DOI:
10.1039/D0RA07773A
(Paper)
RSC Adv., 2020,
10, 39814-39821
New strategy for synthesis of hydroxyl-terminated poly(3-hexylthiophene) and its block copolymer using an η3-π-allyl hydroxyl-functionalized Ni(I) initiator†
Received
10th September 2020
, Accepted 23rd October 2020
First published on 1st November 2020
Abstract
A novel hydroxyl-terminated π-allyl nickel initiator [η3-Ni(CH2CHCHCH2OCOCH3)][BPhF4] is synthesized for the first time. 1H-NMR analysis confirms that the Ni initiator possesses a typical π-allyl structure with a protected hydroxyl group. The Ni initiator is applied to synthesize hydroxyl-terminated poly(3-hexylthiophene). It successfully initiates the homopolymerization of thiophene, and yields narrow molecular weight distribution, hydroxyl-terminated poly(3-hexylthiophene). Moreover, it is found that [η3-Ni(CH2CHCHCH2OCOCH3)][BPhF4] has high initiating activity in the polymerization of styrene and butadiene. A hydroxyl-terminated polybutadiene-b-poly(3-hexylthiophene) block copolymer is synthesized by using [η3-Ni(CH2CHCHCH2OCOCH3)][BPhF4] to improve the workability and brittleness of poly(3-hexylthiophene).
1. Introduction
Poly(3-hexylthiophene) (P3HT) and its derivatives have been widely studied owing to their excellent thermal and environmental stability, high conductivity in doped form, and feasibility of adjusting their optical and electronic characteristics by structural modification.1
Hydroxyl-terminated poly(3-hexylthiophene) and its block copolymers have a wide range of applications in engineering plastics and heat convertible resins. Monnaie et al.2 synthesized a series of end-group functionalized P3HTs, these functional end-groups were used to prepare hybrid materials from a broad variety of nanoparticles. Secondary modification of such polymers affords block, or star-shaped polymers with various functional groups that show excellent performance. In addition, functional group-terminated polymers can also be cross-linked to afford thermosetting resins.
Living Ni-catalyzed Kumada catalyst-transfer polycondensation (KCTP), based on the milestone discovery by Yokozawa,3 is usually used to prepare well-defined regioregular P3HT homopolymers.
However, unlike semiconductor block copolymers, the homopolymer of P3HT cannot control its morphology through the self-assembly approach. Hence, a series of block copolymers of P3HT, including P3HT-b-poly(dimethylsiloxane),4 P3HT-b-poly(pyridiniumphenylene),5 P3HT-b-polylactide,6 P3HT-b-poly(alkylacrylate),7 and P3HT-b-polystyrene,8 i.e.9–16 have been synthesized. Although, P3HT has advantages in many fields, it is a solid powder that hard to be dissolved or be melted, hence, processing properties of P3HT are poor. Moreover, conjugation of P3HT lead to poor compatibility with many kinds of general-purpose plastics and rubber, which means that it is difficult to exhibit advantages of P3HT by using simple physical blending. Therefore, linking P3HT and rubber substrate by means of block copolymerization and introducing reactive group (such as hydroxyl) at the end of macromolecule make it possible to curing with the crosslinking agent (such as isocyanate). P3HT segment will be existed in substrate with the form of microphase separation after curing, which simultaneously solve the brittleness defect of P3HT and the dispersion problem between P3HT and substrate. Moreover, block copolymer containing poly(3-hexylthiophene) can form a highly ordered in film. The accumulation of structures enhanced charge separation interface area, and provided effective charge transfer. Hydroxyl-terminated polybutadiene (HTPB) is an important liquid rubber with high transparency, low viscosity. Because of its excellent physical properties, such as water proof; acid and alkali resistance; anti-abrasion; marked low temperature mechanics and strong electrical insulation, HTPB was widely used as the special coatings and functional adhesives.17 Thus, it is significative to synthesis hydroxyl-terminated PB-b-P3HT block copolymer.
Nevertheless, existing strategy does not afford an end-functionalized block copolymer, and the synthesized polymers cannot be further modified or crosslinked.
Therefore, it is significant to design a novel Ni initiator that not only furnishes functional group-terminated P3HT but also is applicable to the preparation of a block copolymer of various monomers. In other words, the novel initiator must have a functional group in its structure and be applicable to a wide variety of other monomers.
Therefore, we designed and synthesized a novel π-allyl nickel initiator containing protected hydroxyl. It have the following characteristics:
(i) It is a single component initiator and can be conveniently applied to study the reaction mechanism.
