DOI:
10.1039/D0RA07658A
(Paper)
RSC Adv., 2020,
10, 37080-37085
One-step-synthesized D-gluconic acetal-based supramolecular organogelators with effective phase-selective gelation†
Received
7th September 2020
, Accepted 2nd October 2020
First published on 8th October 2020
Abstract
Two effective and one-step-synthesized organogelators based on D-gluconic acetal derivatives have been developed to show phase-selective gelation behaviours towards aromatic solvents from their biphasic mixtures with water. The dominant factors that drive gelation have been studied using FT-IR and temperature-dependent 1H NMR spectroscopy. Particularly, gelator GAA-2 in powder form could selectively congeal toluene, benzene and o-xylene at room temperature under mild stirring. Additionally, GAA-2 could gelate the aromatic solvents within 10 min and the recovery rate of the aromatic solvents could reach about 82% under a certain condition. The benefits of wide source availability, being easy to synthesize, and recyclable performance of the gelator make GAA-2 ideal for real-world remediation of aromatic solvents.
Introduction
With the rapid development of industry and the increasing demand for substances in people's daily lives, the pollution of freshwater arising from organic solvent pollutants such as grease, aliphatic hydrocarbons, and aromatic hydrocarbons has become a serious environmental and economic problem, prompting people to develop advanced functional materials to deal with this situation.1–4 In recent years, the phase-selective supramolecular gelators (PSSGs) have attracted much attention because they could selectively transform organic solvent phases into gels from the organic solvent/water two-phase systems. The obtained gel would realize the reversible phase transition of gel–sol under certain external stimuli (such as heating, mechanical shearing, etc.), which was beneficial to the recycling of gelators and organic solvent.5–7 Therefore, many scientists have been committed to finding suitable PSSGs for application research in organic solvent pollution control.8–10 The current self-assembly process of PSSGs needed to be achieved by heating–cooling, ultrasonic and mechanical stirring methods.11–14 It was obvious that heating–cooling and ultrasonic operation were not feasible in practical situations from the perspective of technical feasibility. In addition, the co-solvent could also be used to dissolve PSSGs in advance, and then evenly dispersed into the organic phase to form a gel.15–19 However, this method always introduces toxic water-soluble solvents (such as tetrahydrofuran, acetonitrile), which may cause secondary pollution and limit the application of PSSGs in practice. Therefore, it is necessary to formulate a more effective strategy to solve the above problems.
An ideal alternative is to spray solid powder PSSGs directly on the surface of the organic phase, and then form a room-temperature gel, which has the advantages of simple operation, safety and environmental protection.20–24 For example, K. M. Sureshan et al.25 synthesized a series of glucose-based PSSGs that could be used to congeal crude oils from the crude oil/water mixed system in powder form. J. R. Li et al.26 prepared a phase-selective organogelator based on diurea, and they claimed that the solid powder of this PSSG could selectively transform gasoline into gels from the gasoline/water mixing system. However, the above-mentioned PSSGs exhibited quite slow gelation time (3 h and 5 h, respectively), which limited their wide application in the actual pollution control. Recently, H. Q. Zeng et al.27 reported a wetting powdered PSSGs which could effectively decrease the gelation time, but co-solvent acetonitrile might still leak into the water body if they were employed on a large scale. H. T. Yu28 and J. Song29 found that the formation of the gel could be effectively accelerated by increasing the amount of powdered PSSGs, but the cost was relatively high. Therefore, the development of the more economical and environmentally friendly PSOGs that can be directly applied in solid form and has excellent performance still remains a major challenge and is in great requirement.
Previously, we have designed a simple phase-selective gelator based on a sorbitol acetal compound that exhibited excellent gelation ability in nonpolar solvents and could selectively congeal the gasoline layer in gasoline–water mixture by adding the co-solvent THF. However, it still failed to gel the crude oils in powder form. Based on this, we herein used inexpensive gluconic acid as raw material to synthesize two gluconic acid acetal compounds in one step, which combined aromatic ring structures with different substituents and polyhydroxy groups. They are expected to be effective PSSGs for the treatment of aromatics solvent contaminants in water body.
