. 3e and f). Then, if required, one can record the 1,1-ADEQUATE spectrum only of D-xylose separately using higher concentration if possible.
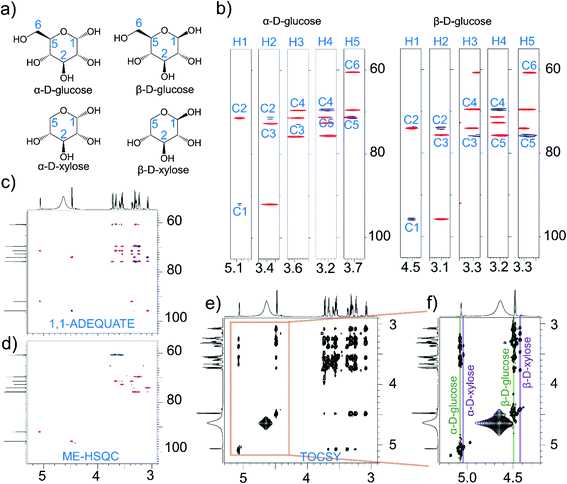 |
| Fig. 3 1,1-ADEQUATE (b and c), ME-HSQC (d), and TOCSY (e and f) spectra obtained from a single NOAH-AST recorded on a mixture of D-glucose (∼110 mM) and D-xylose (∼6 mM) molecules (a) dissolved in D2O, using an 800 MHz magnetic field strength. | |
NOAH-AST and NOAH-ASTPS NMR pulse sequences
The pulse schemes developed in the present study, NOAH-AST and NOAH-ASTPS are shown respectively in the Fig. 4a and b, as block diagrams. The full pulse schemes (Fig. S3 and S4†) and experimental details are given in the ESI.† These NOAH-AST and NOAH-ASTPS experiments require significant user intervention in optimization, which is not simple for non-NMR specialists. Hence, we wrote two python scripts (see ESI†), which take care of the entire experimental setup with minimal user intervention.
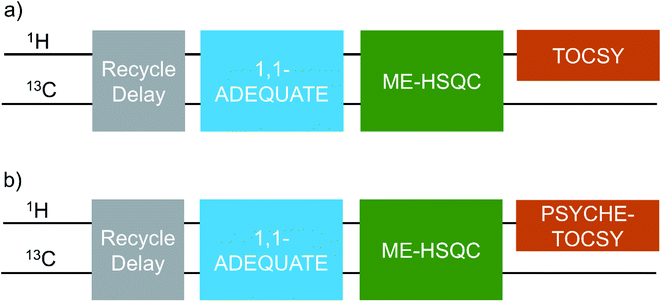 |
| Fig. 4 Schematic of NOAH-AST (a) and NOAH-ASTPS (b) pulse schemes developed in the present study. Full pulse schemes and experimental details are given in the ESI.† | |
Conclusions
Overall, the present work describes development of a new set of NOAH pulse sequences, NOAH-AST and NOAH-ASTPS. The applications of these methods are demonstrated on different kinds of organic molecules, artemisinin (terpene), strychnine (alkaloid), estradiol (steroid), and a mixture of D-glucose and D-xylose (monosaccharides). As has been demonstrated herein, complete 1H and 13C chemical shift assignments could be obtained from a single NOAH-AST experiment (13C–13C from 1,1-ADEQUATE, 1H–13C from ME-HSQC, and 1H–1H from TOCSY), which works well for the well-resolved chemical shifts. On the other hand, for molecules which exhibit complex NMR spectra, NOAH-ASTPS experiments can provide pure shift TOCSY. This NOAH-ASTPS experiment demands a larger number of indirect increments (having PSYCHE homodecoupling), which can be reduced to a significant extent by invoking a non-uniform-sampling approach. The present set of NOAH-AST/ASTPS experiments may require rather high concentrations of samples due to intrinsic low sensitivity of the 1,1-ADEQUATE experiment. However, this limitation would be overcome by having access to special probes such as cryogenically cooled 1.7 mm probe. On the other hand, if samples are available at moderate concentrations, acquiring NOAH-AST with more scans helps in obtaining the desired results in an overnight experiment, even without such special probes. Thus, the proposed NOAH methods may provide an efficient alternative to acquisition of several 2D data sets on small and complex organic molecules.
