DOI:
10.1039/D0RA08070E
(Paper)
RSC Adv., 2020,
10, 39396-39405
Impact of heavy metals on Ciconia boyciana feathers and Larus saundersi egg shells in the Yellow River delta estuary
Received
21st September 2020
, Accepted 15th October 2020
First published on 27th October 2020
Abstract
In this study, the Ciconia boyciana and Larus saundersi, typical wetland birds in the Yellow River estuary, were selected as research objects. The feathers and egg shells of Ciconia boyciana and Larus saundersi were collected to determine the contents of heavy metals Cd, Cr, Cu, Zn, Mn, Ni and Pb in the samples. Correlation analysis (CA), principal component analysis (PCA), principal factor analysis (PFA) and potential ecological risk index (RI) were used to analyse and treat the measured heavy metal content data to determine the heavy metal pollution status, source and potential ecological risk in the Yellow River estuary. The results of CA, PCA and PFA showed that the content of Cd in the Yellow River estuary was much higher than the background value and its pollution was the most serious. Cr pollution was second and Mn pollution was the lowest. Heavy metal pollution mainly came from human activities such as oil exploitation, industrial production, use of pesticides and fertilizers, and the pollution caused by natural sources was relatively low. Based on the RI value of heavy metals in the study area, heavy metal Cd had an extremely high ecological risk status, and other heavy metals were all low. The results of this study can provide some reference and theoretical support for bird protection, heavy metal pollution control and ecological restoration in the Yellow River delta.
Introduction
Rapid industrialization and economic development have caused serious heavy metal pollution, especially in coastal areas.1–3 Crude oil pollution, seawater intrusion, overexploitation and other problems have aggravated heavy metal pollution in the Yellow River delta wetland,4 which leads to wetland and vegetation degradation, biodiversity decline and destruction of the original ecological structure.5–8 It is urgent to solve the threat to the ecosystem and biological population caused by the increase of the heavy metal concentration in the Yellow River delta wetland.9,10
The Yellow River delta is the largest and youngest wetland ecosystem in China's warm temperate zone, with a vegetation coverage of up to 55.1%, forming the largest beach vegetation in coastal China.11 Abundant wetland vegetation and aquatic biological resources provide numerous habitats for birds, making the region an important “transit station”, overwintering and breeding ground for bird migration in the inland and western Pacific Rim of Northeast Asia.12 The concentration of heavy metals in wetlands is high, and the main sources of pollution include crude oil pollution, pesticide and fertilizer, atmospheric deposition and so on.13,14 Low-grade organisms transport to higher trophic levels through food webs after ingestion of heavy metals and accumulate in vivo to influence animal growth, development, reproduction and behavior patterns.15–17 Ultimately, it has adverse effects on the human nervous system, endocrine and hematopoietic systems.18–20 Heavy metals have posed a serious threat to human health and ecological environment due to their toxicity, non-degradability and cumulative amplification.21,22 Over the past decades, a great deal of investigations and monitoring have been carried out to restore the ecological environment of the Yellow River delta wetland, mainly focused on sediment, hydrology and soil.23–25 However, the degree of heavy metal pollution in different regions was also different due to biological factors and abiotic factors,26 and many data obtained were often difficult to explain. Previous studies on heavy metal pollution in the Yellow River delta mainly focused on soil and other aspects, while the research on heavy metals and organisms were less, and studies on the effects of heavy metals on birds were rarely reported. Therefore, this study was based on the comprehensive understanding of the Yellow River delta, it was of great theoretical and practical significance to conduct more accurate source analysis and potential ecological risk assessment of heavy metal pollution in the Yellow River estuary. In this study, birds were associated with heavy metal pollution. Typical wetland birds in the Yellow River estuary-Ciconia boyciana and Larus saundersi-were taken as research objects to collect the feathers of Ciconia boyciana and the egg shells of Larus saundersi. Besides, birds were particularly sensitive to environmental changes and can better reflect the harm of heavy metal pollution to humans than most invertebrates and were often used as environmental monitoring organisms.27,28 Numerous studies have used feathers, eggs or other tissues to monitor heavy metal contamination,29 allowing samples to be obtained without harming birds, and making large-scale and repeated sampling possible.30
Through the determination of heavy metals in the feathers of Ciconia boyciana and the egg shells of Larus saundersi, the pollution status, sources and potential ecological risk of the Yellow River estuary were analyzed by means of CA, PCA, PFA and RI. The results provide a theoretical basis for ecological restoration and bird population protection in the Yellow River delta wetland.
Materials and methods
Study site
The Yellow River Delta Nature Reserve (37°35′–38°12′ N, 118°33′–119°20′ E) is located in Dongying City, Shandong Province, China, at the junction of Bohai Sea and Laizhou Bay. The study area is located in the estuary of the Yellow River (Fig. 1). It belongs to the typical warm temperate continental monsoon climate, cold in winter and hot in summer, with four distinct seasons, and an average annual precipitation of 530–630 mm. The salt content of soil is high, mainly coastal tidal soil and coastal saline soil. According to the salt content, there are various kinds of halophytic vegetation, such as reeds, ragwort and tamarix. The Yellow River Delta Nature Reserve is mainly established for the protection of wetland ecosystem and rare and endangered waterfowl.31 Because of its superior geographical location and unique ecological type, it has become a representative example of estuarine wetland ecosystem. There are 7 species of national first-class protected birds, 33 species of national second-class protected birds,32 and there are many rare and endangered birds such as Larus saundersi.
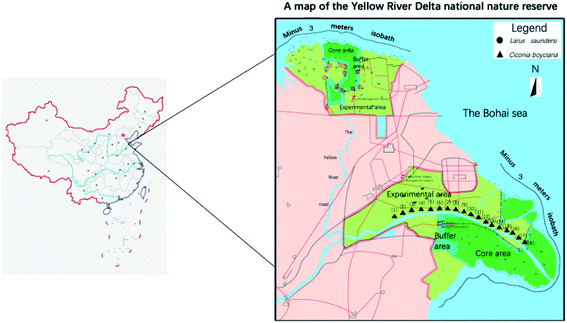 |
| Fig. 1 Location of study area and sampling point in the Yellow River estuary. | |
Sample collection and analysis
Sampling points were set up at the nests of Ciconia boyciana and Larus saundersi. There were 18 sampling points near the Ciconia boyciana nest, and each sampling point made 3 parallel samples, a total of 54 Ciconia boyciana feather samples. There were 12 sampling points for successful hatching egg shells (samples with only remaining egg shell after hatching) and failed hatching egg shells (samples from failed hatching, fetal dead eggs) in the breeding ground of Larus saundersi. In each sampling site, 3 parallel samples were made for successful hatching and failed hatching samples, and a total of 72 samples were obtained. The collected samples were labelled separately into the sealed bags and stored at 4 °C.
