DOI:
10.1039/D0RA04965D
(Paper)
RSC Adv., 2020,
10, 38409-38415
Study on inhibitors from acid pretreatment of corn stalk on ethanol fermentation by alcohol yeast
Received
15th June 2020
, Accepted 25th September 2020
First published on 19th October 2020
Abstract
The inhibitory effects of the main inhibitors formed during acid pretreatment of corn stalk were studied through ethanol fermentations of model substrates and hydrolysates from corn stalk by alcohol yeast. Experimental results showed that the tested inhibitors had no significant effect on ethanol fermentations when they were added separately at a concentration according to analysis results from hydrolysate of corn stalk. However, when they were added as a mixture, the inhibitory effects became obvious. With the increase of concentration, there was a delay in ethanol productivity. But complete inhibition was observed at 5.0 g L−1 furfural, 10.0 g L−1 acetic acid, 7.0 g L−1 ferulic acid, and 7.0 g L−1 p-coumaric acid, respectively. The inhibitory effect decreased in the order: furfural > acetic acid > ferulic acid > p-coumaric acid > HMF. These results suggest that a high concentration of inhibitor has a strong negative influence on ethanol fermentation, but the inhibiting abilities of various inhibitors are different.
1. Introduction
The threat of imminent global warming coupled with diminishing crude oil reserves worldwide and rising energy demands entail the urgent need to replace fossil-based fuels with green biofuels. Biomass-based fuels, such as bioethanol, provide a promising alternative, since their energies are already included in the global carbon cycle, implying a significant reduction in carbon dioxide release.1 According to the development program for renewable energy in China, biomass energy resources are composed of the following aspects: agricultural crop straw, waste of forestry and forest product processing, energy crops, poultry and livestock manure, municipal waste, and organic wastewater.2
Lignocellulosic material is a promising alternative energy to fossil resources because it is the most abundant natural renewable organic material that exists on Earth and there are concerns over CO2 emissions from fossil fuels.3 In many countries, including Spain, crop straw is a plentiful by-product from agricultural activities, corn stalk having been selected as the raw material in the present study.
Lignocellulosic materials are rigid structure consisting of a carbohydrate polymer matrix (mainly cellulose and hemicelluloses).4 They are cross-linked and strongly bound to lignin. This structural complexity, defined as biomass recalcitrance, severely restricts enzymatic and microbial accessibility.5 The pretreatment technology can make structure of lignocellulose loose, and make more cellulose and hemicellulose expose to the surface.6 This will help promote the late hydrolysis saccharification.7 Investigations of pretreatment methods included physical method, chemical method, and both combining methods.8 Among these pretreatments, the widely used method is based on dilute acid. Pretreatment corn stalk with dilute sulphuric acid can effectively remove hemicellulose and thereby greatly increased glucose productivity from cellulose hydrolyzation.
Currently, the production of ethanol heavily relies on the fermentation of carbohydrates and this biological.9,10 On the other hand, some of the compounds formed during pretreatment of lignocellulosic biomass such as sugar decomposition products and lignin degradation products may have a potential inhibitory effect on the fermentation process. Inhibitory compounds in lignocellulosic hydrolysates comprise aliphatic acids (i.e. acetic), furaldehydes (i.e. furfural and, 5-hydroxymethylfurfural (HMF)), aromatic compounds and extractives.11,12 The number and identity of these toxic compounds varies with the type of the raw material and pretreatment conditions. Currently an extensive study about the effects of each pretreatment has been done, but a rare study about the inhibitory effect with different pretreatment for ethanol fermentation.
The aim of this study is to investigate the influence of inhibitors formed during pretreatment corn stalk with different concentration of sulfuric acid on ethanol fermentation by alcohol yeast. The different concentrations of inhibitor compounds (acetic acid, furfural, HMF, p-coumaric acid, and ferulic acid) were added into a model substrate medium, alone or in combination, to study their effects on ethanol production.
2. Experimental
2.1. Materials and chemicals
Raw corn stalk (hereinafter RCS) used in experiments was provided by Yinchuan Helan Branch of Ningxia, China. It was cleaned, grinded, and size-reduced to pass a 80-mesh sieve and kept in an oven at 105 °C prior to pretreatment. National Renewable Energy Laboratory (NREL) analytical method was followed to determine raw material composition in terms of structural carbohydrates and lignin13. The cellulose (NS50013) was purchased from Novozymes. Alcohol yeast was obtained from preservation of our lab. All other chemicals were of reagent grade. All experiments were carried out in triplicate and average date was shown.
