DOI:
10.1039/D0RA04101G
(Paper)
RSC Adv., 2020,
10, 35941-35948
The fabrication of a covalent triazine-based organic framework for the solid-phase extraction of fourteen kinds of sulfonamides from meat samples†
Received
7th May 2020
, Accepted 15th September 2020
First published on 30th September 2020
Abstract
A novel covalent triazine-based organic framework (CTF), SCAU-2, was fabricated and used as an adsorbent for the solid-phase extraction of fourteen kinds of sulfonamides (SAs) from meat. Another CTF, SCAU-1, was adopted as a comparison material, as it has a similar motif. A series of structural characterization steps was carried out on the synthesized materials and several parameters were investigated during the extraction process, including the amount of adsorbent, the dilution ratio, the pH of the sample, and the washing and elution solvents. After detection with UHPLC-Q/TOF-MS/MS, the results revealed that SCAU-2 showed high extraction efficiencies towards the selected SAs. The LOD values are from 0.05 to 0.54 ng g−1, and the recoveries are from 84.1% to 91.9%, with RSDs ranging from 3.2% to 4.8% for SCAU-2 when spiked at 50 ng g−1. The results demonstrated that the proposed method has good applicability for the determination of SAs in complicated samples.
Introduction
Sulfonamides (SAs), which are synthetic antimicrobials, have been widely used for the prevention and treatment of animal diseases due to their low costs, high efficiencies, and broad-spectrum antimicrobial activities.1,2 Generally, humans will inevitably consume a certain amount of these drugs from meat if they are overused. An excessive SA content may result in allergic reactions, poisoning, and even potentially carcinogenic effects.3,4 The European Union has established a maximum SA reside limit of 100 mg kg−1 in food products. Therefore, it is important to establish a simple, sensitive, and reliable method to separate and detect SA residues in animal-origin food, especially meat.
Owing to the low levels of SAs and complicated matrices of food samples, the concentration and purification of analytes are usually required prior to detection. Among the methods for sample pretreatment, solid-phase extraction (SPE) has been widely used as a simple and efficient technique. The SPE adsorbent is a key factor affecting the extraction efficiency, and many kinds of porous materials have been studied for this purpose, such as graphene and other carbon-based materials, molecularly imprinted polymers, modified silicone, magnetic sorbents, etc.5–8 However, SAs differ greatly in hydrophilicity and size and, thus, high recoveries are difficult to achieve for various types simultaneously. Therefore, there is considerable interest in developing new selective sorbents for extracting and isolating SAs from complicated matrices.
Covalent organic frameworks (COFs) have attracted much attention in the porous materials field recently, and they are constructed via strong covalent bonds between light elements, typically C, N, O, and/or B.9,10 They not only have regular porous structures and ordered spatial arrangements, but their structures, types and cavity polarities can also be adjusted through rational chemical design and synthesis.11,12 Due to their good thermal stabilities, large surface areas, and low densities, they have diverse potential applications, including in catalysis,13 gas storage,14,15 and the adsorption of contaminants.16 Several reports have also involved the exploration of COFs as SPE adsorbents or SPME layers;17–19 of these, covalent triazine-based organic frameworks (CTFs) are particularly interesting because these COFs are constructed from triazine with high nitrogen content, which can be prepared economically using easily available starting chemicals.20–23 However, to enrich the types of available CTFs and widen their applications, the design of functional monomers is still needed. The adoption of monomers with multi-heterocyclic rings is an effective method, which may not only increase the π–π interactions but also provide more hydrogen-bond donors. To our knowledge, such a strategy has rarely been reported for the synthesis of COFs. Moreover, further research is still needed to ascertain the recognition mechanism between analytes and the functional groups of the material.
Herein, a novel porous CTF, SCAU-2, was fabricated via the condensation of an aldehyde and amine, adopting 2,2′-bithiophene-5,5′-dicarboxaldehyde as the dialdehyde and melamine as the amine source. Another reported CTF, SCAU-1, was also studied as a comparison material.24 After being characterized via infrared spectroscopy (IR), X-ray powder diffraction (XRPD), thermogravimetric analysis (TGA), scanning electron microscopy (SEM), and N2 adsorption, the obtained material was used to purify and concentrate fourteen SAs from meat. The extraction process was optimized, and the results were determined via UHPLC-Q/TOF-MS/MS. The extraction efficiencies of the two CTFs were evaluated under optimized conditions, and three kinds of real meat samples (pork, chicken, and beef) were also tested to validate the feasibility of the established method.
Experimental
Reagents and materials
The chemical reagents employed were all commercially available. Mass spectrum pure-grade methanol (MeOH), acetonitrile (ACN), formic acid (FA), and water were used as the mobile phase for LC-MS and purchased from Merck (Shanghai, China). Analytical grade solvents, like dichloromethane and ethanol, were purchased from Guangzhou Chemical Regent Factory (Guangzhou, China). All other reagents were purchased from Aladin (Shanghai, China). Empty SPE columns (3 mL) were bought from Bona-Angel (Tianjin, China). Meat samples were bought from the local Changban market (Guangzhou, China).