Result and discussion
Synthesis and gelation behaviours of the gelators
Firstly, the target gelators could be easily synthesized in one step outlined in Scheme 1. It was worth noting that D-gluconic acid as a raw material (commercially available) reacted with 1 equivalent of the corresponding aldehyde to obtain acetals GAA-1 and GAA-2, respectively. The gelation behaviors of compounds 1 in various solvents was tested by heating–cooling method (Table 1). Obviously, GAA-2 showed gelation ability in eight organic liquids, including various alcohols (n-butanol and n-octanol), aromatic solvents (chlorobenzene, nitrobenzene, toluene, o-xylene and benzene) and acetonitrile, but GAA-1 displayed gelation behaviors just in five of them. In addition, the critical gel concentration (CGC) values for toluene and o-xylene were lower than 0.1 wt%, elevating gelator GAA-2 to the category of supergelator.15 Moreover, the gelation ability would not change even after multiple repetitions of the sol–gel cycles, indicating that these gels had complete thermal reversibility. Then all the obtained gels could remain stable at room temperature and no obvious changes were observed when stored at room temperature for 2 months, demonstrating the stability of the gels.
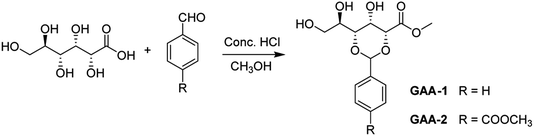 |
| Scheme 1 The synthetic route of gelators GAA-1 and GAA-2. | |
Table 1 Gelation ability results of gelator 1 in various solventsa
Solvents |
GAA-1 |
GAA-2 |
State |
CGC (wt%) |
Tgel (°C) |
State |
CGC (wt%) |
Tgel (°C) |
These tests were performed by adding 30 mg of 1 into 1 mL of a known liquid and then heating the tube until the gelators were dissolved or could not be dissolved ever. A “stable to inversion test tube method” was adopted. I: insoluble, OG: opaque gel, TG: transparent gel. Each experiment was done in duplicate. |
Hexane |
I |
— |
— |
I |
— |
— |
Cyclohexane |
I |
— |
— |
I |
— |
— |
n-Octane |
I |
— |
— |
I |
— |
— |
Kerosene |
I |
— |
— |
I |
— |
— |
Gasoline |
I |
— |
— |
I |
— |
— |
Acetonitrile |
P |
— |
— |
OG |
0.19 |
52 |
Chlorobenzene |
TG |
0.11 |
78 |
TG |
0.10 |
75 |
Nitrobenzene |
S |
— |
— |
TG |
0.15 |
81 |
Toluene |
TG |
0.16 |
86 |
TG |
0.09 |
89 |
o-Xylene |
TG |
0.11 |
91 |
TG |
0.07 |
99 |
p-Xylene |
TG |
0.09 |
94 |
TG |
0.06 |
102 |
m-Xylene |
TG |
0.13 |
89 |
TG |
0.09 |
95 |
Benzene |
TG |
0.19 |
66 |
TG |
0.16 |
72 |
Characterization of the gels
To shed light on the self-assembly mechanism of the gelators, we took the gelator GAA-2 as an example for FT-IR investigations (Fig. 1a). In the chloroform solution, the stretching band of OH was observed at 3489 cm−1, whereas it shifted to 3419 cm−1 in the acetonitrile xerogel, indicating that the OH groups were involved in the hydrogen bonding. The stretching vibration peaks of C
C and C–O–C from the methyl ester group appeared at 1724, 1147 cm−1, the corresponding bands of the xerogel appeared at 1726, 1147 cm−1, respectively. These data indicated that the methyl ester groups were involved in the hydrogen bonding. In order to further study the interaction force between the gelators, a temperature-dependent 1H NMR spectroscopy study was performed in acetonitrile (Fig. 1c), and it was found that as the temperature increased from 20 °C to 60 °C, the chemical shift of the hydroxyl proton moved to a high field, which further proves that hydroxyl protons participate in intermolecular hydrogen bonding in the gel phase.4 At the same time, the signals of the aromatic protons at 8.09 (Ha) and 7.68 ppm shifted slightly to the high field, to 8.02 and 7.72 ppm, respectively, indicating that the π–π stacking effect is slightly changed because the compound is still in the gel state.24 The proposed π–π interactions (J-aggregation) can lead to the red shift in the absorption features of the gelator GAA-2 in solutions with high gelator concentrations as compared with those with low concentrations (Fig. S5†). The XRD pattern of acetonitrile xerogel (Fig. 1b) shows that the d-spacing values are 1.65, 0.82 and 0.55 nm, and the ratio is 1
:
1/2
:
1/3, indicating a layered arrangement.21 In addition, scanning electron microscope (SEM) revealed the morphologies of the xerogels prepared from different solvents of GAA-2, and well-defined three-dimensional (3D) networks intertwined by fibrous structure can be observed (Fig. 2). These results indicate that the synergistic effect of hydrogen bonding and π–π stacking was the driving forces for the self-assembly of GAA-2.
