DOI:
10.1039/D0RA04624H
(Paper)
RSC Adv., 2020,
10, 24352-24361
Understanding human salivary esterase activity and its variation under wine consumption conditions†
Received
25th May 2020
, Accepted 22nd June 2020
First published on 25th June 2020
Abstract
Salivary esterase enzymes have been related to the in vitro hydrolysis of carboxylic esters associated with fruity and pleasant aroma nuances in many types of wine. However, very little is known about human total salivary esterase activity (TSEA) under physiological conditions. The purpose of this study is to gain understanding of TSEA and its relevance under wine consumption conditions. To do this, a methodology for TSEA measurement was optimised and applied to examine inter-individual differences (n = 10). Furthermore, TSEA was correlated with other salivary parameters (flow, pH, total protein content). The effect of the oral exposure to different types of wine-like solutions with different composition (ethanol, phenolic and aroma compounds) on TSEA was also assessed. Results showed large inter-individual differences, up to 86%, on TSEA values. Additionally, TSEA was positively correlated with the total salivary protein content (TPC) and negatively correlated with salivary pH and flow. After the oral exposure to wine-like solutions, the combined presence of ethanol, carboxylic esters and phenolic compounds produced the highest TSEA value. Results from this work prove that human salivary esterase is active during wine consumption, and adds support to the involvement of this enzymatic activity on wine aroma perception during wine intake, which will require future studies.
1 Introduction
Esters are one of the most important groups of volatile compounds contributing to the wine aroma profile.1 They can be classified as ethyl esters of organic acids, acetate esters of higher alcohols or esters of fatty acids.2 Wine esters are mainly produced during alcoholic fermentation,3 but they can also be formed from the esterification of alcohols and acids during wine ageing.4 The importance of esters lies in their contribution to the pleasant and fruity aroma of wines and therefore in their quality.5–7 Although many esters can be present in wines at concentrations below their threshold value, they can also reinforce the fruity character of wines by a synergic effect.8 However, at higher concentrations, esters might have negative connotations in wine aroma.9 Because of this, small changes in their concentration might have relevant consequences on wine aroma.
Despite the transformations that could affect wine esters during the winemaking process, which have been widely studied,2–4,10 it is also important to take into account the chemical and biochemical changes of these odorant compounds that might take place during the oral processing of wine.
The time that wine is in the mouth when drinking is short, but large enough to affect the release pattern of volatile compounds in the mouth,11,12 or in the nose,13 and therefore aroma perception.14 There are diverse oral physiological factors that might affect aroma compounds during ingestion, for instance, saliva and its components.15
Saliva is made up of 99% water, but it is also composed of several inorganic salts (calcium, sodium, potassium, etc.)16 and organic compounds (enzymes, proteins).17,18 The composition of saliva varies among individuals and depends not only on genetics, but also on diet, age, gender, oral hygiene, functional status, pathologies, etc.18–20
Saliva plays a significant role in wine aroma perception, which cannot be explained by a single effect. Numerous studies have demonstrated different mechanisms of saliva influencing volatile compounds such as, dilution, retention by salivary proteins, salting-out effects or enzymatic conversion.21–27 In this way, the capacity of salivary enzymes to metabolize certain groups of aroma compounds such as thiols, ketones, aldehydes and esters have been previously reported.17,20,21,26,28
In the specific case of esters, synthesis or hydrolysis processes catalysed by enzymes could be particularly likely, since it is well known that human saliva presents esterase activity.26,29–31 There are different human saliva enzymes that might contribute to the total salivary esterase activity (TSEA) such as carboxylesterase,29 carbonic anhydrase isozyme IV,32,33 cholesterol esterase,34 choline esterase,31,35 lipase,35 or even albumin.36,37
In a recent work26 using static headspace analysis, authors showed a decrease in the release of esters when they were incubated with human saliva (120 min 37 °C), which did not occur in the case of inactive saliva (without enzymatic capacity). These results are in agreement with previous works,17,28,38,39 confirming the capacity of esterase activity from human saliva to hydrolyse odorant carboxylic esters. Furthermore, in the above mentioned work,26 the corresponding products of ester hydrolysis (carboxylic acids) were also found in the systems with human saliva, which have been associated with very different odour nuances and threshold values.
Although ester degradation in the presence of human saliva has been demonstrated, salivary esterase activity has hardly been investigated. For instance, the individual variations on TSEA or how this activity could be affected by typical wine components during wine consumption is still unknown. This information would be of great relevance in order to consider the importance of this enzymatic activity for wine aroma perception.
In view of this, the purpose of this work was to gain understanding on TSEA and its relevance under wine consumption conditions. For this, a methodology for the measurement of TSEA was first optimised and applied to check for inter-individual differences (n = 10). Additionally, the correlation of TSEA with other salivary parameters (flow, pH, total protein content) was also assessed. Finally, the two volunteers with the highest and lowest TSEA values, were selected to determine the effect of the oral exposure to different types of wine-like solutions with different composition (ethanol, phenolic and aroma composition) on TSEA.
2 Materials and methods
2.1 Saliva collection and characterisation
Stimulated saliva was collected from ten healthy subjects (four men and six women) between 20 and 35 years old. They were non-smokers, had not taken any food or drink and had not brushed their teeth 1 hour prior sampling. All the volunteers were informed of the nature of the study and completed a written consent form before their participation. The experimental protocol was approved by the CSIC Bioethics Committee for Research.
For saliva collection, volunteers chewed a piece of Parafilm™ and spat the saliva produced directly into sterile tubes for 5 minutes. These tubes were weighed before and immediately after saliva collection in order to determine salivary flow, which was calculated as mL min−1. A pH meter (Metler, Zabrze, Poland) was used for pH measurement. Subsequently, salivary samples were centrifuged at 15
000 g for 15 min at 4 °C (ref. 27) and supernatants were stored at −80 °C until required. Prior to the saliva storing, total protein concentration (TPC) was determined in supernatants using the commercial kit Pierce™ BCA Protein Assay Kit (Pierce Thermo Scientific, Illinois, USA). The TSEA determination was performed over the next 7 days. The effect of saliva freezing storage on TSEA was previously checked confirming the lack of effect within the whole duration of the study (seven days) (Fig. 1S†) as previously established.40,41
2.2 Total salivary esterase activity (TSEA) methodology
TSEA was determined by using a previously published colorimetric method42 with some modifications and adapted to saliva samples.
