DOI:
10.1039/D0RA08185J
(Paper)
RSC Adv., 2020,
10, 39943-39953
Interactions between arbuscular mycorrhizal fungi and soil properties jointly influence plant C, N, and P stoichiometry in West Lake, Hangzhou†
Received
24th September 2020
, Accepted 28th October 2020
First published on 2nd November 2020
Abstract
Arbuscular mycorrhizal fungi (AMF) play important roles in terrestrial plants via mutualistic symbiosis. However, knowledge about the functions of AMF in aquatic plants remains limited. Here, four dominate emergent plant communities in West Lake, Hangzhou were chosen, the characteristics of AMF, plant C, N, and P stoichiometry, and soil properties were investigated. The results showed that both AMF infection rates and the number of AMF spore species increased, suggesting a great mutualism between AMF and emergent plants. Contents of C, N, and P in aboveground biomass and roots and their ratios varied greatly among these four emergent plants. Moreover, AMF infection frequency showed a significant negative correlation with aboveground biomass N (p < 0.05), whereas the rates of arbuscular mycorrhiza formation and vesicular formation after root infection showed significant negative correlations with root N and root N/P. Soil total C, soil total N, soil total P, and oxidation–reduction potential (ORP) were significantly associated with AMF infection characteristics. Our main findings are that the results of redundancy analysis and path analysis further indicated that soil C, N, and P contents, and ORP affected plant C, N, and P contents and their stoichiometry directly. Meanwhile, soil properties can also regulate plant ecological stoichiometry indirectly via altering AMF mycorrhiza. Our findings highlight that interactions between AMF and soil play crucial roles in regulating plant ecological stoichiometry and can be treated as a whole in investigating the relationships between plant and soil.
1. Introduction
Arbuscular mycorrhizal fungi (AMF), as a kind of obligate soil symbiotic microorganism in the phylum Glomeromycota, exist in the rhizosphere of plants. It is well known that arbuscular mycorrhizas are capable of establishing the most common terrestrial mutualistic symbiosis with approximately 80% of terrestrial plant species.1,2 Numerous studies have reported that AMF exhibit large amounts of beneficial effects on terrestrial plants, such as improving uptake of nutrients from the soil,3 alleviating water stress,4 and enhancing resistance to salinity, pH, heavy metals and drought.5–8
Little attentions have been paid to exploring the roles of AMF in aquatic plants.9 This might be partly ascribed that the relative low concentration of oxygen in the soil of aquatic environments limited the growth of AMF and their symbiosis with plants. Meanwhile, it might be also attributed to the fact that the plants in aquatic ecosystems directly absorb nutrients from water through their roots and shoots rather than AMF. However, in the recent years, AMF are widely found in many species of plants in aquatic ecosystems around the world.10,11 Thus, there is a pressing need to better understand the effects of AMF on aquatic plants.
Ecological stoichiometry focused on the dynamical balance of several chemical elements such as C, N, and P between organisms and their environments.12,13 It successfully integrated into the studies at various levels, from microscopic molecules to macroscopic ecosystems,14 and played an important role in investigating the functions of ecosystems, competition among plants, and nutrition limitation.15,16 The main focus of ecological stoichiometry were plant C, N, and P stoichiometric ratios, which can be affected by many factors such as nutrients, diseases, water and AMF, etc.
AMF can forms symbiotic relationship with plants and improves plant N and P uptake.15 It appeared to be closely related with plant C, N, and P stoichiometry, but this mutualistic symbiosis relationship were likely to be influenced by a series of environmental factors, especially soil properties such as total N, pH, Zn2+ concentration17 and nutrients.10 A previous study reported that C, N, and P contents in soils regulated C, N, and P ecological stoichiometry of aboveground biomass via root uptake of organic matters and different types of nutrients.18 Similarly, leaf N and P contents in trees, shrubs, and herbs species could be affected by soil C/N ratio and pH.19 Moreover, soil N concentration and Mn2+ jointly affected AMF diversity and colonization in Boehmeria nivea root.20 Additionally, Collins & Foster (2009) found that AMF played a vital role for mediating plant community diversity at lower soil P level.21 Thus, a better comprehensive knowledge of interaction between AMF and soil will be conductive to uncover the effects of AMF on aquatic plants.
In the last four decades of twenty century, the quality of West Lake water, located in Hangzhou of China as a China-internationally renowned tourist and cultural base, was affected by the activities related to the fast economic development. The deterioration of water quality was mainly due to the relative high ratio of heavy fraction organic matter (HFOM)/light fraction organic matter (LFOM) rather than zinc, boron or manganese in the lake sediments.22 In the current study, we conducted four kinds of emergent plants and rhizosphere soil samples from four different sites in the West Lake. The AMF infection characteristics, C, N, and P contents and their stoichiometric ratios in emergent plants, and soil properties were investigated. We expected that arbuscular mycorrhizal fungi, soil properties, and their interactions jointly regulated C, N, and P stoichiometry in emergent plants. The objectives of this study were as following: (1) to investigate the AMF infection characteristics in emergent plants; (2) to evaluate the correlations among AMF infection characteristics, plant C, N, and P stoichiometry, and soil properties; (3) to elucidate potential pathways of the effect of AMF infection characteristics on plant C, N, and P stoichiometry when soil properties was considered.