The samples were put into a clean glassware and removed the leaves, weeds, soil, etc. on its surface, and cleaned three times each with tap water, distilled water and acetone after removal. After washing, it was put into the oven and dried for 12 hours at a constant temperature of 80 °C. The dried feather samples were taken out and chopped with stainless steel scissors, weighing 0.5 g into PTFE crucible. Then add concentrated HNO3 (65%) and H2O2 (30%) at a ratio of 5
:
1, shake evenly after capping and place overnight. The temperature of the electric heating plate was controlled between 250 °C and 300 °C. In order to make the reaction shake out of time completely during the heating process, the PTFE crucible was removed after digestion to light yellow, and the volume was determined by 25 ml volumetric bottle after cooling. The egg shell samples were ground into powder by agate mortar after drying, and the 60 mesh nylon sieve was sifted and weighed 0.5 g into PTFE crucible. Add 16 ml concentrated nitric acid and 6 ml perchloric acid, shake evenly after capping and place overnight. Dissolve to colorless in the same way, and then cooled with a 25 ml volumetric bottle for measurement. Total analysis of seven heavy metals (Cd, Cr, Cu, Zn, Mn, Ni, Pb) in feather and egg shell samples using acid digestion, heavy metal content determination using flame atomic absorption spectrophotometry (Shimadzu AA-7000), heavy metals with low content were detected by graphite furnace. Heavy metals with high content were diluted and then detected. The measured data were analyzed by CA, PCA and PFA, and Pearson rank correlation was used to test the correlation between heavy metal concentration and pollution source. When the P value is less than 0.05, it is considered that there is a significant linear regression relationship between the heavy metal concentration and the pollution source, and there is an extremely significant linear regression relationship when it is less than 0.01.
Potential ecological risk assessment method
Based on the Hakanson sedimentary principles,33 and the existing potential risk assessment studies,34,35 the potential ecological risk index of heavy metals in the Yellow River estuary was evaluated. The risk assessment method used in this study is based on the following basic formulas:
where, Eri i reflects the pollution degree and potential ecological risk index of a single heavy metal, while RI reflects the sum of the potential ecological risks of all heavy metals. Eri i is the potential ecological risk index of heavy metal i. Tri i is the toxic reaction factor of specific heavy metal i (Cd = 30, Cr = 2, Cu = 5, Mn = 2, Ni = 5, Pb = 5, Zn = 1). Ci i is the actual measured concentration of heavy metal i in the sample (mg kg−1), and Cni i is the background values of heavy metal i in the study area. The soil background values of Shandong Province (Cd: 0.084; Cr: 66; Cu: 24; Mn: 644; Ni: 25.8; Pb: 25.8; Zn: 63.5) (mg kg−1) is used.
The pollution degree and potential ecological risk of a heavy metal (Eri) are divided into low degree (Eri ≤ 40), medium degree (40 < Eri ≤ 80), considerable degree (80 < Eri ≤ 160), high degree (160 < Eri ≤ 320) and extremely high degree (320 < Eri). The comprehensive potential RI of heavy metals is divided into low risk (RI < 50), medium risk (50 ≤ RI < 100), high risk (100 ≤ RI < 200) and very high risk (RI ≥ 220).
Statistical analysis
Data processing using Excel 2000 and data analysis using SPSS for Mac26.0.
Results and discussion
Analysis of heavy metals in Ciconia boyciana feathers
From the average value of heavy metal content in each sample, the average value of Cd, Cr, Zn, Cu and Ni in the sample was higher than the background values of heavy metal content in Shandong soil, which were 8.14, 3.58, 1.83, 1.80 and 1.14 times of the background values respectively, indicating that these heavy metal elements had local pollution phenomenon, among which Cd pollution was the most obvious. The average content of heavy metals Mn and Pb were lower than the background values of heavy metal content in Shandong soil, and the maximum value of Mn was also much lower than the background values, which indicating that the Ciconia boyciana is not polluted by Mn, and Pb pollution is also light. Table 1 showed the specific values of heavy metal content at each sampling point in Fig. 2. Fig. 2 combined with Table 1 showed that the overall content of heavy metal Cr was the highest, and Cr content of sampling points 14–18 were significantly reduced, mainly because the sampling points 14–18 were located in a protected lotus root pond, while the sampling points 1–13 were located in the beach near the oil well. The overall content of Zn was the second; the content of Cu was relatively stable; the content of Mn and Ni in sampling points 1–13 were relatively stable, and the content of sampling points 14–18 were lower than the average; the content of Pb and Cd had no obvious regularity. As a whole, the heavy metal content of Ciconia boyciana feather was Cr > Zn > Mn > Cu > Ni > Pb > Cd. The content of heavy metals in Ciconia boyciana feathers were moderate or significantly higher than the background value of soil heavy metal concentration in Shandong, indicating that feathers had a strong accumulation ability to heavy metals.36 The contents of the seven heavy metals in sampling points no. 0.3, no. 0.5 and no. 0.6 were higher than those in other sampling points, which may be due to their proximity to the protection areas, the large number of workers and tourists, the human activities such as vehicle exhaust, tourist garbage and atmospheric deposition had all aggravated the heavy metal pollution in the surrounding environment.37,38 The heavy metal content of sampling point no. 18 decreased obviously, mainly because it was located near the core area, which was less affected by human activities and less polluted.