2.2. Pretreatment of corn straw
RCS was added to different concentrations of sulfuric acid (1%, 3%, 5%, 7%, wt/wt) with 1
:
20 of the solid–liquid. Then they were reacted at 80 °C in a reaction kettle for 2 hours. After pretreatment, the slurry was recovered and residual solid was separated by filtration. Liquid fraction was stored in a refrigeration chamber for further treatment. Depending on the experiment, the whole data of hydrolysate inhibitor or just the liquid fraction (separated by vacuum filtration) were used for fermentation process. All experiments were conducted in triplicate and the results were averaged.
2.3. Fermentation with alcohol yeast
2.3.1. Microorganism and growth culture. Alcohol yeast was obtained from preservation of our lab in a refrigeration chamber. Growth culture was composed of 40 g L−1 glucose, 10 g L−1 peptone, 3.0 g L−1 glycine, 15 g L−1 yeast extract, 0.5 g L−1 (NH4)2SO4, 2.6 g L−1 KH2PO4, and 1.0 g L−1 MgSO4·7H2O. The inoculum was autoclaved at 120 °C for 30 min. This inoculum was grown aerobically on a rotatory shaker at 120 rpm and 30 °C for 48 h.
2.3.2. Fermentation with model substrate media. Model fermentation medium was composed of 40 g L−1 glucose, 3.0 g L−1 glycine, 5.0 g L−1 yeast extract, 1.0 g L−1 (NH4)2SO4, 2.5 g L−1 KH2PO4, and 1.0 g L−1 MgSO4·7H2O. The medium pH was adjusted to 4.8 and autoclaved at 120 °C for 20 min. Inhibitory compounds (acetic acid, furfural, HMF, p-coumaric acid, ferulic acid) were added either individually or as mixtures to the model substrate medium at a certain concentration according to analysis result from the hydrolysate of corn straw. Also, the different concentrations of acetic acid (1.0–7.0 g L−1), furfural (0.1–5.0 g L−1), HMF (0.1–7.0 g L−1), p-coumaric acid (0.1–7.0 g L−1), and ferulic acid (0.1–7.0 g L−1) were added separately to the medium to determine their effects on the ethanol fermentation. Model fermentation experiments were carried out with no oxygen supply in sterile 250 mL bottles with cap. Each bottle was filled with 100 mL of fermentation medium inoculated with 5% (v/v) growth culture.
2.4. Analytical methods
2.4.1. High pressure liquid chromatography (HPLC) analysis. The glucose and acetic acid in the hydrolyzate were determined by a method of HPLC. The liquid phase fraction was filtered through a 0.22 μm filter prior to analysis, and then 20 μL of sample was injected. A HPLC method was carried out using a Bio-Rad (USA) Aminex® HPX-87H column (300 mm × 7.8 mm, Organic Acid Analysis Column) at 65 °C and 0.005 M sulfuric acid as a mobile phase (flow rate was 0.6 mL min−1). A refraction index detector (Shimadzu, Japan) was used for sugars and acetic acid analysis. The contents of furfural, HMF, ferulic acid, and p-coumaric acid were determined by a HPLC method using a diode array detector (Shimadzu, Japan) and an InertSustain C18 column at 40 °C. Acetic acid (0.5 wt%) and methanol ratio of 9
:
1 (v/v) at flow rate of 1 mL min−1 was used as mobile phase, the detection wavelength was 280 nm and the injection volume was 20 μL.
2.4.2. Gas chromatography (GC) analysis. The ethanol content in the fermentation broth was determined using a GC method. The liquid phase fraction was filtered through a 0.22 μm filter prior to analysis and then 1.0 μL of sample was injected. A GC method was carried out using a CP-Wax57CB column (50 m × 0.25 mm × 0.2 μm) at 35 °C keep 5 minutes, then at the rate of 3.5 °C min−1 to 200 °C and keep 5 minutes (flow rate: 3.0 mL min−1).
3. Results and discussion
3.1. Main composition of RCS
In this study, the main compositions of RCS were cellulose 41.13%, hemicellulose 24.43%, acid soluble lignin 10.29%, acid insoluble lignin 15.83%, ash 2.49%, and extract 5.28%. Wu et al.14 reported that different stalks produced different inhibitor content after acid pretreatment due to the different contents of the main components. Wang et al.15 reported that furfural and 5-HMF are produced from the depolymerization of cellulose and hemicellulose to form the dehydration condensation of monosaccharides, while phenolic compounds mainly come from lignin.