Briefly, TSEA procedure was based on the hydrolysis of the substrate 4-nitrophenyl acetate (4-NPA) to 4-nitrophenol by salivary enzymes with esterase capacity. This hydrolysis produces a colorimetric change (absorbance) which is measured to calculate TSEA.
For this assay, a Tris 50 mM solution at pH = 8 was prepared by mixing 50 mL of Tris (0.2 M) (GE Healthcare Uppsala, Sweden) with 26, 8 mL of HCl (0.2 M) provided by Sigma-Aldrich (Steinheim, Germany). This mixture was made up to 200 mL with milliQ water. A second buffer (McIlvaine buffer pH = 5) was also prepared with 23.4 mL of citric acid (Panreac Química S.A. Barcelona, Spain) at 0.1 M concentration and 25.7 mL of Na2HPO4·12H2O (Merck. Darmstadt, Germany) at 0.2 M. The mixture was then made up to 100 mL with milliQ water. For the substrate preparation, 63 mg of 4-NPA (Sigma-Aldrich. Steinheim, Germany) were dissolved in 5 mL of methanol and made up to 10 mL with McIlvaine buffer.
The TSEA measurements were carried out in m96 microplates (VWR, Pennsylvania, EEUU) The final reaction mixture in each microwell using the optimised conditions contained 25 μL of Tris 50 mM (pH = 8), 74 μL of saliva (previously defrosted at 4 °C) and 25 μL of 4-NPA. The microplates were incubated for 60 minutes at 37 °C in a Multiskan™ FC Microplate Photometer (Thermo Fisher Scientific, Massachusetts, USA). The colorimetric change was measured every 5 min until 35 min at 410 nm, pH 7.4 and 37 °C. One unit of esterase activity (UI) was defined as a change of absorbance of 0.01 optical density. TSEA was calculated as UI per min.34 All the measurements were performed in triplicate.
2.3 Oral exposure to wine like solutions
Four different wine-like solutions (WLS) were prepared (Table 1) using typical wine components (all food grade ingredients). They were all prepared from a tartaric acid (Manuel Riesgo, Madrid, Spain) water solution with pH 3.5 (WLS1). From this, a second solution (WLS2) was prepared by adding 10% ethanol (Panreac Química S.A., Barcelona, Spain). Additionally, another solution (WLS3) similar to WLS2, but containing a commercial grape seed extract (GSE) (500 mg L−1) (Vitaflavan®, DRT Nutraceutics, Dax, France) mainly composed of procyanidins43 was prepared. The procyanidin concentration employed was in the typical range of red wines.44 Finally, a fourth solution (WLS4) was prepared with the same composition as WLS3, but including an aroma mixture of six typical wine esters. For this, six ester solutions were prepared in food grade ethanol. Each of them contained one of the following esters: ethyl butanoate (105-54-4), isoamyl acetate (123-92-2), ethyl pentanoate (539-82-2), ethyl hexanoate (123-66-0), ethyl octanoate (106-32-1) and ethyl decanoate (110-38-3); all of them were food grade and supplied by Sigma-Aldrich (Steinheim, Germany). The final concentration of each odorant compound in WLS4 was 2 mg L−1.
Table 1 Wine-like solutions (WLS) employed to determine TSEA under wine consumption conditions
Code |
Types of “Wine-like” solutions |
GSE (grape seed extract). |
WLS 1 |
Water pH = 3.5 |
WLS 2 |
Water pH = 3.5, 10% ethanol |
WLS 3 |
Water pH = 3.5, 10% ethanol and GSEa (500 mg L−1) |
WLS 4 |
Water pH = 3.5, 10% ethanol, GSEa (500 mg L−1) and a mixture of 6 esters (2 mg L−1) |
From the 10 volunteers recruited for this study, the two with the highest and lowest TSEA were selected. The two individuals rinsed their mouth during 30 seconds with 15 mL of the corresponding wine like solution and then spat it out. After two swallowing events of the remaining saliva in the mouth, their saliva was collected in centrifuge tubes following the same procedure previously explained in Section 2.1. A pectin-water (1 g L−1) solution, followed by a bicarbonate solution and finally, still water were used as palate cleaners between rinses with different types of wine-like solutions (one rinse with each solution). This ensured an adequate cleaning of the oral cavity avoiding possible carryover of phenolic and aroma compounds. The individuals then waited for fifteen minutes until the next WLS sample was tried. Previous trials in the lab showed that most salivary parameters (included TSEA) returned to their initial values after 15 minutes of an oral intervention with wine-like solutions. Additionally, a saliva control (SC) sample from the two volunteers was collected prior to the assays with the WLS. This salivary sample was the reference of their esterase salivary status before the assay. Fig. 1 shows a schematic representation of this procedure. All the saliva samples (saliva control and saliva collected after the rinsing with WLS) were collected the same day. Saliva was kept at 4 °C until their analysis (within the two hours of their collection) and TSEA measurements were performed at the same time.
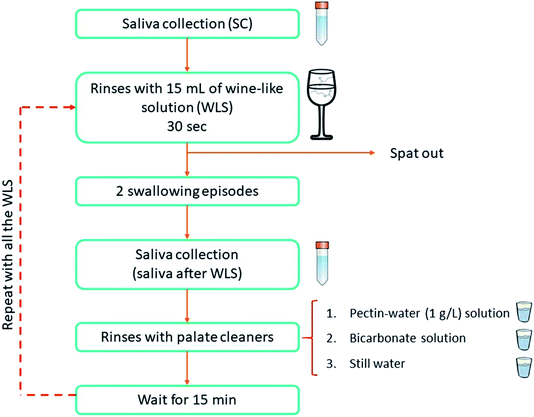 |
| Fig. 1 Experimental procedure followed for saliva collection before and after oral rinsing with wine-like solutions. | |
2.4 Statistical analyses
Different statistical analyses were used depending on the experiment. Regression analysis and the lack-of-fit test were applied to ascertain the linearity of the TSEA method using different amounts of saliva. One-way ANOVA was used to examine differences in TSEA among individuals. Cluster Analysis was carried out to group the subjects depending on their high or low TSEA values. Principal component analysis was used to show the relationship between saliva composition and interindividual differences in TSEA values. Finally, one-way ANOVA was employed to determine differences in TSEA values after rinsing with the different wine-like solutions (WLS). The level of significance fixed was p < 0.05 for all the statistical analyses. Data treatments were carried out using XLSTAT (Addinsoft, Paris, France) and Statgraphics softwares (STSC Inc., Rockville, MD, USA).