(ii) A protected hydroxyl group is introduced into its molecular structure to obtain the hydroxyl-terminated polymer.
(iii) It can be widely applied to various monomers because of the π-allyl metal structure.
In this study, the initiator was used in the polymerization of 2,5-dibromothiophene to synthesize hydroxyl-terminated poly(3-hexylthiophene). Moreover, two typical monomers (styrene, and butadiene) were used as model compounds to verify the reaction mechanism for universality of functional group-terminated polymers, in which the polybutadiene(PB)-b-P3HT block copolymer was design to verify the advantage of hydroxyl-functionalized π-allyl nickel initiator in synthesis of block copolymers containing P3HT. Moreover, hydroxyl-terminated PB-b-P3HT also can improve the fragility and workability and can be secondary modification and crosslinking to obtained electric elastomer.
2. Materials and methods
Materials
Bis(1,5-cyclooctadiene)nickel(0) (96%, Aladdin); 2-butene-1,4-dioldiacetate; (99%, Aladdin), butadiene (15 wt% in toluene, TCI Chemicals); styrene (AR, Acros); 2,5-dibromo-3-hexylthiophene, (97%, Aladdin); 3-iodopropanol (99%, Aladdin), isopropylmagnesium chloride solution (2 mol L−1 in THF, Aladdin); triphenylphosphine (99%, Aladdin); sodium methylate (30 wt% in methanol, Aladdin); diethyl ether (AR, Aladdin); n-hexane (AR, Aladdin); tetrahydrofuran (THF, AR, Aladdin); sodium tetrakis[3,5-bis(trifluoromethyl)phenyl]borate (99%, Aladdin).
General methods
All solvents and styrene monomer were purified by distillation in the presence of calcium hydride, and then stored with molecular sieves.
The molecular weight and molecular weight distribution of polymer were measured by gel permeation chromatography (GPC) using a DAWN EOS type gel osmotic chromatography-polygon laser light scattering (SEC-MALL). The THF was used as the eluent; polymer concentration in eluent was 1 mg mL−1; flowrate is 0.5 mL min−1; temperature was 25 °C. For block copolymer structure analyzed, the crude product of block copolymer was washed by acetone and n-hexane to remove homogeneous polymers, and then the product was refined repeatedly by fractional precipitation thought mixed solvent of acetone and n-hexane until the GPC chromatogram is unimodal, which can guarantee the purity of block copolymer. Then the refined product was diluted in several gradient concentration to measure dn/dc of block copolymer. Finally, molecular weight data can be measured. The 1H NMR analyses were performed on a Bruker Avance-400 NMR (German Bruker); using CDCl3 as solvent and tetramethylsilane (TMS) as an internal standard; instrument conditions: magnetic field intensity is 9.4 T; frequency was 400 MHz; scan times was 16; work temperature was 25 °C. Scanning electron microscopy (SEM) images were obtained using a FEI. Quanta 200 SEM at 25 kV under high vacuum conditions. A Mettler-Toledo STARe equipment was used to evaluate the glass transition of polymer by differential scanning calorimetry (DSC). Procedure: first stage is room temperature to 200 °C, heating rate 20 °C min−1, keep 200 °C for 10 min; second stage is 200 °C to −130 °C, cooling rate 10 °C min−1, keep −130 °C for 20 min; third stage is −130 °C to 200 °C, heating rate 5 °C min−1. Mettler-Toledo Q50 is used in the test of thermogravimetric analysis (TGA), 25 °C to 600 °C, temperature rate is 10 °C min−1.
Synthesis of [η3-Ni(CH2CHCHCH2OCOCH3)][BPhF4]
Bis(1,5-cyclooctadiene)nickel(0) (55 mg, 0.2 mmol) was added to a dry flask under argon atmosphere and then diethyl ether (1 mL) was added slowly under argon atmosphere, as well. After cooling the system to 0 °C, 2-butene-1,4-dioldiacetate (34 mg, 0.2 mmol dissolved in 2 mL diethyl ether) was added dropwise. The solution was stirred at 0 °C for 30 min and the color gradually changed from yellow to red. Then sodium tetrakis[3,5-bis(trifluoromethyl)phenyl]borate (177.2 mg, 0.2 mmol) was added to the system at 0 °C and the solution was stirred for another 30 min. Finally, the diethyl ether was removed under vacuum and the mixture was washed with n-hexane under argon atmosphere. A yellow-orange solid was obtained with yield of 53%. More detail of synthesis can be found in ref. 17 and 18.