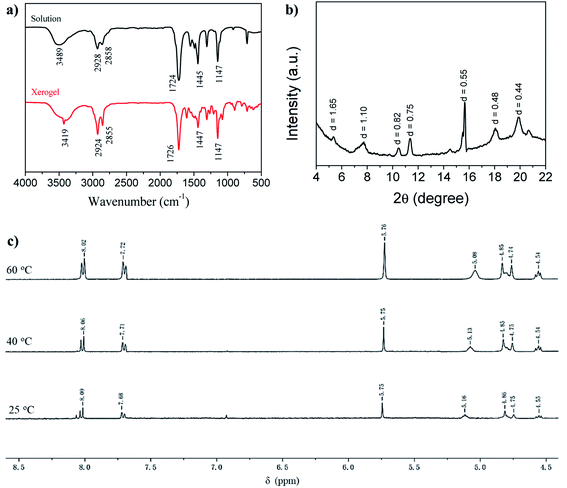 |
| Fig. 1 (a) FTIR spectra of solution (0.1 mM) and xerogel (1.0% w/v) of GAA-2 in acetonitrile. (b) WXRD spectra of GAA-2 xerogels made from acetonitrile. (c) Change in 1H NMR chemical shift of hydroxyl and aromatic protons of gelator GAA-2 in CD3CN at different temperatures. | |
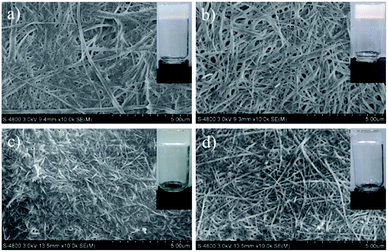 |
| Fig. 2 SEM images of GAA-2 xerogels obtained from (a) n-butanol, (b) acetonitrile, (c) toluene, (d) o-xylene. | |
Phase-selective gelation performances in gelator powder form
Then, we explored the applications of gelators in the fields of room-temperature phase-selective gelation. To our surprise, gelator GAA-2 has been found to be able to congeal selectively benzene, toluene and xylene, from their water mixtures (0.5 mL/2 mL) with a gentle agitation (Table S1†), whereas GAA-1 fails to have such behaviours. Furthermore, we carried out the experiment to simulate aromatic solvents recovery in large scale: when 500 mg of GAA-2 powder was sprinkled into the toluene/water mixture (20 mL/200 mL), after being gently stirred at room temperature for 65 min, a toluene gel was formed which could be easily skimmed off with a net spoon to achieve separation (Fig. 3). Next, toluene could be separated from the gel via simple distillation with a recovery of 75% by weight, and the gelator was left in the flask with a recovery of 85% which could also be reused. The phase-selective gelation ability could slow down the diffusion of solvents in water, indicating its potential application in the recovery of actual aromatic solvents.
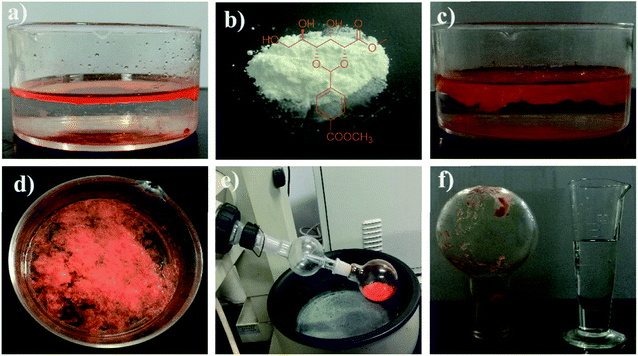 |
| Fig. 3 Demonstration of aromatic solvents recovery. (a) Biphasic system was prepared by adding 20 mL of toluene (dyed with Methyl Red) into 200 mL of water. (b) Powders of GAA-2. (c) 500 mg of gelator powders were dispersed over the solvent surface after being gently stirred at room temperature for 65 min. (d) Formation of a stable gel. (e) Distillation of toluene extracted from the gel. (f) Toluene was recycled from water by GAA-2. | |
Given that the amount of the gelator would directly affect its gelation behavior, it was necessary to study the effect of the dosage of the gelator on the gelation time and the recovery rate of the aromatic solvents. In the experiment, the gelation time means the time for the solution turns into a semi-solid that can be easily removed from water. As shown in Fig. 4a, with the increase in the gelator amount, the gelation time gradually decreases. Moreover, upon the addition of 20 mg of gelator GAA-2, the gelation time could be reduced to less than 10 min. In order to calculate the recovery rate, the separated gel was subjected to distillation to recycle the aromatic solvents. It has been found that the recovery rate undergo an enhancement with the increase in the gelator amount, as shown in Fig. 4b. In addition, upon the addition of 20 mg of gelator GAA-2, the recovery rates of benzene, toluene and o-xylene could reach about 82%. More interestingly, these gels with such a dosage of gelator had excellent mechanical strength, which was implied by its rheological data. For all the gel samples, the storage modulus (G′) exhibited much higher values than the loss