Table 1 Heavy metal contents in Ciconia boyciana feathers
Sampling points |
Cd |
Cr |
Cu |
Mn |
Ni |
Pb |
Zn |
1 |
1.17 |
402.39 |
44.66 |
37.68 |
48.26 |
6.06 |
96.66 |
2 |
2.35 |
362.16 |
46.09 |
44.02 |
45.81 |
6.31 |
100.61 |
3 |
1.13 |
341.7 |
41.16 |
70.32 |
48.95 |
14.8 |
120.38 |
4 |
0.4 |
376.88 |
41.63 |
48.4 |
45.98 |
13.51 |
107.56 |
5 |
1.73 |
382.44 |
39.49 |
66.5 |
50.08 |
17.71 |
149.15 |
6 |
0.19 |
357.52 |
43.96 |
56.21 |
45 |
32.28 |
88.63 |
7 |
0.11 |
221.94 |
44.33 |
54.34 |
37.23 |
7.35 |
117.29 |
8 |
0.21 |
271.81 |
43.2 |
36.36 |
32.04 |
6.37 |
95.3 |
9 |
0.8 |
313.84 |
46.64 |
51.44 |
37.61 |
17.67 |
128.61 |
10 |
1.27 |
240.78 |
42.63 |
53.81 |
31.84 |
18.8 |
107.89 |
11 |
0.57 |
255.11 |
44.45 |
41.56 |
37.48 |
19.15 |
135.57 |
12 |
0.34 |
291.99 |
45.54 |
35.6 |
33.38 |
12.12 |
120.05 |
13 |
0.53 |
225.54 |
39.94 |
48.03 |
32.63 |
16.45 |
106.5 |
14 |
0.25 |
32.45 |
42.66 |
16.21 |
0.28 |
15.58 |
129.31 |
15 |
0.33 |
48.11 |
43.11 |
26.63 |
0.43 |
15.78 |
123.48 |
16 |
0.48 |
46.57 |
44.91 |
39.53 |
0.96 |
23.78 |
124.73 |
17 |
0.4 |
40.31 |
40.54 |
36.44 |
0.44 |
25.38 |
123.75 |
18 |
0.06 |
39 |
44.05 |
37.44 |
0.24 |
19.66 |
114.6 |
n |
18 |
18 |
18 |
18 |
18 |
18 |
18 |
SD |
0.60 |
131.60 |
2.02 |
12.93 |
18.81 |
6.81 |
15.03 |
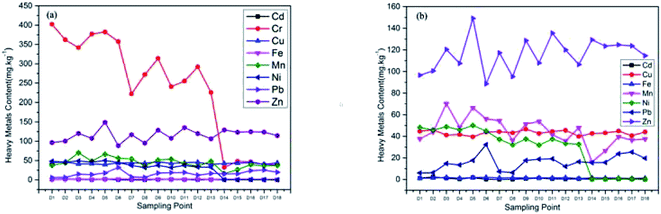 |
| Fig. 2 Ciconia boyciana feathers (a) and no Cr (b) heavy metal content. | |
Table 2 showed that Cd was positively correlated with Cr and Cd was positively correlated with Ni. There was a highly significant positive correlation between Cr and Mn, Cr and Ni, and Mn and Ni. There was no significant correlation between other heavy metals. It showed that there was a certain relationship between Cd and Cr, Ni pollution sources, and Cr, Mn and Ni were more likely to come from the same pollution sources. According to the results of the initial eigenvalues in Table 3, three principal components were considered, which account for about 74% of the total variance, and all elements were well represented by these three principal components. Among them, the heavy metals Ni, Cr, Mn and Cd had higher positive load values on the first principal component, and the load values were all greater than 0.7, which was consistent with the significant correlation between the four heavy metals. According to Table 3, the load values of Ni and Cr on the first principal component were the highest, which were 0.948 and 0.927, respectively. Ni was an important element of plant growth, but it could cause damage to human health and soil quality when its concentration exceeded the allowable limit.39 Ni mainly came from waste, waste water and dust in the smelting process. The natural sources of Cr were mainly rock weathering, and Cr exhaust gas, waste residue and waste water produced by metal smelting and fossil fuel combustion were also important sources of pollution. The load value of Cu in the second principal component was the highest, and the main pollution sources of Cu were mining, smelting, soot etc., and the co-existence with other heavy metals will lead to synergistic effect and aggravate the pollution. In Fig. 2, the average heavy metal contents of Cr, Ni and Cu were higher than the background value of soil in Shandong, so the first and second principal components mainly represent the influence of human factors such as industrial activities and wastewater discharge on Ciconia boyciana. The loading value of Pb in the third principal component was the highest, which was 0.726. Chemical fertilizers and pesticides applied in agricultural production and batteries used in industrial production can produce a large amount of Pb.40 In addition, because Pb was one of the crustal constituent elements, annual rock weathering will also lead to the increase of heavy metal Pb. In general, the level of heavy metal pollution caused by slow weathering and other natural activities is low. Combined with Fig. 2, we could see that the content of Pb was lower than the background value of soil in Shandong, so principal component 3 mainly represented the heavy metal pollution caused by natural sources. Therefore, heavy metal pollution in this area was closely related to industrial production, agricultural production, mining and other human activities.41 The comprehensive potential ecological risk values of heavy metals can reflect the pollution degree and potential ecological risk of toxic substances or pollutants to biological communities. As shown in Table 4, the average potential ecological RI of heavy metals in Ciconia boyciana feathers was 271.39, indicating that the pollution in this area was relatively serious. The Eri of heavy metal Cd was the highest, with an average value of 244.44, indicating that Cd in the study area had extremely high potential ecological risk. Cd was highly toxic and had obvious biomagnification effect during the transmission of food web, which was likely to have a negative impact on survival and reproduction.42,43 Cd pollution mainly came from fuel combustion, atmospheric deposition, industrial wastewater and so on. The Eri of the other six heavy metals were all less than 10, indicating that the pollution degree and ecological risk level of the remaining heavy metals were relatively low. Therefore, Cd may be the main potential ecological risk factor. Cd had the lowest heavy metal content, but the potential ecological risk value was the highest. There were two main reasons for this difference: on the one hand, the toxicity coefficient of each heavy metal was different, and Cd had the highest toxicity coefficient; on the other hand, although the content of heavy metals such as Cr was much higher than that of Cd, they could be mineralized and buried in soil to reduce their biological toxicity and ecological risk. According to the above results, the heavy metals Ni and Cr had the most significant effect on Ciconia boyciana. Cd and Cr pollution in this area were serious, and Cd had an extremely high potential ecological risk. Heavy metal pollution was mainly related to large-scale oil extraction, metal smelting, fertilizer application and other human activities.