Synthesis of hydroxyl-terminated P3HT
2,5-Dibromo-3-hexylthiophene (656 mg, 2 mmol) was added to a dry flask, with THF (2 mL) as the solvent, and an isopropylmagnesium chloride solution (1 mL, 2 mmol, 2 mol L−1 in THF)was added to the system under argon atmosphere stirring at 25 °C for 4 h. Then, a THF solution of the Ni complex was added, at this time, the solution turned deep red immediately. Then 0.2 mL 3-iodopropanol was added and stirred for 1 h, then 0.5 mL of methanol was added to terminate reaction. The product was quenched by a large amount of methanol, whereby a red precipitate appeared. The crude product was washed with methyl alcohol 3 times to obtain a deep red powder.
The hydrolysis process of hydroxyl-terminated P3HT: hydroxyl-terminated P3HT was dissolved in THF. The system was cooled down to 0 °C, and then CH3ONa (0.2 mL, 1 mmol, 30 wt% in methanol) was added to the system. After stirring at 0 °C for 4 h, 1 mL water was added to terminate the reaction. THF was removed under vacuum. The polymers were washed with methyl alcohol for 3 times. After drying, the hydroxyl-terminated P3HT was obtained.
Synthesis of hydroxyl-terminated PS
Styrene (520 mg, 5 mmol) was added to a dry flask with tetrahydrofuran (THF) as the solvent. A THF solution of the Ni complex was added to the system under argon atmosphere. The colorless system immediately turned deep red after reaction at 50 °C for 4 h. Then 0.2 mL 3-iodopropanol was added and stirred for 1 h. The solution was quenched with a large amount of methanol to obtain a white precipitate. The product was subjected to centrifugation at a rotation speed of 10
000 rpm. The yield is 93%. The hydrolysis process of hydroxyl-terminated PS is similar with hydroxyl-terminated P3HT.
Synthesis of hydroxyl-terminated PB
Experiments were followed the Schlenk operation, butadiene (540 mg, 10 mmol) was added in a Schlenk bottle. Ni complex (125 mg, 0.1 mmol) was added under argon atmosphere and stirred for 4 hours at room temperature, during this time the colorless solution becomes yellow. After 4 hours 0.2 mL 3-iodopropanol was added and stirred for 1 h, then 0.5 mL of methanol was added to terminate reaction. The solvent was concentrated via vacuum. The polymer was again dissolved in THF, and washed 3 times with methanol. The polymer product which showed with a colorless and transparent viscous liquid was obtained. The yield was 98%. The hydrolysis process of hydroxyl-terminated PB is similar with hydroxyl-terminated P3HT.
Synthesis of hydroxyl-terminated PB-b-P3HT
2,5-Dibromo-3-hexylthiophene (3260 mg, 10 mmol) was added to a dry flask, with THF (2 mL) as the solvent, and an isopropylmagnesium chloride solution (1 mL, 2 mmol, 2 mol L−1 in THF)was added to the system under argon atmosphere stirring at 25 °C for 4 h. Meanwhile, butadiene (2700 mg, 50 mmol) was added in a Schlenk bottle. The butadiene was dissolved in toluene, which was purchased from the TCI chemicals company, the butadiene solution was stored in a glove box with argon atmosphere. Ni complex (125 mg, 0.1 mmol) (dissolve in 2 mL THF) was added under argon atmosphere and stirred for 4 hours at room temperature, during this time the colorless solution becomes yellow. After stirring for 4 h at r. t., triphenylphosphine (26 mg, 0.1 mmol) was added and stirring for 1 h. Then, the solution of 2,5-dibromo-3-hexylthiophene abovementioned was added to the solution of polybutadiene, at this time, the mixed solution turned deep red immediately. Then stirring for 4 h at 50 °C. Finally, 0.2 mL 3-iodopropanol was added and stirred for 1 h, then 0.5 mL of methanol was added to terminate reaction. The product was quenched by a large amount of methanol, whereby a red precipitate appeared. The crude product was washed with methyl alcohol 3 times. The yield of PB-b-P3HT block copolymer is 87%.
3. Results and discussion
The synthesized route of hydroxyl-terminated PB, PS, P3HT was shown in Scheme 1.