Fourteen SA standards, sulfadoxine (SDX), sulfamethizole (SMT), sulfaphenazole (SPZ), sulfachloropyridazine (SCP), sulfamonomethoxine (SMM), sulfadimidine (SDM), sulfathiazole (STZ), sulfisoxazole (SXZ), sulfamethoxypyridazine (SMP), sulfameter (SM), sulfamerazine (SMZ), sulfapyridine (SPD), sulfamethoxazole (SMX), and sulfadimethoxine (SDT), were purchased from Sigma-Aldrich (Saint Louis, USA). Stock solutions of the standards were prepared at 10 mg L−1 in MeOH/water (1
:
1, v/v) and stored at 4 °C in darkness. Five standard solutions were prepared via the dilution of stock solutions with MeOH/water (1
:
4, v/v) at concentrations ranging from 25–1000 μg L−1. SPZ-D6 was prepared at 1 μg mL−1 with MeOH/water (1
:
4, v/v) and used as an internal standard. 100 μL of standard solutions of different concentrations (25, 100, 200, 500, and 1000 μg L−1) and 10 μL of SPZ-D6 solution were added to 1 g blank meat samples before treatment. The study of each concentration was repeated three times to research the linearity. For validation studies, three different spike levels of 10, 50, and 100 ng g−1 were tested with six replicates during a day and repeated for five successive days. The LOD values were determined according to a linearity calculation process, in which the spiked level corresponds to the instrument LOD (S/N = 3).
Instruments
X-ray powder diffraction (XRPD) patterns were recorded using a Rigaku-D/max 2500 V X-ray diffractometer equipped with a source of Cu-Kα radiation (λ = 1.54178 Å) (Rigaku, Tokyo, Japan). Scanning electron micrographs (SEM) were obtained using a Sigma-300 scanning electron microscope (Zeiss, Jena, Germany). Infrared spectroscopy (IR) and thermogravimetric analysis (TGA) were carried out using an EQUINOX 55 spectrometer (Bruker, Germany) and STA449C apparatus (NETZSCH, Selb, Germany), respectively. The BET surface areas and pore distributions were tested based on N2 adsorption using GEMINI VII 2390 apparatus (Micromeritics, Georgia, USA) at 77 K. Before BET testing, the powder was degassed at 200 °C for 8 h. The sample extracts were flowed through SPE columns using a vacuum pump (Yiheng, Shanghai, China). The chromatographic separation and detection of SAs were carried out using a Waters Acquity UHPLC system coupled to a Waters Xevo G2 QTOF mass spectrometer (Waters, USA) equipped with an ACQUITY UHPLC® BEH C18 column (2.1 mm × 50 mm, 1.7 μm).
Preparation of SCAU-2
The synthesis of SCAU-1 proceeded according to the literature,24 and SCAU-2 was prepared with slight modifications. 0.345 g of melamine and 0.83 g of 2,2′-bithiophene-5,5′-dicarboxaldehyde were mixed in DMSO (32 mL) and under ultrasound for 15 min, then poured into a Teflon-lined stainless-steel vessel (50 mL). The vessel was heated to 180 °C and held for 10 h. After cooling to room temperature gradually, 0.76 g of red-orange powder was filtered out (yield: 65%). The obtained powder was rinsed with dichloromethane and ethanol, respectively, and dried at 150 °C for 2 h in a vacuum oven.
SPE procedure and LC conditions
Three kinds of meat samples (pork, chicken, and beef) were all bought from a local market. 1 g of meat sample was cut into pieces and homogenated for 2 min, then 3 mL of ACN with 0.2% acetic acid was added. After extraction under ultrasound for 20 min, the sample was centrifuged, and the supernatant was collected. This process was carried out twice. The extracts were merged and concentrated to 1.5 mL under a N2 atmosphere, then diluted before sampling.
Upper and lower sieve plates were used to avoid the release of powder. Initially, the SPE column with 40 mg of CTF was rinsed with 2 mL of MeOH and 2 mL of water, respectively. The extract was diluted to 10 mL using water, then poured into the column, with outflow under vacuum at a flow rate of 3 mL min−1. After washing with 1 mL of water with 10% MeOH, the analytes were eluted with 1.5 mL of MeOH with 5% NH3 at an elution rate of 3 mL min−1. The elute was blown dry under a N2 atmosphere. 0.5 mL of the initial mobile phase was used to dissolve the extract before determination.
For chromatographic analysis, the column temperature was set at 40 °C and the flow rate was 0.3 mL min−1. The volume of the sample injected was 2 μL. Mobile phase A was water and 0.1% formic acid, while phase B was acetonitrile and 0.1% formic acid. The elution gradient was as follows: 0–4 min, 90% A; 4.1–6 min, 90% A varied to 60% A; 6.1–8 min, 100% B; 8.1–10 min, 90% A. Mass spectrometry analysis of the compounds was performed in positive ion and multiple reaction monitoring (Tof MRM) mode via electrospray ionization. The detailed parameters were as follows: capillary voltage, 3000 V; desolvation gas temperature and flow rate, 350 °C and 650 L h−1; source temperature, 120 °C; gas flow of the cone, 600 L h−1.