Table 2 Correlation of heavy metal content in Ciconia boyciana feathers
|
Cd |
Cr |
Cu |
Mn |
Ni |
Pb |
Zn |
Significantly correlation at 0.05 level (bilateral). Significant correlation at 0.01 level (bilateral). |
Cd |
1 |
|
|
|
|
|
|
Cr |
0.523a |
1 |
|
|
|
|
|
Cu |
−0.001 |
0.066 |
1 |
|
|
|
|
Mn |
0.443 |
0.679b |
−0.272 |
1 |
|
|
|
Ni |
0.506a |
0.98b |
0.016 |
0.751b |
1 |
|
|
Pb |
−0.285 |
−0.327 |
−0.206 |
0.085 |
−0.332 |
1 |
|
Zn |
0.06 |
−0.294 |
−0.206 |
0.029 |
−0.249 |
0.176 |
1 |
Table 3 Principal component load values and analysis results of heavy metals in Ciconia boyciana feathers
Item |
Principal component 1 |
Principal component 2 |
Principal component 3 |
Eigenvalue |
Variance contribution rate/% |
Cumulative variance contribution rate/% |
Ni |
0.948 |
0.188 |
|
3.268 |
40.851 |
40.851 |
Cr |
0.927 |
0.248 |
|
1.557 |
19.466 |
60.317 |
Mn |
0.816 |
−0.255 |
0.371 |
1.172 |
14.644 |
74.961 |
Cd |
0.709 |
−0.133 |
−0.265 |
0.908 |
11.356 |
86.317 |
Cu |
−0.11 |
0.802 |
|
0.525 |
6.557 |
92.874 |
Zn |
−0.13 |
−0.687 |
|
0.383 |
4.793 |
97.667 |
Pb |
−0.344 |
−0.353 |
0.726 |
0.011 |
0.136 |
100 |
Table 4 Ecological risk assessment results of heavy metals in Ciconia boyciana feathers
|
Eri |
RI |
Cd |
Cr |
Cu |
Mn |
Ni |
Pb |
Zn |
Max |
839.29 |
12.19 |
9.72 |
0.12 |
9.71 |
6.26 |
2.35 |
879.64 |
Min |
21.43 |
0.98 |
8.23 |
0.05 |
0.05 |
1.17 |
1.40 |
33.31 |
Average |
244.44 |
7.16 |
9.02 |
0.14 |
5.69 |
3.11 |
1.83 |
271.39 |
Analysis of heavy metals in egg shells of Larus saundersi
Analysis of heavy metals in hatching success egg shells of Larus saundersi. Heavy metal Cd was not detected in hatching success egg shell samples of Larus saundersi, indicating that the content of Cd in samples was lower than the minimum measured value. From the average value of heavy metal content in each sample, only the average value of heavy metal Cr in the samples was higher than the background value of heavy metal content in Shandong soil, which was 1.35 times of the background value. The maximum value of the remaining five heavy metals was also much lower than the background value, indicating that there was local Cr pollution in this region. Table 5 showed the specific values of heavy metal content at each sampling point in Fig. 3. As can be seen from Fig. 3 and Table 5, the Cr content was the highest, and the Cr content of H5, H6, H7 three sampling points was significantly lower than that of other sampling points. It may be due to the high terrain of these three sampling sites, the heavy metal content in this area was low after the long-term scouring by high tide and rain, and the heavy metal content of fish and worms in the tidal flat was lower, which was consistent with the research results of low heavy metal concentration in the strong hydrodynamic zone.44 Besides, the content of Mn was irregular, and the content of the other four heavy metals was similar and stable. Heavy metal contents in the hatching success egg shells of Larus saundersi were Cr > Mn > Zn > Ni > Cu > Pb > Cd, which was quite different from that of Ciconia boyciana feathers. The contents of heavy metals in the successful hatching egg shells of Larus saundersi were lower than that of Ciconia boyciana, indicating that the accumulation capacity of heavy metals in different individuals and parts was different.45
Table 5 Heavy metal contents in hatching success egg shells of Larus saundersi
Sampling points |
Cd |
Cr |
Cu |
Mn |
Ni |
Pb |
Zn |
1 |
0 |
119.41 |
11.73 |
19.86 |
17.53 |
13.63 |
23.85 |
2 |
0 |
104.2 |
18.66 |
18.77 |
15.4 |
10.61 |
21.38 |
3 |
0 |
104.52 |
21.07 |
11.74 |
15.06 |
11.48 |
16.96 |
4 |
0 |
105.1 |
11.93 |
31.84 |
16.84 |
11.72 |
20.37 |
5 |
0 |
11.88 |
18.71 |
21.59 |
16.34 |
12.21 |
18.47 |
6 |
0 |
12.15 |
18.59 |
9.08 |
14.92 |
12.83 |
23.06 |
7 |
0 |
11.99 |
11.59 |
4.33 |
15.65 |
11.85 |
17.51 |
8 |
0 |
134.83 |
16.09 |
9.31 |
18.48 |
13.63 |
21.87 |
9 |
0 |
117.95 |
14.46 |
13.64 |
16.85 |
12.4 |
15.44 |
10 |
0 |
112.21 |
17.06 |
32.9 |
18.24 |
15.23 |
18.7 |
11 |
0 |
116.64 |
16.41 |
37.79 |
15.65 |
11.72 |
12.74 |
12 |
0 |
121.43 |
16.59 |
31.11 |
16.51 |
13.01 |
10.81 |
n |
12 |
12 |
12 |
12 |
12 |
12 |
12 |
SD |
0 |
45.40 |
2.96 |
10.56 |
1.14 |
1.18 |
3.85 |
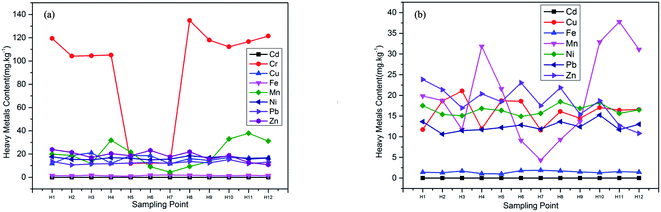 |
| Fig. 3 Hatching success egg shells (a) and no Cr (b) heavy metal content of Larus saundersi. | |
Table 6 showed that there was a significant positive correlation between Ni and Pb, and there was no significant correlation between other heavy metals. It indicated that the heavy metals Ni and Pb were more likely to come from the same pollution source. According to Table 7, the load values of heavy metals Ni and Pb on the first principal component were the highest, which were 0.941 and 0.833, respectively, which were consistent with the correlation analysis results. The natural sources of Ni and Pb were mainly the weathering of olivine and shale, and the man-made sources include “three wastes” in industrial production. Fig. 3 showed that the maximum values of Ni and Pb were also much lower than the soil background value in Shandong, so the first principal component represented the effect of natural sources on the successful hatching egg shells of Larus saundersi. The load values of the second principal component were all negative, and Cr load values of the third principal component are the highest. Cr pollution mainly came from the waste gas, waste residue, waste water and rock weathering produced by industrial activities. Because the content of Cr was higher than the background value, the third principal component represented heavy metal pollution caused by human activities. Table 8 showed the assessment results of potential ecological risks of heavy metals in hatching success egg shells, RI was less than 50, indicating that the heavy metal pollution degree and potential ecological risks in the habitat of Larus saundersi were relatively low. Cu had the highest potential ecological risk value, followed by Cr, Ni and Pb. The content of Cr was the highest, but the potential ecological risk value was lower than that of Cu, mainly because of the small toxicity coefficient of Cu. From this we can see that Ni and Pb had the greatest influence on successful hatching egg shells of Larus saundersi, and had little relation with heavy metal pollution caused by human activities. The pollution degree of the region was relatively light but there was some Cr pollution, and Cu was the main potential ecological risk factor.