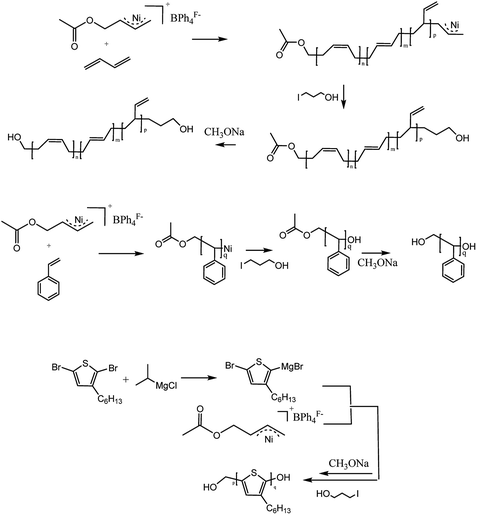 |
| Scheme 1 The synthesized routes of hydroxyl-terminated PB, PS, P3HT. | |
Synthesis of [Ni(η3-CH2CHCHCH2OOCH3)][BPh4F] initiator
Because 2-butene-1,4-dioldiacetate possesses two allyl acetate (CH3COOCH2CH
CHCH2OCOCH3) in which one could react with bis(1,5-cyclooctadiene)nickel [Ni(cod)2] to obtain a π-allyl Ni structure; the other could provide a protected hydroxyl group, we choose 2-butene-1,4-dioldiacetate as the allylation agent. A strong electronegativity structure, [BPh4F]−, was also selected as the counterion. Fig. 1 is the synthetic routes of [Ni(η3-CH2CHCHCH2OCOCH3)][BPh4F] including its 1H-NMR characterization data. Evidently, the chemical shift of C
C in cis-1,4-diacetoxy-2-butene was moved to low field (from 5.7 ppm to 3.6 ppm) due to the electronic shield effect of Ni. The results suggested that the allylation reaction was completed and the allyl nickel structure was obtained successfully. Furthermore, the chemical shifts at 7.2–7.5 ppm represent the characteristic peak of benzene ring with which 3, 5–2 (3 fluorinated methyl) phenyl borate was confirmed as a counterion [BPh4F]− to be located at a right place.
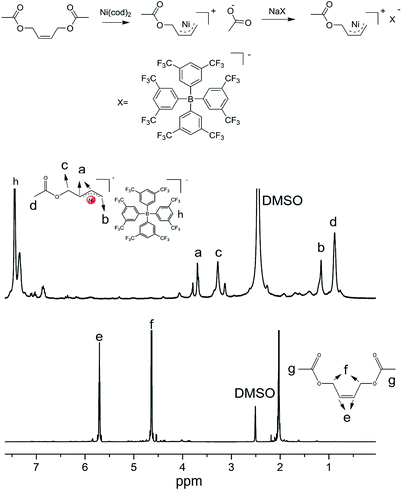 |
| Fig. 1 Synthesis route of [Ni(η3-CH2CHCHCH2OCOCH3)][BPh4F] initiator and its 1H-NMR characterization. | |
Application of [Ni(η3-CH2CHCHCH2OCOCH3)][BPh4F] in hydroxyl terminated poly(3-hexylthiophene)
Fig. 2 shows the 1H-NMR spectrum of hydroxy terminated poly(3-hexylthiophene) (HO-P3HT-OH) synthesized using [Ni(η3-CH2CHCHCH2OCOCH3)][BPh4F]. The chemical shifts at δ 2.83 (ppm) and 7.01 ppm are the characteristic peaks of P3HT, confirming the formation of hydroxyl-terminated P3HT (the GPC chromatogram of hydroxyl-terminated P3HT is shown in Fig. S1†).
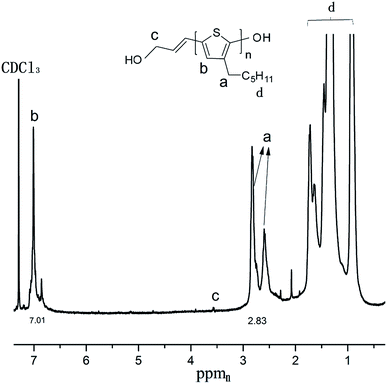 |
| Fig. 2 The 1H-NMR of hydroxyl-terminated P3HT synthesized by [Ni(η3-CH2CHCHCH2OCOCH3)][BPh4F]. | |
Application of hydroxyl-terminated π-allyl Ni complex to styrene and butadiene
In order to demonstrate the catalytic performance of [Ni(η3-CH2CHCHPhOCH3)(PPh3)][BPh4F] for the polymerization of different types of monomers, styrene and butadiene were selected.
Fig. 3 illustrates the 1H-NMR spectrum of hydroxyl-terminated PS. The chemical shift at 3.8 ppm was characteristic of –CH2– next to –OH, which suggested that hydroxyl-terminated PS was successfully synthesized (the GPC chromatogram of hydroxyl-terminated PS is shown in Fig. S2†).