Results and discussion
Characterization of the prepared SCAU-2
The structures of the fourteen SAs are given in Fig. S1.† The design scheme is shown in Fig. 1a and S2a.† Through adopting dithiophene and piperazine rings, S and N atoms may offer more lone pair electrons and stronger polar forces toward analytes. To verify the extent of the reaction and characterize the final structure of the prepared powder, IR, XRPD, TGA, SEM, and BET surface tests were carried out successively. For SCAU-2, the strong peak at 3394 cm−1 corresponds to the stretching vibration of –NH– groups (Fig. 2b). The substantial weakening of the peak at 1654 cm−1 indicated that most –CHO groups participated in the reaction. The peaks at 1560 and 1477 cm−1 are from triazine rings, and the peaks at 1340 and 1157 cm−1 represent the –C–N–C– stretching vibrations of imides, which means that melamine has reacted with dialdehyde. The IR spectrum was consistent with SNW-1,18 a porous CTF reported by Thomas, showing that the prepared CTF has a similar structure as SNW-1 (Fig. S2b†). From the XRPD pattern (Fig. S2c†), it can be seen that SCAU-2 was basically an amorphous material with certain crystallinity.
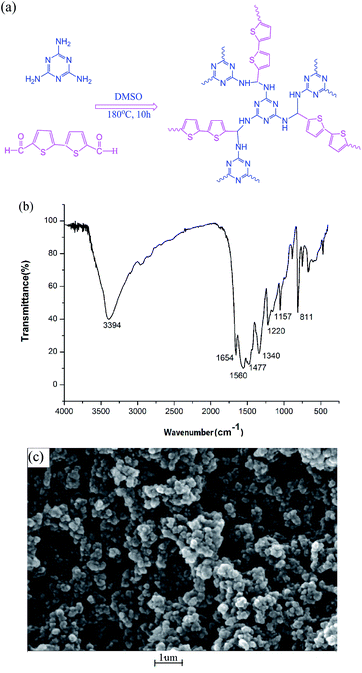 |
| Fig. 1 (a) The synthesis scheme of SCAU-2; (b) the FTIR spectrum of the prepared SCAU-2; and (c) a scanning electron micrograph of SCAU-2. | |
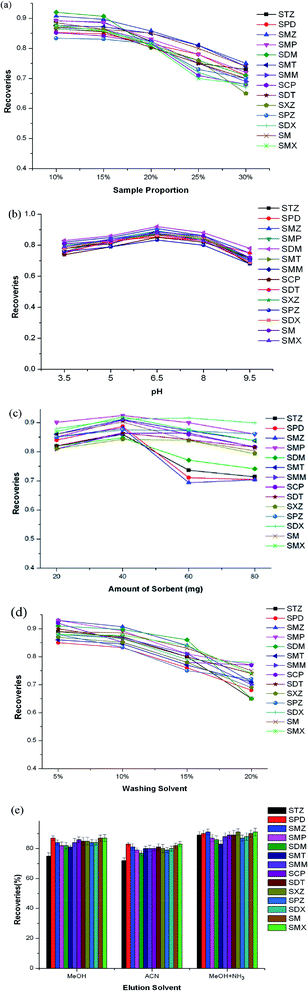 |
| Fig. 2 (a) The effects of sample proportion on the recoveries of SAs (conditions: pH, 6.5; washing solvent, 1 mL of water with 5% MeOH; elution solvent, 2 mL of MeOH; spike level, 50 ng g−1). (b) The effects of pH value on the recoveries of SAs. (c) The effects of sorbent amount on the recoveries of SAs. (d) The effects of the washing solvent on the recoveries of SAs. (e) The effect of elution solvent on the recoveries of SAs. | |
Scanning electron microscopy (SEM) characterization (Fig. 1c) shows that the morphology of SCAU-2 involved uniform particles with diameters of hundreds of nanometers. The TGA curve shows that the solvent molecules in the cavity were lost at about 200 °C, and then the framework gradually collapsed with an increase in temperature (Fig. S2d†). N2 adsorption experiments were also carried out to verify the adsorptivity of the prepared powder. The BET surface of SCAU-2 was 142.05 m2 g−1, which was slightly larger than SCAU-1 (110.27 m2 g−1) due to the bigger size of the bithiophene monomers. The idle –NH2 groups could undergo more H-bond interactions with the analytes, which may promote adsorption to some extent.
Optimization of the SPE conditions
A series of influencing factors, including the dilution ratio and pH of the sample, the amount of sorbent, and the washing and elution solvents, was investigated in detail. Each cycle of the SPE process was repeated three times, and the final extraction recoveries were averages of three results. To reduce the outflow of analytes when loading samples, the extract was concentrated to 1.5 mL first. Then, different sample/water dilution ratios, including 10%, 15%, 20%, 25%, and 30%, were tested, in which the loading volumes were equivalent to 15, 10, 7.5, 6, and 5 mL, respectively. As seen in Fig. 2a, the recoveries decreased slightly when the dilution ratio was increased from 10% to 15%, then significantly declined from 15% to 30%. To reduce the loading losses of analytes and shorten the sampling time, 10 mL of sample with a dilution ratio of 15% was chosen as the final sample volume for subsequent optimization.