Table 6 Correlation of heavy metal content in hatching success egg shells of Larus saundersi
|
Cr |
Cu |
Mn |
Ni |
Pb |
Zn |
Significant correlation at 0.01 level (bilateral). |
|
|
Cr |
1 |
|
|
|
|
|
Cu |
−0.83 |
1 |
|
|
|
|
Mn |
0.425 |
−0.03 |
1 |
|
|
|
Ni |
0.51 |
−0.387 |
0.218 |
1 |
|
|
Pb |
0.203 |
−0.138 |
0.154 |
0.756a |
1 |
|
Zn |
−0.188 |
−0.118 |
−0.423 |
0.182 |
0.149 |
1 |
Table 7 Principal component load values and analysis results of heavy metals in hatching success egg shells of Larus saundersi
Item |
Principal component 1 |
Principal component 2 |
Principal component 3 |
Eigenvalue |
Variance contribution rate/% |
Cumulative variance contribution rate/% |
Ni |
0.941 |
−0.195 |
|
2.432 |
34.744 |
34.744 |
Pb |
0.833 |
|
|
1.699 |
24.266 |
59.01 |
Cu |
−0.486 |
|
0.209 |
1.033 |
14.762 |
73.772 |
Mn |
0.114 |
−0.742 |
0.55 |
0.647 |
9.239 |
95.613 |
Zn |
0.282 |
|
−0.848 |
0.261 |
3.73 |
99.343 |
Cr |
0.487 |
−0.161 |
0.613 |
0.046 |
0.657 |
100 |
Table 8 Ecological risk assessment results of heavy metals in hatching success egg shells of Larus saundersi
|
Eri |
RI |
Cd |
Cr |
Cu |
Mn |
Ni |
Pb |
Zn |
Max |
0 |
4.09 |
4.39 |
0.12 |
3.58 |
2.95 |
0.38 |
15.51 |
Min |
0 |
0.36 |
2.41 |
0.01 |
2.89 |
2.06 |
0.17 |
7.9 |
Average |
0 |
2.71 |
3.35 |
0.06 |
3.19 |
2.43 |
0.29 |
12.03 |
Analysis of heavy metals in hatching failure egg shells of Larus saundersi. Fig. 4 showed the values of heavy metal content at each sampling point. The comparison between Fig. 4 and 3 showed that there were many similarities between heavy metal content of the failed hatching egg shells and the successful hatching egg shell: Cd was not detected, Cr content was the highest, Mn content changed greatly, and the other four heavy metal contents were relatively stable. Only the average value of heavy metal Cr in the sample was higher than the background value of heavy metal content in Shandong soil, which was 1.82 times. The maximum value of the remaining five heavy metals was also far lower than the background value, which indicated that there was local Cr pollution in this area. The overall trend of heavy metal content in hatching failure egg shells of Larus saundersi was Cr > Mn > Zn > Cu > Ni > Pb > Cd, and the content of five heavy metals except Ni was higher than that of hatching success egg shells. Theoretically, all the heavy metal contents of the failed hatching egg shells of Larus saundersi should be higher than that of successful hatching egg shells. However, in this study, we detected that the Ni contents of six sampling points were lower than that of hatching success egg shell, and the Ni contents difference of the other six sampling points were not significant. Probably because different organisms had different tolerance values to various heavy metals, and the Ni content in egg shells didn't reach the value that affected the hatching success. In addition, the contents of heavy metals in failed hatching egg shells of Larus saundersi were also lower than that of Ciconia boyciana. The results showed that different individuals had different ability to absorb and enrich heavy metals, and the tolerance value of heavy metals was also different.46
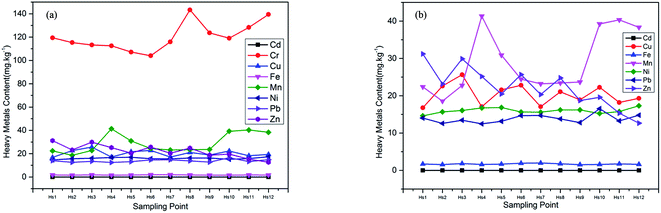 |
| Fig. 4 Hatching failure egg shells (a) and no Cr (b) heavy metal content of Larus saundersi. | |
As shown in Table 9, there was no significant correlation between heavy metals in hatching failure egg shells of Larus saundersi, indicating that the heavy metals that pollute the hatching failure egg shells were not come from the same pollution source. Table 10 showed that the load values of heavy metals Mn and Ni on the first principal component were higher than that of other heavy metals, and olivine weathering were also bring a lot of Mn and Ni. Mn was one of the essential trace elements for human body, and the main source of Mn pollution was the waste produced by alloys, catalysts and batteries in industrial production. The second principal component and the third principal component were closely related to Pb and Cu, respectively. Because the maximum values of the Mn, Ni, Pb and Cu were far lower than the background values, the three principal components all represented the influence of natural sources on hatching failure of Larus saundersi. Table 11 showed the potential ecological risk index results of heavy metals in failed hatching egg shells, RI were all less than 50. Cu had the highest potential ecological risk value, followed by Cr, Ni and Pb. Synthesizing the above results, we can see that the pollution degree of heavy metal in the study area was low, and there was partial Cr pollution, and Cu was the main potential ecological risk factor. Larus saundersi hatching failure egg shells was mainly contaminated by Mn and Ni.
