DOI:
10.1039/D0RA06462A
(Paper)
RSC Adv., 2020,
10, 32616-32627
Preparation, characterization, antioxidant and antiglycation activities of selenized polysaccharides from blackcurrant
Received
25th July 2020
, Accepted 28th August 2020
First published on 2nd September 2020
Abstract
An ultrasound-assisted enzymatic method was used to extract the polysaccharides from blackcurrant fruits (BP), and then a nitric acid-sodium selenite method was employed to prepare twelve kinds of selenized blackcurrant polysaccharides (SBPs). Among them, SBP-1, SBP-2 and SBP-3 with different selenium contents of 250 ± 11, 312 ± 15 and 643 ± 24 μg g−1, displayed relatively higher 2,2-diphenyl-1-picrylhydrazyl radical (DPPH˙) scavenging activities than the others. After treating with a Sepharose-6B chromatography column, the purified blackcurrant polysaccharide (PBP) and three selenized polysaccharides (PSBP-1, PSBP-2, PSBP-3) with high purity were obtained. Compared with PBP, PSBPs possessed a larger absolute value of zeta potential (ZP) and smaller particle size, indicating the positive influence of selenized modification on physical stability of polysaccharides. Ultraviolet (UV), Fourier transform infrared (FT-IR) and circular dichroism (CD) spectra confirmed that selenium had been introduced onto the polysaccharide structure. X-ray diffraction (XRD) and I2–KI reaction results indicated that selenized modification did not cause an obvious change in crystal form and branch structure of blackcurrant polysaccharides. In addition, PSBPs were superior to PBP in antioxidant and antiglycation capacities, and the bioactivities of PSBPs were significantly improved in positive correlation with selenium content. This study suggested that PSBPs may be a potential selenium source and serve as functional food and medicine.
1. Introduction
Polysaccharides are a kind of important high-molecular carbohydrate, formed of multiple monosaccharide units joined together by glycosidic bonds.1,2 During recent years, natural polysaccharides from vegetables and fruits have attracted more and more attention for their multiple biological and potential pharmacological activities, such as free radical scavenging capacity, inhibition of oxidative damage and tumor-cell proliferation, hypoglycemic and radioprotective effect.2–5 Therefore, the polysaccharides extracted from natural resources have promising application in food, nutraceutical and pharmaceutical industries.
Berry fruits, such as strawberry, blueberry, blackcurrant and bayberry, are delicious and powerful disease-fighting foods and are popularly consumed in the human diet.6 Among these berries, blackcurrant (Ribes nigrum L.) is recognized as one of the major commercial berries, and displayed diuretic, diaphoretic and febrifuge effects in traditional Chinese medicine.7,8 Recently, the polysaccharides from blackcurrant were demonstrated as being responsible for various health beneficial effects, including immunostimulatory, antimicrobial, antitumor, antioxidant and anti-inflammatory activities,2,9 and thereby it is an excellent natural product with broad development prospects.
In general, the polysaccharides contain hydroxyl, carboxyl and other groups that are easy to react with other compounds, providing a basis for the chemical modification.4,13 Meanwhile, chemical modification can improve the physicochemical properties, functional properties and biological activities of natural polysaccharides.5,13 As a result, more and more studies have focused on the chemical modification of the natural polysaccharides.4,5,14 Currently, selenized treatment is the most concerned method for polysaccharides modification. When selenium combines with polysaccharides, the selenized polysaccharides will possess the duple activities of selenium and polysaccharides.13 Moreover, the special selenium–oxygen bonds are formed in selenized polysaccharides, which makes it possible to be easily absorbed and utilized by human body as an organic selenium supplement.15,16 Many research findings have confirmed the positive effects of selenized modification on native polysaccharides from various resources, such as Radix hedysari,16 Momordica charantia L.4 and Cordyceps militaris,17 etc. Our previous studies found that several native polysaccharides extracted from blackcurrant displayed apparent antioxidant and glycation inhibitory activities.10–12 However, the relatively high molecular weight may affect the biological activities and applications of the polysaccharides.2 Some reports showed that selenization modification could not only cause the degradation of polysaccharides, but also improve the biological activities and functional properties of polysaccharides.4,14 Therefore, the investigation on the selenized modification of blackcurrant polysaccharides is needed.
In the present study, the selenized blackcurrant polysaccharides were prepared using nitric acid–sodium selenite (HNO3–Na2SeO3) method. The physicochemical, functional properties, structural characteristics and bioactivities in vitro of three selenized polysaccharides with different selenium contents were evaluated and compared with non-modified polysaccharides. The results of this study will provide valuable information for the selenized modification of natural polysaccharides from other berries.
2. Materials and methods
2.1. Materials
The fully mature blackcurrant fruits (Heifeng) were obtained from College of Horticulture, Northeast Agricultural University and kept frozen at −20 °C.
D4006 macroporous resin and Sepharose-6B were purchased from Nankai University Chemical Plant (Tianjin, China). 2,2-Diphenyl-1-picrylhydrazyl (DPPH), 2,2′-azinobis(3-ethylbenzthiazoline-6-sulphonic acid) (ABTS), phenanthroline, nicotinamide adenine dinucleotide (NADH), nitroblue tetrazolium (NBT), phenazine methosulfate (PMS), ascorbic acid (VC), albumin from bovine serum (BSA) and T-series dextrans were purchased from Sigma-Aldrich Co. (St. Louis, MO, USA). All other reagents used were of analytical grade.
2.2. Extraction of the polysaccharides
Prior to extract, the blackcurrant fruits were defrosted at room temperature and then crushed by a homogenizer (JJ-2, Changzhou Guohua Electric Appliance Co., Ltd, Jiangsu, China). Afterwards, a certain amount of homogenate (50.0 g) and deionized water (1000.0 mL) were mixed in a beaker, and the cellulase (150 mg) and pectinase (75 mg) were added in sequence. Polysaccharides were then extracted at a temperature of 50 °C and a power of 640 W in an ultrasonic cleaning bath (SB-800 DTD, Ningbo Scientz Biotechnology Co., Ltd., Ningbo, China) for 60 min. After heating at 100 °C for 15 min to inactivate the enzymes, the extract was filtrated, concentrated, and precipitated with a four-fold volume of ethanol at 4 °C for 24 h. Subsequently, the precipitate collected by membrane filtration (0.45 μm, New Asia Pharmaceutical Co., Ltd., Shanghai, China) was dialyzed against distilled water (molecular weight cutoff 3500 Da) for 72 h and then the dialysate was concentrated and lyophilized to obtain the crude polysaccharides. Finally, D4006 macroporous resin was used for the preliminary purification of the crude polysaccharides based on previous reported method,2 and the main fraction was collected, concentrated, lyophilized, and designated “BP” for further preparation of the selenized polysaccharides.
2.3. Preparation of the selenized polysaccharides
Twelve groups of modification conditions were designed to prepare the selenized polysaccharides (shown in Table 1). Under fully stirring, 500 mg of BP was mixed with 250 mL of HNO3 (0.5%, v/v) containing 2.5 g BaCl2. The selenized reactions were then started by adding 500 mg of Na2SeO3 (5 mg mL−1) under different ultrasonic powers (500 and 800 W) at various temperatures (50 and 80 °C) for various periods (3, 5 and 7 h). Afterwards, the mixtures were cooled to room temperature and the pH was adjusted to 5.0–6.0 with the saturated Na2CO3. Anhydrous Na2SO4 (1.6 g) was subsequently added and the supernatant without Ba2+ was gathered by centrifuging at 3500 rpm (SC-3610, Anhui USTZ Zookia Scientific Instruments Co., Ltd, Anhui, China) for 20 min. The resulting aqueous fraction was extensively dialyzed against distilled water (molecular weight cutoff 3500 Da) until no free sodium selenium was detected by ascorbic acid method.16 Finally, the dialysates were concentrated and lyophilized to obtain a series of selenized polysaccharides (SBPs).