modulus (G′′) in the entire range of frequency sweep, suggesting their gel nature (Fig. 4c). In addition, the high G′ values between 2 × 104–7.5 × 104 Pa explained the excellent mechanical strength. More importantly, the yield stress values of all the gels were more than 100 Pa, which favours the ability of the gels to withstand high pressure (Fig. 4d and S6†). We then explored the influence of water surface waves on the stability of the thin gel films. The cylindrical gel samples with the same size (10 cm in diameter, 0.2 cm in height) was deposited on the surface of a water bath and the shaking amplitude was slowly increased at a constant frequency until the gel layer broke (Table S2†). For the 4 wt% GAA-2 in the benzene gel, the amplitude of the waves at which the layer was broken was 0.86 mm at 10 Hz, 0.49 mm at 20 Hz and 0.07 mm at 30 Hz, respectively. The breaking amplitude was increased for toluene gel and o-xylene gel, indicating that these gels had higher resistance to water waves compared with benzene gel, which was consistent with the above results of mechanical strength. Once again, no droplets were observed at the breaking amplitude, and the gel film was irreversibly broken into large fragments, which remained on the water surface and could continue to be salvaged. Moreover, the temperature-consecutive frequency spectra for benzene gel, toluene gel and o-xylene gel with 3 wt% GAA-2 were provided in Fig. S7–S9† and demonstrated that an increase in temperature promoted a systematic reduction in G′ and G′′ over the entire ω range examined. These spectra were then shifted along the frequency axis until they aligned to generate master curves. As shown in the right panels of Fig. S7–S9,† the well-behaved master curves mean that these gels exhibit linear viscoelasticity over a wide frequency range.30,31 Therefore, the above results indicated that gelator GAA-2 could be an efficient and sustainable material for the recovery of aromatic solvents from their water mixtures.
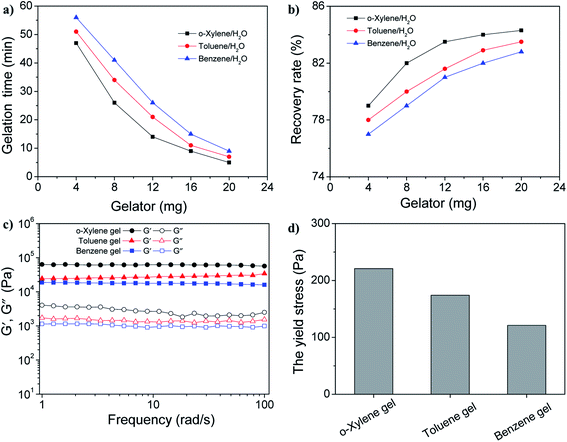 |
| Fig. 4 (a) The gelation time and (b) the recovery rates of gelator GAA-2 in aromatics solvents/water ratios of 1 : 100 (0.1 mL : 10 mL) upon the addition of different amounts of GAA-2. Rheological studies of gels formed by spreading 30 mg of gelator GAA-2 powders over the crude oil surface (0.1 mL) in 10 mL of water. (c) Frequency sweep. G′ = storage modulus, G′′ = loss modulus. (d) The yield stress of gelator GAA-2 in different aromatics solvents. | |
Conclusions
In conclusion, we report two one-step-synthesized organogelators based on the D-gluconic acetal derivatives, which exhibited phase-selective gelation for aromatics solvents. Particularly, GAA-2 can congeal benzene, toluene and o-xylene in powder form at room temperature under mild stirring. Systematic experiments indicate that the gelation time can be realized within 10 min and the recovery rate of the aromatic solvents can reach about 82% under a certain condition. Besides, the discovery of the PSSGs with the features of wide source, easy to be synthetic, and recyclable performance, lead us to propose gelator GAA-2 to the real practical use of powdery room-temperature gelators for the treatment of aromatics solvents recovery.