The pH of the sample can affect the protonation of SA in solution, and the charge states of functional groups existing on the surface or inside the cavities of the COF could also be affected by protons in the solution. To improve the extraction efficiency, different pH values of 3.5, 5, 6.5, 8, and 9.5 were all tested (Fig. 2b). It can be seen that the highest recoveries occurred at pH 6.5. At pH 3.5, most SAs exist in neutral form, which is favorable for hydrophobic interactions between the heterocyclic rings and aromatic ring of SAs.23 At pH 9.5, the anionic SAs easily outflowed during the sampling step, owing to the sorbent being negatively charged and the π–π interactions being weakened.
The amount of sorbent used was also investigated to save on the usage of sorbent. 20, 40, 60, and 80 mg were used as different levels. As seen from Fig. 2c, the recoveries increased slightly from 20 to 40 mg, then decreased from 40 to 80 mg. The reason may be that when the amount is more than 40 mg, the adsorbed SAs cannot be eluted adequately with the specific volume of organic solvents. Considering the overall recoveries, 40 mg was chosen as the final amount to save on the usage of sorbent.
To reduce matrix effects, different MeOH/water amounts were also studied in the washing step. From Fig. 2d, it can be seen that with an increase in the amount of MeOH, the recoveries gradually decreased up to 10%, then declined obviously from 10% to 20%. To minimize matrix effects and obtain higher recoveries, 10% was chosen as the optimum washing solvent level.
The elution solvent was also an important factor affecting extraction efficiencies. In this study, MeOH, ACN, and MeOH with 5% NH3 were investigated as elution solvents (Fig. 2e). It can be seen that for STZ, SMZ, SMP, SXZ, SPZ, SDX, SM, and SMX, MeOH with 5% ammonia showed obvious superiority compared to the other two solvents. For the other six SAs, the studied elution solvents showed similar efficiencies. This may be ascribed to the idea that basic elution may weaken π–π interactions between SAs and the sorbent, and thus SAs could be easily eluted by MeOH with 5% NH3.
The adsorption capacities of the prepared CTFs
To characterize the adsorption abilities of the two CTFs, 40 mg of sorbent was loaded into the SPE column and a series of standard solutions (200, 400, 800, 1000, and 1500 μg L−1) of SAs (10 mL) were tested. The SPE process was carried out according to the given procedure, with three repetitions at each level. For SCAU-2, the recoveries remain nearly stable up to 800 μg L−1. When the concentration increased to 1000 μg L−1, the recoveries slight decline, indicating that the adsorption capacity of SCAU-2 was close to 1000 μg L−1 at a sample volume of 10 mL (Fig. 3a). For SCAU-1, the recoveries decreased up to 800 μg L−1, which meant that the adsorption capacity was approximately 800 μg L−1 for 10 mL of sample (Fig. S2e†). The different capacities of SCAU-2 and SCAU-1 may be ascribed to the idea that the BET surface of SCAU-2 was relatively larger; also, the existence of multiple heterocyclic rings could provide stronger affinity between the sorbent and analytes.
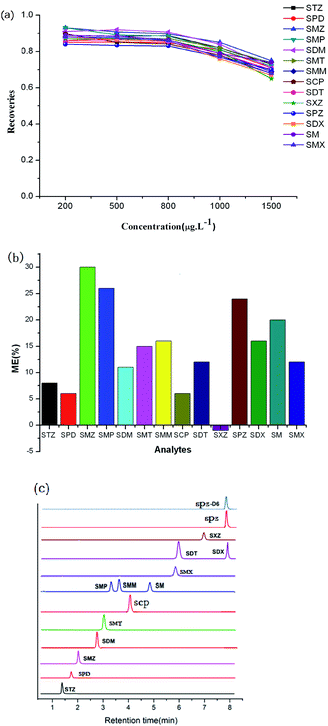 |
| Fig. 3 (a) The adsorption capacities of 40 mg of SCAU-2; each recovery was the average of three repetitions. (b) SA matrix effects in meat samples. (c) Selected ion chromatograms from pork samples spiked at 50 ng g−1. | |
Reusability of the prepared columns
Regeneration is an important parameter and it was evaluated whether the as-prepared adsorbent could be reused and recycled. The reusability of the prepared columns was evaluated through the performance over five consecutive cycles of the SPE process under the optimum conditions. After each cycle, the SPE column was rinsed with 2 mL of MeOH and then with 2 mL of pure water prior to the next cycle. The results show that the RSDs varied from 3.8% to 6.2% for SCAU-2 and from 3.8% to 6.0% for SCAU-1. All RSDs were lower than 10%, meaning that the extraction efficiencies were approximately similar after five cycles, and showing that both kinds of SPE column could be reused at least five times.
