DOI:
10.1039/D0RA03684F
(Paper)
RSC Adv., 2020,
10, 24483-24490
New chalcone derivatives: synthesis, antiviral activity and mechanism of action†
Received
24th April 2020
, Accepted 19th June 2020
First published on 26th June 2020
Abstract
In this work, twenty-eight chalcone derivatives containing a purine (sulfur) ether moiety were synthesized and their antiviral activities were evaluated. Biological results showed that compound 5d exhibited outstanding inactive activity against tobacco mosaic virus (TMV) in vivo (EC50 = 65.8 μg mL−1), which is significantly superior to that of ribavirin (EC50 = 154.3 μg mL−1). Transmission electron microscopy indicated that compound 5d can break the integrity of TMV particles. The results of microscale thermophoresis, fluorescence titration and molecular docking showed that compound 5d had stronger combining affinity (Ka = 1.02 ×105 L mol−1, Kd = 13.4 μmol L−1) with TMV coat protein (TMV-CP), which is due to the formation of five hydrogen bonds between compound 5d and the amino-acid residues of TMV-CP. These findings revealed that compound 5d can effectively inhibit the infective ability of TMV. This work provides inspiration and reference for the discovery of new antiviral agents.
1. Introduction
As an important plant virus, tobacco mosaic virus (TMV) can cause serious plant diseases and huge economic losses to agricultural production. Statistics showed that the loss caused by TMV is up to $ 100 million worldwide each year.1 Owing to the absolute parasitic nature of plant virus to host cell and the lack of its complete immune system, and the diversity of virus transmission modes, the prevention and control of plant virus disease is still a pivotal problem in agricultural production. Few chemical agents for completely controlling the virus are currently unavailable.2 Ribavirin and Ningnanmycin as two successful antiviral agents were widely used to prevent TMV disease, but only exhibit moderate field control effects.3 Therefore, it is an extremely urgent demand to develop more novel, high-efficient and low risk agents against TMV.
Natural products are always used as models or inspiration for the discovery of new agrochemicals with broad-spectrum biological activities.4 Chalcone, a kind of natural organic compound, has typical diaryl ketone scaffold with wide range of biological activities, such as anti-inflammatory,5 antimicrobial,6,7 insecticidal,8 antiviral9–11 and so on. Purine widely spread in nature, themselves and their derivatives also have a broad spectrum of biological activities, for example, antimicrobial,12 antiviral13,14 and antibacterial.15 Furthermore, we reported some compounds containing purine and chalcone moieties. Biological results indicated that the introduction of purine (sulfur) ether and the addition of diethyl malonate or nitromethane in the double bond are beneficial to the antiviral activity of the chalcone compounds.16–18
TMV-CP plays an indispensable role in translation of mRNA, transcription of tRNA, extension, and self-assembly of TMV.19,20 It is always seen as a potential functional protein for development of antiviral drugs and exploration of the interaction of molecular–protein.21 In our previous works, we obtained some chalcone compounds with good anti-TMV activity based on TMV-CP.16,21,22
To discover new antiviral agents with highly active and continue our research on chalcone antiviral drugs. We designed and synthesized a series of chalcone derivatives containing a purine (sulfur) ether moiety (Fig. 1), and evaluated their anti-TMV activities in vivo. Then, the preliminary interaction mechanism of the target compounds was performed via transmission electron microscopy (TEM), fluorescence titration (FT), microscale thermophoresis (MST) and molecular docking.
 |
| Fig. 1 Design strategy of the title compounds. | |
2. Experimental
2.1 General synthetic procedure of the intermediates 1a–1f
Firstly, aqueous sodium hydroxide solution (5% NaOH, 5.0 mmol) was added to a round-bottomed flask containing 1-(4-hydroxyphenyl)ethan-1-one (1.0 mmol) and differently substituted aldehydes (2.0 mmol). The mixture was stirred at room temperature for 24 h. After the reaction was completed, the mixture was poured into ice water and slowly dripped 5% HCl until a large amount of yellow solids were precipitated. Finally, the mixture was filtered under vacuum, and the residue was dried to yield the intermediates 1a–1f.
2.2 General synthetic procedure of the intermediates 2a–2f
The intermediates 1a–1f (1.0 mmol) and potassium carbonate (1.5 mmol) in acetonitrile (30 mL) were stirred for 1 h, and 1,2-dibromoethane (3.0 mmol) was slowly added, the reaction mixture was refluxed at 76 °C for 8 h. After completion of the reaction, the reaction system was poured into water, extracted with ethyl acetate, the solvent was removed under depressurization, and the residue was purified by column chromatography on silica gel with a mixture of ethyl acetate/petroleum ether = 1
:
5 (v/v) to obtain the intermediates 2a–2f.