2. Materials and methods
2.1 Study site description
West Lake located in the west region of urban area of Hangzhou, China, with a lake area of 6.38 km2. It is surrounded by mountains except the east side. The nearly oval lake owns a flat and shallow bottom with an average depth of 2.27 m. For the west Lake, its average annual total solar radiation ranged from 100 to 110 kcal cm−1,2 whereas its average annual sunshine hours ranged from 1800 to 2100 hours. From the 1950s to the 1980s, due to the rapid economic development and the increasing pressure of the West Lake tourism load, water quality for West Lake was worsening. The eutrophication of the water body frequently occurred, cyanobacteria multiplied, the aquatic vegetation system collapsed, and the water environment deteriorated. Although water quality of West Lake has been improved as a result of ecological restoration projects in the past four decades, further studies were still needed to remediate the water quality and elucidate the underlying mechanisms.
2.2 Field sampling
Four artificial emergent plants restoration sites Jin Sha Gang (S1), Mao Jia Bu (S2), Wu Gui Tan (S3), Yu Hu Wan (S4) were chosen (Fig. 1). The dominated emergent plants Phragmites australis, Zizania latifolia, Scirpus validus, and Lythrum salicaria and corresponding surface soil samples (0–10 cm depth) were collected from four sites S1–S4, respectively. In each site, three plots of 2 m × 2 m (length × width) following similar environmental characteristics and vegetation were established. Plants derived from three 0.25 m2 quadrats at random locations within every sampling sites were selected and divided into two different parts: aboveground biomass (including the leaf and stem) and root. Root systems with adherent soils were carefully collected to protect the feeder roots, sealed in polyethylene bags and brought back to the lab at 4 °C for further analysis.
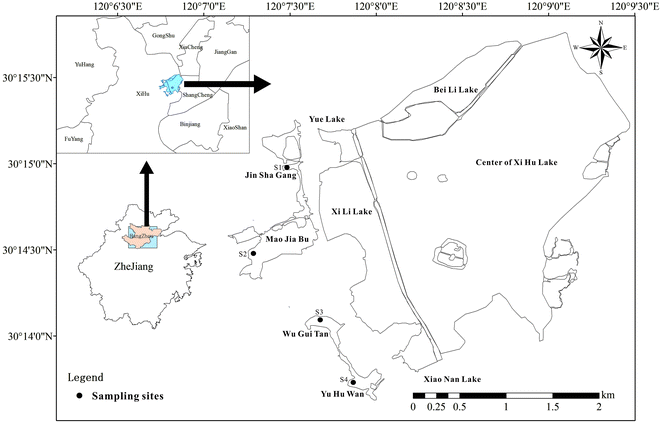 |
| Fig. 1 Locations of four sampling sites along the West Lake, Hangzhou, China. | |
2.3 AMF infection in roots of emergent plants
To determine the characteristics of AMF infection in the roots of emergent plants, the rates of AMF infection were calculated as previously reported.23 Briefly, the roots were cut into 1.0 cm segments, fixed in 3% glutaraldehyde at 4 °C for 24 h, washed thrice in 0.1 M sodium cacodylate buffer followed by a dehydration in a graded series of acetone. The stained root segments suspended in lactoglycerol was randomly chosen, spread in a 60 mm Petri dish, and observed under a stereomicroscope Nikon Eclipse 80i (Nikon, Tokyo, Japan). The different colonization patterns such as the structures of arbuscular, vesicular, and hyphae were investigated. The different colonization patterns such as the frequency of infection (F), the rate of infection (R), the rate of arbuscular formation (AR), and the rate of vesicular formation (VR) were performed using the magnified intersection method.24
2.4 Isolation and identification of AMF spore in soils
The isolation of AMF spores were performed using wet-sieving and sucrose density gradient centrifugation methods.25 Briefly, AMF spores were picked up using a micropipetter under a stereomicroscope from 10 g soil. The isolated spores were identified morphologically based on their sizes, colors, surface ornamentation, spore contents and wall structure.26
2.5 Chemical analysis of plants
The plants were washed thrice with deionized water, dried at 105 °C for 15 min and 80 °C until reaching constant weight.27 Then, the dried plant samples were grounded, sieved, and aliquoted for further analysis. After digestion in sulfuric acid, total C (TC) and total N (TN) in the plants were analyzed using a high sensitivity elemental analyzer system (Vario TOC cube, Hanau, Germany), whereas total P (TP) contents were digested by sulfuric acid and analyzed via a spectrophotometer using the molybdenum blue method (UV-1900, SHIMADZU, Japan).28 Plant TC, TN, and TP contents were presented as mg TC/TN/TP per g plant dry weight.
2.6 Chemical analysis of soils
The soils were air dried for 30 days, ground, and sieved. Soil total C (STC), soil total N (SCN), and soil total P (STP) were determined as above mentioned. In order to measure the contents of STP, the soils were heated at 450 °C for 3 h, digested in muriatic acid for 16 h, and determined by the ascorbic acid method.29 Soil STC, STN, and STP contents were presented as mg STC/STN/STP per g soil dry weight. Oxidation–reduction potential (ORP) were determined using an Orion 5-Star Portable Multimeter. Soil pH was determined using a pH/conductivity meter using a soil-to-water solution diluted in 1
:
5.
2.7 Statistics
The normality of data distribution was firstly assessed using the Shapiro–Wilk test. When necessary, data were logarithmically or Box-Cox transformed to achieve normality. One-way ANOVAs were performed to analyze the differences among AMF infection characteristics, plant C, N, and P contents and their stoichiometric ratios in four types of emergent plants, followed by a Tukey post-hoc tests. Pearson correlation coefficient's r, with p < 0.05, were utilized to determine the correlations between AMF infection characteristics and plant stoichiometric ratios or soil properties. Then, redundancy analysis (RDA) were employed to determine relative contributions of soil properties to the composition of plant communities using the Canoco for Windows 4.5 package. Statistical procedures were performed using the SPSS 20.0 software. Differences were declared significant at P < 0.05 unless otherwise stated.