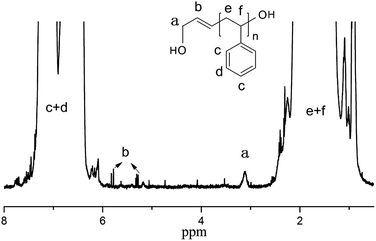 |
| Fig. 3 1H-NMR of hydroxyl-terminated PS synthesized by [Ni(η3-CH2CHCHCH2OCOCH3)][BPh4F]. | |
Fig. 4 illustrates the 1H-NMR spectrum of hydroxyl-terminated PB. 5.45 (ppm) and 5.01 ppm are the characteristic peaks of PB. The chemical shift at 3.6 ppm was characteristic of –CH2– next to –OH, which suggested that hydroxyl-terminated PB was successfully synthesized (the GPC chromatogram of hydroxyl-terminated PB is shown in Fig. S3†).
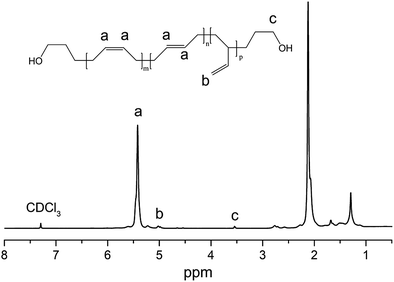 |
| Fig. 4 1H-NMR spectrum of hydroxyl-terminated PB synthesized by [Ni(η3-CH2CHCHCH2OCOCH3)][BPh4F]. | |
Table 1 is the molecular weight data of P3HT, PS, PB, which suggested that the Mn of three monomers is consistent with molar ratio of monomer/initiator, and there are average 2 hydroxys in pre macromolecule.
Table 1 GPC data of three kinds of polymers synthesized by [Ni(η3-CH2CHCHPhOCH3)(PPh3)][BPh4F]
Monomers |
Mn (g*mol−1) |
Mw/Mn |
hydroxyl value |
0.1 mmol initiator, HT amount: 1 mmol. 0.1 mmol initiator, St amount: 5 mmol. 0.1 mmol initiator, Bd amount: 10 mmol. 0.1 mmol initiator, HT amount: 10 mmol. 0.1 mmol initiator, St amount: 25 mmol. 0.1 mmol initiator, Bd amount: 25 mmol. |
P3HTa |
1673 |
1.04 |
1.98 |
PSb |
5314 |
1.08 |
2.03 |
PBc |
5520 |
1.03 |
1.99 |
P3HTd |
16 540 |
1.04 |
1.98 |
PSe |
26 718 |
1.08 |
1.96 |
PBf |
14 132 |
1.03 |
1.97 |
Application of hydroxyl-terminated π-allyl Ni complex to synthesized hydroxyl-terminated P3HT-b-PB block copolymer
To improve the workability and brittleness of poly(3-hexylthiophene) and to prove the advantage of [Ni(η3-CH2CHCHCH2OCOCH3)][BPh4F] in block copolymer containing P3HT. The hydroxyl-terminated P3HT-b-PB block copolymer was designed and synthesized. The 1H NMR of hydroxyl-terminated P3HT-b-PB block copolymer was shown in Fig. 5, 2.83 (ppm) and 7.01 ppm are the characteristic peaks of P3HT and 5.45 (ppm) and 5.01 ppm are the characteristic peaks of PB which confirm that the P3HT-PB copolymer was obtained. Moreover, 3.53 ppm is the characteristic proton peaks of –CH2OH, and there are no –OH in the structure of monomers, hence, it mean that there are terminal –OH group in the structure of copolymer.
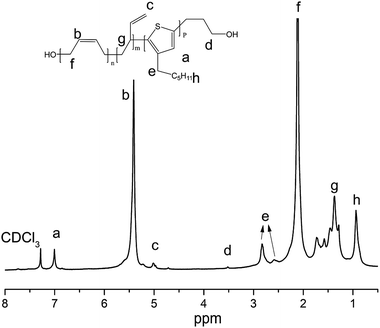 |
| Fig. 5 The 1H-NMR of hydroxyl-terminated PB-b-P3HT synthesized by [Ni(η3-CH2CHCHCH2OCOCH3)][BPh4F]. | |
To further prove the copolymer is an block copolymer, GPC and SEM was used to track the polymerization process, which were shown in Fig. 6–8. Fig. 6 is GPC result which shown unimodal after copolymerization process which suggested the block copolymer was obtained.