3.2. Composition of hydrolysate from the pretreated RCS
After acid pretreatment, the composition of corn straw biomass was altered due to chemical depolymerization. The acid treatment mainly hydrolyzed the hemicellulose and partly removed lignin, which led to the weight loss of RCS. The remaining solid (mainly cellulose and lignin) was porous and easy to be enzymatically digested to glucose.
Inhibitor compounds in water-soluble fraction were analyzed and given in Table 1. The mainly inhibitory compounds found in hydrolysates were acetic acid (a typical by-product from acid hydrolysis), furfural, HMF, and phenolics (p-coumaric acid and ferulic acid), which were degradation products of lignin. The highest 7.22 mg mL−1 acetic acid, 2.41 mg mL−1 HMF, and 2.94 mg mL−1 furfural were detected with 7% sulfuric acid pretreatment which go through cycles of depolymerization. However, this result does not suggest that the yields of inhibitor compounds always increase with the increase of acid concentration. For example, the highest 2.93 mg mL−1 p-coumaric acid and 2.86 mg mL−1 ferulic acid were observed with 5% sulfuric acid pretreatment. The reason of this may be that these factors also affect each other.
Table 1 Inhibitor concentration in hydrolysates from corn straw with different acid hydrolysis
Sulfuric acid (%) |
Inhibitors in hydrolysates (g L−1) |
Acetic acid |
5-HMF |
Furfural |
p-Coumaric |
Ferulic acid |
1 |
1.235 |
0.001 |
0.005 |
0.014 |
0.004 |
3 |
1.381 |
0.002 |
0.026 |
0.026 |
0.029 |
5 |
1.281 |
0.006 |
0.032 |
0.035 |
0.050 |
7 |
1.444 |
0.024 |
0.056 |
0.028 |
0.041 |
Inhibitors formed during dilute acid hydrolysis of biomass include furan aldehydes furfural and 5-hydroxymethylfurfural (HMF) derived from sugar degradation, acetate from hemicellulose, and a number of aromatic aldehydes and acids from lignin.16 Table 2 is monosaccharide compositions of the hydrolyzate from corn after pretreatment with different concentrations of sulfuric acid. The concentrations of monosaccharide decreased with the increases of sulfuric acid concentration. This result was consistent with a tendency of the increasing concentrations of furfural and 5-HMF in hydrolysate.
Table 2 The chemical composition (g L−1) of the tested hydrolyzate from corn after pretreatment with different concentration of sulfuric acid
Sulfuric acid (%) |
1 |
3 |
5 |
7 |
Glucose |
25.32 |
23.21 |
21.37 |
18.47 |
Xylose |
40.52 |
34.45 |
29.17 |
24.25 |
Arabinose |
17.41 |
13.80 |
10.74 |
9.64 |
3.3. Effect of inhibitor at a concentration determined from hydrolysate on fermentation
The presence and concentration of inhibitory compounds can significantly affect enzymatic hydrolysis and fermentation processes and thereby decreases the ethanol production. The performance of alcohol yeast was evaluated on model fermentation media containing inhibitory compounds as well as glucose as carbon source in this study. The influence of inhibitors, individually or as a mixture, was studied in terms of ethanol production, considering two parameters: ethanol yield and sugar consumption.
Table 3 summarises ethanol concentration, as well as the concentration of residual sugar obtained in such solution after 60 h of fermentation when inhibitory compounds were added individually to the model substrate medium with a concentration according to analysis result from the hydrolysate of corn straw. As it can been seen in Table 3, the tested compounds in this concentration mentioned above had a no significant effect on ethanol fermentation. Ethanol content was maintained in the range of 14–16 g L−1 and sugar almost was depleted. The reason of this may be that the contents of inhibitors are low in hydrolysate (see Table 1).