2.3 General synthetic procedure of the intermediate 3
A solution of 6-chloro-9H-purine (1.0 mmol), thiourea (1.2 mmol) in ethanol (30 mL) was added to a reaction flask, and the mixture was refluxed for 3 h. The reaction system was poured into a saturated aqueous solution of sodium hydroxide, adjust the pH acidic with glacial acetic until a large amount of yellow solid appeared. The intermediate 3 was obtained by suction filtration and desiccation.
2.4 General synthetic procedure of the target compounds 4a–4f
The intermediates 2a–2f (0.5 mmol), potassium hydroxide (2.0 mmol) in DMF (10 mL) were stirred at room temperature for 1 h, the intermediate 3 (1.0 mmol) was added and the reaction system was stirred at 40 °C for 2–6 h. After the reaction was completed, the mixture was poured into saturated salt water. The precipitated solids were washed with small amount of CH2Cl2 and filtrated. The crude product was purified by recrystallization with EtOH or column chromatography on silica gel with a mixture of ethyl acetate/petroleum ether = 6
:
1 (v/v) to yield the target compounds 4a–4f.
2.5 General synthetic procedure of the target compounds 5a–5l
A mixture of compounds 4a–4f (1.0 mmol), nitromethane (20.0 mmol) or diethyl malonate (5.0 mmol) in ethanol was stirred for 0.5 h, potassium hydroxide (1.0 mmol/1.2 mmol) was dissolved in ethanol, then added to the above mixture. The reaction system was stirred at room temperature for 2.0–4.0 h. After the reaction was completed, the reaction solvent was evaporated in vacuo, dichloromethane was added for extraction. The organic phase was dried (MgSO4), filtrated and evaporated. The residue was purified by column chromatography on silica gel with a mixture of ethyl acetate/petroleum ether = 2
:
1 (v/v) or recrystallized with EtOH to obtain the target compounds 5a–5l.
2.6 General synthetic procedure of the intermediates 6a–6b
A solution of 6-chloro-9H-purine (1.0 mmol) and potassium carbon (3.0 mmol) in DMF (10 mL) was stirred at room temperature for 1 h. Then, 1-bromopropane or 3-bromoprop-1-ene (1.1 mmol) was added slowly and the reaction was heated at 50 °C for 9 h. After the reaction was completed, the reaction system was poured into water, extracted with dichloromethane. The solvent was removed under depressurization, and the residue was purified by column chromatography on silica gel with a mixture of ethyl acetate/petroleum ether = 1
:
5 (v/v) to yield the intermediates 6a–6b.
2.7 General synthetic procedure of the target compounds 7a–7e
A solution of the intermediates 1a–1f (1.2 mmol), anhydrous potassium carbonate (3.0 mmol) in acetonitrile (30 mL) was stirred at room temperature for 1 h. Then the intermediates 6a–6b (1.0 mmol) was added, and the reaction was refluxed at 78 °C for 9–12 h. After completion of the reaction, the reaction solvent was evaporated in vacuo, extracted with dichloromethane. The organic phase was dried (MgSO4), filtrated and evaporated. The target compounds 7a–7e were obtained by column chromatography on silica gel with a mixture of ethyl acetate/petroleum ether = 1
:
3 (v/v).
2.8 General synthetic procedure of the target compounds 8a–8e
A mixture of compounds 7a–7e (1.0 mmol) and diethyl malonate (5.0 mmol) in ethanol was stirred for 30 min. Then, potassium hydroxide (1.0 mmol) was dissolved in ethanol, and added into the above mixture. The reaction system was stirred at room temperature for 2.0–4.0 h. After the reaction was completed, the reaction solvent was evaporated in vacuo, dichloromethane was added for extraction. Then the organic phase was dried (MgSO4), filtrated and evaporated. The residue was purified by column chromatography on silica gel with a mixture of ethyl acetate/petroleum ether = 2
:
1 (v/v) or recrystallized with EtOH to obtain the target compounds 8a–8e.