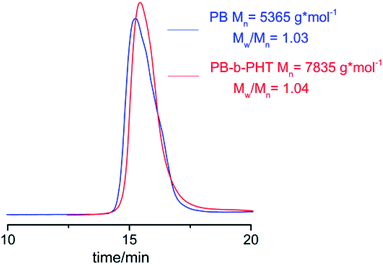 |
| Fig. 6 The GPC chromatogram of hydroxyl-terminated PB-b-P3HT block copolymer synthesized by [Ni(η3-CH2CHCHCH2OCOCH3)][BPh4F]. | |
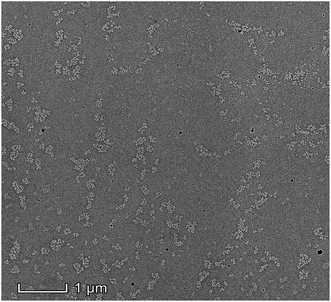 |
| Fig. 7 The SEM image of hydroxyl-terminated PB-b-P3HT block copolymer dissolved in THF. | |
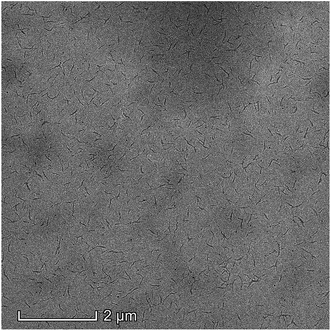 |
| Fig. 8 The SEM image of hydroxyl-terminated PB-b-P3HT block copolymer dissolved in n-hexane. | |
Fig. 7 is the SEM image in which the copolymer was dissolved in THF. Due to the block copolymer effect, it shown typically microphase separation phenomenon when the copolymer was dissolved in THF (good solvent for both block components).
To further explore the block copolymer effect of hydroxyl-terminated PB-b-P3HT, the copolymer was dissolved in n-hexane (good solvent of PB component, poor solvent of P3HT component) which was shown in Fig. 8. Due to the difference of the solubility between P3HT cmponent and PB component in hexane, the copolymer was assembled in hexane and exhibited a wormlike assembly in SEM image. Hence, according to the GPC and SEM results, the hydroxyl-terminated PB-b-P3HT block copolymer was successfully obtained.
“To further prove the PB-b-P3HT is block copolymer, the copolymer was test by differential thermal scanning (DSC) and thermogravimetric analysis (TGA), which shown in Fig. 9 and Fig. 10, The DSC and TGA curves of hydroxy-terminated PB homopolymer are shown in Fig. S4 and S6.† The DSC and TGA curves of hydroxy-terminated P3HT homopolymer are shown in Fig. S5 and S7.† DSC data suggested that there are two kinds of Tg in PB-b-P3HT block copolymer which represented PB block and P3HT block respectively. Moreover, there is a Tm peak at 24.8 °C. Similarly, there are two kinds of thermogravimetric temperature range in copolymer represented PB block(380 °C to 475 °C) and P3HT block (475 °C to 550 °C) respectively, which also mean copolymer is block copolymer.”
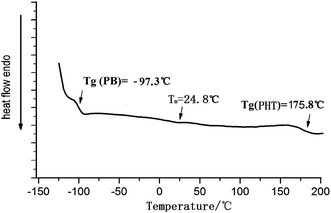 |
| Fig. 9 DSC data of hydroxy-terminated PB-b-P3HT. | |
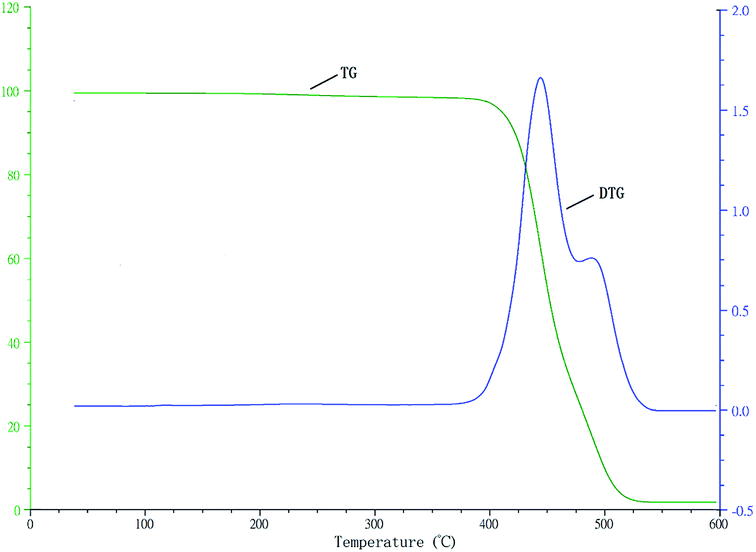 |
| Fig. 10 TGA curve of hydroxy-terminated PB-b-P3HT. | |
To study the relationship between copolymerization proportion and monomer proportion. A series of monomer proportion was designed and the results was shown in Table 2. The result of Table 2 shown that the copolymerization proportion is consistent with the monomer proportion, which suggested that the copolymerization process is a living (controlled) process.