Table 3 Effect of individually inhibitor concentration found in hydrolysates on ethanol fermentation
|
Fermentation time (h) |
Final ethanol concentration (g L−1) |
Final sugars concentration (g L−1) |
Sulfuric acid concentration |
Sulfuric acid concentration |
1% |
3% |
5% |
7% |
1% |
3% |
5% |
7% |
Acetic acid |
0 |
0 |
0 |
0 |
0 |
40 |
40 |
40 |
40 |
12 |
12.97 |
13.63 |
12.80 |
10.58 |
5.86 |
4.64 |
5.81 |
14.08 |
24 |
15.57 |
14.80 |
15.02 |
14.07 |
0.11 |
0.10 |
0.22 |
0.65 |
36 |
16.23 |
15.07 |
15.28 |
14.34 |
0 |
0 |
0 |
0 |
60 |
16.32 |
15.32 |
15.25 |
14.30 |
0 |
0 |
0 |
0 |
Furfural |
0 |
0 |
0 |
0 |
0 |
40 |
40 |
40 |
40 |
12 |
11.36 |
10.08 |
12.42 |
10.89 |
8.75 |
10.52 |
12.38 |
9.54 |
24 |
13.83 |
13.41 |
14.65 |
14.92 |
0.42 |
1.15 |
0.20 |
1.00 |
36 |
14.70 |
14.57 |
15.03 |
14.97 |
0 |
0 |
0 |
0 |
60 |
15.02 |
14.58 |
14.99 |
14.88 |
0 |
0 |
0 |
0 |
5-HMF |
0 |
0 |
0 |
0 |
0 |
40 |
40 |
40 |
40 |
12 |
13.75 |
11.15 |
10.53 |
10.52 |
7.58 |
8.58 |
10.22 |
12.65 |
24 |
14.71 |
15.32 |
14.89 |
13.75 |
0.30 |
0.40 |
0.64 |
1.00 |
36 |
15.31 |
15.43 |
14.93 |
14.84 |
0 |
0 |
0 |
0 |
60 |
16.30 |
15.59 |
15.29 |
14.83 |
0 |
0 |
0 |
0 |
Ferulic acid |
0 |
0 |
0 |
0 |
0 |
40 |
40 |
40 |
40 |
12 |
11.50 |
11.18 |
9.99 |
9.69 |
8.44 |
10.23 |
9.61 |
9.49 |
24 |
14.42 |
15.05 |
13.98 |
13.86 |
0.63 |
0.97 |
1.39 |
1.13 |
36 |
15.12 |
15.37 |
14.63 |
14.07 |
0 |
0 |
0 |
0 |
60 |
15.11 |
15.27 |
15.33 |
14.81 |
0 |
0 |
0 |
0 |
p-Coumaric |
0 |
0 |
0 |
0 |
0 |
40 |
40 |
40 |
40 |
12 |
10.44 |
10.18 |
9.87 |
9.86 |
17.49 |
9.99 |
1.53 |
10.39 |
24 |
14.79 |
12.83 |
13.96 |
13.91 |
1.09 |
1.35 |
0.81 |
1.57 |
36 |
14.80 |
14.01 |
13.99 |
14.41 |
0 |
0 |
0 |
0 |
60 |
14.85 |
14.09 |
13.94 |
14.46 |
0 |
0 |
0 |
0 |
As compared with the individual effect, obvious inhibition against ethanol production was observed in medium when they were added as a mixture, ethanol yield decreased from about 15 g L−1 to 8 g L−1 (Fig. 1). When they added together, the highest ethanol concentration of 8.50 g L−1 was obtained in 7% sulfuric acid hydrolysate and the lowest ethanol concentration of 7.50 g L−1 was obtained in 5% sulfuric acid hydrolysate. However, this result does not mean that the yields of ethanol always decrease with the increase of acid concentration. The highest inhibition effect on fermentation was found in 5% sulfuric acid hydrolysate. Despite lower concentrations of acetic acid, furfural, HMF, p-coumaric acid and ferulic found in hydrolysates from pretreatment, the inhibition effect on fermentation was higher than that used individually. Wang et al.17 reported that most of byproducts had no obvious inhibition on the production of ethanol. However, high concentration of some byproducts in lignocellulose hydrolysate exhibited a high inhibition on the growth of Lactobacillus orientalis HN-1. Díaz et al.18 affirmed that acetic acid, formic acid or furfural, when appearing individually and not in a concentration high enough to totally inhibit cell growth, exert a positive effect on ethanol yield. However, these inhibitors had a negative influence on ethanol yield when they were added together. Yeasts may suffer stress as a result of high osmotic pressure or high concentrations of inhibitory compounds and the combination of these factors can act synergistically, affecting ethanol yields.19
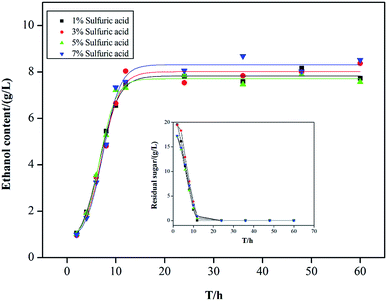 |
| Fig. 1 Effect of inhibitor as a mixture found in hydrolysates on ethanol fermentation. | |
3.4. Effect of high concentration of inhibitor on fermentation