Matrix effects
Owing to the complexity of meat samples, matrix effects (MEs) were investigated to evaluate our method. The ME values were estimated based on a comparison of the slopes of standard calibrations and matrix-matched calibrations, according to the following equation: ME (%) = [(slope of a standard calibration)/(slope of a matrix-matched calibration) − 1] × 100. The matrix was thought to be ion suppressing when the value of ME was positive. Otherwise, the matrix was ion enhancing. When the value was larger than 20% or smaller than −20%, MEs were supposed to be obvious. The results are shown in Fig. 3b. Obvious suppressions of the signal were observed in the cases of SMZ, SMP, and SPZ with ME >20%. Slight suppressions of the responses were observed for SDX, SMT, SCP, SMM, SDM, STZ, SM, SPD, SMX, and SDT with MEs in the range of 0–20%. Only a slight signal enhancement was observed for SXZ with ME < 0. These results showed that a suppression effect was observed for most SAs, thus a matrix-matched calibration curve was applied for the determination of SAs in meat samples. From the selected TIC chromatograms (Fig. 3c), it can be seen that few impurity peaks existed in the initial 8 min.
Method validation and real sample analysis
The optimal separation and determination conditions for SAs were also optimized (Table S1†). With the standards (1 mg L−1) flowing into the ionization chamber, the cone voltage was modified from 30 to 60 V for each standard, and the collision energies were varied from 15 to 45 eV. Under the optimum conditions, the analytical data from the proposed method for the determination of SAs are summarized in Table 1 (SCAU-2) and Table S2† (SCAU-1). The method presented good linearity in the range of 2.5–100 ng g−1 for SAs, with coefficients between 0.9980 and 0.9995. The limits of detections (LODs) for the method based on a signal-to-noise (S/N) ratio of 3 were in the range of 0.05 to 0.54 ng g−1. The average recoveries (n = 3) were from 86.1% to 91.9% and from 82.3% to 89.1% for SCAU-2 and SCAU-1, respectively. For SCAU-2, the within-lab reproducibility (n = 5) and repeatability (n = 6) (RSD, %) values were determined to be 3.5–6.9% and 3.2–4.8%, respectively, when spiked at 50 ng g−1. For SCAU-1, the within-lab reproducibility and repeatability values were 3.9–6.6% and 2.9–4.8%, respectively. Another two spike levels (10 and 100 ng g−1) were also tested for SCAU-2 and SCAU-1, and the results are given in Table S2.†
Table 1 Figures of merit for the determination of SAs with SCAU-2, and a comparison of the recovery and intraday assay results from SCAU-1 and SCAU-2 at the same spike level
Antibiotic |
Linear range (ng g−1) |
Correlation coefficient (R2) |
LOD (ng g−1) |
Within-lab reproducibility RSDa (%, n = 5) |
SCAU-1 |
SCAU-2 |
Recovery% |
Repeatability RSDa (%, n = 6) |
Recovery% |
Repeatability RSDa (%, n = 6) |
Spike level of 50 ng g−1. |
STZ |
2.5–100 |
0.9981 |
0.17 |
4.2 |
85.6 |
3.9 |
88.6 |
4.1 |
SPD |
2.5–100 |
0.9984 |
0.12 |
3.5 |
83.2 |
3.3 |
84.1 |
3.4 |
SMZ |
2.5–100 |
0.9986 |
0.16 |
4.3 |
86.2 |
3.8 |
90.5 |
4.3 |
SMP |
2.5–100 |
0.9988 |
0.26 |
5.4 |
89.1 |
4.3 |
91.3 |
4.5 |
SDM |
2.5–100 |
0.9995 |
0.52 |
4.8 |
88.2 |
3.3 |
91.6 |
3.8 |
SMT |
2.5–100 |
0.9994 |
0.37 |
6.5 |
82.3 |
3.4 |
88.0 |
4.5 |
SMM |
2.5–100 |
0.9980 |
0.54 |
5.2 |
85.2 |
3.5 |
88.7 |
3.9 |
SCP |
2.5–100 |
0.9995 |
0.24 |
5.6 |
89.4 |
3.9 |
91.0 |
4.5 |
SDT |
2.5–100 |
0.9994 |
0.15 |
5.1 |
86.2 |
4.1 |
89.7 |
3.8 |
SXZ |
2.5–100 |
0.9986 |
0.40 |
4.9 |
83.4 |
4.5 |
88.0 |
3.8 |
SPZ |
2.5–100 |
0.9982 |
0.12 |
5.8 |
86.2 |
3.7 |
87.7 |
3.9 |
SDX |
2.5–100 |
0.9984 |
0.05 |
6.8 |
84.6 |
3.5 |
87.4 |
3.7 |
SM |
2.5–100 |
0.9980 |
0.49 |
4.8 |
85.4 |
3.9 |
88.5 |
3.8 |
SMX |
2.5–100 |
0.9986 |
0.35 |
6.9 |
87.6 |
4.8 |
87.7 |
4.8 |
The method was further applied to the detection of SAs in real samples, including pork, chicken, and beef. The results are given in Table S3,† and they are mainly in accordance with literature reports,29 showing the applicability of the established method for real analysis.