Table 9 Correlation of heavy metal content in hatching failure egg shells of Larus saundersi
|
Cr |
Cu |
Mn |
Ni |
Pb |
Zn |
Cr |
1 |
|
|
|
|
|
Cu |
−0.299 |
1 |
|
|
|
|
Mn |
0.135 |
−0.283 |
1 |
|
|
|
Ni |
0.146 |
0.045 |
0.38 |
1 |
|
|
Pb |
0.114 |
0.115 |
0.211 |
−0.319 |
1 |
|
Zn |
−0.34 |
0.234 |
−0.546 |
−0.476 |
−0.18 |
1 |
Table 10 Ecological risk assessment results of heavy metals in Ciconia boyciana feathers
Item |
Principal component 1 |
Principal component 2 |
Principal component 3 |
Eigenvalue |
Variance contribution rate/% |
Cumulative variance contribution rate/% |
Zn |
−0.829 |
|
0.247 |
2.389 |
34.133 |
34.133 |
Mn |
0.798 |
|
−0.193 |
1.38 |
19.71 |
53.843 |
Ni |
0.69 |
−0.492 |
0.182 |
1.099 |
15.703 |
69.546 |
Pb |
0.213 |
0.935 |
|
0.807 |
11.531 |
81.077 |
Cu |
|
0.101 |
0.863 |
0.377 |
5.385 |
97.44 |
Cr |
0.252 |
|
−0.681 |
0.179 |
2.56 |
100 |
Table 11 Ecological risk assessment results of heavy metals in Ciconia boyciana feathers
|
Eri |
RI |
Cd |
Cr |
Cu |
Mn |
Ni |
Pb |
Zn |
Max |
0 |
4.34 |
5.34 |
0.13 |
3.36 |
3.21 |
0.49 |
16.87 |
Min |
0 |
3.15 |
3.50 |
0.06 |
2.83 |
2.41 |
0.20 |
12.15 |
Average |
0 |
3.64 |
4.23 |
0.09 |
3.10 |
2.68 |
0.35 |
14.09 |
Comparative analysis of heavy metals in egg shells of Larus saundersi hatching success and hatching failure. The results of independence test between the hatching success and hatching failure of Larus saundersi showed that there was significant difference in the contents of Cr, Mn and Pb, and extremely significant differences in the contents of Cu, which indicated that the contents of these heavy metals may affect the hatching success rate of Larus saundersi eggs.Previous studies had shown that heavy metals were easy to accumulate during the growth and development of birds, making the egg shell thinner, causing egg or excessive evaporation of water in the egg contents, and finally reducing the hatchability of eggs.16 Therefore, heavy metals Cr, Mn, Pb and Cu were one of the factors affecting the hatching of Larus saundersi, while the contents of Zn and Ni may not reach the value of toxic effect on embryo development and egg hatching success. In addition, it was not only heavy metal pollution, but also rainfall, plant invasion, natural enemies and other factors that lead to the decrease of the success rate and quantity of bird reproduction.47,48
Comparative analysis of heavy metals between Ciconia boyciana and Larus saundersi. The heavy metal pollution of Ciconia boyciana was more serious than that of Larus saundersi sampling points, probably because the Larus saundersi sampling points were close to the core area and less disturbed by human factors, which was consistent with the PCA results. The heavy metals Ni and Cr had the most significant effect on Ciconia boyciana feathers; Ni and Pb had the most significant effect on hatching successful Larus saundersi egg shells; while the failed hatching Larus saundersi egg shells were mainly affected by heavy metals Mn and Ni. This was not consistent with the overall trend of heavy metal content measured, which may be due to the different tolerance and enrichment degree of heavy metals in different organisms and tissues,27,29 and there was no unified standard value of heavy metal content in birds at present. The hatching success and hatching failure egg shells of Larus saundersi were not detected Cd, while the heavy metal Cd was detected in the feathers of Ciconia boyciana. This was because feather tissue was the place for heavy metals accumulation and excretion. Birds discharged heavy metals and other substances into growing feathers during their activities. The accumulation of heavy metals in feathers reflected the presence of pollutants in the environment.27,49 The two kinds of birds were mainly affected by heavy metals Cr, Ni and Pb, Cr, Ni and Pb were also the key factor for soil adsorption of metal elements, which was consistent with the previous research results of coastal wetlands in the Yellow River delta.38 Due to the enrichment of heavy metals, the heavy metals in wetlands will accumulate in Ciconia boyciana and Larus saundersi along with the food chain, and remain on them during the formation of feathers and eggshells. Therefore, the heavy metal content in the Ciconia boyciana feathers and Larus saundersi egg shells reflected the heavy metal pollution in the Yellow River estuary to some extent. Heavy metal Cd in the Yellow River estuary had great potential ecological risk, and heavy metal pollution included natural and human factors. Natural factors mainly referred to natural activities, such as rock weathering and volcanic eruptions. There were many oil fields in the Yellow River estuary, such as Shengli Oilfield. The large-scale oil exploitation and oil leakage made the heavy metal content in atmosphere, water and soil exceed the safety limit,10,50,51 which may be the main reason for heavy metal pollution detected in this study. In addition, sewage irrigation and the use of pesticides, herbicides and the development of mining industry had also caused serious heavy metal pollution to water quality and soil (Table 12).
Table 12 Independence test results of egg shell samples for hatching success and hatching failure of Larus saundersi
|
F |
T |
df |
P |
Significantly correlation at 0.05 level (bilateral). Significant correlation at 0.01 level (bilateral). |
Cr |
15.461 |
−2.208 |
22 |
0.038a |
Cu |
0.006 |
−3.492 |
22 |
0.002b |
Mn |
0.734 |
−2.21 |
22 |
0.038a |
Ni |
2.822 |
1.138 |
22 |
0.267 |
Pb |
0.016 |
−2.684 |
22 |
0.014a |
Zn |
1.343 |
−1.941 |
22 |
0.065 |
Conclusion
The contents of seven kinds of heavy metals in Ciconia boyciana feathers and Larus saundersi egg shells, the source, potential ecological risk and pollution status of heavy metal pollution in the Yellow River estuary were analyzed. The statistical analysis draws the following conclusions: (1) CA, PCA and PFA results show that the heavy metal pollution in the study area of Ciconia boyciana is more serious than that of Larus saundersi, and the influence of Ni on the two kinds of birds is more significant. (2) Heavy metal pollution is mainly related to man-made activities such as oil exploitation, industrial production and application of chemical fertilizer, and the pollution caused by natural factors is relatively light. (3) Potential ecological risk assessment results show that heavy metal Cd has an extremely high potential ecological risk, and other heavy metals are all low. (4) The degree of heavy metal pollution in the Yellow River estuary varies greatly, Cd pollution is the most serious, Mn pollution is the lightest, and the maximum value of Mn content is far lower than the background value of Shandong soil. The results of this paper not only have guiding significance for the protection of endangered species, but also provide a solid theoretical basis for the future habitat protection and wetland restoration, which is in line with the sustainable development strategy.
Conflicts of interest
There are no conflicts of interest to declare.
Acknowledgements
Thanks to Mr Junfeng Chen for his guidance and help with this research paper. This research was supported by the National Natural Science Foundation of China (no. 31672314 and no. 31700433).
References
- S.-L. Wang, X.-R. Xu, Y.-X. Sun, J.-L. Liu and H.-B. Li, Heavy metal pollution in coastal areas of South China: a review, Mar. Pollut. Bull., 2013, 76(1–2), 7–15 CrossRef CAS.
- B. Hu, G. Li, J. Li, J. Bi, J. Zhao and R. Bu, Spatial distribution and ecotoxicological risk assessment of heavy metals in surface sediments of the southern Bohai Bay, China, Environ. Sci. Pollut. Res., 2013, 20(6), 4099–4110 CrossRef CAS.
- L. Zhang, X. Ye, H. Feng, Y. Jing, T. Ouyang and X. Yu, et al., Heavy metal contamination in western Xiamen Bay sediments and its vicinity, China, Mar. Pollut. Bull., 2007, 54(7), 974–982 CrossRef CAS.