3.4.1. Acetic acid. Acetic acid is one of the common weak acids in the hydrolysis of acid-treated straw biomass. It is produced from hemicellulose during the process of acid hydrolysis. As shown in Fig. 2a and b, the inhibitory effect increased with the increasing concentration of acetic acid. Cellular growth and ethanol production were considerably affected when the concentration of acetic acid was increased to 3 g L−1. At the same time, the fermentation lag period was changed from 24 hours to 48 hours. Complete inhibition of ethanol production was observed in medium containing 10 g L−1 of acetic acid and residual sugar yield remained at 40 g L−1 (Fig. 2b). Toquero et al.20 reported that the medium containing 4 g L−1 of acetic acid displayed almost complete inhibition of ethanol production with 2.1% theoretical ethanol yield. Björling and Lindman21 found complete inhibition of ethanol production by Pichia stipitis in synthetic medium containing 3.9 g L−1 acetic acid at pH 4, while Díaz et al.18 observed cellular growth in fermentations by P. stipitis on 20 g L−1 glucose and 15 g L−1 xylose synthetic medium with 6 g L−1 of acetic acid, but as far as ethanol yields are concerned, also reported an increase with acetic concentration in fermentations performed with 3 and 6 g L−1 of acetic acid. Therefore, there was no significant effect of low concentration of acetic acid on ethanol production, but higher concentration had a stronger effect on the yield of ethanol. When 6 g L−1 of acetic acid was added in hydrolysate, ethanol yield was reduced by 50.83%.22 In this study, there was a delay on ethanol productivity when the concentration of acetic acid was more than 3.0 g L−1, but complete inhibition was observed at 10.0 g L−1 acetic acid. This result was similar to the investigation of Wang et al.,23 who reported that 5 g L−1 of acetic acid had an obvious inhibition effect on glucose and xylose fermentation, 10 g L−1 of acetic acid completely inhibited the cell growth of Kluyveromyces marxianus and ethanol production.
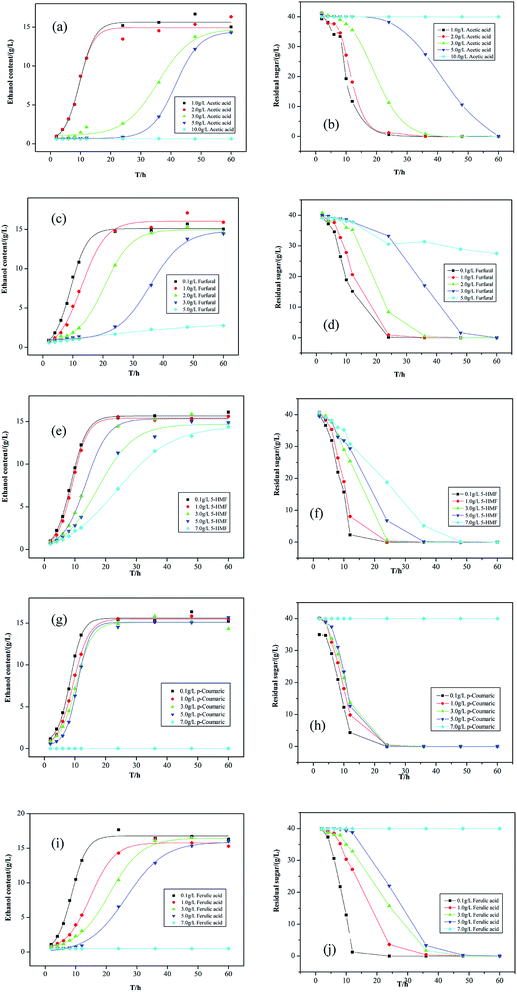 |
| Fig. 2 Concentration of ethanol and residual sugar with inhibitor: (a) and (b) acetic acid; (c) and (d) furfural; (e) and (f) 5-HMF; (g) and (h) p-coumaric; (i) and (j) ferulic acid. | |
The organic acid molecules are dissociated and by the simple diffusion into cells, increase the H+ concentration within the cell membrane. This leads to the ATP to not normally be generated by destroying the membrane potential, and eventually causes the cell growth to slow or die.24
3.4.2. Furfural and HMF. Both furfural and HMF are the main inhibitors of aldehyde in lignocellulosic hydrolysate. As can be seen from Fig. 2c–f, the ethanol fermentation was not obviously affected when the concentrations of furfural and HMF were 0–2 g L−1 and 0–3 g L−1, respectively. However, there was an obvious inhibition effect on ethanol fermentation and fermentation period become longer delay with 3 g L−1 of furfural and 5 g L−1 of 5-HMF. At this time, the consumption of sugar becomes slow. With the increase of inhibitor concentration, complete inhibition of ethanol production was observed in medium containing 5 g L−1 of furfural. In comparison, the inhibition effect of HMF was not greater than that of furfural. When the concentration of furfural and HFM was 2 g L−1, the inhibition rate was 38.8% and 13.2%, respectively. This result was in agreement with the investigation of Sun et al.25