3 Results and discussion
3.1 Methodology for TSEA determination
The colorimetric method for TSEA was based on measuring the change in absorbance of 4-NPA when it is hydrolysed by esterase enzymes. In order to optimize a previous methodology42 for saliva samples, some adjustments were necessary. Some of them were related to the preparation of the buffer and the volume of saliva. In the present work, the McIlvaine buffer was prepared with 0.1 M citric acid and 0.2 M Na2HPO4·12H2O instead of 0.1 M citric acid and 0.2 M K2HPO4,42 following the protocol previously described.45 Additionally, a second enzymatic buffer (Tris 50 mM pH = 8), which has also been previously employed for the measurement of esterase activity33,46 was used in the present work. The optimized concentration of the substrate (4-NPA) was also increased from 1 mM previously employed in the reference method,42 to 1.4 mM in the case of our optimised procedure.
In order to select the adequate amount of saliva for the TSEA assay, three assays using different saliva volumes (37 μL, 74 μL and 148 μL) collected from one donor (but the same amount of 4-NPA substrate) were used for TSEA determination. The relationship between saliva volumes and TSEA was determined by linear regression analysis. These different volumes covered a wide range of TSEA values. To judge the adequacy of the linear models, the F-ratio for lack-of-fit was calculated. The regression results are depicted in Fig. 2. As it can be seen, the higher the salivary volume, the higher the TSEA. A linear response between TSEA and saliva volume was corroborated after the application of the lack-of-fit test. A significant correlation between TSEA values and the volume of saliva employed (p-value < 0.0001) was obtained with this test. The coefficient of determination of the regression line was R2 = 0.95. Additionally, as shown in Fig. 2, a higher precision (better repeatability) was observed when TSEA values were higher, which accounted for when using larger salivary volumes. Nonetheless, the best repeatability was obtained when using 74 μL of saliva. In this case, the precision expressed as a percentage of relative standard deviation (% RSD) was 2.30%, while in the case of 37 μL and 148 μL of saliva, the RSD were higher (74.4% and 8.85%, respectively). Therefore, the final saliva volume selected for the TSEA was set to 74 μL.
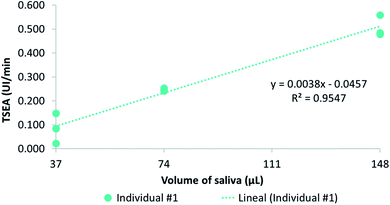 |
| Fig. 2 Representation of the linear regression models of the TSEA (UI per min) and different volumes of saliva (37, 74 and 148 μL) from saliva collected from one donor. | |
To determine the total time for the TSEA measurement, the changes in the absorbance measured at 410 nm in one saliva sample (active saliva, AS), was followed for 60 minutes (Fig. 3). The variation in absorbance was also measured in the same saliva with CaCl2 (0.33 mg mL−1) (inactivated saliva, IS), which had been previously used to inhibit the activity of saliva esterase enzymes.11,17,26 Additionally, the figure also shows the results corresponding to a control sample of water (WC). As it can be seen in the figure (Fig. 3), a continuous increase in the absorbance during the first 30 minutes of the measurement was observed for the active saliva (AS). From this moment on, absorbance was stable until the end of the measurement. As it is shown in the Fig. 3, absorbance remained unchanged in the case of the inactive saliva (IS) with CaCl2, confirming the inhibition of salivary esterase enzymes exerted by this salt. This inhibitory action can be linked to the changes in the ionic strength and dielectric constant affecting the rate in which enzymatic reactions occur, and it seems to depend on the concentration on the salt.47 As expected, the absorbance measured for the control sample (with water) did not change either during the whole measurement time. Therefore, the total time for TSEA measurements was set to 35 minutes.
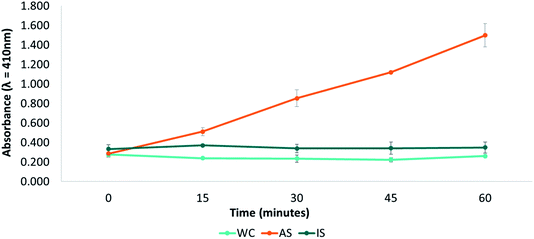 |
| Fig. 3 Absorbance (λ = 410 nm) values during the measurement of TSEA in active saliva (AS), saliva inactivated with CaCl2 (IS) and in a water control (WC). Error bars represent ±SD, at specific times of the measurement. | |
3.2 Individual differences in TSEA and relationship with salivary parameters
As previously suggested by different authors, salivary parameters could be highly variable among individuals.18 To determine interindividual differences in TSEA, saliva samples from 10 volunteers were collected and their TSEA was determined by following the optimised methodology explained in Section 3.1. One-way ANOVA test was run to check for significant differences (p < 0.05) among individuals. These results are shown in Fig. 4. As it is shown, a great variability among volunteers was observed. These values ranged between the lowest for individual #2 (0.28 UI per min) and the highest corresponding to individual #4 (2.63 UI per min). In fact, differences in TSEA values varied as much as 86% among different individuals. Results from the mean comparison test (Tukey test) revealed seven significance levels (described with the letters from a to f in the graph) depending on the TSEA values (Fig. 4). These findings are in agreement with those previously reported,31 in which the authors also observed large interindividual differences in esterase activity in saliva collected from seven donors when using different types of esterase substrates. When they used 4-NPA, as in the present work, the interindividual variations in TSEA values were up to 65% between subjects.31
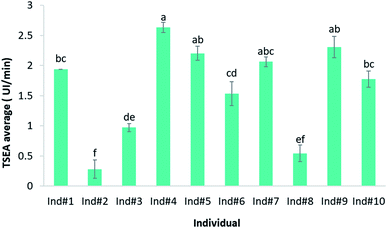 |
| Fig. 4 Average values of TSEA determined for the individuals who participated in this study (n = 10). Error bars represent ±SD. Different letters above the error bars denote statistical significant differences (p < 0.05) from Tukey test. | |
A cluster analysis was applied with the aim to group the subjects based on the similarities in their TSEA values. From the hierarchical clustering procedure (Fig. 2S†), two main groups of individuals were obtained depending on the higher or lower TSEA values. The first group included individuals with higher TSEA values (individuals #4, #5, #7, #9, #1, #10 and #6), between 2.6 UI per min and 1.53 UI per min. The second group was formed by the volunteers with lower esterase activity (individuals #3, #8 and #2) with TSEA values between 0.97 UI per min and 0.28 UI per min.