A comparison of the extraction efficiencies of the prepared CTFs and other adsorbents
To evaluate the performance of SCAU-2, the extraction efficiencies of the two kinds of SPE column were tested. From Table 1, it can be seen that the average recoveries with SCAU-2 were basically equivalent to those with SCAU-1. However, the adsorption capacity of SCAU-2 was larger than that of SCAU-1, which may be ascribed to the BET surface of SCAU-2 being larger than that of SCAU-1 and, also, the existence of more thiophene rings in SCAU-2 providing more π–π interactions with SAs. The results indicated that π–π interactions and H-bond interactions played a main role in the adsorption process, which meant that the prepared CTF showed excellent selectivity towards analytes of medium polarity containing benzene or heterocyclic rings.
The established method was also compared with other reported methods, comparing the amounts of sorbent, sample amounts, elution volumes, LODs, and recoveries. From Table 2, it can be seen that the amount of sorbent, and the sample and elution volumes of the established method were at an intermediate level among the listed methods, and the LODs were lower than or close to the literature values. However, the one-step preparation method in our work was simpler, and the cost of materials was lower. Only a few minutes were needed for the SPE process, showing that the established method possessed good applicability.
Table 2 A comparison of the proposed method with other detection methods for SAs in food samples from the literature
Sorbent |
Method |
Sample/amount |
Amount of sorbent (mg) |
Sample volume (mL) |
Elution volume (mL) |
LOD |
Recovery% |
Reference |
Capillary zone electrophoresis. |
C18 |
DSPE/HPLC |
Meat/5 g |
25 |
1 |
0.4 |
1–5 μg kg−1 |
92–95 |
26 |
MWCNTs |
DSPE/HPLC |
Meat/2 g |
150 |
20 |
10 |
112–129 μg kg−1 |
89.2–117.9 |
27 |
Graphene |
SPE/CZEa |
Meat/5 g |
40 |
1 |
2 |
28–63 μg kg−1 |
66.6–111.4 |
28 |
SNW-1@PAN nanofibers |
PT-SPE/HPLC |
Milk/10 mL |
12.5 |
4 |
1 |
1.7–2.7 ng mL−1 |
88–103 |
25 |
Fe3O4@JUC-48 nanocomposite |
MSPE/HPLC |
Meat/6 g |
25 |
8 |
0.8 |
1.73–5.23 μg kg−1 |
76.1–102.6 |
1 |
Fe3O4@COFs nanospheres |
MSPE/HPLC |
Meat/6 g |
10 |
20 |
0.4 |
0.2–1 ng mL−1 |
65.3–107.3 |
29 |
Fe3O4-SiO2-phenyl |
MSPE/HPLC |
Milk/10 mL |
100 |
10 |
3 |
7–14 ng mL−1 |
81.88–114.98 |
30 |
HCP-Fe3O4 |
MSPE/HPLC |
Milk/25 mL |
20 |
25 |
2 |
2–2.5 ng mL−1 |
92–105 |
31 |
CoFe2O4-graphene |
MSPE/HPLC |
Milk/100 mL |
15 |
100 |
0.5 |
1.16–1.59 ng mL−1 |
62–104.3 |
32 |
PCONFs |
SPE/LC–MS/MS |
Milk/2 g |
100 |
2 |
6 |
0.14–2 ng L−1 |
87.6–107.6 |
33 |
UiO-67 |
SPE/HPLC |
Meat/5 g |
15 |
5 |
1.6 |
0.7–6.5 μg kg−1 |
83.4–103.8 |
34 |
CD-MOF |
SPE/HPLC |
Meat/10 g |
40 |
5 |
1 |
0.32–1.7 μg kg−1 |
76–102 |
35 |
SCAU-2/SCAU-1 |
SPE/UHPLC-MS/MS |
Meat/1 g |
40 |
10 |
1.5 |
0.05–0.54 μg kg−1 |
84.1–91.9 |
This method |
Conclusions
A novel triazine-based CTF and a comparison CTF were prepared and successfully used as SPE sorbents to extract fourteen kinds of SAs from meat. Both sorbents achieved high extraction efficiencies and selectivities towards SAs, and the adsorption capacity of SCAU-2 is larger than that of SCAU-1. The results showed that using monomers containing heterocyclic rings could be an effective strategy, which could be extended to the synthesis of other COFs and motivate studies involving main-skeleton modifications of monomers. With determination via UHPLC-QTOF-MS/MS, the established method exhibited low LODs, wide linear ranges, and good repeatability. Hence, the prepared CTF may be a promising material for the enrichment of organic pollutants with benzene rings.
Conflicts of interest
There are no conflicts to declare.
Acknowledgements
This work was supported by Guangdong Basic and Applied Basic Research Foundation (2019B1515210016), the Youth Teacher Award Foundation of South China Agricultural University (provided by Guangdong Wens Dahuanong Biotechnology Co., Ltd; Grant No. 5500-A17003), and the National Natural Science Foundation of China (21707128).
References
- L. Xia, L. J. Liu, X. X. Lv, F. Qu, G. L. Li and J. M. You, Towards the determination of sulfonamides in meat samples: a magnetic and mesoporous metal–organic framework as an efficient sorbent for magnetic solid phase extraction combined with high-performance liquid chromatography, J. Chromatogr. A, 2017, 1500, 24–31 CrossRef CAS.