3. Results and discussion
3.1 Synthesis
As shown in Schemes 1 and 2, firstly, the intermediates 1a–1f, 2a–2f, 3 and 6a–6b were obtained according to previously reported methods.23,24 Then, the target compounds 4a–4f and 7a–7e were synthesized by removing the halogen hydride.17,24 Lastly, the target compounds 5a–5l and 8a–8e were obtained by Michael addition reaction.23,24 In order to improve the yield of the target compounds, the reaction solvent, temperature and catalyst were optimized using the reaction of compound 5d as the template. The molar ratio of 4b: diethyl malonate: catalyst is 1.0
:
5.0
:
1.0, and reaction time is 4.0 h. The results showed that the reaction solvent has no significant effect on the yield, but the catalyst and temperature have great influence on it. When the reaction temperature was 23 °C (room temperature), with KOH as catalyst and EtOH as solvent, the yield of the compounds is highest. A good yield also can be obtained by appropriately raising the reaction temperature (50 °C). In addition, increasing the temperature to reflux will obviously decrease the yield (Table 1).
Table 1 Optimization of the reaction conditions for compound 5d
No. |
Solvent |
Catalyst |
Temperature (°C) |
Yielda (%) |
The crude yield of the reaction. |
1 |
EtOH |
Et3N |
23 |
51 |
2 |
EtOH |
K2CO3 |
23 |
35 |
3 |
EtOH |
KOH |
23 |
92 |
4 |
MeOH |
Et3N |
23 |
48 |
5 |
MeOH |
K2CO3 |
23 |
42 |
6 |
MeOH |
KOH |
23 |
85 |
7 |
EtOH |
KOH |
50 |
91 |
8 |
EtOH |
KOH |
76 |
60 |
9 |
MeOH |
KOH |
50 |
80 |
10 |
MeOH |
KOH |
76 |
64 |
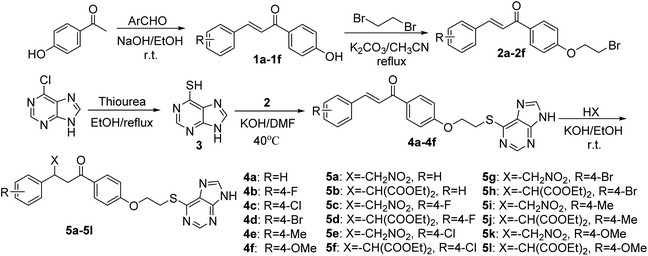 |
| Scheme 1 The synthetic route of the target compounds 4a–4f and 5a–5l. | |
 |
| Scheme 2 The synthetic route of the target compounds 7a–7e and 8a–8e. | |
3.2 Spectral properties
1H-NMR, 13C-NMR and HRMS spectra of all the target compounds are provided in the ESI.† For example, the analysis of the spectral data of 5d is given below. In the 1H NMR spectrum, 3H in the δ 13.58 ppm, δ 8.73 ppm and 8.47 ppm, can be attributed to the purine, whereas 8H appearing in the δ 7.86–6.98 ppm can be attributed to the benzene ring. Furthermore, 7H of δ 4.36 ppm, δ 4.21–4.07 ppm, 3.96 ppm and δ 3.59 ppm, can be attributed to the presence of –OCH2–, –CO–CH2–, –SCH2– and Ph–CH– groups. Besides, 11H of δ 3.88–3.72 ppm, 3.28 ppm and 1.15 ppm can be attributed to the –CH–(COOEt)2 group. In the HRMS spectrum of the target compounds, the characteristic absorption signals of [M + Na]+ ions were obtained, which were consistent with their molecular weights.
3.3 Antiviral activity
The purification of TMV, and anti-TMV activities in vivo of the target compounds were performed by the methods reported in the literature.25,26 The implementation details of bioassay methods are showed in the ESI.†
As shown in Table 2, some of the target compounds exhibited good anti-TMV activities in vivo. Of which, the curative effects of compounds 5c, 5f, 5j, 7a and 8b are 47.0%, 47.2%, 47.8%, 51.1% and 48.8%, respectively, which are better than that of ribavirin (35.7%). The protective effects of compounds 5f, 7c and 8d are 61.0%, 55.2% and 56.3%, respectively, similar to that of ribavirin (54.7%). Meanwhile, the compounds 5d, 5f, 5h, 8a, 8b and 8c have good inactive activity with the values of 89.5%, 80.9%, 84.0%, 81.7%, 82.7% and 87.4%, respectively, superior to the value of ribavirin (69.8%). The further anti-TMV activity results (Table 3, Fig. 2) showed that compounds 5d, 5h and 8c possessed excellent inactive activity with the EC50 values of 65.8, 85.5 and 69.2 μg mL−1, respectively, which are significantly superior to that of ribavirin (EC50 = 154.3 μg mL−1).