Table 1 Modification conditions, yields, selenium contents and DPPH˙ scavenging rates of SBPsa
No. |
Time/power/temperature (h/W/°C) |
Yield (%) |
Se content (μg g−1) |
DPPH˙ scavenging rate |
Means with different letters for the same species are significantly different (p < 0.05). |
1 |
3 h/500 W/50 °C |
40.41 ± 0.98d |
171 ± 10a |
18.27 ± 0.33a |
2 |
5 h/500 W/50 °C |
43.19 ± 1.55h |
343 ± 15f |
27.79 ± 1.17c |
3 |
7 h/500 W/50 °C |
41.27 ± 2.07f |
424 ± 22g |
34.15 ± 1.09e |
4 |
3 h/800 W/50 °C |
43.47 ± 0.87i |
182 ± 10b |
40.71 ± 1.61h |
5 |
5 h/800 W/50 °C |
45.35 ± 2.41k |
250 ±11c |
50.90 ± 1.34j |
6 |
7 h/800 W/50 °C |
50.03 ± 0.99l |
312 ± 15d |
52.00 ± 1.21k |
7 |
3 h/500 W/80 °C |
43.98 ± 1.41j |
643 ± 24i |
55.55 ± 1.12l |
8 |
5 h/500 W/80 °C |
38.31 ± 1.39c |
541 ± 16h |
36.46 ± 1.13f |
9 |
7 h/500 W/80 °C |
35.25 ± 2.14a |
732 ± 21k |
46.00 ± 1.21i |
10 |
3 h/800 W/80 °C |
42.58 ± 1.68g |
709 ± 13j |
32.93 ± 1.32d |
11 |
5 h/800 W/80 °C |
40.82 ± 1.85e |
821 ± 16l |
37.57 ± 1.10g |
12 |
7 h/800 W/80 °C |
36.90 ± 1.22b |
318 ± 12e |
20.20 ± 0.86b |
2.4. Determination of selenium content
20.00 mg of SBPs were soaked in 4.0 mL of mixed acid (VHClO4
:
VH2SO4
:
VHNO3 = 1
:
1
:
4) at room temperature overnight. The mixture was then heated at 95 °C for 1.5 h and diluted to 25.0 mL with deionized water after cooling. Following steps were performed in a separatory funnel (125.0 mL). Firstly, 30.0 mL of deionized water was mixed with the solution obtained above, and then the pH was adjusted to 2.0 with NaOH solution (10 mol L−1). Subsequently, 3.0 mL of o-phenylenediamine hydrochloride solution (1%) was added, and the mixture was incubated in the dark for 1 h. Finally, 7.0 mL of toluene each was used to extract selenium in the extract for five times. The sample in toluene layer was collected, concentrated, and the absorbance at 333 nm was measured using a UV spectrophotometer (T6 New Century, Beijing Purkinje General Instrument Co., Ltd, Beijing, China). Selenium content was calculated according to the standard curve: y = 0.0119x + 0.0184, R2 = 0.9968, where, y is the absorbance value at 333 nm, x is the corresponding selenium content (μg).
2.5. Purification of the polysaccharides
BP and three selected selenized polysaccharides (1.0 mL, 10.0 mg mL−1) were loaded on a Sephrose-6B chromatography column (1.5 × 30 cm) and then eluted with deionized water at a flow rate of 0.8 mL min−1.12 The concentrations of polysaccharide in each eluted aliquot (1.0 mL per tube) were determined by using phenol-sulfuric acid method,10 and the elution curves of four polysaccharides were constructed. The main fractions of four polysaccharides were collected, concentrated, lyophilized and separately termed as “PBP”, “PSBP-1”, “PSBP-2” and “PSBP-3” for subsequent experiments.
2.6. Physicochemical properties of PBP and PSBPs
2.6.1 Chemical analysis. 3,5-Dinitrosalicylic acid (DNS) method was used to determine the reducing sugar content using D-glucose as a standard.18 The method of Coomassie Brilliant Blue G-250 was employed for the determination of protein using BSA as a standard.19 The content of polyphenols was measured by the method of Folin–Ciocalteu using gallic acid as a standard.20 Uronic acid content was evaluated according to carbazole-sulfuric acid method by using D-galacturonic acid as a standard.10
2.6.2 Molecular weight determination. Molecular weight determination was performed on a high-performance liquid chromatography (HPLC, LC-10AVP, Shimadzu Corporation, Japan) with a Waters Ultrahydrogel Linear Column (7.8 × 300 mm) and a differential refraction index detector (WATERS-2414). The measurement conditions refer to our previous reports and T-series dextrans were used as the calibrated standards.11
2.6.3 Particle size and ZP analysis. The stability of polysaccharide disperse system could be estimated according to the values of particle size and ZP. Each polysaccharide samples were diluted with deionized water to a final concentration of 2.0 mg mL−1 prior to measurement. The supernatant was gathered by centrifuging at 3500 rpm for 10 min, and then the average particle size and ZP values were determined using a NanoZS90 zeta-sizer (Malvern Instrument Ltd., Worcestershire, UK) at 25 °C with a fixed scattering angle of 90.
2.7. Structural characterization of PBP and PSBPs
2.7.1 UV and FT-IR spectroscopy analysis. UV spectra of samples (2.0 mg mL−1) were recorded on a double beam UV-visible spectrophotometer (TU-1901, Beijing Purkinje General Instrument Co., Ltd., Beijing, China) in the range of 190–630 nm to determine the presence of selenium in the polysaccharides. For FT-IR spectral measurement, which was used for the analysis of organic functional groups in the polysaccharides, the samples were firstly ground with KBr powder and pressed into pellets, and then the spectra ranged from 4000 to 500 cm−1 were acquired on an FT-IR spectrometer (ALPHA-T, BRUKER Co., DE).
2.7.2 XRD analysis. The crystal structure of native polysaccharide and three selenized polysaccharides was determined on an X-ray diffractometer (X'Pert Pro 3040/60, PANalytical, Holland). The voltage and current were maintained as 40 kV and 40 mA, separately. Scan speed was controlled as 10°min−1 and the diffraction angle was changed from 2θ = 5° to 2θ = 70° to detect any changes in the crystal structure.
2.7.3 CD analysis. CD spectra of samples (0.1 mg mL−1) were analyzed in the wavelength range of 190–340 nm by using an MOS-4S0 circular dichroism instrument (Bio-Logic Corporation, France) at 25 °C to detect the conformational variation of the polysaccharides after selenization modification. A total of three scans were averaged at a speed of 50 nm min−1 and the results were expressed in terms of ellipticity. The slit width was 1 nm and the time constant was 1 s, respectively.
2.7.4 I2–KI reaction. I2–KI reaction was performed to explore the existence of branches in the polysaccharide skeleton structure and the starch. 5.0 mL of different polysaccharide solutions (1.0 mg mL−1) were put into the tubes, and then 0.8 mL of iodine solution (0.2% KI solution containing 0.02% I2) was added. After drastic shaking for 10 min, the reaction systems were scanned using a UV-vis spectrophotometer in a range of 300–700 nm. Used to detect whether the polysaccharide main chain has branches and whether it contains starch.