3.4.3. p-Coumaric acid and ferulic acid. Phenolic compounds, such as ferulic acid, p-coumaric acid, syringaldehyde, and vanillin, mainly come from lignin and its derivatives. Fig. 2g–j summarize the effect of p-coumaric acid and ferulic acid on ethanol fermentation and residual sugar. From the chart it can be seen that the inhibitory effect of ferulic acid is higher than that of p-coumaric acid. A concentration of 5 g L−1 of p-coumaric acid had no obvious effect on ethanol fermentation, but 3 g L−1 of ferulic acid exhibited a significantly effect on ethanol fermentation and the fermentation lag period become longer delay. When the concentrations of p-coumaric acid and ferulic acid were 7 g L−1, the fermentation was completely inhibited.Dae et al.26 founded that the inhibition rates of 1 g L−1 of p-coumaric acid, ferulic acid, syringaldehyde, and vanillin on Clostridium beijerinckii growth were 74%, 70%, 3% and 22%, respectively, with little or no butanol. Thus it can be seen that there are some different results obtained from various experimental strategy. This attributes to the discrepancy of species or the tolerance of different alcohols. Phenolic compounds have a strong inhibition on the growth of bacteria, which may be due to the combination of phenolic substances and the hydrophobic sites of bacteria, and then enhances the fluidity of cell membrane, which leads to severe cellular content spillover.27 When the concentration of phenolic substances is relatively low, the fluidity of cell membrane can be reduced by increasing the content of saturated fatty acids in the cell membrane, so as to counteract the toxic effect of phenols against bacteria.
3.5. Effect of inhibitor compounds on fermentation with fed-batch
In order to increase the final substrate concentration while avoiding the increase of viscosity, fed-batch culture was used in the fermentation process in this study. Based on the initial model substrate medium concentration and inhibitor concentration that obtained from corn straw hydrolysates with 7% acid pretreatment, an additional substrate with the same concentration was added during the first 24 h and 48 h of fermentations. The results are shown in Fig. 3. The alcohol content increased gradually along with the constant consumption of sugar. The final ethanol concentration after 60 h of fermentation increased to more than 17 g L−1 in all fed-batch experiments when the glucose source were almost exhausted. In this study, fed-batch fermentation produced the highest amount of ethanol yield, at 17.5 g L−1. Ethanol content increased by 59.5% as compared to the first feeding.
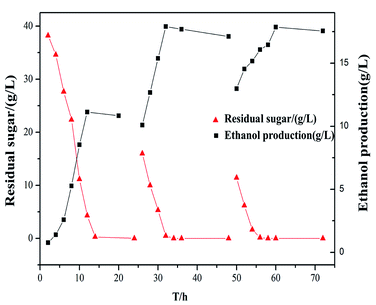 |
| Fig. 3 Concentration of ethanol and residual sugar using batch feeding. | |
Yang et al.28 found that the alcohol content increased by 19.3% in two times feeding with Pichia yeast M4. The fed-batch strategy can alleviate the high viscosity problem caused by high dry matter concentration, which is essential for generation of high ethanol amounts. Approximately 90% of ethanol was produced during the first 48 h of fermentation. From a process-economy aspect, it may be desirable to further shorten this residence time.29
4. Conclusions
Inhibitor compounds from RCS play an important role in ethanol production based on fermentation strategies. As regards the results from acid hydrolysis and fermentation of RCS, the ethanol concentrations obtained from the model substrate medium that inhibitor compounds were added individually were higher than those that were added as a mixture. When the tested inhibitors were used together, however, they negatively affected ethanol yield. The inhibitory effect decreased in the order: furfural > acetic acid > ferulic acid > p-coumaric acid > HMF. Fed-batch fermentation produced a higher amount of ethanol yield as compared with disposable feeding.