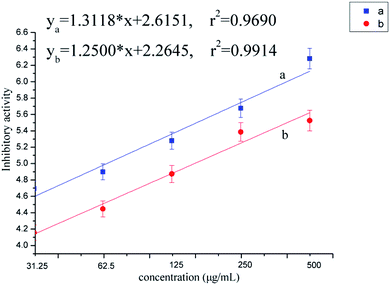 |
| Fig. 2 The EC50 curve of the compound 5d (a) and ribavirin (b), inhibitory activity = 5 + NORMSINV(inhibition ratio). | |
Table 2 The antiviral activity of the target compounds against TMV at 500 μg mL−1 in vivo (%)a
Compd |
Curative activity |
Protective activity |
Inactive activity |
Average of three replicates. A commercial agricultural antiviral agent ribavirin as the positive control. |
4a |
29.2 ± 5.5 |
37.6 ± 2.4 |
40.2 ± 5.2 |
4b |
29.0 ± 5.3 |
40.6 ± 2.8 |
40.6 ± 2.8 |
4c |
32.0 ± 2.1 |
40.6 ± 1.3 |
51.5 ± 0.9 |
4d |
41.0 ± 1.3 |
28.1 ± 0.9 |
54.1 ± 3.2 |
4e |
32.8 ± 2.4 |
15.8 ± 3.7 |
47.0 ± 2.0 |
4f |
35.2 ± 2.0 |
42.0 ± 2.9 |
54.3 ± 3.9 |
5a |
34.9 ± 2.0 |
47.7 ± 1.8 |
52.2 ± 2.0 |
5b |
45.4 ± 2.3 |
39.0 ± 2.1 |
43.3 ± 1.9 |
5c |
47.0 ± 2.1 |
34.2 ± 2.5 |
62.7 ± 2.8 |
5d |
41.3 ± 1.3 |
47.6 ± 3.3 |
89.5 ± 1.9 |
5e |
29.6 ± 1.2 |
32.0 ± 1.3 |
56.0 ± 2.3 |
5f |
47.2 ± 2.4 |
61.0 ± 0.4 |
80.9 ± 0.5 |
5g |
43.3 ± 2.8 |
27.0 ± 2.5 |
55.8 ± 2.3 |
5h |
39.2 ± 3.4 |
47.9 ± 2.0 |
84.0 ± 1.9 |
5i |
39.0 ± 1.0 |
41.0 ± 1.8 |
47.3 ± 2.8 |
5j |
47.8 ± 2.5 |
37.8 ± 1.2 |
51.0 ± 1.9 |
5k |
30.0 ± 1.1 |
44.0 ± 3.5 |
35.5 ± 2.2 |
5l |
32.5 ± 0.4 |
40.9 ± 2.6 |
50.3 ± 1.0 |
7a |
51.1 ± 8.5 |
38.6 ± 8.3 |
65.9 ± 2.1 |
7b |
39.1 ± 5.2 |
41.0 ± 4.6 |
75.3 ± 2.4 |
7c |
38.4 ± 6.3 |
55.2 ± 1.6 |
60.8 ± 4.1 |
7d |
36.3 ± 4.4 |
40.4 ± 2.5 |
67.4 ± 3.5 |
7e |
30.8 ± 1.6 |
47.0 ± 5.1 |
66.2 ± 2.7 |
8a |
45.0 ± 6.3 |
46.4 ± 2.6 |
81.7 ± 1.5 |
8b |
48.8 ± 2.4 |
39.7 ± 2.1 |
82.7 ± 1.6 |
8c |
44.0 ± 6.0 |
38.6 ± 0.2 |
87.4 ± 6.9 |
8d |
41.8 ± 1.7 |
56.3 ± 2.1 |
72.4 ± 2.3 |
8e |
38.4 ± 6.3 |
40.0 ± 3.5 |
74.2 ± 0.7 |
Ribavirinb |
35.7 ± 4.9 |
54.7 ± 1.7 |
69.8 ± 1.3 |
Table 3 The EC50 values of inactive activity of the addition compounds against TMV in vivoa
Compd |
EC50 (μg mL−1) |
Regression equation |
r2 |
Average of three replicates. A commercial antiviral agent ribavirin as the positive control. |
5a |
312.9 ± 3.0 |
y = 1.2640x + 1.8458 |
0.9718 |
5b |
509.3 ± 4.0 |
y = 1.2851x + 1.5212 |
0.9619 |
5c |
341.3 ± 3.0 |
y = 1.3394x + 1.6071 |
0.9940 |
5d |
65.8 ± 2.9 |
y = 1.3118x + 2.6151 |
0.9690 |
5e |
337.4 ± 5.0 |
y = 1.2495x + 1.8411 |
0.9659 |
5f |
138.2 ± 3.4 |
y = 1.2045x + 2.4217 |
0.9299 |
5g |
190.2 ± 2.0 |
y = 1.0730x + 2.5545 |
0.9834 |
5h |
85.5 ± 1.1 |
y = 1.1354x + 2.7897 |
0.9553 |
5i |
372.5 ± 4.4 |
y = 1.4516x + 1.2678 |
0.9332 |
5j |
238.5 ± 5.1 |
y = 1.3185x + 1.8651 |
0.9039 |
5k |
591.1 ± 6.0 |
y = 1.2019x + 1.6687 |
0.9979 |
5l |
572.4 ± 6.4 |
y = 1.3389x + 1.3077 |
0.9618 |
8a |
127.9 ± 2.9 |
y = 1.2447x + 2.3777 |
0.9516 |
8b |