2.8. Functional properties of PBP and PSBPs
2.8.1 Determination of water holding capacity (WHC). WHC was measure as previously described by Jeddou et al.21 Briefly, 20 mL of polysaccharide solution (1%, w/v) was placed in a centrifuge tube and weighed (m). This solution was stirred with a magnetic stirrer for 30 min, and then centrifuged at 3000 rpm for 25 min. The obtained upper phase was discarded and the centrifuge tube was placed in an oven and dried at 50 °C until constant weight (m1). WHC was calculated by the following formula: WHC (g g−1) = m1/m.
2.8.2 Determination of oil holding capacity (OHC). OHC was determined by the method of Song et al.19 Firstly, 0.1 g of polysaccharide sample was put into a centrifuge tube, and the weight was recorded as m. Then, 10.0 mL of soybean oil was fully mixed with sample and standing for 30 min at room temperature. Finally, the solution was centrifuged at 3500 rpm for 25 min to remove the supernatant, and the centrifuge tube was decanted on a filter paper for 30 min until the weight was no longer changed (m1). OHC was calculated using the equation: OHC (g g−1) = m1/m.
2.8.3 Determination of emulsifying capacity. The emulsifying properties of four polysaccharides were investigated according to the method of Romdhane et al.22 In brief, 5.0 mL of soybean oil was mixed with 15.0 mL of polysaccharides solution (1.0%, w/v), and homogenized at 12
000 rpm for 2 min. The volume fraction of oil (%) and the mass of polysaccharide (g) were marked as φ and m. Subsequently, 100 μL of the emulsion was immediately blended with 5.0 mL of SDS (0.1%, w/v), and the absorbance A0 was measured at a wavelength of 500 nm. Ten minutes later, the absorbance A10 was also read by the same method. The emulsifying activity index (EAI) and emulsion stability (ES) of the polysaccharides were calculated as follows: EAI (m2 g−1) = (2 × 2.303 × A0)/(φ × m), ES = A10/A0 × 100%.
2.9. Determination of antioxidant activities in vitro
2.9.1 DPPH˙ scavenging assay. According to the method mentioned by Muniandy et al.,20 2.0 mL of SBPs solutions (2.0 mg mL−1) were firstly mixed with 2.0 mL of DPPH˙ solution (0.2 mmol L−1 DPPH in dehydrated alcohol). Subsequently, the mixture was incubated in dark at room temperature for 30 min. Finally, the absorbance of each mixture at 515 nm was measured to compare the DPPH˙ scavenging activity of SBPs.
2.9.2 2,2′-Azinobis(3-ethylbenzothiazoline-6-sulphonic acid) radical (ABTS+˙) scavenging assay. The ABTS+˙ scavenging capability was assessed as described by Yang et al.12 Before ABTS+˙ assay, ABTS+˙ solution was prepared by incubating 7 mmol L−1 ABTS (2.0 mL) with 140 mmol L−1 potassium persulphate (35 μL) at room temperature under dark condition for 15 h. Then, 4.0 mL of ABTS+˙ solution was mixed thoroughly with 0.2 mL of four polysaccharide (PBP, PSBP-1, PSBP-2 and PSBP-3) solutions with different concentrations (0.2–1.0 mg mL−1). After incubation in the dark for 6 min, the absorbance of the mixture at 734 nm was read and VC was considered as a positive control.DPPH˙ and ABTS+˙ scavenging rates were calculated based on the following equation:
|
 | (1) |
where,
A0 is the absorbance of DPPH˙/ABTS
+˙ solution with test polysaccharide;
A1 is the absorbance of DPPH˙/ABTS
+˙ solution with deionized water;
A2 is the absorbance of polysaccharide solution with deionized water.
2.9.3 Hydroxyl radical scavenging assay. The hydroxyl radical scavenging activity was measured using a reported method with slight modification.1 In essence, 0.20–1.0 mg mL−1 of four polysaccharide solutions (1.0 mL) were respectively reacted with a mixture including 0.75 mmol L−1 of FeSO4 (1.0 mL), 0.01% H2O2 (1.0 mL), and 0.75 mmol L−1 phenanthroline–ethanol solution (1.0 mL) at 37 °C for 60 min. The absorbance of the reaction solution was then recorded at 510 nm, and the VC was used as a positive control. The capability of scavenging hydroxyl radical was assessed with the following formula: |
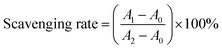 | (2) |
where A0 is the absorbance of the sample when the polysaccharide was replaced with deionized water, A1 is the absorbance of the tested sample system, A2 is the absorbance of the sample when the polysaccharide and H2O2 were replaced with deionized water.
2.9.4 Superoxide anion radical scavenging assay. The superoxide anion radical scavenging assay was determined by the method of Xu et al.2 Briefly, four polysaccharide solutions (1.0 mL, 0.20–1.00 mg mL−1) were successively mixed with NADH solution (1.0 mL, 338 μmol L−1), NBT solution (1.0 mL, 72 μmol L−1) and PMS solution (1.0 mL, 30 μmol L−1). The reaction solution was incubated at room temperature for 5 min and the absorbance at 560 nm was then measured. VC was used as the positive control, and the NADH, NBT and PMS solutions were prepared with Tris–HCl (16 mmol/l L pH 8.0). The scavenging rate was evaluated by the formula (3): |
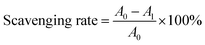 | (3) |
where A0 is the absorbance of the solution without polysaccharide, A1 is the absorbance in the presence of polysaccharide.
2.10. Determination of antiglycation activities
2.10.1 Glycation of BSA. The model of BSA/glucose was established based the method in our previous study.23
2.10.2 Determination of Amadori products. NBT reductive assay was conducted to analyze the Amadori products. Briefly, the solution containing 2.0 mL NBT reagent (30 mmol L−1) and 2.5 mL sodium carbonate buffer (100 mmol L−1, pH 10.35) was added to the glycated solution (0.5 mL). The mixture was incubated at room temperature for 20 min, and then the absorbance at 530 nm was measured against the phosphate buffer blank.
2.10.3 Determination of dicarbonyl compounds. Dicarbonyl compound was tested by a Girard-T assay described by Xu et al.23 In short, the glycated substance (0.4 mL) was transferred into a test tube, Girard-T stock solution (500 mmol L−1, 0.2 mL) and sodium formate (500 mmol L−1, 3.4 mL, pH 2.9) were then added. After reaction at 25 °C for 1 h, the absorbance was measured at 294 nm. Sodium formate solution replaced glycated substance was considered as a control.
2.10.4 Determination of advanced glycation end products (AGEs). The contents of AGEs were determined using previously reported method.10 Simply, the glycated solution (0.5 mL) was completely mixed with sodium buffer (200 mmol L−1, pH 7.4) and the fluorescence value was subsequently quantified by a LS45 fluorescence spectrofluorometer (PerkinElmer Co., Ltd, Waltham, MA, USA). The excitation and emission wavelength was 370 nm and 440 nm, respectively.
2.11. Statistical analysis
The results were represented as mean of three independent replicates ± standard deviation. Statistical analysis was analyzed using SPSS 18.0, and p < 0.05 was taken as statistically significant.