Table 2 Relationship between copolymerization proportion and monomer proportion
Initiator : Bd : HT (molar ratio) |
Expected P3HT content |
Measured P3HT content |
Expected Mn/g mol−1 |
Measured Mn/g mol−1 |
Mw/Mn |
1 : 25 : 10 |
55.1% |
55.3% |
3010 |
3012 |
1.08 |
1 : 50 : 10 |
38.1% |
40.5% |
4360 |
4399 |
1.04 |
1 : 50 : 20 |
32.7% |
30.2% |
6020 |
5796 |
1.05 |
1 : 100 : 10 |
32.2% |
35.8% |
7960 |
7945 |
1.06 |
1 : 100 : 0 |
0 |
0 |
5400 |
5520 |
1.03 |
1 : 0 : 10 |
100 |
100 |
1660 |
1673 |
1.04 |
1 : 250 : 100 |
55.1% |
54.3% |
30 100 |
31 104 |
1.12 |
1 : 250 : 200 |
71.1% |
68.5% |
46 700 |
46 384 |
1.10 |
A possible mechanism of copolymerization was shown in Scheme 2. The Ni initiator initiated butadiene become a macroinitiator. Meanwhile, the 2,5-dibromo-3-hexylthiophene react with isopropylmagnesium chloride, and a intermediate product appeared,15 which can react with PB macroinitiator to obtain a block copolymer. Finally, oxidation addition occurred when 3-iodopropanol react with copolymer macroinitiator, –OH group was introduced in the end of copolymer. After hydrolysis, the other –OH appears in the end of copolymer. The detail copolymerization mechanism is still under study.
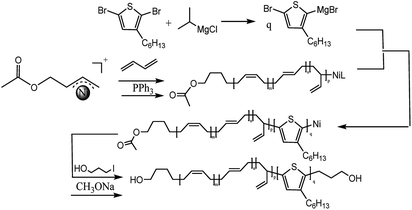 |
| Scheme 2 Possible mechanism of PB-b-P3HT copolymerization. | |
4. Conclusions
According to allylation reaction principle, a novel kind of hydroxyl-terminated Ni based initiator [Ni(η3-CH2CHCHCH2OCOCH3)][BPh4F] was designed and synthesized. The 1H-NMR was used to characterise this kind of Ni based initiator. This kind of initiator provide a new route for the polymerization of 2,5-dibromo-3-hexylthiophene. 1H-NMR of poly(3-hexylthiophene) suggested that the protected hydroxyl was successfully introduced in the structure of polymer. In addition, this kind of initiator expand the application range of monomer. It is not only can be used in polymerization of thiophene, but aslo can be used in other monomers such as styrene and butadiene. To improve the workability and brittleness of poly(3-hexylthiophene) and to prove the advantage of [Ni(η3-CH2CHCHCH2OCOCH3)][BPh4F] in block copolymer containing P3HT, the hydroxyl-terminated P3HT-b-PB block copolymer was designed and synthesized. The results of 1H-NMR and GPC and SEM suggested that the P3HT-b-PB block copolymer was successfully obtained, moreover, the study of the relationship between copolymerization proportion and monomer proportion shown that the copolymerization process is a living (controlled) process.
Author contributions
Conceptualization, X. M.; methodology, X. M.; software, C. C. Z. Y. P.; validation, Z. G. Y.; formal analysis, X. M.; investigation, X. M.; resources, C. C. Z.; data curation, X. M.; writing—original draft preparation, X. M.; writing—review and editing, C. C. Z.; visualization, C. C. Z.; supervision, C. C. Z.; project administration, Y. P.; funding acquisition, Y. P.
Funding
This work was supported by the National Natural Science Foundation of China (Grant No. 51562016), Jiangxi Province Natural Science Foundation of China (Grant No. 20171BAB206015) and Science and Technology Project of Jiujiang University (Grant No. 2016KJ005).
Conflicts of interest
The authors declare no conflict of interest.
Acknowledgements
This work was supported by Jiujiang Key Laboratory of Organosilicon Chemistry and Application, Jiujiang, 332005, P. R. China.