To further investigate potential direct and/or indirect effects of crucial explanatory variables on AMF infections in the roots of emergent plants, we have performed path analysis using full information maximum likelihood estimation method.30 Firstly, a conceptual path model has been built on the basis of the literature and the author's experience of wetland restoration (Fig. S1†). Secondly, potential explanatory variables were chosen and used in the path analysis on the basis of Pearson's analysis and RDA. Correspondence analysis (CA) was performed to reduce variable numbers of AMF infection characteristics, soil properties, plant stoichiometry (Table S1†). The first principal component for each CA analysis was assigned into the next path analysis. Thirdly, the path coefficients, direct and indirect effects, and the model fit parameters were analyzed using AMOS version 20.0 software packages. The path model finally confirmed was considered as acceptable fit to the data when the value for the comparative fit index was greater than 0.9 and root square error of approximation was less than 0.1, respectively.
3. Results
3.1 Determination of AMF infection characteristics and the diversity of AMF spores
Infection characteristics of AMF in roots of emergent plants were shown in Table 1. The infection frequencies (F) of AMF in Phragmites australis (85.67%) and Phalaris arudicacea (87.79%) were significantly lower than those in Scirpus validus (97.73%) and Lythrum salicaria groups (97.53%) (p < 0.05). Meanwhile, the highest infection rate (R) and the rate of arbuscular mycorrhiza formation (AR) of four emergent plants were detected in Lythrum salicaria (87.38% and 82.24%). For the rate of vesicle formation (VF), the values in Phragmites australis and Scirpus validus were 55.04% and 44.22%, which were considerably lower than those in Phalaris arundinacea (79.19%) and Lythrum salicaria (73.19%) (p < 0.05).
Table 1 AMF infection characteristics of emerged plant in lakeshore wet zonea
Plants |
Types |
AMF |
F |
R |
AR |
VR |
F: infection frequency (%); R: infection rate (%); AR: rate of arbuscular mycorrhiza formation after root infection (%); FR: rate of vesicle formation after root infection (%). The different lowercase letters indicated significant differences (p < 0.05) among different plant communities. |
Phragmites australis |
Intermediate |
85.67 ± 3.05a |
67.49 ± 2.40b |
62.56 ± 2.23a |
55.04 ± 1.96b |
Phalaris arundinacea |
Intermediate |
87.79 ± 4.88a |
39.01 ± 2.17a |
70.93 ± 3.94a |
79.19 ± 4.40c |
Scirpus validus |
Arum |
97.93 ± 3.58b |
76.53 ± 3.41c |
65.85 ± 2.93a |
44.22 ± 1.97a |
Lythrum salicaria |
Arum |
97.53 ± 4.27b |
87.38 ± 5.00d |
82.84 ± 4.74b |
73.19 ± 4.18c |
AMF spores was isolated and identified from the rhizosphere soil of the plants (Fig. 2). There were 24 types of known species of AMF spores. Among them, the most abundant spore species was Glomus melanosporum, which was 24.58 in 100 g dry soil and accounted for 25.10% in the whole spores. Meanwhile, there were five other types of spore species, Scutellospora nigra, Glomus mosseae, Scutellospora calospora, Acaulospora dilatata, and Glomus aureum, whose ratios were 13.77%, 12.78%, 3.93%, 3.34%, and 3.05%, respectively.
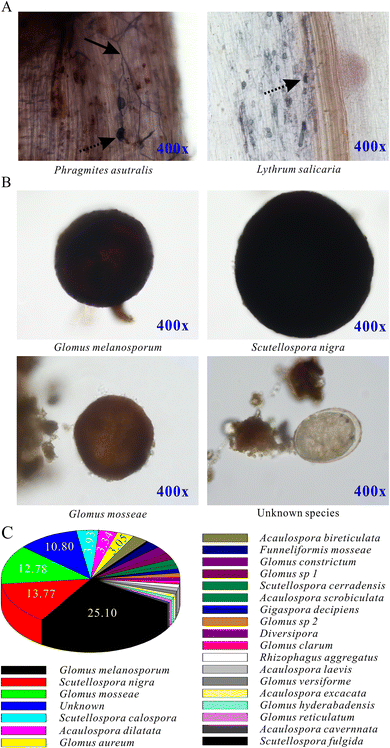 |
| Fig. 2 Infection characteristics of AMF in emerged aquatic plants in West Lake. (A) Representative microscopic images of typical AMF infection in emerged aquatic plants Phragmites australis and Lythrum salicaria. Solid arrow: dark septate hyphae; dotted arrow: vesicle. Magnifications: 400×; (B) representative images of AMF spores observed in the roots of emerged aquatic plants. Magnifications: 400×; (C) biodiversity and relative ratios of AMF spore species in emerged plants. | |
3.2 Measurement of soil properties
The concentrations of STC and STN in root rhizosphere soils ranged from 38.29 g kg−1 to 130.33 g kg−1, and 1.12 g kg−1 to 5.07 g kg−1, respectively, and the highest contents were detected in Lythrum salicaria (Table 2). Meanwhile, the contents of STP in root rhizosphere soils varied from 0.67 g kg−1 to 2.11 g kg−1, while the value in Phragmites australis was significantly higher compared with those in the other three emergent plants. Soil ORP and pH covered the range from −35.19 to 34.32, and 6.19 to 7.16, respectively. Phragmites australis had the lowest soil ORP, while there was no significant differences in pH among the four emergent plants.