Additionally, in order to explore the relationship of TSEA with other salivary parameters previously measured in the saliva samples of the ten individuals (pH, flow, total protein content) (Table 1S†), a principal components analysis was also run. The TSEA group (low or high) obtained from the cluster analysis was considered as a supplementary variable. From the PCA, the three first components (PC) explained 96.21% of data variability. Fig. 5 showed the projection of the first two PCs, which explained 57.99% (PC1) and 24.64% (PC2) of data variation. The first component (PC1) was positively correlated to salivary flow (factor loading = 0.853) and pH (factor loading = 0.809), and negatively with the total protein content (factor loading = −0.776). Except for individuals #1 and #9, this component separated the individuals with low TSEA values from those with high TSEA. Both of them presented a high salivary pH compared to the other high TSEA individuals. The second component (PC2) was positively correlated to TSEA (factor loading = 0.719). This component allowed the discrimination of individuals #2, #3 and #8 (low TSEA group) from most of the individuals from the group with high TSEA values (except for individuals #6, #5 and #10).
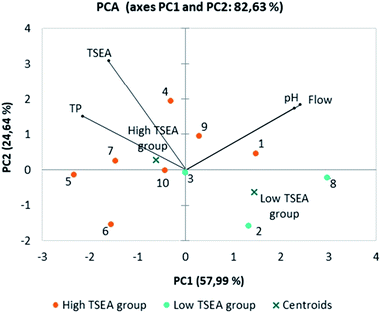 |
| Fig. 5 Principal Component Analysis (PCA) performed with the salivary compositional data (averages) from the 10 individuals. The TSEA group (low or high) obtained from the cluster analysis was considered as a supplementary variable. TPC: total protein concentration; TSEA: total salivary esterase activity. | |
As it can be seen in the PCA (Fig. 5), salivary flow was positively correlated with pH and negatively with the total protein concentration (TPC). The effect of salivary flow in the saliva buffer capacity (bicarbonate, phosphate buffers) which influences saliva pH48,49 could explain this correlation.48,50,51 Additionally, the dilution of proteins at higher flow rates have been also described.52 Interestingly, a positive relationship between TPC and TSEA was also shown in the PCA. This positive correlation was also confirmed using a Spearman correlation test (data not shown) and is in agreement with results from previous works30 in which the authors showed a positive significant correlation between esterase activity of human saliva and α-amylase, one of the main salivary proteins.53
3.3 TSEA under wine consumption conditions
Finally, an interesting aspect to check was how TSEA can be affected under wine consumption conditions, which could be indicative of the potential impact of this salivary activity on wine ester hydrolysis, and therefore, on wine aroma perception. To check this, from the 10 individuals participating in this study, the two with the lowest and the highest TSEA (Ind #2 and Ind #4, respectively) were selected as saliva donors. TSEA was measured in the stimulated saliva collected from these individuals after the oral rinsing with four types of wine-like solutions (WLS1, WLS2, WLS3, and WLS4) as explained in Section 2.1. They all differed in their ethanol, polyphenol (grape seed extract) and odorant (carboxylic esters) content and also had an increasing complexity (Table 1). This means that WLS4 was the most complex wine like solution and the most similar to a wine (pH 3.5, 10% ethanol, GSE and a mixture of odorant compounds). Additionally, saliva control samples (CS) from the two individuals before rinsing their mouths with the different solutions were also collected and TSEA was also measured.
Fig. 6 shows the average TSEA values determined in the two individuals after the oral rinsing with the four synthetic wines. As it can be observed in the figure, great differences in TSEA values were obtained between the two subjects, with as expected, individual #2 presenting lower TSEA values than individual #4.
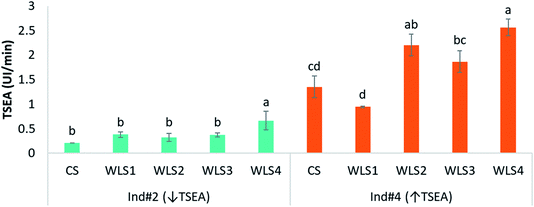 |
| Fig. 6 Comparison of the TSEA values in the control saliva (CS) and in the saliva collected after the oral exposure to the four types of wine like solutions (Table 1) in saliva from two individuals: with lower (Ind #2) and higher (Ind #4) TSEA values. Different letters above the bars denote significant differences from Tukey test (p < 0.005). | |
A one-way ANOVA (one per each subject) was performed in order to check the significant differences among TSEA values. In the case of individual #2, who was chosen because he exhibited the lowest TSEA values from the ten initial volunteers, no significant differences were observed among TSEA values determined in his control saliva compared to his saliva samples collected after the oral exposure to WLS1, WLS2 and WLS3. However, a significant increase in TSEA (about 69% compared to the control saliva) after the oral application of the most complex wine solution (WLS4) was observed. Interestingly, only the presence of a mixture of odorant esters in WLS4 was the difference between this wine type and WLS3 (without them).
One hypothesis to explain these results may be related to the fact that the presence of aromas could have affected salivary secretions, some of which, as shown in Fig. 5, were related to TSEA. Although, to the authors knowledge, there are no studies on the direct effect of the application of odorants in the mouth on salivary secretions, some previous studies have observed that salivary secretions, such as flow, could be altered by olfactory stimulus.54–57 Thus, these results suggest that the presence of odorants could affect TSEA, although further studies are required to understand the mechanisms behind this phenomenon.