231.4 ± 3.5 |
y = 1.8949x + 0.5196 |
0.9417 |
8c |
69.2 ± 4.0 |
y = 1.1626x + 2.8607 |
0.9515 |
8d |
199.2 ± 5.1 |
y = 1.4583x + 1.6469 |
0.9839 |
8e |
267.3 ± 5.9 |
y = 1.5904x + 1.1400 |
0.9393 |
Ribavirinb |
154.3 ± 2.1 |
y = 1.2500x + 2.2645 |
0.9914 |
3.4 Structure–activity relationships (SARs) of antiviral activities
The structure–activity relationships (SARs) showed that the addition compounds exhibited better antiviral activity than that of the corresponding precursor compounds, such as 5a > 4a, 5b > 4b, 8a > 7a, 8b > 7b, and so on. Among compounds 5, electron-withdrawing groups of R can enhance the inactive activity, for example, 5c, 5e, 5g > 5i, 5k; 5d, 5f, 5h > 5j, 5l. Meanwhile, all of the others groups of R except H, the addition products with diethyl malonate have better inactive activity than the corresponding addition products with nitromethane, for instance, 5d > 5c, 5f > 5e, 5h > 5g, 5j > 5i, and 5l > 5k. Among compounds 8, the propyl substituent compounds showed the higher inactive activity than the corresponding allyl substituent compounds, such as 8a > 8d, and 8b > 8e. In addition, ethyoxyl is favor to activity, such as 5d > 8a, 8d; 5f > 8b, 8e.
3.5 TEM study
The morphology of TMV particles after treatment with compounds was observed by TEM.27 The normal morphology of TMV particles (CK) was nearly complete rod-like structures (Fig. 3A). However, compared to the positive control (ribavirin, Fig. 3B), compound 5d caused TMV particles had significant rupture and damage. The results revealed that compound 5d can severely destroy the integrity of TMV particles (Fig. 3C), and probably affect the infectivity of TMV.
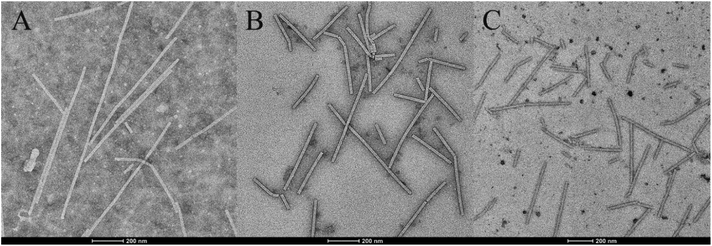 |
| Fig. 3 The morphology changes of TMV particles treated with the compounds, CK (A), ribavirin + TMV (B) and 5d + TMV (C). | |
3.6 FT and MST
The binding affinities of 5d, 5g, 5k, and ribavirin with TMV-CP were obtained via MST and FT.28,29 The details of assay methods are showed in the ESI.† As shown in Fig. 4, the association constant (Ka) between 5d and TMV-CP was 1.02 × 105 L mol−1. The binding affinity of the compound 5d with TMV-CP is stronger than that between ribavirin (6.92 × 103 L mol−1), 5g (1.62 × 103 L mol−1), 5k (2.24 × 102 L mol−1) and TMV-CP. Then, the dissociation constant (Kd) of the interaction between compounds 5d, 5g, 5k and ribavirin was measured by MST, with the values of 13.4, 33.2, 215.1 and 119.8 μM (Fig. 5). It suggested that the compound 5d exhibited stronger combining capacity. These binding constants are consistent with the trend of inactive activity of the target compounds against TMV.