Conflicts of interest
There are no conflicts to declare.
Acknowledgements
This research was supported by the National Natural Science Foundation of China (No. 21606211 and 21671178), Foundation of He’nan Educational Committee (21A530009), Scientific Research Foundation for the Doctoral Program (No. 2014BSJJ061) and Space Star Incubation Project (No. 2019ZCKJ212).
Notes and references
- B. O. Okesola and D. K. Smith, Applying low-molecular weight supramolecular gelators in an environmental setting-selfassembled gels as smart materials for pollutant removal, Chem. Soc. Rev., 2016, 45(15), 4226–4251 RSC.
- C. Narayana, P. Kumari, G. Tiwari and R. Sagar, Triazole linked N-acetylglucosamine based gelators for crude oil separation and dye removal, Langmuir, 2019, 35(51), 16803–16812 CrossRef CAS.
- K. Fan, Y. Ma, X. Wang, S. Yu, G. Han, Z. Yin and J. Song, Sorbitol-based supramolecular organogelators with effective phase-selective gelation and significant self-healing property, Soft Mater., 2018, 16(1), 1–6 CrossRef CAS.
- X. Guan, K. Fan, T. Gao, A. Ma, B. Zhang and J. Song, A novel multi-stimuli responsive gelator based on D-gluconic acetal and its potential applications, Chem. Commun., 2016, 52(5), 962–965 RSC.
- J. Li, H. Wei, Y. Peng, L. Geng, L. Zhu, X.-Y. Cao, C.-S. Liu and H. Pang, A multifunctional self-healing G-PyB/KCl hydrogel: smart conductive, rapid room-temperature phase-selective gelation, and ultrasensitive detection of alpha-fetoprotein, Chem. Commun., 2019, 55(55), 7922–7925 RSC.
- S. R. Chowdhury, S. K. Nandi, D. Podder and D. Haldar, conformational heterogeneity and self-assembly of α,β,γ-hybrid peptides containing fenamic acid: multistimuli-responsive phase-selective gelation, ACS Omega, 2020, 5(5), 2287–2294 CrossRef.
- X. Ran, Y. J. Li, Q. Q. Gao, W. H. Qiu and L. J. Guo, A smart phase-selective gelator for recycling aromatic solvents, the removal of toxic dyes, and molecular delivery, Asian J. Org. Chem., 2017, 6(1), 95–101 CrossRef CAS.
- Rajkamal, D. Chatterjee, A. Paul, S. Banerjee and S. Yadav, Enantiomeric organogelators from D-/L-arabinose for phase selective gelation of crude oil and their gel as a photochemical micro-reactor, Chem. Commun., 2014, 50(81), 12131–12134 RSC.
- S. Bhattacharya and Y. Krishnan-Ghosh, First report of phase selective gelation of oil from oil/water mixtures. Possible implications toward containing oil spills, Chem. Commun., 2001,(2), 185–186 RSC.
- S. R. Jadhav, P. K. Vemula, R. Kumar, S. R. Raghavan and G. John, Sugar-derived phase-selective molecular gelators as model solidifiers for oil spills, Angew. Chem. Int. Ed., 2010, 49(42), 7695–7698 CrossRef CAS.
- K. Fan, X. Wang, H. Yang, L. Gao, G. Han, L. Zhou and S. Fang, Semiquantitative naked-eye detection of Cu(ii) with a standard colorimetric card via a hydrogel-coated paper sensor, Anal. Methods, 2020, 12(12), 1561–1566 RSC.
- K. Fan, X. Wang, Y. Ma, Y. Li, G. Han, Z. Yin and J. Song, Water-soluble lanthanide coordination polymers particles with white-light emission and color tuning, RSC Adv., 2019, 9(55), 32137–32140 RSC.
- Y. P. Zhang, Y. Ma, M. Y. Deng, H. X. Shang, C. S. Liang and S. M. Jiang, An efficient phase-selective gelator for aromatic solvents recovery based on a cyanostilbene amide derivative, Soft Matter, 2015, 11(25), 5095–5100 RSC.
- D. Wang, J. Niu, Z. G. Wang and J. Jin, Monoglyceride-based organogelator for broad-range oil uptake with high capacity, Langmuir, 2015, 31(5), 1670–1674 CrossRef CAS.
- K. Fan, X. Wang, S. Yu, G. Han, D. Xu, L. Zhou and J. Song, A chitosan-based fluorescent hydrogel for selective detection of Fe2+ ions in gel-to-sol mode and turn-off fluorescence mode, Polym. Chem., 2019, 10(37), 5037–5043 RSC.