Funding sources
University Grants Commission (UGC), India (CH/17-18/0123) Raja Ramanna Fellowship, Department of Atomic Energy.
Conflicts of interest
The authors declare no competing financial interest.
Acknowledgements
VMRK gratefully acknowledge University Grants Commission (UGC), India (CH/17-18/0123) for the award of Dr D. S. Kothari Postdoctoral fellowship. RVH is a Department of Atomic Energy sponsored Raja Ramanna Fellow. Authors are also thankful to National NMR Facility, TIFR, Mumbai.
References
- Ē. Kupče and T. D. W. Claridge, Angew. Chem., Int. Ed., 2017, 56, 11779–11783 CrossRef PubMed.
- Ē. Kupče and T. D. W. Claridge, J. Magn. Reson., 2019, 307, 106568 CrossRef PubMed.
- K. Motiram-Corral, M. Pérez-Trujillo, P. Nolis and T. Parella, Chem. Commun., 2018, 54, 13507–13510 RSC.
- E. Kupče and T. D. W. Claridge, Chem. Commun., 2018, 54, 7139–7142 RSC.
- V. M. R. Kakita, K. Rachineni, M. Bopardikar and R. V. Hosur, J. Magn. Reson., 2018, 297, 108–112 CrossRef CAS PubMed.
- L. Paudel, R. W. Adams, P. Kiraly, J. A. Aguilar, M. Foroozandeh, M. J. Cliff, M. Nilsson, P. Sandor, J. P. Waltho and G. A. Morris, Angew. Chem., Int. Ed., 2013, 52, 11616–11619 CrossRef CAS PubMed.
- B. Reif, M. Koeck, R. Kerssebaum, H. Kang, W. Fenical and C. Griesinger, J. Magn. Reson., Ser. A, 1996, 118, 282–285 CrossRef CAS.
- B. Reif, M. Koeck, R. Kerssebaum, J. Schleucher and C. Griesinger, J. Magn. Reson., Ser. B, 1996, 112, 295–301 CrossRef CAS PubMed.
- M. Koeck, R. Kerssebaum and W. Bermel, Magn. Reson. Chem., 2003, 41, 65–69 CrossRef.
- R. D. Boyer, R. Johnson and K. Krishnamurthy, J. Magn. Reson., 2003, 165, 253–259 CrossRef CAS PubMed.
- J. Cavanagh and M. Rance, J. Magn. Reson., 1990, 88, 72–85 CAS.
- T. M. Nagy, T. Gyöngyösi, K. E. Kövér and O. W. Sørensen, Chem. Commun., 2019, 55, 12208–12211 RSC.
- J. Saurí, W. Bermel, A. V. Buevich, E. C. Sherer, L. A. Joyce, M. H. Sharaf, P. L. Schiff Jr, T. Parella, R. T. Williamson and G. E. Martin, Angew. Chem., Int. Ed., 2015, 54, 10160–10164 CrossRef.
- M. Foroozandeh, R. W. Adams, M. Nilsson and G. A. Morris, J. Am. Chem. Soc., 2014, 136, 11867–11869 CrossRef CAS PubMed.
- T. D. W. Claridge, M. Mayzel and Ē. Kupče, Magn. Reson. Chem., 2019, 57, 946–952 CrossRef CAS PubMed.
Footnote |
† Electronic supplementary information (ESI) available: NOAH-AST, NOAH-ASTPS NMR pulse sequences and experimental details are given. See DOI: 10.1039/d0ra03417g |
|
This journal is © The Royal Society of Chemistry 2020 |
Click here to see how this site uses Cookies. View our privacy policy here.