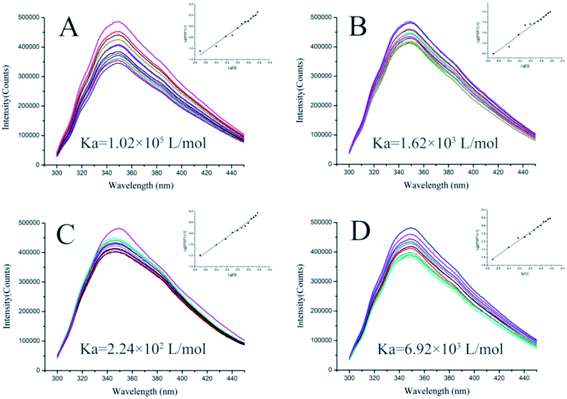 |
| Fig. 4 The FT measurement results of compounds 5d (A), 5g (B), 5k (C) and ribavirin (D) to TMV-CP. | |
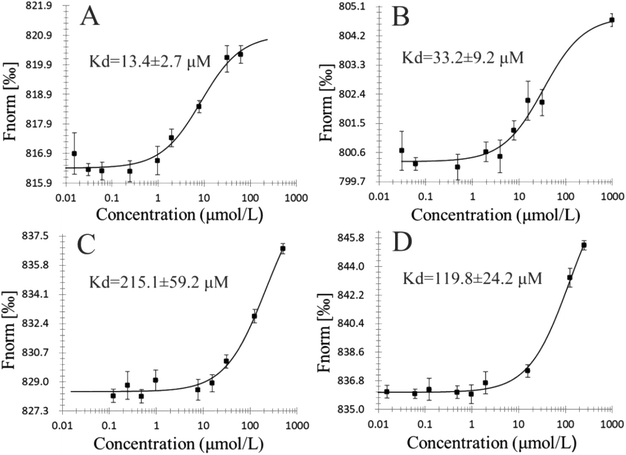 |
| Fig. 5 The MST measurement results of compounds 5d (A), 5g (B), 5k (C) and ribavirin (D) to TMV-CP. | |
3.7 Molecular docking
Molecular docking was performed to further investigate the binding modes of the compounds and TMV-CP (PDB code: 1EI7) by Discovery Studio 4.5.30,31 As shown in Fig. 6, the compound 5d formed five conventional hydrogen bonds with different amino-acid residues of TMV-CP, including C
O⋯TYR139, 2.38 Å; C
O⋯ASN73, 2.21 Å; C
O⋯GLN257, 3.08 Å; NH⋯TRP217, 1.39 Å and NH⋯ASP219, 2.34 Å. Compound 5g formed two hydrogen bonds with the amino-acid residues of TMV-CP, they are NH⋯ARG134 (2.58 Å) and NH⋯TRP217 (1.88 Å). Compound 5k formed two hydrogen bonds with the amino-acid residues of TMV-CP, they are NH⋯SER147 (3.06 Å) and NH⋯ARG261 (2.46 Å). Finally, ribavirin formed three conventional hydrogen bonds with the amino-acid residues GLU222 (4.54 Å), ASP219 (3.93 Å), and ASP266 (5.50 Å) of TMV-CP. Moreover, we can learn from relevant research that the hydrogen bonds are beneficial to the stability of the drug–protein complexes.32 Noteworthy, the compounds 5d, 5g, 5k and ribavirin all formed the pi-alkyl with the amino-acid residue LYS253 of TMV-CP, which may be a key binding site of the interaction between the compounds and TMV-CP. In addition, Libdock scores of the compounds 5d, 5g, 5k and ribavirin are 143.05, 136.24, 134.11 and 105.15, respectively. The binding energy is 21.21, 36.03, 39.92 and 31.42 kcal mol−1, which the low binding energy is more conducive to the binding of the receptor and ligand.33 The above results manifested that the interaction between the compound 5d and TMV-CP is stronger, and the complex formed by them was more stable. The interaction of the molecule-protein probably hindered the interaction between two subunits of TMV-CP, which play a negative role in the translation of mRNA, transcription of tRNA, extension, and self-assembly of TMV. It may be attributed to the inactive activity of the compounds against TMV.