3. Results and discussion
3.1. Preparation of the polysaccharides
Through ultrasound-assisted enzymatic extraction, ethanol precipitation, filtration, dialysis, concentration and lyophilization, the crude polysaccharide was obtained with a purity of 43.61% ± 0.33%. After one run treatment with D4006 macroporous resin, the eluted polysaccharide solution was gathered, concentrated, lyophilized, and the polysaccharide (BP) with a purity of 52.34% ± 0.26% and a recovery yield of 66.90% ± 0.32% was obtained.
3.2. Screening of selenized polysaccharides
As listed in Table 1, twelve kinds of selenized polysaccharides with different yields, selenium contents and DPPH˙ scavenging activities were prepared by varying conditions. It could be found that the yield of selenized polysaccharide reached the peak value of 50.03% ± 0.99% at groups 6, followed by group 5 (45.35% ± 2.41%) and groups 7 (43.98% ± 1.41%). Concurrently, the selenized polysaccharides obtained at these three groups showed the relatively higher DPPH˙ scavenging activities (50.90–55.55%) than the other groups (18.27–46.00%). In addition, the selenium content determined by spectrophotometry revealed the significantly different presence at groups 5–7, which were 250 ± 11, 312 ± 15 and 643 ± 24 μg g−1, respectively. Generally, selenium can bind to oxygen of –OH groups on the polysaccharide in Se–O form, and the binding rate was related to the approachability and number of hydroxyl group of polysaccharide. Therefore, different monosaccharide units and their sequence in the polysaccharides from different plant sources result in different selenium binding rate.13 In addition, the prepared selenized polysaccharides in this study displayed higher selenium content (171–821 μg g−1) than the selenium modified polysaccharide from Cordyceps militaris (16.3–576.4 μg g−1) reported by Zhu et al.,17 which may be caused by the difference in the structure of polysaccharides extracted from different materials.5,13
Based on the comprehensive consideration of yield, selenium content and DPPH˙ scavenging activity, the selenized polysaccharides obtained at groups 5–7 were selected for the subsequent study and were termed as “SBP-1”, “SBP-2” and “SBP-3”, respectively.
3.3. Purification of the polysaccharides
As shown in Fig. 1, the elution curves of the four polysaccharide samples (BP, SBP-1, SBP-2 and SBP-3) displayed sharp and symmetric chromatography peaks. The main fractions were pooled, concentrated, and lyophilized, the homogeneous native (PBP) and selenized polysaccharides (PSBP-1, PSBP-2, PSBP-3) were acquired, whose purity reached to 83.10% ± 0.45%, 82.12% ± 0.13%, 83.58% ± 0.44% and 85.50% ± 0.62%, and the recovery yields were 75.60% ± 0.14%, 72.14% ± 0.19%, 71.31% ± 0.50% and 73.24% ± 0.37%, respectively.
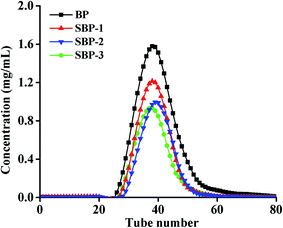 |
| Fig. 1 Elution profile of the BP and SBPs on Sephrose-6B chromatography column. | |
3.4. Effect of selenized modification on the physicochemical properties
3.4.1 Chemical components and molecular weight. As exhibited in Table 2, after Sephrose-6B chromatography purification, the protein and polyphenol contents in PBP and PSBPs were very low. Moreover, their contents in PSBPs were obviously lower than those in PBP, suggesting the efficient removal after selenization. It was worth noting that uronic acid content of selenized polysaccharides was higher than that of untreated polysaccharide, and increased slightly with the increase of selenium content. This may because that the action of ultrasound during selenization in would cause considerable exposure of uronic acid.4 Similar conclusion was also reported by Wang et al.18 Simultaneously, the strong acid circumstance and severe mechanical action also could lead to extensive degradation of polysaccharide chains. Therefore, compared to PBP, the reducing sugar content of PSBPs increased, while the molecular weight of PSBPs decreased.
Table 2 The purity, recovery, Mw and chemical components of PBP and PSBPsa
Sample |
Polyphenol (%) |
Protein (%) |
Reducing sugar (%) |
Uronic acid (%) |
Purity (%) |
Recovery (%) |
Mw (kDa) |
Means with different letters for the same species are significantly different (p < 0.05). |
PBP |
0.22 ± 0.05a |
1.85 ± 0.02a |
0.74 ± 0.15a |
26.67 ± 0.13a |
83.10 ± 0.45 |
75.60 ± 0.14 |
2.04 × 104 |
PSBP-1 |
0.17 ± 0.01b |
0.98 ± 0.03b |
4.40 ± 0.11b |
27.66 ± 0.18b |
82.12 ± 0.13 |
72.14 ± 0.19 |
1.55 × 104 |
PSBP-2 |
0.16 ± 0.04b |
0.87 ± 0.03c |
5 ± 0.26c |
29.26 ± 0.11c |
83.58 ± 0.44 |
71.31 ± 0.50 |
1.29 × 104 |
PSBP-3 |
0.15 ± 0.07b |
0.91 ± 0.02b |
6.50 ± 0.19d |
31.85 ± 0.16d |
85.50 ± 0.62 |
73.24 ± 0.37 |
9.09 × 103 |
3.4.2 Zeta potential. The ZP of PBP and PSBPs were presented in Table 3. ZP values confirmed that both PBP (−12.9 mV) and PSBPs (−14.6 to −18.6 mV) exhibited electronegativity. Moreover, the amount negative charges of PSBPs were proportional to the selenium content. This phenomenon could be attributed to the introduction of electronegative selenium ions onto PBP molecules. These results were in agreement with a research published by Meng et al.24 Additionally, ZP could elucidate the mutually repulsive forces among the particles, and the larger absolute value of ZP indicates that the solution system is more stable.3,25 As revealed in Table 3, the absolute value of ZP of PBP was obviously lower than those of three selenized polysaccharides, signifying the positive effect of selenized modification on solution stability. Generally, the large repulsive force could prevent the flocculation and aggregation of the particles in a colloidal system. PSBPs displayed the higher surface negative charge, implied that electrostatic repulsion between the droplets would be increased, which was in favor of preventing droplet aggregation and increased the stability of the system.25,26
Table 3 Average particle size, PDI and zeta potential of PBP and PSBPs
Sample |
Average particle size (nm) |
PDI |
Zeta potential (mV) |
PBP |
632.9 |
0.50 |
−12.9 |
PSBP-1 |
406.3 |
0.26 |
−14.6 |
PSBP-2 |
342.9 |
0.21 |
−16.3 |
PSBP-3 |
250.4 |
0.18 |
−18.6 |
3.4.3 Particle size. Based on the Stokes' law, a larger particle size of system results in a faster sedimentation rate of particles, and further causing the poor stability. Thus, the stability of a mixture can be indicated by the particle size. As shown in the particle size distribution (Fig. 2), the unmodified polysaccharide PBP was mainly dominated by large particles and was uneven, while the particles of PSBPs were mainly smaller than 1000 nm. Moreover, PSBP-3 with relatively high selenium content had the largest volume fraction of small particles among four polysaccharides. The average particle size order was PBP (632.9 nm) > PSBP-1 (406.3 nm) > PSBP-2 (342.9 nm) > PSBP-3 (250.4 nm) (shown in Table 3). These results indicated that selenized modification could improve the physical stability of polysaccharides dispersions, which was in accordance with the results of zeta potential. Simultaneously, the polydispersity index (PDI) as a quantitative indicator for the uniformity of particle size distribution was also decreased after selenized treatment. Compared with PBP (0.50), PDI were separately reduced to 0.26 for PSBP-1, 0.21 for PSBP-2 and 0.18 for PSBP-3. Therefore, PSBPs were more homogenous polysaccharide in size, which agreed with the finding reported by Li et al.27
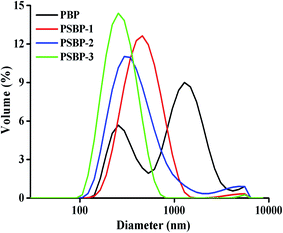 |
| Fig. 2 Particle size distributions of PBP and PSBPs. | |
3.5. Effect of selenized modification on the structural characteristics
3.5.1 UV and FT-IR. From the UV spectra of PBP (Fig. 3A), a downward trend with the increasing of wavelength was observed, which was consistent with the general characteristics of polysaccharides.1 Compared with PBP, PSBPs displayed a characteristic peak at 277 nm, indicating the existence of selenium in the formation of PSBPs.15,17 In addition, the absorption at 260, 280, and 520 nm was not found, suggesting that PBP and PSBPs hardly contained proteins, nucleic acids and pigments.