Table 2 Soil properties in the rhizosphere of emerged plants in lakeshore wet zonea
Plants |
STC (g kg−1) |
STN (g kg−1) |
STP (g kg−1) |
OPR |
pH |
The different lowercase letters indicated significant differences (p < 0.05) among different plant communities. |
Phragmites australis |
38.29 ± 4.29a |
1.12 ± 0.21a |
2.11 ± 0.27c |
−35.19 ± 3.07a |
6.19 ± 0.65 |
Phalaris arundinacea |
57.84 ± 11.87ab |
1.77 ± 0.35a |
1.62 ± 0.09b |
26.65 ± 10.18b |
6.89 ± 0.30 |
Scirpus validus |
83.30 ± 6.30b |
3.21 ± 0.76b |
1.27 ± 0.08b |
34.32 ± 2.76b |
7.06 ± 0.33 |
Lythrum salicaria |
130.33 ± 28.08c |
5.07 ± 0.69c |
0.67 ± 0.20a |
32.49 ± 8.37b |
7.16 ± 0.39 |
3.3 C, N, and P contents and their stoichiometric ratios in emergent plants
For the aboveground biomass of emergent plants, their average contents of C, N, and P elements were 439.88 g kg−1, 16.06 g kg−1, and 2.20 g kg−1, respectively. Meanwhile, for the roots of emergent plants, their contents of C, N, and P elements averaged at 405.82 g kg−1, 11.60 g kg−1, and 2.03 g kg−1, respectively (Fig. 3). For the C content, the value of Phragmites australis in aboveground biomass was higher compared with those in the other three emergent plants, while the highest C content in root has been observed in Lythrum salicaria. The N content in aboveground biomass of Phragmites australis was significantly higher compared with those of the other three emergent plants, and Scirpus validus the highest value in root. Besides, the P values of Zizania latifolia in aboveground biomass and root were remarkably higher compared with those of the other three groups.
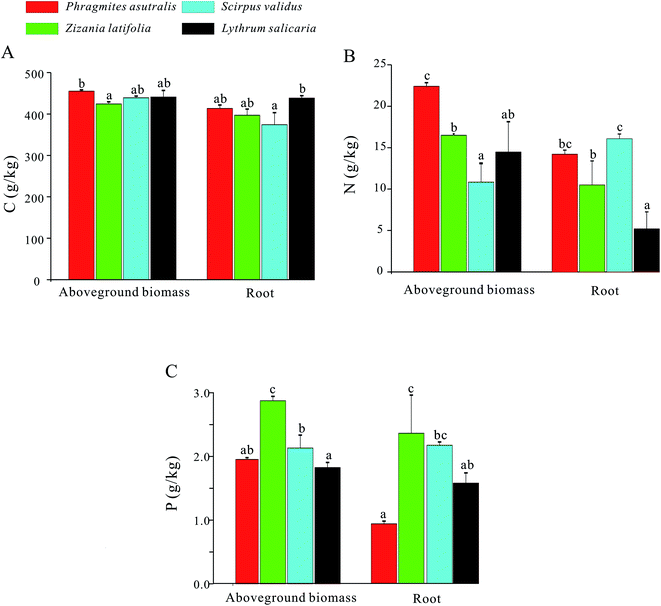 |
| Fig. 3 C, N, and P contents of the emerged plants in West Lake. (A) C contents of the aboveground biomass (left panel) and roots (right panel) of the emerged plants, Phragmites australis, Zizania latifolia, Scirpus validus, and Lythrum salicaria; (B) the N contents of the aboveground biomass (left panel) and roots (right panel) of the emerged plants, Phragmites australis, Zizania latifolia, Scirpus validus, and Lythrum salicaria; (C) the P contents of the aboveground biomass (left panel) and roots (right panel) of the emerged plants, Phragmites australis, Zizania latifolia, Scirpus validus, and Lythrum salicaria. | |
For the C/N, the ratio of Scirpus validus in aboveground biomass was significantly higher compared with those in the other three groups, while the highest C/N ratio in root has been observed in Lythrum salicaria (Fig. 4). Meanwhile, the C/P ratio in aboveground biomass of Lythrum salicaria was remarkably higher than those of the other three groups, and Phragmites australis had the highest value in root. Besides, the N/P ratios of Phragmites australis in both aboveground biomass and root were considerably higher than those of the other three groups.
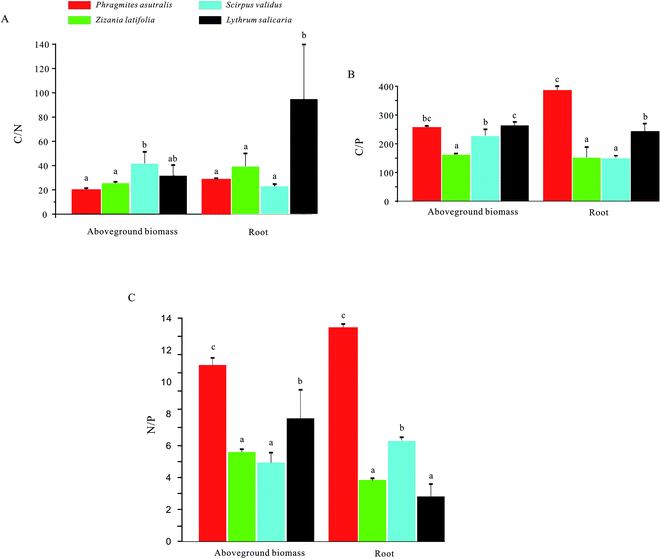 |
| Fig. 4 C, N, and P stoichiometry of the emerged plants in West Lake. (A) The C/N ratios of the aboveground biomass (left panel) and roots (right panel) of the emerged plants, Phragmites australis, Zizania latifolia, Scirpus validus, and Lythrum salicaria, respectively; (B) the C/P ratios of the aboveground biomass (left panel) and roots (right panel) of the emerged plants, Phragmites australis, Zizania latifolia, Scirpus validus, and Lythrum salicaria, respectively; (C) the N/P contents of the aboveground biomass (left panel) and roots (right panel) of the emerged plants, Phragmites australis, Zizania latifolia, Scirpus validus, and Lythrum salicaria, respectively. | |
3.4 Relationships among AMF infection characteristics, plant C, N, P stoichiometry, and soil properties
AMF infection frequency exhibited a significant and negative correlation with the content of N in aboveground biomass (Table 3). The rate of infection was significantly positive associated with the aboveground biomass P and aboveground biomass C/P (p < 0.01). Meanwhile, both AR and FR exhibited significant and negative relationships with root N, and root N/P, respectively (p < 0.01). Besides, root C/N was positively related to AR, and negatively correlated with FR (p < 0.01).