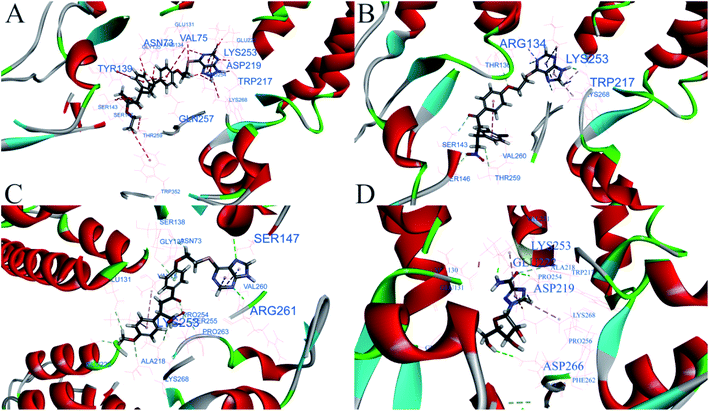 |
| Fig. 6 The binding models of the compounds 5d (A), 5g (B), 5k (C) and ribavirin (D) to TMV-CP. | |
4. Conclusions
In summary, twenty-eight chalcone derivatives containing a purine (sulfur) ether moiety were obtained, and the structures of the target compounds were characterized by NMR and HRMS. Bioassay results revealed that some target compounds displayed desirable antiviral activities against TMV in vivo. Of which, compound 5d exhibited outstanding inactive activity against TMV with the EC50 value of 65.8 μg mL−1. The preliminary SARs manifested that the addition compounds with diethyl malonate exhibited better inactive activity. Furthermore, the preliminary antiviral mechanism was conducted. The results of TEM showed that compound 5d can destroy the integrity of TMV particles, and probably affected the infectivity of TMV. The FT, MST and molecular docking results revealed that compound 5d had stronger combining capacity with TMV-CP (Ka = 1.02 ×105 L mol−1, Kd = 13.4 μM), due to the formation of strong hydrogen bonding with the active sites of amino-acid residues of TMV-CP and the lower binding energy. These results suggested that the antiviral activity of the compound 5d may depend on its stronger binding affinity with TMV-CP. This work provides beneficial reference for the design and development of new anti-TMV agents based on natural products.
Conflicts of interest
The authors declare that they have no competing interests.
Acknowledgements
We gratefully acknowledge the National Natural Science Foundation of China (21562013), the Innovative Talents Project of Guizhou Province in China (No. 201503) and the Construction Project of Key Laboratories from the Education Department of Guizhou Province (QJHKY [2018] 001).
References
- L. Bos, Trends Microbiol., 2000, 8, 82–87 CrossRef CAS.
- Z. W. Wang, P. Wei, L. Z. Wang and Q. M. Wang, J. Agric. Food Chem., 2012, 60, 10212–10219 CrossRef CAS PubMed.
- B. Liu, R. Li, Y. N. Li, S. Y. Li, J. Yu, B. F. Zhao, A. C. Liao, Y. Wang, Z. W. Wang, A. D. Yu, Y. X. Liu and Q. M. Wang, J. Agric. Food Chem., 2019, 67, 1795–1806 CrossRef CAS PubMed.
- T. C. Sparks, D. R. Hahn and N. V. Garizi, Pest Manag. Sci., 2017, 73, 700–715 CrossRef CAS PubMed.
- Z. Y. Fu, Q. H. Jin, Y. L. Qu and L. P. Guan, Bioorg. Med. Chem. Lett., 2019, 29, 1909–1912 CrossRef CAS PubMed.
- P. L. Zhao, C. L. Liu, W. Huang, Y. Z. Wang and G. F. Yang, J. Agric. Food Chem., 2007, 55, 5697–5700 CrossRef CAS PubMed.
- T. Guo, R. J. Xia, M. Chen, J. He, S. J. Su, L. W. Liu, X. Y. Li and W. Xue, RSC Adv., 2019, 9, 24942–24950 RSC.
- Y. K. Yan, Q. Xu, Y. Guo, H. Liu and X. L. Tang, Chin. J. Org. Chem., 2018, 38, 1763–1771 CrossRef CAS.
- X. H. Gan, D. Y. Hu, Y. J. Wang, L. Yu and B. A. Song, J. Agric. Food Chem., 2017, 65, 4367–4377 CrossRef CAS PubMed.
- X. Tang, S. J. Su, M. Chen, J. He, R. J. Xia, T. Guo, Y. Chen, C. Zhang, J. Wang and W. Xue, RSC Adv., 2019, 9, 6011–6020 RSC.
- Z. Z. Wang, D. D. Xie, X. H. Gan, S. Zeng, A. W. Zhang, L. M. Yin, B. A. Song, L. H. Jin and D. Y. Hu, Bioorg. Med. Chem. Lett., 2017, 27, 4096–4100 CrossRef CAS PubMed.
- G. Dilek Celik, A. Disli, Y. Oner and L. Acik, Med. Chem. Res., 2013, 22, 1470–1479 CrossRef CAS.
- M. M. Wang, P. Srivastava, C. Liu, R. Snoeck, G. Andrei, S. De Jonghe and P. Herdewijn, Eur. J. Med. Chem., 2018, 150, 616–625 CrossRef CAS PubMed.