- R. Xiao, M. Zhang, X. Yao, Z. Ma, F. Yu and J. Bai, Heavy metal distribution in different soil aggregate size classes from restored brackish marsh, oil exploitation zone, and tidal mud flat of the Yellow River Delta, J. Soils Sediments, 2016, 16(3), 821–830 CrossRef CAS.
- H. Wang, R. Wang, Y. Yu, M. J. Mitchell and L. Zhang, Soil organic carbon of degraded wetlands treated with freshwater in the Yellow River Delta, China, J. Environ. Manage., 2011, 92(10), 2628–2633 CrossRef CAS.
- X. Yao, R. Xiao, Z. Ma, Y. Xie, M. Zhang and F. Yu, Distribution and contamination assessment of heavy metals in soils from tidal flat, oil exploitation zone and restored wetland in the Yellow River Estuary, Wetlands, 2016, 36(1), 153–165 CrossRef.
- X. Bi, B. Wang and Q. Lu, Fragmentation effects of oil wells and roads on the Yellow River Delta, North China, Ocean Coast. Manag., 2011, 54(3), 256–264 CrossRef.
- Y.-c. Gao, J.-n. Wang, S.-h. Guo, Y.-L. Hu, T.-t. Li and R. Mao, et al., Effects of salinization and crude oil contamination on soil bacterial community structure in the Yellow River Delta region, China, Appl. Soil Ecol., 2015, 86, 165–173 CrossRef.
- J. Bai, L. Huang, D. Yan, Q. Wang, H. Gao and R. Xiao, et al., Contamination characteristics of heavy metals in wetland soils along a tidal ditch of the Yellow River Estuary, China, Stoch. Environ. Res. Risk Assess., 2011, 25(5), 671–676 CrossRef.
- X. Miao, D. Miao, Y. Hao, Z. Xie and S. Zou, Potential health risks associated to heavy metal contamination of soils in the Yellow River Delta, China, J. Coast Conserv., 2019, 23(3), 643–655 CrossRef.
- B. Cui, Q. Yang, Z. Yang and K. Zhang, Evaluating the ecological performance of wetland restoration in the Yellow River Delta, China, Ecol. Eng., 2009, 35(7), 1090–1103 CrossRef.
- Y. Junbao, D. Hongfang, L. Yunzhao, W. Huifeng, G. Bo and G. Yongjun, et al., Spatiotemporal Distribution Characteristics of Soil Organic Carbon in Newborn Coastal Wetlands of the Yellow River Delta Estuary, Clean, 2013, 1–8 Search PubMed.
- Z. Xie, Z. Sun, H. Zhang and J. Zhai, Contamination assessment of arsenic and heavy metals in a typical abandoned estuary wetland—a case study of the Yellow River Delta Natural Reserve, Environ. Monit. Assess., 2014, 186(11), 7211–7232 CrossRef CAS.
- X. Miao, Y. Hao, F. Zhang, S. Zou, S. Ye and Z. Xie, Spatial distribution of heavy metals and their potential sources in the soil of Yellow River Delta: a traditional oil field in China, Environ. Geochem. Health, 2019, 1–20 Search PubMed.
- P. Frederick and N. Jayasena, Altered pairing behaviour and reproductive success in white ibises exposed to environmentally relevant concentrations of methylmercury, Proc. R. Soc. London, Ser. B, 2011, 278(1713), 1851–1857 CrossRef.
- S. Espín, E. Martínez-López, P. Jiménez, P. María-Mojica and A. J. García-Fernández, Effects of heavy metals on biomarkers for oxidative stress in Griffon vulture (Gyps fulvus), Environ. Res., 2014, 129, 59–68 CrossRef.
- M. Javed, I. Ahmad, N. Usmani and M. Ahmad, Studies on biomarkers of oxidative stress and associated genotoxicity and histopathology in Channa punctatus from heavy metal polluted canal, Chemosphere, 2016, 151, 210–219 CrossRef CAS.
- S. Cao, X. Duan, X. Zhao, Y. Chen, B. Wang and C. Sun, et al., Health risks of children's cumulative and aggregative exposure to metals and metalloids in a typical urban environment in China, Chemosphere, 2016, 147, 404–411 CrossRef CAS.
- Y. Wang, R. Wang, L. Fan, T. Chen, Y. Bai and Q. Yu, et al., Assessment of multiple exposure to chemical elements and health risks among residents near Huodehong lead-zinc mining area in Yunnan, Southwest China, Chemosphere, 2017, 174, 613–627 CrossRef CAS.
- K. Khan, Y. Lu, H. Khan, S. Zakir, S. Khan and A. A. Khan, et al., Health risks associated with heavy metals in the drinking water of Swat, northern Pakistan, J. Environ. Sci., 2013, 25(10), 2003–2013 CrossRef CAS.
- H. Qiu, Studies on the potential ecological risk and homology correlation of heavy metal in the surface soil, J. Agric. Sci., 2010, 2(2), 194–201 Search PubMed.
- X. Yang, J. Duan, L. Wang, W. Li, J. Guan and S. Beecham, et al., Heavy metal pollution and health risk assessment in the Wei River in China, Environ. Monit. Assess., 2015, 187(3), 111 CrossRef.
- Q. Rao, Z. Sun, L. Tian, J. Li, W. Sun and W. Sun, Assessment of arsenic and heavy metal pollution and ecological risk in inshore sediments of the Yellow River estuary, China, Stoch. Environ. Res. Risk Assess., 2018, 32(10), 2889–2902 CrossRef.
- B. Cui, N. Tang, X. Zhao and J. Bai, A management-oriented valuation method to determine ecological water requirement for wetlands in the Yellow River Delta of China, J. Nat. Conserv., 2009, 17(3), 129–141 CrossRef.
- J. Bai, R. Xiao, K. Zhang and H. Gao, Arsenic and heavy metal pollution in wetland soils from tidal freshwater and salt marshes before and after the flow-sediment regulation regime in the Yellow River Delta, China, J. Hydrol., 2012, 450, 244–253 CrossRef.
- Z. Sun, X. Mou, C. Tong, C. Wang, Z. Xie and H. Song, et al., Spatial variations and bioaccumulation of heavy metals in intertidal zone of the Yellow River estuary, China, Catena, 2015, 126, 43–52 CrossRef CAS.
- M. Janaydeh, A. Ismail, S. Z. Zulkifli, M. H. Bejo, N. A. A. Aziz and A. Taneenah, The use of feather as an indicator for heavy metal contamination in house crow (Corvus splendens) in the Klang area, Selangor, Malaysia, Environ. Sci. Pollut. Res., 2016, 23(21), 22059–22071 CrossRef CAS.
- Y. Hua, B. Cui and W. He, Changes in water birds habitat suitability following wetland restoration in the Yellow River Delta, China, Clean, 2012, 40(10), 1076–1084 CAS.