Table 3 Pearson correlation coefficients between AMF infection characteristics and C, N, and P contents and their stoichiometric ratios of plant communitiesc
Items |
AMF infection characteristics |
F (%) |
R (%) |
AR (%) |
FR (%) |
p < 0.05. p < 0.01. F: infection frequency (%); R: infection rate (%); AR: rate of arbuscular mycorrhiza formation after root infection (%); FR: rate of vesicular formation after root infection (%). |
Aboveground biomass C (g kg−1) |
−0.049 |
0.528 |
−0.194 |
−0.445 |
Root C (g kg−1) |
−0.002 |
0.326 |
0.573 |
0.478 |
Aboveground biomass N (g kg−1) |
−0.607a |
−0.237 |
−0.223 |
0.189 |
Root N (g kg−1) |
−0.205 |
−0.183 |
−0.864b |
−0.768b |
Aboveground biomass P (g kg−1) |
0.324 |
0.873b |
0.198 |
−0.357 |
Root P (g kg−1) |
0.228 |
−0.389 |
0.103 |
0.174 |
Aboveground biomass C/N |
0.566 |
0.344 |
0.054 |
−0.401 |
Root C/N |
−0.209 |
−0.246 |
0.868b |
−0.739b |
Aboveground biomass C/P |
0.275 |
0.858b |
0.146 |
−0.383 |
Root C/P |
−0.417 |
0.251 |
−0.212 |
−0.144 |
Aboveground biomass N/P |
−0.364 |
0.222 |
−0.082 |
0.002 |
Root N/P |
−0.487 |
−0.029 |
−0.810b |
−0.634a |
STC and STN showed significantly positive correlations with AMF infection frequency (p < 0.01), infection rate (p < 0.05), and AR (p < 0.01), respectively (Table 4). Similarly, STP was significantly negatively correlated with AMF infection frequency, infection rate, and AR (p < 0.01), respectively. Besides, ORP had significant positive association with AMF infection frequency and AR, respectively. No significant correlation was detected between pH and infection frequency, infection rate, AR, and FR, respectively.
Table 4 Pearson correlation coefficients between AMF infection characteristics and soil propertiesc
Items |
AMF infection characteristics |
F (%) |
R (%) |
AR (%) |
FR (%) |
p < 0.05. p < 0.01. F: infection frequency (%); R: infection rate (%); AR: rate of arbuscular mycorrhiza formation after root infection (%); FR: rate of vesicle formation after root infection (%). |
STC (g kg−1) |
0.721b |
0.641a |
0.810b |
0.226 |
STN (g kg−1) |
0.739b |
0.688a |
0.770b |
−0.246 |
STP (g kg−1) |
−0.780b |
−0.578a |
−0.820b |
0.147 |
ORP |
0.686a |
0.106 |
0.592a |
0.226 |
pH |
0.347 |
0.326 |
0.221 |
−0.135 |
RDA analysis results in Fig. 5 demonstrated that axis 1 and axis 2 accounted for 42.37% and 29.42% of the total variation, respectively. Soil properties explained 72.9% of the total variation in the contents of C, N, and P elements and their stoichiometric ratios in emergent plants. The results of Monte Carlo permutation test demonstrated that all axes were significant (F-ratio = 3.2, P-value = 0.006). According to the centroid principle and distance rule, the contents of P in soils showed positive correlations with N contents of aboveground biomass, root N contents and root N
:
P ratios. Meanwhile, there were positive associations between soil C and N contents, and root C
:
N ratios and C contents, respectively. Additionally, the aboveground biomass C
:
N ratios and P contents, and root P contents, showed positive correlations with soil ORP and pH, respectively, whereas C content, N/P ratio of aboveground biomass, and root C/P showed significantly negative relationships with soil ORP and pH, respectively (Fig. 5).
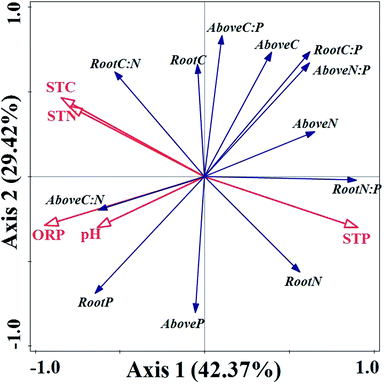 |
| Fig. 5 RDA ordination plots of C, N, and P contents and their stoichiometric ratios of different plants and soil properties in West Lake. Abbreviations: Above C, aboveground biomass C; Above N, aboveground biomass N; Above P, aboveground biomass P; Above C : N, aboveground biomass C : N; Above C : P, aboveground biomass C : P; Above N : P, aboveground biomass N : P. | |
3.5 Direct and indirect effects of explanatory variables on plant C, N, P stoichiometry
To further elucidate the interaction between AMF and soil properties jointly influences plant C, N, and P stoichiometry, pathway analysis was performed and shown in Fig. 6. In the path model, soil C, N, and P contents and ORP could regulate plant C, N, and P stoichiometry both directly and indirectly. Soil C, N, and P contents had significant direct influences on plant C, N, and P contents and AMF infection characteristics. Similarly, ORP showed strong and significant negative effects on both plant C, N, and P contents and their stoichiometric ratios. Besides, AMF infection characteristics had relatively large but nonsignificant effects on both plant C, N, and P contents and their stoichiometric ratios.