- F. C. He, J. Shi, Y. J. Wang, S. B. Wang, J. X. Chen, X. H. Gan, B. A. Song and D. Y. Hu, J. Agric. Food Chem., 2019, 67, 8459–8467 CrossRef CAS PubMed.
- W. N. Wu, M. N. Gao, H. Tu and G. P. Ouyang, J. Heterocycl. Chem., 2016, 53, 2042–2048 CrossRef CAS.
- X. H. Gan, Y. J. Wang, D. Y. Hu and B. A. Song, Chin. J. Chem., 2017, 35, 665–672 CrossRef CAS.
- Y. J. Wang, D. G. Zhou, F. C. He, J. X. Chen, Y. Z. Chen, X. H. Gan, D. Y. Hu and B. A. Song, Chin. Chem. Lett., 2018, 29, 127–130 CrossRef CAS.
- Z. H. Wan, D. Y. Hu, P. Li, D. D. Xie and X. H. Gan, Molecules, 2015, 20, 11861–11874 CrossRef CAS PubMed.
- S. Asurmendi, R. H. Berg, J. C. Koo and R. N. Beachy, Proc. Natl. Acad. Sci. U.S.A., 2004, 101, 1415–1420 CrossRef CAS PubMed.
- X. Y. Li, B. A. Song, X. Chen, Z. C. Wang, M. J. Zeng, D. D. Yu, D. Y. Hu, Z. Chen, L. H. Jin, S. Yang, C. G. Yang and B. E. Chen, PloS One, 2013, 8, e77717 CrossRef CAS PubMed.
- X. H. Gan, D. Y. Hu, Z. Chen, Y. J. Wang and B. A. Song, Bioorg. Med. Chem. Lett., 2017, 27, 4298–4301 CrossRef CAS PubMed.
- D. G. Zhou, D. D. Xie, F. C. He, B. A. Song and D. Y. Hu, Bioorg. Med. Chem. Lett., 2018, 28, 2091–2097 CrossRef CAS PubMed.
- L. R. Dong, D. Y. Hu, Z. X. Wu, J. X. Chen and B. A. Song, Chin. Chem. Lett., 2017, 28, 1566–1570 CrossRef CAS.
- M. H. Chen, P. Li, D. Y. Hu, S. Zeng, T. X. Li, L. H. Jin, W. Xue and B. A. Song, Bioorg. Med. Chem. Lett., 2016, 26, 168–173 CrossRef CAS PubMed.
- G. V. Gooding and T. T. Hebert, Phytopathology, 1967, 57, 1285–1287 Search PubMed.
- B. A. Song, H. P. Zhang, H. Wang, S. Yang, L. H. Jin, D. Y. Hu, L. L. Pang and W. Xue, J. Agric. Food Chem., 2005, 53, 7886–7891 CrossRef CAS PubMed.
- Y. J. Wang, J. Zhang, F. C. He, X. H. Gan, B. A. Song and D. Y. Hu, Bioorg. Med. Chem. Lett., 2019, 29, 2218–2223 CrossRef CAS PubMed.
- X. Y. Li, Z. Chen, L. H. Jin, D. Y. Hu and S. Yang, Int. J. Mol. Sci., 2016, 17, 252 CrossRef PubMed.
- M. Jerabek-Willemsen, C. J. Wienken, D. Braun, P. Baaske and S. Duhr, Assay Drug Dev. Technol., 2011, 9, 342–353 CrossRef CAS PubMed.
- L. R. F. De Sousa, H. M. Wu, L. Nebo, J. B. Fernandes, M. F. D. G. F. Da Silva, W. Kiefer, M. Kanitz, J. Bodem, W. E. Diederich, T. Schirmeister and P. C. Vieira, Bioorg. Med. Chem., 2015, 23, 466–470 CrossRef CAS PubMed.
- A. Klug, Philos. Trans. R. Soc., B, 1999, 354, 531–535 CrossRef CAS PubMed.
- J. H. Jiang and P. Deng, Int. J. Mol. Sci., 2019, 20, 4090 CrossRef CAS PubMed.
- Y. H. Tseng, P. H. Chuang, Y. R. Huang and C. L. Chen, Int. J. Mol. Sci., 2017, 18, 144 CrossRef PubMed.
Footnote |
† Electronic supplementary information (ESI) available. See DOI: 10.1039/d0ra03684f |
|
This journal is © The Royal Society of Chemistry 2020 |
Click here to see how this site uses Cookies. View our privacy policy here.