- T. Su, K. H. Hong, W. Zhang, F. Li, Q. Li, F. Yu, G. Luo, H. Gao and Y. P. He, Scaleable two-component gelator from phthalic acid derivatives and primary alkyl amines: acid-base interaction in the cooperative assembly, Soft Matter, 2017, 13(22), 4066–4073 RSC.
- A. Prathap and K. M. Sureshan, A mannitol based phase selective supergelator offers a simple, viable and greener method to combat marine oil spills, Chem. Commun., 2012, 48(43), 5250–5252 RSC.
- M. Xue, D. Gao, K. Liu, J. Peng and Y. Fang, Cholesteryl derivatives as phase-selective gelators at room temperature, Tetrahedron, 2009, 65(17), 3369–3377 CrossRef CAS.
- X. Zhang, J. F. Song, W. Ji, N. Xu, N. Gao, X. H. Zhang and H. T. Yu, Phase-selective gelators based on closed-chain glucose derivatives: their applications in the removal of dissolved aniline/nitrobenzene, and toxic dyes from contaminated water, J. Mater. Chem. A, 2015, 3(37), 18953–18962 RSC.
- K. Soundarajan and T. M. Das, Sugar-benzohydrazide based phase selective gelators for marine oil spill recovery and removal of dye from polluted water, Carbohydr. Res., 2019, 481, 60–66 CrossRef CAS.
- A. M. Vibhute, V. Muvvala and K. M. Sureshan, A Sugar-based gelator for marine oil-spill recovery, Angew. Chem., Int. Ed., 2016, 5, 7782–7785 CrossRef.
- A. Prathap and K. M. Sureshan, Sugar-based Organogelators for Various Applications, Langmuir, 2019, 35, 6005–6014 CrossRef CAS.
- L. Liao, X. Zhong, X. Jia, C. Liao, J. Zhong, S. Ding, C. Chen, S. Hong and X. Luo, Supramolecular organogels fabricated with dicarboxylic acids and primary alkyl amines: controllable self-assembled structures, RSC Adv., 2020, 10, 29129–29138 RSC.
- N. P. Pathak, Rajkamal and S. Yadav, A gelator–starch blend for dry powder based instant solidification of crude oil at room temperature, Chem. Commun., 2020, 56, 2999–3002 RSC.
- A. M. Vibhute, V. Muvvala and K. M. Sureshan, A sugar-based gelator for marine oil-spill recovery, Angew. Chem. Int. Ed., 2016, 55(27), 7782–7785 CrossRef CAS.
- H. Yao, L. P. Yang, Z. F. He, J. R. Li and W. Jiang, A phase-selective, bis-urea organogelator with a curved bis-naphthalene core, Chin. Chem. Lett., 2017, 28(4), 782–786 CrossRef CAS.
- C. L. Ren, J. Shen, F. Chen and H. Q. Zeng, Rapid room-temperature gelation of crude oils by a wetted powder gelator, Angew. Chem., 2017, 129(14), 3905–3909 CrossRef.
- X. Zhang, R. Dai, H. Sun, Y. T. Zhang, D. Liu, M. Wang, M. M. Sun and H. T. Yu, Mandelic acid-derived organogelators: applications of their solid form in rapid and efficient remediation of marine oil spills, Mater. Chem. Front., 2020, 4(1), 222–230 RSC.
- B. H. Zhang, S. P. Chen, H. Luo, B. Zhang, F. M. Wang and J. Song, Porous amorphous powder form phase-selective organogelator for rapid recovery of leaked aromatics and spilled oils, J. Hazard. Mater., 2020, 384, 121460 CrossRef CAS.
- T. Shikata, T. Nishida, B. Isare, M. Linares, R. Lazzaroni and L. Bouteiller, Structure and dynamics of a bisurea-based supramolecular polymer in n-dodecane, J. Phys. Chem. B, 2008, 112, 8459–8465 CrossRef CAS.
- E. A. Wilder, C. K. Hall, S. A. Khan and R. J. Spontak, Effects of composition and matrix polarity on network development in organogels of poly(ethylene glycol) and dibenzylidene sorbitol, Langmuir, 2003, 19, 6004–6013 CrossRef CAS.
Footnote |
† Electronic supplementary information (ESI) available. See DOI: 10.1039/d0ra07658a |
|
This journal is © The Royal Society of Chemistry 2020 |
Click here to see how this site uses Cookies. View our privacy policy here.