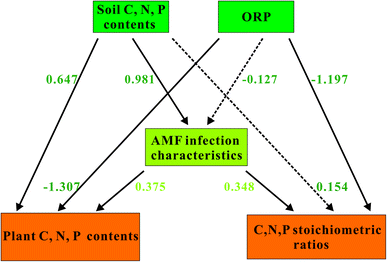 |
| Fig. 6 Path diagrams estimating the effects of soil environmental factors, and AMF infection characteristics on plant C, N, and P contents and their stoichiometric ratios in emerged plants. Solid lines indicate significant effects (p < 0.05), while dashed lines demonstrate insignificant effects. The values for standard path coefficients were marked adjacent to the arrows. | |
4. Discussion
4.1 AMF infection characteristics, spore count, and diversity
Our results demonstrated that both AMF infection frequencies (>85.67%) and infection rates (39.01–87.38%) in four emergent plants were relative high (Table 1), suggesting a potential good mutualistic symbiosis between AMF and emergent plants. A total of 28 AMF spore species observed in emergent plants in the present study was nearly the same as that (29 species) reported in soils from three plantation sites in Meghalaya, northeast India.31 Compared with approximately 240 recognized AMF species,32 the number of AMF spores observed in the current study were relative high due to the lack of host specificity.33 Meanwhile, the number of AMF spore species observed here was also higher than the numbers of those (21–23) reported in the Tibet Plateau.34,35 This difference on the AMF spore diversity may be due to the altitude, which was negatively related to the diversities of AMF.36–38 Moreover, the AMF infection rates observed in the present study were much higher than those reported in acidic soils (21–43.67%).39,40 This discrepancy may be ascribed to the differences in pH, which may negatively affect root colonization in the acidic range.41 The pH values of plant rhizosphere soils in this study were all close to 7.0, which was beneficial for the growth of AMF and plants. However, it should be noted that many other factors such as temperature,42,43 light,44 plant competitors45 and pathogens46 may also be involved in determining the mutualism between AMF and emergent plants.
4.2 Correlations between AMF and plant C, N, and P contents and their stoichiometry
C, N, and P contents and their stoichiometric ratios were powerful indicators of multiple ecological processes, which played important roles in physiological traits47 and the evaluation of nutrient limitation for plant growth.48 For example, an N
:
P ratio >16 might suggest a limitation of P element, while the value <14 meant a limitation of N element.49,50 In the current study, all values of plant N
:
P ratios were <12, suggesting the presence of N limitation in these plants. This revealed that limited supply of N may be a possible explanation of high infection rates of AMF observed in the present study, which promote the uptake of N element and plant growth.51 Our results supported the conclusion of Vries et al. (2011), who reported that a higher AMF biomass was probably the result of low N concentration rather than the direct cause of higher N retention in two grassland soils.52 Moreover, AMF infection rates (R) exhibited a significant positive relationship with P contents in aboveground biomass, suggesting that mycorrhizal growth response in plants was mainly due to increased photosynthesis and P allocation in the aboveground biomass (Table 3). Additionally, high AMF infection rates increased uptake of other growth-limiting nutrients such as K+, and then enhanced the uptake of P and plant growth.39
The negative correlations between AR/FR rates and root N/root N/P shown in Table 3, indicated that high AR/FR rates of AMF reduced the allocation of N element in the plant roots. This reduced N allocation was consistent with the observation that AMF negatively affects nitrogen acquisition and grain yield of maize in a N-deficient soil.53 Meanwhile, N limitation in soils can impair plant control over the AMF-plant symbiosis and result in the a shift of symbiosis to commensalism even parasitism.54 Therefore, homeostatic regulation of C
:
N
:
P ratio rather than simple N supply in the soil of West Lake is necessary to improve the interaction between AMF and plants. This was because that a reduction of N content below the minimum requirement for maintaining cell functions resulted in the senescence of tissues,55 whereas excessive N supply will lead to toxic effects in plants, especially those adapted to nutrient-poor soils.56 In addition, it's also generally acknowledged that the magnitude of the effect of AMF on the performance of plant species was species specific.57 Therefore, the exact relationship between AMF infection characteristics and plant C, N, and P contents and stoichiometric ratios in emergent plants still needs further investigations.
4.3 Relationship between AMF biodiversity and soil properties
The relationship between AMF and soil properties was interdependent. On the one hand, AMF can provide multiple benefits for the soils. A previous study reported that AMF can enhance the health conditions of soil by regulating soil food web and maintaining soil structure through their external hyphae.58 Similarly, AMF can regulate soil respiration and its response to precipitation changes a semiarid steppe.59 Moreover, the presence of AMF in soil can induce shifts in soil microbial communities, such as N-cycling related denitrification organisms,60 which also participated in the reduction of N loss. On the other hand, based on the trade balance model hypothesized by Johnson, the functions of AMF symbiosis mainly depended on N, P availability and their stoichiometric ratios.61 According to Johnson's theory, the mutualistic benefits were mostly expected when the contents of N and P were high and low, respectively, in the rhizosphere soils. However, the contents of both N and P in the soils were low, both the frequencies and rates of AMF infection were very high in three of four sampled plants in the present study. The content of P < 3 g kg−1 observed in the current study was higher than that in Lythrum salicaria L., where it was colonized by AMF only when the content of P in hydroponic sand culture was below 1 g L−1.62 The discrepancy on the AMF infection rates between our experiment and White's may be ascribed to the limited levels of P availability in Lythrum salicaria L., which were only set at 0, 0.1, 1, 10, and 47.5 g PO4 per L, respectively.62 Meanwhile, Pearson's analysis in Table 4 demonstrated that ORP showed significant and positive correlations with AMF infection frequency and rate of AR, respectively (p < 0.05). This positive correlation may be due to that a higher ORP means relative sufficient oxygen, which will promote the growth of eutrophic fungi AMF.