Conflicts of interest
There are no conflicts to declare.
Acknowledgements
The authors thank the National Natural Science Foundation of China (21868026), and National First-rate Discipline Construction Project of Ningxia (NXYLXK2017A04).
References
- A. N. Balder and L. S. Rizza, Efficient saccharification of microalgal biomass by Trichoderma harzianum enzymes for the production of ethanol, Algal Res., 2020, 48, 101926 CrossRef.
- Q. Q. Wu, Y. L. Ma, X. Chang and Y. G. Sun, Optimization and kinetic analysis on the sulfuric acid – catalyzed depolymerization of wheat straw, Carbohydr. Polym., 2015, 129, 79–86 CrossRef CAS.
- R. Xiao, W. Yang and X. Cong, Thermogravimetric analysis and reaction kinetics of lignocellulosic biomass pyrolysis, Energy, 2020, 201, 117537 CrossRef CAS.
- Y. G. Sun, Y. L. Ma, Z. Wang and J. K. Yao, Evaluating and optimizing pretreatment technique for catalytic hydrogenolysis conversion of corn stalk into polyol, Bioresour. Technol., 2014, 158, 307–312 CrossRef CAS.
- Y. Pu, F. Hu, F. Huang, B. H. Davison and A. J. Ragauskas, Assessing the molecular structure basis for biomass recalcitrance during dilute acid and hydrothermal pretreatments, Biotechnol. Biofuels, 2013, 6, 15 CrossRef CAS.
- Y. G. Sun, Y. L. Ma, L. Q. Wang, F. Z. Wang, Q. Q. Wu and G. Y. Pan, Physicochemical properties of corn stalk after treatment using steam explosion coupled with acid or alkali, Carbohydr. Polym., 2015, 117, 486–493 CrossRef CAS.
- N. N. Wang, X. L. Xie and X. H. Yang, Research on the pretreatment of corn stalks by diluted acid at low temperature, Sci. Technol. Eng., 2011, 11, 2607 Search PubMed.
- X. L. Chen, J. Yu, Z. B. Zhang and C. H. Lu, Study on structure and thermal stability properties of cellulose fibers from rice straw, Carbohydr. Polym., 2011, 85, 245–250 CrossRef CAS.
- H. Song, P. Wang, Li Shi and W. Deng, Direct conversion of cellulose into ethanol catalysed by a combination of tungstic acid and zirconia-supported Pt nanoparticles, Chem. Commun., 2019, 55, 1–3 RSC.
- F. Wirawan, C.-L. Cheng and Y.-C. Lo, Continuous cellulosic bioethanol co-fermentation by immobilized Zymomonas mobilis and suspended Pichia stipitis in a two-stage process, Appl. Energy, 2020, 266, 114871 CrossRef CAS.
- A. Mahboubi, S. Elyasi and W. Doyen, Concentration-driven reverse membrane bioreactor for the fermentation of highly inhibitory lignocellulosic hydrolysate, Process Biochem., 2020, 92, 409–416 CrossRef.
- F. Z. Wang, X. Chang, Y. L. Ma, Y. G. Sun and Q. Q. Wu, Products and mechanism of corn stalk after depolymerization with acid, J. Chin. Cereals Oils Assoc., 2015, 30, 1–6 CAS.
- A. Sluiter, B. Hames, R. Ruiz, C. Scarlata, J. Sluiter, D. Templeton and D. Crocker. Determination of structural carbohydrates and lignin in biomass. Laboratory Analytical Procedure (LAP), National Renewable Energy Laboratory, 2008, vol. 4 Search PubMed.
- Q. Q. Wu, Y. L. Ma, X. Chang and Y. G. Sun, Optimization and kinetic analysis on the sulfuric acid – Catalyzed depolymerization of wheat straw, Carbohydr. Polym., 2015, 129, 79–86 CrossRef CAS.