References
- B. C. Thompson and J. M. J. Fréchet, Polymer–Fullerene Composite Solar Cells, Angew. Chem., Int. Ed., 2008, 47, 58 CrossRef CAS
. - M. Frederic, et al., Synthesis of End-Group Functionalized P3HT: General Protocol for P3HT/Nanoparticle Hybrids, Macromolecules, 2013, 46(21), 8500–8508 CrossRef
. - A. Yokoyama and T. Yokozawa, Chain-Growth Polymerization for Poly(3-hexylthiophene) with a Defined Molecular Weight and a Low Polydispersity, Macromolecules, 2004, 37, 1169 CrossRef CAS
. - S. K. Patra, R. Ahmed, G. R. Whittell, D. J. Lunn, E. L. Dunphy, M. A. Winnik and I. Manners, Cylindrical Micelles of Controlled Length with a π-Conjugated Polythiophene Core via Crystallization-Driven Self-Assembly, J. Am. Chem. Soc., 2011, 133, 8842 CrossRef
. - J. H. Tsai, Y. C. Lai, T. Higashihara, C. J. Lin, M. Ueda and W. C. Chen, Enhancement of P3HT/PCBM Photovoltaic Efficiency Using the Surfactant of Triblock Copolymer Containing Poly(3-hexylthiophene) and Poly(4-vinyltriphenylamine) Segments, Macromolecules, 2010, 43, 6085 CrossRef
. - B. W. Boudouris, C. D. Frisbie and M. A. Hillmyer, Polylactide−Polythiophene−Polylactide Triblock Copolymers, Macromolecules, 2010, 43, 3566 CrossRef
. - Z. Li, R. J. Ono, Z. Q. Wu and C. W. Bielawski, Synthesis and self-assembly of poly(3-hexylthiophene)-block-poly(acrylic acid), Chem. Commun., 2011, 47, 197 RSC
. - T. Higashihara, K. Ohshimizu, A. Hirao and M. Ueda, Facile Synthesis of ABA Triblock Copolymer Containing Regioregular Poly(3-hexylthiophene) and Polystyrene Segments via Linking Reaction of Poly(styryl)lithium, Macromolecules, 2008, 41, 9505 CrossRef
. - A. Smeets, End Group-Functionalization and Synthesis of Block-CoPoly(3-hexylthiophene)s by Modified Nickel Initiators, Macromolecules, 2011, 44(15), 6017–6025 CrossRef
. - D. Meinhard, et al., New Nickel(II) Diimine Initiators and the Control of Polyethylene Microstructure by Catalyst Design, J. Am. Chem. Soc., 2007, 129(29), 9182–9191 CrossRef
. - J. Liu, et al., Polymerization of α-Olefins Using a Camphyl α-Diimine Nickel Catalyst at Elevated Temperature, Macromolecules, 2014, 47(10), 3325–3331 CrossRef
. - J. Cámpora., et al., h6-Arene initiators of Ni(II), efficient catalysts for 1,3-butadiene and styrene polymerization, Chem. Commun., 2003, 78–79 RSC
. - M. Jiménez-Tenorio, et al., Cationic Nickel Initiators Containing Bulky Phosphine Ligands: Catalyst Precursors for Styrene Polymerization, Organometallics, 2004, 23(13), 3139–3146 CrossRef
. - Z. Yu, et al., Polyallene-block-polythiophene-block-polyalleneCopolymers: One-Pot Synthesis, Helical Assembly, and Multiresponsiveness, Macromolecules, 2016, 49(4), 1180–1190 CrossRef
. - L. Gao, et al., Facile Preparation of Regioregular Poly(3-hexylthiophene) and Its Block Copolymers with π-Allylnickel Complex as External Initiator, Macromolecules, 2014, 47(15), 5010–5018 CrossRef
. - Z. Yu, et al., Polyallene-block-Poly(3-hexylthiophene)-block-polyalleneCopolymers: One-Pot Synthesis, Helical Assembly, and Multiresponsiveness, Macromolecules, 2016, 49(4), 1180–1190 CrossRef
. - M. Xin, et al., η3-π-Allyl Acetoxyl Ni Complex, an Efficient Initiator for Synthesis of Hydroxyl-Terminated Polybutadiene with High cis-1,4 Unit Content, Macromol. Chem. Phys., 2018, 219(24), 1800479 CrossRef
. - M. Xin, et al., A New Strategy for the Synthesis of Hydroxyl Terminated Polystyrene-b-Polybutadiene-b-Polystyrene Triblock Copolymer with High Cis-1, 4 Content, Polymers, 2019, 11, 598 CrossRef
.
Footnote |
† Electronic supplementary information (ESI) available. See DOI: 10.1039/d0ra07773a |
|
This journal is © The Royal Society of Chemistry 2020 |
Click here to see how this site uses Cookies. View our privacy policy here.