4.4 Interactions between AMF and soil properties altering plant C, N, and P stoichiometry
Our results of RDA analysis in Fig. 5 demonstrated that the soil properties explained 72.9% of the total variation in the contents of C, N, and P and their stoichiometric ratios in plants. These data suggest that soil properties affect the plant C, N, and P contents and their stoichiometric ratios. This conclusion was consistent with the findings in plants, where C, N, and P ecological stoichiometric ratios of plant aboveground biomass were regulated by STC, STN and STP.18,27 Meanwhile, the result of path analysis in Fig. 6 demonstrated that soil ORP also exerted significant and negative effect on plant C, N, and P contents (−1.307) and their stoichiometry (−1.197), respectively. This could be attributed to that a lower ORP would be beneficial for the metabolism of the plant, which in turn promoted the uptake of soil nutrient especially C, N, and P.63 These data revealed that soil properties can alter plant ecological stoichiometry directly.
The West Lake as an urban lake with a long history, was mainly polluted by high organic matter pollution load with a high degree of sediment humification.22 Meanwhile, the contents of N, P in the sediments of the West Lake were also very high and exerts important impact on the release of endogenous nitrogen and phosphorus.22 These characteristics of West Lake sediments increased the difficulty of lake water restoration. Moreover, we found that the pH of rhizosphere soil of emergent plants mainly ranged from 6.89 to 7.16, which showed no significant correlation with the C, N, and P stoichiometry or AMF infection characteristics in this study (Table 2). Based on these information, the control of endogenous nitrogen and phosphorus release need to be take into consideration during the future of West Lake water restoration.
The result of path analysis in Fig. 6 demonstrated that STC, STN, and STP (0.981) had positive and significant effects on AMF. Meanwhile, the effects of AMF on plant C, N, and P contents (0.375) and stoichiometry (0.348) was marginally significant. These data suggested that soil properties could also affect plant ecological stoichiometry via AMF indirectly, which may be a possible explanation for the unexplained variations in plant C, N, and P contents and their stoichiometry. Meanwhile, our results were in consist with that of Battini et al., who reported that AMF and their associated bacteria could enhance the growth and P uptake in maize.64
Together, soil properties can affect the plant ecological stoichiometry directly and indirectly via the AMF mycorrhizal. Interactions between soil properties and AMF also play a crucial role in regulating plant ecological stoichiometry, which may be a possible explanation for the uncertain roles of AMF in different ecological systems. Based on the tight relation between soil and AMF, we propose that soil and AMF can be treated as a whole in exploring their effects on plant ecological stoichiometry and/or performances when necessary.
5. Conclusions
In summary, our data demonstrated that the frequencies and rates of AMF infection in emergent plants in West Lake, Hangzhou, China were relatively high. Both AMF infection rates and AMF spore species showed a high diversity, suggesting a good mutualism between AMF and plant. The limitation of N element in the rhizosphere soil reduced the N/P ratio to promote the growth of aboveground biomass. Soil properties can affect the plant ecological stoichiometry directly and indirectly via the AMF mycorrhizal pathway. Therefore, AMF and plant root rhizosphere soil should be considered as a whole in exploring the factors, which affected the growth and fitness of plants. This new perspective will be helpful in clarifying the relationships among AMF, plants and soils and providing theoretical support for the restoration and reconstruction of lake aquatic vegetation. Further investigations are needed to the elucidate the possible relations between C
:
N
:
P stoichiometry and compounds/biomolecules, which could be considered as possible biomarkers of the infection process of emergent plants by the AMF.
Conflicts of interest
There are no conflicts to declare.
Acknowledgements
This work was supported by the National Natural Science Foundation of China [Grant number 51709255]; the CRSRI Open Research Program [grant number CKWV2019769/KY]; and Key Laboratory Construction of Hubei Province [grant number 2018BFC360].
References
- Z. Liu, L. Ma, X. He and C. Tian, Water strategy of mycorrhizal rice at low temperature through the regulation of PIP aquaporins with the involvement of trehalose, Appl. Soil Ecol., 2014, 84, 185–191 CrossRef.
- I. C. Dodd and F. Perez-Alfocea, Microbial amelioration of crop salinity stress, J. Exp. Bot., 2012, 63, 3415–3428 CrossRef CAS.
- M. W. Ansari, D. K. Trivedi, R. K. Sahoo, S. S. Gill and N. Tuteja, A critical review on fungi mediated plant responses with special emphasis to Piriformospora indica on improved production and protection of crops, Plant Physiol. Biochem., 2013, 70, 403–410 CrossRef CAS.
- M. Ghorchiani, H. Etesami and H. A. Alikhani, Improvement of growth and yield of maize under water stress by co-inoculating an arbuscular mycorrhizal fungus and a plant growth promoting rhizobacterium together with phosphate fertilizers, Agric., Ecosyst. Environ., 2018, 258, 59–70 CrossRef CAS.