- M. Izanloo, A. Esrafili, M. Behbahani, M. Ghambarian and H. R. Sobhi, Trace quantification of selected sulfonamides in aqueous media by implementation of a new dispersive solid-phase extraction method using a nanomagnetic titanium dioxide graphene-based sorbent and HPLC-UV, J. Sep. Sci., 2018, 41, 910–917 CrossRef CAS.
- S. G. Dmitrienko, E. V. Kochuk, V. V. Tolmacheva, V. V. Apyari and Y. A. Zolotov, Determination of the total content of some sulfonamides in milk using solid-phase extraction coupled with off-line derivatization and spectrophotometric detection, Food Chem., 2015, 188, 51–56 CrossRef CAS.
- J. H. Zheng, K. K. cheng and P. F. Yu, Simple preparation of fluorescence probe based on CdTe quantum dots combined with MIP for selective detection of sulfadimidine, J. Mater. Sci.: Mater. Electron., 2019, 30, 21177–21184 CrossRef CAS.
- C. F. Poole, New trends in solid-phase extraction, TrAC, Trends Anal. Chem., 2003, 22, 362–373 CrossRef CAS.
- M. Čizmićv, S. Babić and M. Kaštelan-Macan, Multi-class determination of pharmaceuticals in wastewaters by solid-phase extraction and liquid chromatography tandem mass spectrometry with matrix effect study, Environ. Sci. Pollut. Res., 2017, 24, 20521–20539 CrossRef.
- T. T. Wang, Polyethyleneimine-modified hybrid silica sorbent for hydrophilicsolid-phase extraction of thyreostats in animal tissues, J. Chromatogr. A, 2018, 1581–1582, 16–24 CrossRef CAS.
- G. H. Wang, Y. Q. Lei and H. C. Song, Evaluation of Fe3O4@SiO2–MOF-177 as an advantageous adsorbent for magnetic solid-phase extraction of phenols in environmental water samples, Anal. Methods, 2014, 6, 7842–7847 RSC.
- S. Y. Ding and W. Wang, Covalent Organic Frameworks (COFs): From Design to Applications, Chem. Soc. Rev., 2013, 42, 548–568 RSC.
- X. Feng, X. Ding and D. Jiang, Covalent organic frameworks, Chem. Soc. Rev., 2012, 41, 6010–6022 RSC.
- A. P. Cote, A. I. Benin, N. W. Ockwig, M. O. Keeffe, A. J. Matzger and O. M. Yaghi, Porous Crystalline, Covalent Organic Frameworks, Science, 2005, 310, 1166–1170 CrossRef CAS.
- S. Kandambeth, V. Venkatesh, D. B. Shinde, S. Kumari, A. Halder, S. Verma and R. Ban-erjee, Self-templated chemically stable hollow spherical covalent organic framework, Nat. Commun., 2015, 6, 6786–6795 CrossRef CAS.
- P. Zhang, Z. H. Weng, J. Guo and C. C. Wang, Solution- Dispersible, Colloidal, Conjuga-ted porous polymer networks with entrapped palladium nanocrystals for heterogeneous catalysis of the Suzuki–Miyaura coupling reaction, Chem. Mater., 2011, 23, 5243–5249 CrossRef CAS.
- R. Babarao and J. W. Jiang, Exceptionally high CO2 storage in covalent-organic frameworks: Atomistic simulation study, Energy Environ. Sci., 2008, 1, 139–143 RSC.
- D. P. Cao, J. H. Lan, W. Wang and B. Smit, Lithium-doped 3D covalent organic frameworks: high-capacity hydrogen storage materials, Angew. Chem., Int. Ed., 2009, 48, 4730–4733 CrossRef CAS.
- M. L. Chen, Y. L. Liu, X. W. Xing, X. Zhou, Y. Q. Feng and B. F. Yuan, Preparation of a hyper-cross-linked polymer monolithic column and its application to the sensitive determination of genomic DNA methylation, Chem. - Eur. J., 2013, 19, 1035–1041 CrossRef CAS.
- C. X. Yang and X. P. Yan, Application of covalent-organic frameworks in chromatography and sample pretreatment, Chin. J. Chromatogr., 2018, 3611, 1075–1080 CrossRef.
- J. L. Pan, S. Jia, G. K. Li and Y. L. Hu, Organic building block based microporous network SNW-1 coating fabricated by multilayer interbridging strategy for efficient enrichment of trace volatiles, Anal. Chem., 2015, 87, 3373–3381 CrossRef CAS.
- S. J. He, T. Zeng, S. H. Wang, H. Y. Niu and Y. Q. Cai, Facile synthesis of magnetic covalent organic framework with three- dimensional bouquet-like structure for enhanced extraction of organic targets, ACS Appl. Mater. Interfaces, 2017, 9, 2959–2965 CrossRef CAS.
- W. J. Zhao, H. Y. Zuo, Y. Guo, K. J. Liu, S. Wang, L. J. He, X. M. Jiang, G. Q. Xiang and S. S. Zhang, Porous covalent triazine-terphenyl polymer as hydrophilic–lipophilic balanced sorbent for solid phase extraction of tetracyclines in animal derived foods, Talanta, 2019, 201, 426–432 CrossRef CAS.