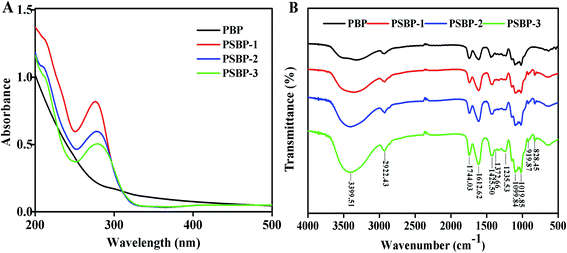 |
| Fig. 3 UV-vis spectra (A) and FT-IR spectra (B) of PBP and PSBPs. | |
As exhibited in Fig. 3B, the FT-IR spectra of PBP and PSBPs displayed the typical absorption peaks of polysaccharides. Among them, the strong band around 3399.51 cm−1 was attributed to the stretching vibration of O–H, which illustrated the presence of intermolecular hydrogen bonding.19,28 The signal at 1744.03 cm−1 represented the vibration of C
O. The obvious peaks toward about 1612.62 cm−1 and 1425.50 cm−1 were assigned to the absorption of deprotonated carboxylic groups, indicating the existence of uronic acid.2 In addition, the specific sharp peaks distributed in the region of 1200–1000 cm−1 were inferred to be the pyranose form of sugar residues.2,12 Compared with PBP, two new absorption peaks appeared at 919.87 cm−1 and 828.45 cm−1 in the spectra of three selenized polysaccharides, which were caused by the stretching vibrations of C–Se–O and Se
O, respectively. Similarly, Gao et al.14 and Wei et al.15 also reported the bands around these wavelength for selenized polysaccharides from alfalfa roots and Radix hedysari. These results signified that selenium was successfully conjunct with the polysaccharides from blackcurrant fruits by O–Se–O or Se
O bond.
3.5.2 CD. Generally, the polysaccharides existed in terms of disordered, extended rigid, collapsed and flexible forms in aqueous solution, and these asymmetric molecular morphologies will produce the Cotton effect.5 Once the changes of polysaccharides conformation occur, the contribution to the static polarizability and the orientation of the chromophores might be influenced, which will lead to the change of the optical activity. CD is a convenient, sensitive and accurate method for determining the conformational changes of polysaccharides. As shown in Fig. 4, the positive Cotton effects at 196 nm and 301 nm, as well as the negative Cotton effect at 223 nm were observed in PBP, suggesting that PBP was ordered and existed as the irregular curl form in aqueous solution.5,29 After the selenized modification, the new chemical group was introduced into the structure of native polysaccharide, and thereby the signals changes in CD spectra happened. In comparison with PBP, the positive Cotton effect of PSBPs was weakened, and PSBPs displayed a strong negative absorption peak at 205 nm, which may be caused by n–π transition of selenate group.5 This finding was consistent with the results of FT-IR spectra.
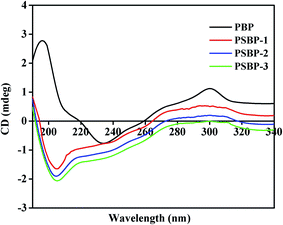 |
| Fig. 4 CD spectra of PBP and PSBPs. | |
3.5.3 XRD. XRD patterns were obtained to determine the crystal configuration and degree of crystallization of polysaccharides. As shown in Fig. 5, four polysaccharides displayed no obvious diffraction peaks in the range of 5 to 70°, and the broad peaks appeared around 21.5° were related to the large and complex polymeric structure of polysaccharides. These results illustrated that both PBP and PSBPs were semi-crystalline or non-crystalline substance. Therefore, selenized modification did not change the crystal form of the blackcurrant polysaccharides. Similar results were also observed in the selenized polysaccharides from Hohenbuehelia serotina.5 Additionally, the maximum peaks location of PSBPs were observed to shift toward amplification compared with that of PBP, which may be due to the introduction of possible stresses in the structural network resulting from new chemical bonds.30 This may cause the variation in physicochemical properties of PSBPs, such as tensile strength, solubility, flexibility and swelling ability.31
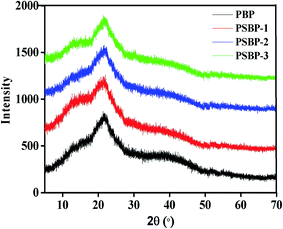 |
| Fig. 5 X-ray diffractograms of PBP and PSBPs. | |
3.5.4 I2–KI reaction. The UV scans of polysaccharides reacted with iodine–potassium iodide reagent before and after selenization were shown in Fig. 6. It could be found that the maximum absorption peaks of all tested polysaccharides (PBP, PSBP-1, PSBP-2, and PSBP-3) were mainly concentrated at 351 nm, while there was no absorption peaks around 565 nm. These results indicated that there were many branches existence in the molecular backbone of PBP and PSBPs.32 In addition, the reaction of four polysaccharides with iodine reagent also confirmed the absence of starch in these polysaccharides.
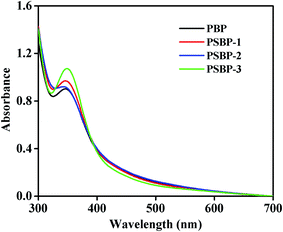 |
| Fig. 6 UV-vis scanning spectra of four mixtures composed of polysaccharides and iodine–potassium iodide reagent. | |
3.6. Effect of selenized modification on the functional properties
Previous studies suggested that hydration property, oil retention capacity and emulsifying ability of polysaccharides in functional foods were greatly associated with the rheological properties of the final products and the mouth-feel experienced by consumers.19,33 Therefore, it is necessary to evaluate these functional properties of polysaccharides.
3.6.1 WHC. WHC represents the ability of a moist material to retain water when subjected to the external forces, and is generally used to evaluate the stability, texture, and sensory quality of sample in food industry. It was reported that dietary fiber containing high WHC can be used for functional components to play an important role in avoiding dehydration and improving the texture and viscosity of certain formula food.19,34 As shown in Table 4, the value of WHC of PBP was 0.42 ± 0.03 g water/g sample, which was slightly higher than that of PSBPs (0.38–0.40 g water/g sample), demonstrating that the selenized modification could not improve the hydration properties of blackcurrant polysaccharide. However, according to the studies of Wang et al.33 and Alpizar-Reyes et al.,34 the WHC of Rosa roxburghii Tratt. fruit polysaccharide and tamarind seed mucilage were separately 0.25 ± 0.04 and 0.22 ± 0.07 g water/g sample, which were much lower than that of PSBPs.