- F. Z. Wang, et al., Products and mechanism of corn stalk after depolymerization with acid, J. Chin. Cereals Oils Assoc., 2015, 30(3), 1–5 CAS.
- N. N. Nichols, R. E. Hector, B. C. Saha, S. E. Frazer and G. J. Kennedy, Biological abatement of inhibitors in rice hull hydrolyzate and fermentation to ethanol using conventional and engineered microbes, Biomass Bioenergy, 2014, 67, 79–88 CrossRef CAS.
- F. Q. Wang, Y. Q. Liu, R. Zhang, Y. Y. Wang, H. Xie and A. D. Song, Effect of byproducts in lignocellulose hydrolysates on ethanol fermentation by Issatchenkia orientalis, Chin. J. Biotechnol., 2014, 30, 753–764 CAS.
- M. J. Díaz, E. Ruiz, I. Romero, C. Cara, M. Moya and E. Castro, Inhibition of Pichia stipitis fermentation of hydrolysates from olive tree cuttings, World J. Microbiol. Biotechnol., 2009, 25, 891–899 CrossRef.
- K. Hoyer, M. Galbe and G. Zacchi, Influence of fiber degradation and concentration of fermentable sugars on simultaneous saccharification and fermentation of high-solids spruce slurry to ethanol, Biotechnol. Biofuels, 2013, 6, 145 CrossRef CAS.
- S. Toquero and S. Bolado, Effect of four pretreatments on enzymatic hydrolysis and ethanol fermentation of wheat straw. Influence of inhibitors and washing, Bioresour. Technol., 2014, 157, 68–76 CrossRef.
- T. Björling and B. Lindman, Evaluation of xylose-fermenting yeasts for ethanol production from spent sulfite liquor, Enzyme Microb. Technol., 1989, 11, 240–246 CrossRef.
- X. C. Song, Q. L. Chu, J. J. Zhu, Y. Xu, S. Y. Yu and Q. Yong, Effects of Degradation Products of Carbohydrates on the Fermentation of Saccharomyces cerevisiae, Chem. Ind. For. Prod., 2011, 31, 9–12 CAS.
- N. Wang, W. J. Yuan, X. T. Han, F. W. Bai and Y. P. Xu, Effect of lignocellulose degradation products on ethanol fermentations using glucose and xylose by Kluyveromyces marxianus, Chin. J. Bioprocess Eng., 2012, 10, 17–22 CAS.
- E. Palmqvist and H. Grage, Main and interaction effects of acetic acid, furfural and P-hydroxybenzoic acid on growth and ethanol productivity of yeasts, Biotechnol. Bioeng., 1999, 63, 446–551 CrossRef.
- Y. P. Sun, Y. L. Jin, X. F. Gao, X. B. Li, Y. Xiao and H. Zhao, Effects of Byproducts from Acid Hydrolysis of Lignocellulose on Butanol Fermentation by Clostridium acetobutylicum CICC 8012, J. Appl. Environ. Microbiol., 2010, 16, 845–850 CAS.
- H. C. Dae, J. L. Yun, U. M. Youngsoon, I. S. Byoung and H. K. Yong, Detoxification of model phenolic compounds in lignocellulosic hydrolysates with peroxidase for butanol production from Clostridium beijerinckii, Appl. Microbiol. Biotechnol., 2009, 83, 1035–1043 CrossRef.
- B. M. C. Giovani, I. M. Solange, J. C. Elisangela and B. A. S. Joao, Comparison of different procedures for the detoxification of eucalyptus hemicellulosic hydrolysate for use in fer mentative processes, J. Chem. Technol. Biotechnol., 2006, 81, 152–157 CrossRef.
- S. R. Yang, C. H. Ding, M. Hui and L. J. Yin, Xylose fermentation to prepare ethanol using Pichia stipitis BY fed-batch method, Journal of Henan University of Technology, 2015, 36, 32–36 Search PubMed.
- M. J. Zhang, F. Wang, R. X. Su, W. Qi and Z. M. He, Ethanol production from high dry matter corncob using fed-batch simultaneous saccharification and fermentation after combined pretreatment, Bioresour. Technol., 2010, 101, 4959–4964 CrossRef CAS.
|
This journal is © The Royal Society of Chemistry 2020 |
Click here to see how this site uses Cookies. View our privacy policy here.