Regarding individual #4, who showed the highest TSEA values from the ten volunteers, significant differences in the TSEA values measured in the CS and the saliva samples collected after the oral exposure to the four types of wine like solutions were found (Fig. 6). As it can be observed, the saliva collected for this individual after oral rinsing with WLS1 produced a drop in TSEA compared to the TSEA measured for his control saliva. This could be due to the fact that oral rinsing with an acid solution (like WLS1) might have increased his salivary flow.58,59 As salivary flow was negatively related to TSEA (Fig. 5), this could explain the low TSEA values in this saliva.
On the contrary, it is worth noting that TSEA values were significantly higher in the case of the oral exposure to the three wines with ethanol (WLS2, WLS3, WLS4) compared to the CS or the WLS1, which were only composed of an aqueous tartaric solution. This clearly shows that the salivary esterase enzymes were activated by ethanol. A lack of effect of ethanol on the inhibition of some human salivary cholinesterase enzymes has been shown,31 although this finding was observed at typical saliva pH (7). However, in agreement with results from the present work, a stimulating effect of ethanol (10–16%) on this enzymatic activity at typical wine pH (3.5) has been shown when studying the effect of ethanol concentration on wine lactic acid bacteria esterase activity.42,60 In order to explain this, an increase in the cell membrane permeability produced by ethanol allowing a better penetration of the substrates and hence, increasing the substrate–enzyme interaction has been proposed.60 Other explanations for the stimulating effect of ethanol on TSEA could be the increasing solubility of the enzyme substrates, the higher stability of the enzyme or a decrease in the water-dependent side-reactions.61–63
Interestingly, a lower TSEA after the oral application of WLS3 (with polyphenols) compared to the TSEA values after oral exposure to WLS2, which presented the same composition as WLS3 but without polyphenols (grape seed extract) was also observed (Fig. 6). The main components of the grape seed extract (GSE) employed in this study were flavonoid type compounds, and specifically, monomers and dimers of procyanidins.43 Many works have previously reported the inhibitory effect of different flavonoids such as catechins on salivary α-amylases,64,65 salivary glucosyltransferase66 or digestive lipases.67 The inhibitory effect of oligomeric procyanidins has also been reported for pancreatic lipases.68 Additionally, it has been shown that some types of esterases such as carbonic anhydrases IV and carboxyl esterases can also be affected by different types of phenolic compounds.69–72 For instance, a strong inhibitory effect of flavonoid-type phenolics on human carboxylesterases has been proven under – in vitro conditions.69,70 Therefore, it can be hypothesized that the presence of GSE could have slightly inhibited the TSEA in individual #4 after oral rinsing with WLS3 compared to WLS2. Results from the present work are also in agreement with the lower hydrolysis and significantly higher amounts of the odorant ethyl hexanoate in the headspace of synthetic wines with flavonoids compared to the same wines without them, that have been recently reported.73
However, saliva recovered after the oral exposure to the compositionally most complex wine (WLS4) was significantly higher than that measured for saliva after rinsing with WLS3 and slightly higher than saliva recovered after rinsing with WLS2. As previously commented for individual #2, the presence of odorants in the synthetic wine could have produced an effect on salivary secretions, minimising the inhibitory effect exerted by procyanidins, which are also present in this synthetic wine. Additionally, a synergistic effect of different factors (presence of ethanol, carboxylic esters) cannot be discarded, as it has also been shown in the case of lactic acid bacteria esterases.42
Lastly, it is important to highlight that the effects of the wine matrix components on TSEA were different between individuals. The relatively low TSEA values for individual #2 could have hidden some significant changes between the control saliva (CS) and the saliva collected after the oral exposure to the other three wine-like solutions (WLS1, WLS2, WLS3), that could be observed for individual #4 (with high TSEA values). Moreover, and as previously mentioned, several types of enzymes could simultaneously contribute to the total esterase activity from human saliva. In fact, it has been suggested that esterase enzymes from human saliva are derived from different sources which includes epithelial cells, salivary glands, inflammatory response, and bacteria.74 The fact that the two individuals could have presented different enzyme profiles in their saliva could have contributed to the different effects that the wine components had on their TSEA. This could be another plausible explanation for the great differences found in the TSEA values between the two volunteers after wine rinsing, which will require future investigations.
4 Conclusions
Results from the present work have proven the large interindividual differences in TSEA, which could be up to 80%. Additionally, it has been demonstrated that there is a positive correlation between some salivary parameters such as total protein content and TSEA. Results have also shown that TSEA values significantly increased after the oral exposure to wine-like solutions with similar composition to that of wine (pH 3.5, 10% ethanol, procyanidins and carboxylic esters). This proves that human salivary esterase is active during wine consumption, adding support to the involvement of this enzymatic activity on ester hydrolysis during wine intake, which might also influence aroma perception. However, is also important to highlight that new studies involving a higher number of individuals presenting a wide range of TSEA should be necessary to confirm these preliminary results.
Funding
This work was supported by the Spanish MINECO through the AGL201678936-R (AEI/FEDER, UE) Project. M. P.-J and C. M.-G. thank MINECO for their respective predoctoral and JdC contracts.
Conflicts of interest
Authors declare they do not have any conflict of interest.
Acknowledgements
The authors would like to thank the volunteers who participated in this study and the technical assistance of N. Rocha-Alcubilla and C. Criado in some saliva analyses.
References
- V. Ferreira, R. López, A. Escudero and J. F. Cacho, The aroma of Grenache red wine: hierarchy and nature of its main odorants, J. Sci. Food Agric., 1998, 77, 259–267 CrossRef CAS.
- P. X. Etievant, Volatile compounds in food and beverages, in Wine, Marcel Dekker, New York, 1991, pp. 483–546 Search PubMed.
- M. Lambrechts and I. Pretorius, Yeast and its importance to wine aroma-a review, S. Afr. J. Enol. Vitic., 2000, 21, 97–129 CAS.