Table 4 The functional properties of PBP and PSBPsa
Sample |
Functional properties |
WHC (g g−1) |
OHC (g g−1) |
EAI (m2 g−1) |
ES (%) |
Means with different letters for the same species are significantly different (p < 0.05). |
PBP |
0.42 ± 0.03c |
1.15 ± 0.08a |
121.35 ± 1.32d |
87.75 ± 1.27d |
PSBP-1 |
0.40 ± 0.05b |
1.18 ± 0.04b |
92.98 ± 1.43c |
79.70 ± 1.39c |
PSBP-2 |
0.39 ± 0.02a,b |
1.19 ± 0.05b,c |
88.93 ± 1.88b |
67.64 ± 1.67b |
PSBP-3 |
0.38 ± 0.02a |
1.20 ± 0.10c |
69.77 ± 1.23e |
60.39 ± 1.12e |
Soy lecithin |
— |
— |
143.27 ± 1.49e |
94.85 ± 1.33e |
3.6.2 OHC. OHC reflects the oil absorption capacity of the dietary fiber, and it depends on the surface properties, overall charge density, thickness and the hydrophobic nature of the fibre particle.35,36 The polysaccharides with higher OHC can replace other edible gum products to help stabilize foods with a high percentage of lotions and fats. As observed in Table 4, OHC values of PSBPs (1.18–1.20 g oil/g sample) were higher than that of PBP (1.15 ± 0.08 g oil/g sample). Therefore, the selenized modification improved the OHC of blackcurrant polysaccharides to a certain extent. Furthermore, these OHC values of PSBPs were also higher than that of bean pod (0.28 g g−1), pea pod (0.13 g g−1) polysaccharides,36 native oat starches (0.29–0.34 g oil/g sample),37 and tamarind seed mucilage (0.07–0.13 g oil/g sample).34 Therefore, PSBPs with an adequate oil-holding capacity had potential applications in the stabilisation of high fat food products and emulsions, flavor retention and increasement of the taste of food.
3.6.3 Emulsifying property. Polysaccharide plays a prominent role in the formulation of food emulsions, and the sugar molecules, hydrophobic functional groups content, degree of branching and rheological characteristics are the key factors that affects the emulsifying property.33,38 As observed in Table 4, EAI of PBP was 121.35 ± 1.32 m2 g−1, which was higher than PSBP-1 (92.98 ± 1.43 m2 g−1), PSBP-2 (88.93 ± 1.88 m2 g−1) and PSBP-3 (69.77 ± 1.23 m2 g−1). Analogously, the ES of four polysaccharides decreased in the order: PBP > PSBP-1 > PSBP-2 > PSBP-3. From these data, selenized modification weakened the emulsifying property of the native polysaccharides. Considering the significant reduction of particle size after selenization, the possible reason may be that the markedly small molecular size of PSBPs contributed less to thickening the continuous phase. As a result, the energy created during the homogenization process could not be efficiently transferred to the oil phase.39,40
3.7. Effect of selenized modification on the antioxidant activities
3.7.1 Scavenging capacity on ABTS+˙. ABTS+˙ scavenging assay could reflect the electron or hydrogen donating ability of antioxidant, and has been broadly applied to assess the total antioxidant activity of natural product.5,12 As shown in Fig. 7A, both PSBPs and PBP exhibited obviously scavenging effects on ABTS+˙ in a dose-dependent manner. The IC50 values were determined as 1.79 mg mL−1 for PBP, 0.92 mg mL−1 for PSBP-1, 0.82 mg mL−1 for PSBP-2, 0.55 mg mL−1 for PSBP-3 and 0.031 mg mL−1 for VC, respectively. At the concentration of 1.0 mg mL−1, the scavenging rates of PBP, PSBP-1, PSBP-2 and PSBP-3 respectively reached to 38.62% ± 1.47%, 52.15% ± 0.82%, 56.03% ± 2.05% and 64.48% ± 3.27%. Compared the results, the scavenging effect of PSBPs against ABTS+˙ was much higher than that of PBP, which was consistent with previous findings that the selenized polysaccharides exhibit higher antioxidant activities than untreated polysaccharide.13,24 Simultaneously, ABTS+˙ scavenging capacity of PSBPs increased with the improvement of selenium content. These results demonstrated that selenium significantly affected the antioxidant activity of blackcurrant polysaccharides, and the introduced selenium groups could excite the hydrogen atom-donates in anomeric region.14,40
 |
| Fig. 7 ABTS+˙ (A), hydroxyl radical (B), and superoxide anion radical (C) scavenging activities of PBP and PSBPs. | |
3.7.2 Scavenging capacity on hydroxyl radical. Hydroxyl is the most aggressive radical among reactive oxygen species, which is mainly produced from mitochondrial electron transport system, and can unspecifically attack adjacent biomolecules, such as proteins, enzymes, and DNA, and cause oxidative damage to cells.2,24 Scavenging abilities of PSBPs and PBP on hydroxyl radicals were shown in Fig. 7B. All the samples exhibited the ability to scavenge hydroxyl radicals in a concentration-dependent fashion. The IC50 value of PBP, PSBP-1, PSBP-2, PSBP-3 and VC were 0.55, 0.49, 0.37, 0.28 and 0.053 mg mL−1, respectively. When the concentration was 1.0 mg mL−1, the scavenging rates of PSBP-1 (61.18 ± 0.66%), PSBP-2 (67.65 ± 0.53%) and PSBP-3 (82.06 ± 0.50%) were notably higher than that of PBP (57.65 ± 0.46%) (p < 0.05). Similarly, the selenized polysaccharides from Radix hedysari possessed the better scavenging capacity on hydroxyl radical than the native polysaccharide.15 The reason for this phenomenon may be that the selenium moiety in the PSBPs can complex the free metal ion, and thereby exerting the stronger antioxidant function on hydroxyl radicals.5,15
3.7.3 Scavenging capacity on superoxide anion radical. The superoxide anion radical (O2−˙) continuously produced by oxygen reduction is a widely distributed free radical in the human body.2,5 O2− radical can induce the oxidative degradation of the important cellular components, and further lead to the disorder and destruction of normal physiological process.2,16 As shown in Fig. 7C, PSBPs showed significantly higher scavenging activities than PBP (p < 0.05), and the scavenging capacity of PSBPs increased with increasing Se content. In addition, the IC50 values of PBP, PSBP-1, PSBP-2 and PSBP-3 were 1.08, 0.92, 0.75 and 0.47 mg mL−1, separately. These results suggested that selenized modification could significantly elevate the scavenging capacity of blackcurrant polysaccharides on superoxide anion radical. Similar conclusions also were reported by Zhu et al.17 and Wang et al.5In summary, the results of ABTS+, hydroxyl and superoxide anion radical scavenging assays above demonstrated that selenized modification is an effective method to enhance the antioxidant activity of blackcurrant polysaccharides. Moreover, the higher the selenium content was, the better the scavenging effect was. The selenium groups in the obtained selenized polysaccharide can activate the hydrogen atom of the anomeric carbon, and thereby contributing to the abilities scavenging free radicals.14,17,40 In addition, it has been reported that selenium as the cofactor of selenium dependent antioxidant enzymes can be transformed into the active center of these enzymes in vivo, such GSH-Px, TRx and SOD.40 Therefore, PSBPs in this study may increase the selenium-dependent antioxidant enzymes activities, and further display potent antioxidant activity in vivo.