- C. Naccari, M. Cristani, F. Cimino, T. Arcoraci and D. Trombetta, Common buzzards (Buteo buteo) bio-indicators of heavy metals pollution in Sicily (Italy), Environ. Int., 2009, 35(3), 594–598 CrossRef CAS.
- R. Moreno, L. Jover, C. Diez and C. Sanpera, Seabird feathers as monitors of the levels and persistence of heavy metal pollution after the Prestige oil spill, Environ. Pollut., 2011, 159(10), 2454–2460 CrossRef CAS.
- Q. Liu, G. Liu, C. Huang and C. Xie. Vegetation patch structure and dynamics at gudong oil field of the yellow river delta, China, Geo-Informatics in Resource Management and Sustainable Ecosystem, Springer, 2013, pp. 177–187 Search PubMed.
- B. Cui, Q. Zhang, K. Zhang, X. Liu and H. Zhang, Analyzing trophic transfer of heavy metals for food webs in the newly-formed wetlands of the Yellow River Delta,
China, Environ. Pollut., 2011, 159(5), 1297–1306 CrossRef CAS.
- L. Hakanson, An ecological risk index for aquatic pollution control. A sedimentological approach, Water Res., 1980, 14(8), 975–1001 CrossRef.
- Y. Liu, Q. Wang, W. Zhuang, Y. Yuan, Y. Yuan and K. Jiao, et al., Calculation of Thallium's toxicity coefficient in the evaluation of potential ecological risk index: a case study, Chemosphere, 2018, 194, 562–569 CrossRef CAS.
- A. Pejman, G. N. Bidhendi, M. Ardestani, M. Saeedi and A. Baghvand, A new index for assessing heavy metals contamination in sediments: a case study, Ecol. Indicat., 2015, 58, 365–373 CrossRef CAS.
- M. Lucia, J.-M. André, K. Gontier, N. Diot, J. Veiga and S. Davail, Trace element concentrations (mercury, cadmium, copper, zinc, lead, aluminium, nickel, arsenic, and selenium) in some aquatic birds of the Southwest Atlantic Coast of France, Arch. Environ. Contam. Toxicol., 2010, 58(3), 844–853 CrossRef CAS.
- Y. Li, H. Zhang, X. Chen, C. Tu, Y. Luo and P. Christie, Distribution of heavy metals in soils of the Yellow River Delta: concentrations in different soil horizons and source identification, J. Soils Sediments, 2014, 14(6), 1158–1168 CrossRef.
- G. Zhang, J. Bai, Q. Zhao, Q. Lu, J. Jia and X. Wen, Heavy metals in wetland soils along a wetland-forming chronosequence in the Yellow River Delta of China: levels, sources and toxic risks, Ecol. Indicat., 2016, 69, 331–339 CrossRef CAS.
- L. Wang, N. A. Coles, C. Wu and J. Wu, Spatial variability of heavy metals in the coastal soils under long-term reclamation, Estuarine, Coastal Shelf Sci., 2014, 151, 310–317 CrossRef CAS.
- Z. Atafar, A. Mesdaghinia, J. Nouri, M. Homaee, M. Yunesian and M. Ahmadimoghaddam, et al., Effect of fertilizer application on soil heavy metal concentration, Environ. Monit. Assess., 2010, 160(1–4), 83 CrossRef CAS.
- H. Yu, S.-J. Ni, Z.-W. He, C.-J. Zhang, X. Nan and B. Kong, et al., Analysis of the spatial relationship between heavy metals in soil and human activities based on landscape geochemical interpretation, J. Geochem. Explor., 2014, 146, 136–148 CrossRef CAS.
- M. Li, W. Yang, T. Sun and Y. Jin, Potential ecological risk of heavy metal contamination in sediments and macrobenthos in coastal wetlands induced by freshwater releases: a case study in the Yellow River Delta, China, Mar. Pollut. Bull., 2016, 103(1–2), 227–239 CrossRef CAS.
- A. Frantz, M.-A. Pottier, B. Karimi, H. Corbel, E. Aubry and C. Haussy, et al., Contrasting levels of heavy metals in the feathers of urban pigeons from close habitats suggest limited movements at a restricted scale, Environ. Pollut., 2012, 168, 23–28 CrossRef CAS.
- J. Liu, P. Wang, C. Wang, J. Qian and J. Hou, Heavy metal pollution status and ecological risks of sediments under the influence of water transfers in Taihu Lake, China, Environ. Sci. Pollut. Res., 2017, 24(3), 2653–2666 CrossRef CAS.
- I. Castro, J. Aboal, J. Fernández and A. Carballeira, Use of raptors for biomonitoring of heavy metals: gender, age and tissue selection, Bull. Environ. Contam. Toxicol., 2011, 86(3), 347–351 CrossRef CAS.
- N. A. Abbasi, V. L. B. Jaspers, M. J. I. Chaudhry, S. Ali and R. N. Malik, Influence of taxa, trophic level, and location on bioaccumulation of toxic metals in bird's feathers: a preliminary biomonitoring study using multiple bird species from Pakistan, Chemosphere, 2015, 120, 527–537 CrossRef CAS.
- B. J. Mattsson and R. J. Cooper, Multiscale analysis of the effects of rainfall extremes on reproduction by an obligate riparian bird in urban and rural landscapes, Auk, 2009, 126(1), 64–76 CrossRef.
- N. Gagnon Lupien, G. Gauthier and C. Lavoie, Effect of the invasive common reed on the abundance, richness and diversity of birds in freshwater
marshes, Anim. Conserv., 2015, 18(1), 32–43 CrossRef.
- V. Llabjani, R. N. Malik, J. Trevisan, V. Hoti, J. Ukpebor and Z. K. Shinwari, et al., Alterations in the infrared spectral signature of avian feathers reflect potential chemical exposure: a pilot study comparing two sites in Pakistan, Environ. Int., 2012, 48, 39–46 CrossRef CAS.
- B. He, Z. Yun, J. Shi and G. Jiang, Research progress of heavy metal pollution in China: sources, analytical methods, status, and toxicity, Chin. Sci. Bull., 2013, 58(2), 134–140 CrossRef CAS.
- M. Nie, N. Xian, X. Fu, X. Chen and B. Li, The interactive effects of petroleum-hydrocarbon spillage and plant rhizosphere on concentrations and distribution of heavy metals in sediments in the Yellow River Delta, China, J. Hazard Mater., 2010, 174(1–3), 156–161 CrossRef CAS.
Footnote |
† The two authors contributed equally. |
|
This journal is © The Royal Society of Chemistry 2020 |
Click here to see how this site uses Cookies. View our privacy policy here.