- G. Styger, B. Prior and F. F. Bauer, Wine flavor and aroma, J. Ind. Microbiol. Biotechnol., 2011, 38, 1145 CrossRef CAS PubMed.
- A. Escudero, E. Campo, L. Fariña, J. Cacho and V. Ferreira, Analytical characterization of the aroma of five premium red wines. Insights into the role of odor families and the concept of fruitiness of wines, J. Agric. Food Chem., 2007, 55, 4501–4510 CrossRef CAS PubMed.
- I. Francis and J. Newton, Determining wine aroma from compositional data, Aust. J. Grape Wine Res., 2005, 11, 114–126 CrossRef CAS.
- A. Rapp and H. Mandery, Wine aroma, Experientia, 1986, 42, 873–884 CrossRef CAS.
- K. Sumby, P. Grbin and V. Jiranek, Microbial modulation of aromatic esters in wine: current knowledge and future prospects, Food Chem., 2010, 121, 1–16 CrossRef CAS.
- E. J. Bartowsky and P. A. Henschke, Acetic acid bacteria spoilage of bottled red wine—a review, Int. J. Food Microbiol., 2008, 125, 60–70 CrossRef CAS PubMed.
- Y. Margalit, Must and wine composition, Wine chemistry, 1997, pp. 40–44 Search PubMed.
- A. Esteban-Fernández, N. Rocha-Alcubilla, C. Muñoz-González, M. V. Moreno-Arribas and M. Á. Pozo-Bayón, Intra-oral adsorption and release of aroma compounds following in-mouth wine exposure, Food Chem., 2016, 205, 280–288 CrossRef PubMed.
- M. Perez-Jimenez and M. A. Pozo-Bayon, Development of an in-mouth headspace sorptive extraction method (HSSE) for oral aroma monitoring and application to wines of different chemical composition, Food Res. Int., 2019, 121, 97–107 CrossRef CAS PubMed.
- C. Munoz-Gonzalez, F. Canon, G. Feron, E. Guichard and M. A. Pozo-Bayon, Assessment Wine Aroma Persistence by Using an in Vivo PTR-ToF-MS Approach and Its Relationship with Salivary Parameters, Molecules, 2019, 24, 1277 CrossRef CAS PubMed.
- C. Criado, C. Chaya, V. Fernández-Ruíz, M. D. Álvarez, B. Herranz and M. Á. Pozo-Bayón, Effect of saliva composition and flow on inter-individual differences in the temporal perception of retronasal aroma during wine tasting, Food Res. Int., 2019, 126, 108677 CrossRef CAS PubMed.
- F. Canon, F. Neiers and E. Guichard, Saliva and flavor perception: perspectives, J. Agric. Food Chem., 2018, 66, 7873–7879 CrossRef CAS PubMed.
- R. K. Drobitch and C. K. Svensson, Therapeutic drug monitoring in saliva, Clin. Pharmacokinet., 1992, 23, 365–379 CrossRef CAS PubMed.
- A. Buettner, Influence of human salivary enzymes on odorant concentration changes occurring in vivo. 1. Esters and thiols, J. Agric. Food Chem., 2002, 50, 3283–3289 CrossRef CAS PubMed.
- E. Neyraud, O. Palicki, C. Schwartz, S. Nicklaus and G. Feron, Variability of human saliva composition: possible relationships with fat perception and liking, Arch. Oral Biol., 2012, 57, 556–566 CrossRef CAS PubMed.
- P. Piombino, A. Genovese, S. Esposito, L. Moio, P. P. Cutolo, A. Chambery, V. Severino, E. Moneta, D. P. Smith and S. M. Owens, Saliva from obese individuals suppresses the release of aroma compounds from wine, PLoS One, 2014, 9, e85611 CrossRef PubMed.
- C. Munoz-Gonzalez, M. Brule, G. Feron and F. Canon, Does interindividual variability of saliva affect the release and metabolization of aroma compounds ex vivo? The particular case of elderly suffering or not from hyposalivation, J. Texture Stud., 2019, 50, 36–44 CrossRef PubMed.
- A. Buettner, Influence of human saliva on odorant concentrations. 2. Aldehydes, alcohols, 3-alkyl-2-methoxypyrazines, methoxyphenols, and 3-hydroxy-4,5-dimethyl-2(5H)-furanone, J. Agric. Food Chem., 2002, 50, 7105–7110 CrossRef CAS PubMed.
- S. Pages-Helary, I. Andriot, E. Guichard and F. Canon, Retention effect of human saliva on aroma release and respective contribution of salivary mucin and alpha-amylase, Food Res. Int., 2014, 64, 424–431 CrossRef CAS PubMed.
- S. Ployon, M. Morzel and F. Canon, The role of saliva in aroma release and perception, Food Chem., 2017, 226, 212–220 CrossRef CAS PubMed.
- P. Piombino, A. Genovese, S. Esposito, L. Moio, P. P. Cutolo, A. Chambery, V. Severino, E. Moneta, D. P. Smith, S. M. Owens, J. A. Gilbert and D. Ercolini, Saliva from obese individuals suppresses the release of aroma compounds from wine, PLoS One, 2014, 9, e85611 CrossRef PubMed.
- A. Genovese, P. Piombino, A. Gambuti and L. Moio, Simulation of retronasal aroma of white and red wine in a model mouth system. Investigating the influence of saliva on volatile compound concentrations, Food Chem., 2009, 114, 100–107 CrossRef CAS.
- M. Pérez-Jiménez, N. Rocha-Alcubilla and M. Á. Pozo-Bayón, Effect of saliva esterase activity on ester solutions and possible consequences for the in-mouth ester release during wine intake, J. Texture Stud., 2018, 50, 62–70 CrossRef PubMed.
- C. Muñoz-González, G. Feron, E. Guichard, J. J. Rodríguez-Bencomo, P. J. Martín-Álvarez, M. V. Moreno-Arribas and M. Á. Pozo-Bayón, Understanding the Role of Saliva in Aroma Release from Wine by Using Static and Dynamic Headspace Conditions, J. Agric. Food Chem., 2014, 62, 8274–8288 CrossRef PubMed.