3.8. Effects of selenized modification on inhibition of non-enzymatic glycation
Diabetes mellitus (DM) is a chronic metabolic disease that threatens the health of the global population. Many complications related to DM such as hyperlipidemia, atherosclerosis, cardiovascular diseases and renal failure prompt that is very urgent for its control.40 Non-enzymatic glycation generally includes three reaction phases. In the early phase, Amadori products are formed by the reaction between the reduced amino groups at the end of proteins and aldehyde groups on the reducing sugars. They are further oxidized, degraded, and rearranged, and generated the dicarbonyl compounds in the intermediate phase. In the late phase, dicarbonyl compounds react with free amino groups on proteins to form AGEs. Reducing the formation of AGEs can help prevent the occurrence and development of DM.10 Therefore, antiglycation assay can be used to evaluate the anti-diabetic potential of the polysaccharide.
As shown in Fig. 8A–C, all three kinds of selenized polysaccharide samples (PSBP-1, PSBP-2 and PSBP-3) displayed the stronger inhibitory activities against the non-enzymatic glycated products formation than PBP and AG at two test concentrations, and the inhibition rate at 0.5 mg mL−1 was higher than that at 0.1 mg mL−1. At the early phase, the inhibitory activities of PSBPs and PBP on Amadori products obviously increased from 1 to 13 days, and reached maximum values at the 15th day (Fig. 8A). The maximum inhibitory rates of PSBP-1, PSBP-2 and PSBP-3 at 0.5 mg mL−1 were 60.11 ± 1.72%, 66.12 ± 1.50% and 69.95 ± 0.38%, which increased 7.65%, 13.66% and 17.49% over that of PBP. As presented in Fig. 8B and C, with the increase of culture time, the inhibitory effect of PSBPs and PBP at two concentrations on the formation of dicarbonyl compounds and AGEs increased significantly. At the 15th day, PSBP-1, PSBP-2 and PSBP-3 at 0.5 mg mL−1 showed the maximum inhibitory rates of 57.54 ± 0.72%, 65.23 ± 1.17% and 67.08 ± 0.55% for dicarbonyl compounds and 64.58 ± 1.56%, 71.88 ± 0.46%, 73.29 ± 1.53% for AGEs, which were 1.09-, 1.24-, 1.27-fold and 1.03-, 1.15-, 1.17-fold higher than that of PBP (52.62 ± 0.49%) and (62.58 ± 1.15%), respectively. Obviously, the inhibitory effects of PSBPs at the late phase were more obvious than that at the early and intermediate phases, which was related to the inhibition of PSBPs on free radicals generation derived from the glycation process.10,23 In addition, at the same concentration, the inhibitory activities increased in the order: PSBP-3 > PSBP-2 > PSBP-1, indicating a positive effect of selenium content. Similarly, in comparison with native polysaccharides, the selenized polysaccharides from Momordica charantia L.4 and sweet potato40 can significantly reduce fasting blood glucose, MDA and LPO levels and increase glycogen and insulin contents to exert therapeutic effect for DM.
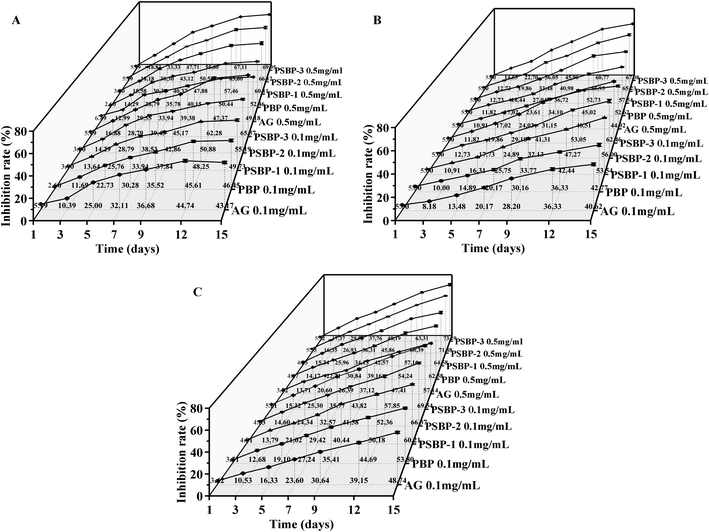 |
| Fig. 8 Inhibitory effects of PBP and PSBPs on the formation of Amadori product (A), dicarbonyl compound (B) and AGEs (C). | |
In this study, PSBPs have been confirmed to possess stronger free radical scavenging activities than PBP in vitro. Therefore, the better inhibitory effects of PSBPs on non-enzymatic glycation may be associated with the excellent radical scavenging activities. After selenized modification, the presence of selenium in PSBPs not only functions as an antioxidant agent but also prevents the progress of diabetes.
4. Conclusion
In the present study, the selenized blackcurrant polysaccharides (PSBPs) with different selenium contents were successfully prepared. Both PBP and PSBPs were non-crystalline substances, and PSBPs displayed the larger zeta potential and smaller particle size compared to PBP. In addition, PSBPs displayed better OHC than PBP, while selenized modification did not improve the WHC and emulsifying property of blackcurrant polysaccharides. Meanwhile, PSBPs exhibited potent radicals scavenging capacities and antiglycation activities. Therefore, selenized modification was an efficient method to improve the biological activities of blackcurrant polysaccharides. However, the precise chemical structures and the structure–effect relationship of PSBPs have to be further investigated in later study.
Conflicts of interest
The authors declare that there are no conflicts of interest.
Acknowledgements
This work was financially supported by National Natural Science Foundation of China (No. 31600276), Natural Science Foundation of Heilongjiang Province of China (No. C2018013), Postdoctoral Scientific Research Start-up Fund Projects of Heilongjiang Province of China (LBH-Q19010, LBH-Q17030) and Student's Innovation Training Program of Northeast Agricultural University (201910224076).
References
- Q. W. Liu, X. D. Ge, L. G. Chen, D. L. Cheng, Z. Yun, W. Xu and R. Shao, Int. J. Biol. Macromol., 2018, 107, 2262–2268 CrossRef CAS.
- Y. Q. Xu, X. J. Niu, N. Y. Liu, Y. K. Gao, L. B. Wang, G. Xu, X. G. Li and Y. Yang, Food Chem., 2018, 243, 26–35 CrossRef CAS.
- X. Y. Li, L. Wang and B. B. Wang, Food Chem., 2017, 229, 479–486 CrossRef CAS.
- Y. Ru, K. X. Liu, X. Y. Kong, X. Y. Li, X. R. Shi and H. M. Chen, Int. J. Biol. Macromol., 2020, 152, 295–304 CrossRef CAS.
- L. Wang, X. Y. Li and B. B. Wang, Int. J. Biol. Macromol., 2018, 120, 1362–1368 CrossRef CAS.
- S. H. Nile and S. W. Park, Nutrition, 2014, 30(2), 134–144 CrossRef CAS.
- M. M. Zhao, J. W. Bai, X. Y. Bu, Y. Tang, W. Q. Han, D. L. Li, L. B. Wang, Y. Yang and Y. Q. Xu, Food Control, 2020, 107449 Search PubMed.