- W. F. Zhang, Y. H. Zhang, Q. Jiang, W. J. Zhao, A. J. Yu, H. Chang, X. M. Lu, F. W. Xie, B. X. Ye and S. S. Zhang, Tetraazacalix[2]arence[2]triazine Coated Fe3O4/SiO2 Magnetic Nanoparticles for Simultaneous Dispersive Solid Phase Extraction and Determination of Trace Multitarget Analytes, Anal. Chem., 2016, 88, 10523–10532 CrossRef CAS.
- X. Wang, T. Feng, J. T. Wang, L. Hao, C. Wang, Q. H. Wu and Z. Wang, Preparation of magnetic porous covalent triazine-based organic polymer for the extraction of carbamates prior to high performance liquid chromatography-mass spectrometric detection, J. Chromatogr. A, 2019, 1602, 178–187 CrossRef CAS.
- Y. L. Chen, W. F. Zhang, Y. H. Zhang, Z. F. Deng, W. Zhao, H. F. Du, X. Ma, D. Yin, F. W. Xie, Y. Chen and S. S. Zhang, J. Chromatogr. A, 2018, 1556, 1–9 CrossRef CAS.
- G. H. Wang, Z. K. Hong and Y. Q. Lei, Exploration of a novel triazine-based covalent organic framework for solid-phase extraction of antibiotics, RSC Adv., 2020, 10, 11557–11564 RSC.
- Z. M. Yan, B. Q. Hua, Q. Li, S. X. Zhang, J. Pang and C. H. Wu, Facile synthesis of covalent organic framework incorporated electrospun nanofiber and application to pipette tip solid phaseextraction of sulfonamides in meat samples, J. Chromatogr. A, 2019, 1584, 33–41 CrossRef CAS.
- A. Posyniak, J. Zmudzki and K. Mitrowska, Dispersive solid-phase extraction forthe determination of sulfonamides in chicken muscle by liquidchromatography, J. Chromatogr. A, 2005, 1087, 259–264 CrossRef CAS.
- X. L. Hou, Y. L. Wu, T. Yang and X. D. Du, Multi-walled carbon nanotubes- dispersive solid-phase extraction combined with liquid chromatography-tandem mass spectrometry for the analysis of 18 sulfonamides in pork, J. Chromatogr. B: Anal. Technol. Biomed. Life Sci., 2013, 929, 107–115 CrossRef CAS.
- N. Ye, P. Shi, Q. Wang and J. Li, Graphene as solid-phase extraction adsorbent for CZE determination of sulfonamide residues in meat samples, Chromatographia, 2013, 76, 553–557 CrossRef CAS.
- J. Zhang, Z. P. Chen, S. Tang, X. G. Luo, J. B. Xi, Z. L. He, J. X. Yu and F. S. Wu, Fabrication of porphyrin-based magnetic covalent organic framework for effective extraction and enrichment of sulfonamides, Anal. Chim. Acta, 2019, 1089, 66–77 CrossRef CAS.
- I. S. Ibarra, J. M. Miranda, J. A. Rodriguez, C. Nebot and A. Cepeda, Magnetic solid phase extraction followed by high-performance liquid chromatography for the determination of sulphonamides in milk samples, Food Chem., 2014, 157, 511–517 CrossRef CAS.
- V. V. Tolmacheva, V. V. Apyari, A. A. Furletov, S. G. Dmitrienko and Y. A. Zolotov, Facile synthesis of magnetic hypercrosslinked polystyrene and its application in the magnetic solid-phase extraction of sulfonamides from water and milk samples before their HPLC determination, Talanta, 2016, 152, 203–210 CrossRef CAS.
- Y. Z. Li, X. W. Wu, Z. Q. Li, S. X. Zhong, W. P. Wang, A. J. Wang and J. R. Chen, Fabrication of CoFe2O4-graphene nanocomposite and its application in the magnetic solid phase extraction of sulfonamides from milk samples, Talanta, 2015, 144, 1279–1286 CrossRef CAS.
- G. J. Xu, B. B. Zhang, X. L. Wang, N. Li, Y. F. Zhao, L. Liu, J. M. Lin and R. S. Zhao, Porous covalent organonitridic frameworks for solid-phase extraction of sulfonamide antibiotics, Microchim. Acta, 2019, 186, 26–32 CrossRef.
- L. Xia, Y. N. Dou, J. Gao, Y. M. Gao, W. J. Fan, G. L. Li and J. M. You, Adsorption behavior of a metal organic framework of University in Oslo 67 and its application to the extraction of sulfonamides in meat samples, J. Chromatogr. A, 2010, 24, 460949–460960 Search PubMed.
- Y. Y. Li, N. Zhu, T. Chen, Y. L. Ma and Q. Li, A green cyclodextrin metal–organic framework as solid-phase extractionmedium for enrichment of sulfonamides before their HPLC determination, Microchem. J., 2018, 138, 401–407 CrossRef CAS.
Footnote |
† Electronic supplementary information (ESI) available. See DOI: 10.1039/d0ra04101g |
|
This journal is © The Royal Society of Chemistry 2020 |
Click here to see how this site uses Cookies. View our privacy policy here.