- O. Lasekan, A comparative analysis of the influence of human salivary enzymes on odorant concentration in three palm wines, Molecules, 2013, 18, 11809–11823 CrossRef CAS PubMed.
- L. Lindqvist and K.-B. Augustinsson, Esterases in human saliva, Enzyme, 1975, 20, 277–291 CrossRef CAS PubMed.
- F. Tecles, A. Tvarijonaviciute, C. De Torre, J. M. Carrillo, M. Rubio, M. García, R. Cugat and J. J. Cerón, Total esterase activity in human saliva: validation of an automated assay, characterization and behaviour after physical stress, Scand. J. Clin. Lab. Invest., 2016, 76, 324–330 CrossRef CAS PubMed.
- Y. Finer and J. Santerre, Salivary esterase activity and its association with the biodegradation of dental composites, J. Dent. Res., 2004, 83, 22–26 CrossRef CAS PubMed.
- C. T. Supuran, Carbonic anhydrases: novel therapeutic applications for inhibitors and activators, Nat. Rev. Drug Discovery, 2008, 7, 168–181 CrossRef CAS PubMed.
- L. Koc Ozturk, K. Ulucan, S. Akyuz, H. Furuncuoglu, H. Bayer and A. Yarat, The investigation of genetic polymorphisms in the carbonic anhydrase VI gene exon 2 and salivary parameters in type 2 diabetic patients and healthy adults, Mol. Biol. Rep., 2012, 39, 5677–5682 CrossRef PubMed.
- B. A. Lin, F. Jaffer, M. D. Duff, Y. W. Tang and J. P. Santerre, Identifying enzyme activities within human saliva which are relevant to dental resin composite biodegradation, Biomaterials, 2004, 26, 4259–4264 CrossRef PubMed.
- H. H. Chauncey, F. Lionetti and V. F. Lisanti, Enzymes of Human Saliva: II. Parotid Saliva Total Esterases, J. Dent. Res., 1957, 36, 713–716 CrossRef CAS PubMed.
- K. Cai, Y. Delaviz, M. Banh, Y. Guo and J. P. Santerre, Biodegradation of composite resin with ester linkages: identifying human salivary enzyme activity with a potential role in the esterolytic process, Dent. Mater., 2014, 30, 848–860 CrossRef CAS PubMed.
- J. J. Ceron, F. Tecles and A. Tvarijonaviciute, Serum paraoxonase 1 (PON1) measurement: an update, BMC Vet. Res., 2014, 10, 74 CrossRef PubMed.
- M. Virumbrales-Munoz, E. Santos-Vizcaino, L. Paz, A. M. Gallardo-Moreno, G. Orive, R. M. Hernandez, M. Doblare, M. L. Gonzalez-Martin, L. J. Fernandez, J. L. Pedraz and I. Ochoa, Force spectroscopy-based simultaneous topographical and mechanical characterization to study polymer-to-polymer interactions in coated alginate microspheres, Sci. Rep., 2019, 9, 20112 CrossRef CAS PubMed.
- C. Muñoz-González, M. Brulé, G. Feron and F. Canon, Does interindividual variability of saliva affect the release and metabolization of aroma compounds ex vivo? The particular case of elderly suffering or not from hyposalivation, J. Texture Stud., 2019, 50, 36–44 CrossRef PubMed.
- R. G. Schipper, E. Silletti and M. H. Vingerhoeds, Saliva as research material: biochemical, physicochemical and practical aspects, Arch. Oral Biol., 2007, 52, 1114–1135 CrossRef CAS PubMed.
- T. Barranco, C. P Rubio, A. Tvarijonaviciute, M. Rubio, E. Damia, E. Lamy, R. Cugat, J. J. Cerón, F. Tecles and D. Escribano, Changes of salivary biomarkers under different storage conditions: effects of temperature and length of storage, Biochem. Med., 2019, 29, 94–111 CrossRef PubMed.
- F. Perez-Martin, S. Sesena, P. M. Izquierdo and M. L. Palop, Esterase activity of lactic acid bacteria isolated from malolactic fermentation of red wines, Int. J. Food Microbiol., 2013, 163, 153–158 CrossRef CAS PubMed.
- F. Sánchez-Patán, C. Cueva, M. Monagas, G. E. Walton, G. R. Gibson, P. J. Martín-Álvarez, M. V. Moreno-Arribas and B. Bartolomé, Gut microbial catabolism of grape seed flavan-3-ols by human faecal microbiota. Targetted analysis of precursor compounds, intermediate metabolites and end-products, Food Chem., 2012, 131, 337–347 CrossRef.
- F. Cosme, J. Ricardo-Da-Silva and O. Laureano, Tannin profiles of Vitis vinifera L. cv. red grapes growing in Lisbon and from their monovarietal wines, Food Chem., 2009, 112, 197–204 CrossRef CAS.
- M. M. Giusti and R. E. Wrolstad, Current protocols in food analytical chemistry, Current Protocols in Food Analytical Chemistry, 2001,(1), F1 Search PubMed.
- J. A. Verpoorte, S. Mehta and J. T. Edsall, Esterase activities of human carbonic anhydrases B and C, J. Biol. Chem., 1967, 242, 4221–4229 CAS.
- J. Pérez-Villaseñor and J. R. Whitaker, Modification of enzymic activity: III. Effect of salts and organic additives on horseradish peroxidase, Arch. Biochem. Biophys., 1967, 121, 541–547 CrossRef.
- A. Bardow, D. Moe, B. Nyvad and B. Nauntofte, The buffer capacity and buffer systems of human whole saliva measured without loss of CO2, Arch. Oral Biol., 2000, 45, 1–12 CrossRef CAS PubMed.
- S. Baliga, S. Muglikar and R. Kale, Salivary pH: a diagnostic biomarker, J. Indian Soc. Periodontol., 2013, 17, 461 CrossRef PubMed.
- M. Lenander-Lumikari and V. Loimaranta, Saliva and dental caries, Advances in Dental Research, 2000, 14, 40–47 CrossRef CAS PubMed.
- A. Lamanda, Z. Cheaib, M. D. Turgut and A. Lussi, Protein buffering in model systems and in whole human saliva, PLoS One, 2007, 2, e263 CrossRef PubMed.