- Y. Q. Xu, L. Zhang, Y. Yang, X. M. Song and Z. Y. Yu, Carbohydr. Polym., 2015, 117, 895–902 CrossRef CAS.
- R. Takata, T. Yanai, R. Yamamoto and T. Konno, Biosci., Biotechnol., Biochem., 2007, 71, 1342–1344 CrossRef CAS.
- Y. Q. Xu, Y. Y. Guo, Y. K. Gao, X. J. Niu, L. B. Wang, X. G. Li, H. C. Chen, Z. Y. Yu and Y. Yang, LWT--Food Sci. Technol., 2018, 93, 16–23 CrossRef CAS.
- Y. Q. Xu, F. Cai, Z. Y. Yu, L. Zhang, X. G. Li, Y. Yang and G. J. Liu, Food Chem., 2016, 194, 650–658 CrossRef CAS.
- Y. Yang, Z. X. Lei, M. M. Zhao, C. Z. Wu, L. B. Wang and Y. Q. Xu, Ind. Crops Prod., 2020, 147, 112249 CrossRef CAS.
- D. J. Chen, H. Y. Sun, Y. X. Shen, M. Luo, X. Xin and Z. M. Xu, J. Funct. Foods, 2019, 61, 103492 CrossRef CAS.
- P. Y. Gao, J. Bian, S. S. Xu, C. F. Liu, Y. Q. Sun, G. L. Zhang, D. Q. Li and X. G. Liu, Int. J. Biol. Macromol., 2020, 149, 207–214 CrossRef CAS.
- D. F. Wei, T. Chen, M. F. Yan, W. H. Zhao, F. Li, W. D. Cheng and L. X. Yuan, Carbohydr. Polym., 2015, 125, 161–168 CrossRef CAS.
- S. L. Qiu, J. Chen, X. Chen, Q. Fan, C. S. Zhang, D. Y. Wang, X. P. Li, X. Y. Chen, X. L. Chen and C. Liu, Carbohydr. Polym., 2014, 103, 148–153 CrossRef CAS.
- Z. Y. Zhu, F. Liu, H. Gao, H. Q. Sun, M. Meng and Y. M. Zhang, Int. J. Biol. Macromol., 2016, 93, 1090–1099 CrossRef CAS.
- L. Wang, Q. H. Huang, Y. F. Huang, J. H. Xie, C. Qu, J. P. Chen, L. Zheng, T. G. Yi, H. F. Zeng and H. L. Li, J. Funct. Foods, 2018, 46, 365–377 CrossRef CAS.
- Q. Q. Song, L. Jiang, X. Q. Yang, L. X. Huang, Y. Yu, Q. Yu, Y. Chen and J. H. Xie, Int. J. Biol. Macromol., 2019, 138, 874–880 CrossRef CAS.
- P. Muniandy, A. B. Shori and A. S. Baba, Food Packag. Shelf Life, 2016, 8, 1–8 CrossRef.
- K. B. Jeddou, F. Chaari, S. Maktouf, O. Nouri-Ellouz, C. B. Helbert and R. E. Ghorbel, Food Chem., 2016, 205, 97–105 CrossRef.
- M. B. Romdhane, A. Haddar, I. Ghazala, K. B. Jeddou, C. B. Helbert and S. Ellouz-Chaabouni, Food Chem., 2017, 216, 355–364 CrossRef.
- Y. Q. Xu, G. J. Liu, Z. Y. Yu, X. M. Song, X. G. Li and Y. Yang, Food Chem., 2016, 199, 694–701 CrossRef CAS.
- Y. B. Meng, Y. Y. Zhang, N. Jia, H. R. Qiao, M. H. Zhu, Q. H. Meng, Q. Lu and Y. G. Zu, Int. J. Biol. Macromol., 2018, 118, 1438–1448 CrossRef CAS.
- F. J. Mao, P. J. Shi, H. Chen, L. Song, Z. Y. Wang, C. Wu and M. Du, Food Chem., 2018, 254, 103–108 CrossRef CAS.
- M. Marchuk, M. J. Selig, G. B. Celli, P. Lawrence, D. M. Smilgies and A. Abbaspourrad, Food Hydrocolloids, 2019, 93, 226–234 CrossRef CAS.
- Q. Li, W. Wang, Y. Zhu, Y. Chen, W. J. Zhang, P. Yu, G. H. Mao, T. Zhao, W. W. Feng and L. Q. Yang, Carbohydr. Polym., 2017, 161, 42–52 CrossRef CAS.
- E. Malinowska, M. Klimaszewska, T. Strączek, K. Schneider, C. Kapusta, P. Podsadni, G. Łapienis, M. Dawidowski, J. Kleps and S. Górska, Carbohydr. Polym., 2018, 198, 407–417 CrossRef CAS.
- Q. Y. Song, Z. Y. Zhu, X. T. Wang, L. T. Chen and D. Y. Wang, J. Mol. Struct., 2019, 1178, 630–638 CrossRef CAS.
- F. Y. Kagimura, M. A. A. D. Cunha, T. V. Theis, C. R. M. Malfatti, R. F. H. Dekker, A. M. Barbosa, S. D. Teixeira and K. Salomé, Carbohydr. Polym., 2015, 127, 390–399 CrossRef CAS.
- R. B. A. Kolsi, H. B. Salah, N. Jardak, R. Chaaben, I. Jribi, A. E. Feki, T. Rebai, K. Jamoussi, N. Allouche and C. Blecker, Carbohydr. Polym., 2017, 170, 148–159 CrossRef.
- M. Z. Sun, Y. M. Li, T. X. Wang, Y. W. Sun, X. Y. Xu and Z. S. Zhang, Carbohydr. Polym., 2018, 184, 127–134 CrossRef CAS.
- L. Wang, B. Zhang, J. Xiao, Q. Huang, C. Li and X. Fu, Food Chem., 2018, 249, 127–135 CrossRef CAS.
- E. Alpizar-Reyes, H. Carrillo-Navas, R. Gallardo-Rivera, V. Varela-Guerrero, J. Alvarez-Ramirez and C. Pérez-Alonso, J. Food Eng., 2017, 209, 68–75 CrossRef CAS.
- M. Elleuch, D. Bedigian, O. Roiseux, S. Besbes, C. Blecker and H. Attia, Food Chem., 2010, 124(2), 411–421 CrossRef.
- I. Mateos-Aparicio, A. Redondo-Cuenca and M. J. Villanueva-Suárez, Food Chem., 2010, 122(1), 339–345 CrossRef CAS.
- A. Shah, F. A. Masoodi, A. Gani and B. A. Ashwar, LWT--Food Sci. Technol., 2017, 80, 19–26 CrossRef CAS.
- X. X. Liu, Y. Y. Yan, H. M. Liu, X. D. Wang and G. Y. Qin, LWT--Food Sci. Technol., 2019, 111, 242–251 CrossRef CAS.
- C. Ai, H. C. Meng, J. W. Lin, T. Zhang and X. M. Guo, Food Hydrocolloids, 2020, 109, 106049 CrossRef CAS.
- L. Z. Cheng, Y. F. Wang, X. X. He and X. L. Wei, Int. J. Biol. Macromol., 2018, 120, 82–92 CrossRef CAS.
Footnote |
† Two authors contributed equally to this work. |
|
This journal is © The Royal Society of Chemistry 2020 |
Click here to see how this site uses Cookies. View our privacy policy here.