- R. E. Cohen, A. Aguirre, M. E. Neiders, M. J. Levine, P. C. Jones, M. S. Reddy and J. G. Haar, Immunochemistry and immunogenicity of low molecular weight human salivary mucin, Arch. Oral Biol., 1991, 36, 347–356 CrossRef CAS PubMed.
- E. Scarano, A. Fiorita, P. M. Picciotti, G. C. Passali, L. Calò, T. Cabras, R. Inzitari, C. Fanali, I. Messana, M. Castagnola and G. Paludetti, Proteomics of saliva: personal experience, Acta Otorhinolaryngol. Ital., 2010, 30, 125–130 CAS.
- H. Noh, Y.-G. Im and B.-G. Kim, The Change of Salivary Flow Rate according to Olfactory Stimulation, Journal of Oral Medicine and Pain, 2017, 42, 62–71 CrossRef.
- C. Proserpio, C. de
Graaf, M. Laureati, E. Pagliarini and S. Boesveldt, Impact of ambient odors on food intake, saliva production and appetite ratings, Physiol. Behav., 2017, 174, 35–41 CrossRef CAS PubMed.
- P. Darriet and A. Pons, Wine, in Springer Handbook of Odor, Springer, Cham, 2017, pp. 25–26 Search PubMed.
- E. Neyraud, C. Septier, C. Tournier and S. Chambaron-Ginhac, Can the odours modulate salivation? Impact of the nature of the odorant, 2015 Search PubMed.
- E. Neyraud, C. I. Heinzerling, J. H. F. Bult, C. Mesmin and E. Dransfield, Effects of Different Tastants on Parotid Saliva Flow and Composition, Chemosens. Percept., 2009, 2, 108–116 CrossRef.
- D. A. Froehlich, R. M. Pangborn and J. R. Whitaker, The effect of oral stimulation on human parotid salivary flow rate and alpha-amylase secretion, Physiol. Behav., 1987, 41, 209–217 CrossRef CAS PubMed.
- A. Matthews, P. R. Grbin and V. Jiranek, Biochemical characterisation of the esterase activities of wine lactic acid bacteria, Appl. Microbiol. Biotechnol., 2007, 77, 329–337 CrossRef CAS PubMed.
- C. B. Faulds, M. Pérez-Boada and Á. T. Martínez, Influence of organic co-solvents on the activity and substrate specificity of feruloyl esterases, Bioresour. Technol., 2011, 102, 4962–4967 CrossRef CAS PubMed.
- S. Cherif and Y. Gargouri, An organic-solvent-tolerant esterase from turkey pharyngeal tissue, Bioresour. Technol., 2010, 101, 3732–3736 CrossRef CAS PubMed.
- P. Heitmann and H. Ruttloff, Industrielle Enzyme, Behr's Verlag, Hamburg, 1994, p. 913 Search PubMed.
- Y. Hara and M. Honda, The inhibition of α-amylase by tea polyphenols, Agric. Biol. Chem., 1990, 54, 1939–1945 CAS.
- L. Kandra, G. Gyémánt, Á. Zajácz and G. Batta, Inhibitory effects of tannin on human salivary α-amylase, Biochem. Biophys. Res. Commun., 2004, 319, 1265–1271 CrossRef CAS PubMed.
- S. Otake, M. Makimura, T. Kuroki, Y. Nishihara and M. Hirasawa, Anticaries effects of polyphenolic compounds from Japanese green tea, Caries Res., 1991, 25, 438–443 CrossRef CAS PubMed.
- D. W. Griffiths, in Nutritional and toxicological significance of enzyme inhibitors in foods, Springer, 1986, pp. 509–516 Search PubMed.
- H. Sugiyama, Y. Akazome, T. Shoji, A. Yamaguchi, M. Yasue, T. Kanda and Y. Ohtake, Oligomeric procyanidins in apple polyphenol are main active components for inhibition of pancreatic lipase and triglyceride absorption, J. Agric. Food Chem., 2007, 55, 4604–4609 CrossRef CAS PubMed.
- Z.-M. Weng, G.-B. Ge, T.-Y. Dou, P. Wang, P.-K. Liu, X.-H. Tian, N. Qiao, Y. Yu, L.-W. Zou, Q. Zhou, W.-D. Zhang and J. Hou, Characterization and structure–activity relationship studies of flavonoids as inhibitors against human carboxylesterase 2, Bioorg. Chem., 2018, 77, 320–329 CrossRef CAS PubMed.
- D. Wang, L. Zou, Q. Jin, J. Hou, G. Ge and L. Yang, Human carboxylesterases: a comprehensive review, Acta Pharm. Sin. B, 2018, 8, 699–712 CrossRef PubMed.
- S. B. Ö. Sarikaya, F. Topal, M. Şentürk, I. Gülçin and C. T. Supuran, In vitro inhibition of α-carbonic anhydrase isozymes by some phenolic compounds, Bioorg. Med. Chem. Lett., 2011, 21, 4259–4262 CrossRef PubMed.
- A. Innocenti, S. B. Ö. Sarıkaya, I. Gülçin and C. T. Supuran, Carbonic anhydrase inhibitors. Inhibition of mammalian isoforms I–XIV with a series of natural product polyphenols and phenolic acids, Bioorg. Med. Chem., 2010, 18, 2159–2164 CrossRef CAS PubMed.
- M. Perez-Jiménez, A. Esteban-Fernández, C. Muñoz-González and M. A. Pozo-Bayón, Interactions among Odorants, Phenolic Compounds,
and Oral Components and Their Effects on Wine Aroma Volatility, Molecules, 2020, 25, 1701 CrossRef PubMed.
- Y. Delaviz, M. Yang and J. P. Santerre, Biodegradation Studies of Novel Fluorinated Di-Vinyl Urethane Monomers and Interaction of Biological Elements with Their Polymerized Films, Polymers, 2017, 9, 365 CrossRef PubMed.
Footnote |
† Electronic supplementary information (ESI) available. See DOI: 10.1039/d0ra04624h |
|
This journal is © The Royal Society of Chemistry 2020 |
Click here to see how this site uses Cookies. View our privacy policy here.