- K. Bencherif, A. Boutekrabt, J. Fontaine, F. Laruelle, Y. Dalpè and A. Lounès-Hadj Sahraoui, Impact of soil salinity on arbuscular mycorrhizal fungi biodiversity and microflora biomass associated with Tamarix articulata Vahll rhizosphere in arid and semi-arid Algerian areas, Sci. Total Environ., 2015, 533, 488–494 CrossRef CAS.
- A. Camprubi, M. Abril, V. Estaun and C. Calvet, Contribution of arbuscular mycorrhizal symbiosis to the survival of psammophilic plants after sea water flooding, Plant Soil, 2012, 351, 97–105 CrossRef CAS.
- J. D. He, T. Dong, H. H. Wu, Y. N. Zou, Q. S. Wu and K. Kamil, Mycorrhizas induce diverse responses of root TIP aquaporin gene expression to drought stress in trifoliate orange, Sci. Hortic., 2019, 243, 64–69 CrossRef CAS.
- M. H. Ryan and J. H. Graham, Little evidence that farmers should consider abundance or diversity of arbuscular mycorrhizal fungi when managing crops, New Phytol., 2018, 220, 1092–1107 CrossRef.
- H. Peat and A. H. Fitter, The distribution of arbuscular mycorrhizas in the British flora, New Phytol., 1993, 125, 845–854 CrossRef.
- Z. Xu, Y. Ban, Y. Jiang, X. Zhang and X. Liu, Arbuscular Mycorrhizal Fungi in Wetland Habitats and Their Application in Constructed Wetland: A Review, Pedosphere, 2016, 26, 592–617 CrossRef.
- M. Søndergaard and S. Laegaard, Vesicular–arbuscular mycorrhiza in some aquatic vascular plants, Nature, 1977, 268, 232–233 CrossRef.
- M. Long, L. Guo, L. Jing, Y. Cui and S. He, Effects of water and exogenous Si on element concentrations and ecological stoichiometry of plantain (Plantago lanceolata L.), J. Plant Nutr., 2018, 41, 1–13 CrossRef.
- A. T. Austin and P. M. Vitousek, Introduction to a Virtual Special Issue on ecological stoichiometry and global change, New Phytol., 2012, 196, 649–651 CrossRef.
- H. Sun, Q. Li, Z. Lei, J. Zhang, X. Song and X. Song, Ecological stoichiometry of nitrogen and phosphorus in Moso bamboo (Phyllostachys edulis) during the explosive growth period of new emergent shoots, J. Plant Res., 2019, 132, 107–115 CrossRef.
- N. C. Johnson, Resource stoichiometry elucidates the structure and function of arbuscular mycorrhizas across scales, New Phytol., 2010, 185, 631–647 CrossRef CAS.
- Z. Y. Yuan and H. Y. H. Chen, Decoupling of nitrogen and phosphorus in terrestrial plants associated with global changes, Nat. Clim. Change, 2015, 5, 465–469 CrossRef CAS.
- X. Xu, C. Chen, Z. Zhang, Z. Sun, Y. Chen, J. Jiang and Z. Shen, The influence of environmental factors on communities of arbuscular mycorrhizal fungi associated with Chenopodium ambrosioides revealed by MiSeq sequencing investigation, Sci. Rep., 2017, 7, 45134 CrossRef CAS.
- X. Zhou, M. A. Bowker, Y. Tao, L. Wu and Y. Zhang, Chronic nitrogen addition induces a cascade of plant community responses with both seasonal and progressive dynamics, Sci. Total Environ., 2018, 626, 99–108 CrossRef CAS.
- H. Gong, Y. Li, T. Yu, S. Zhang, J. Gao, S. Zhang and D. Sun, Soil and climate effects on leaf nitrogen and phosphorus stoichiometry along elevational gradients, Glob. Ecol. Conserv., 2020, 23, e01138 CrossRef.
- Y. Wei, Z. Chen, F. Wu, J. Li, Y. ShangGuan, F. Li, Q. R. Zeng and H. Hou, Diversity of Arbuscular Mycorrhizal Fungi Associated with a Sb Accumulator Plant, Ramie (Boehmeria nivea), in an Active Sb Mining, J. Microbiol. Biotechnol., 2015, 25, 1205–1215 CrossRef CAS.
- C. D. Collins and B. L. Foster, Community-level consequences of mycorrhizae depend on phosphorus availability, Ecology, 2009, 90, 2567–2576 CrossRef.
- J. Li, G. Zhu, M. Zhu, Z. Gong, H. Xu and G. Yang, Composition and Environmental Effects of LFOM and HFOM in “Incense-Ash” Sediments of West Lake, Hangzhou, China (In Chinese), Environ. Sci., 2015, 36, 2038–2045 Search PubMed.
- B. Biermann and R. G. Linderman, Quantifying vercular-arbuscular mycorrhizas: Aproposed method towards standardization, New Phytol., 1981, 87, 63–67 CrossRef.
- M. Giovannetti and B. Mosse, An Evaluation of Techniques for Measuring Vesicular Arbuscular Mycorrhizal Infection in Roots, New Phytol., 1980, 84, 489–500 CrossRef.
- B. A. Daniels and H. D. Skipper, in Methods and principles of mycorrhizal research, ed. N. C. Schenck, American Phytopathological Society, St. Paul, Minn, 1982, pp. 29–35 Search PubMed.
- N. Schenck and Y. Perez, Manual for the identification of va mycorrhizal fungi, Synergistic Publications, Gainesville, Florida, 1990 Search PubMed.
- M. F. Yu, Y. Tao, W. Liu, W. Xing, G. Liu, L. Wang and L. Ma, C, N, and P stoichiometry and their interaction with different plant communities and soils in subtropical riparian wetlands, Environ. Sci. Pollut. Res., 2020, 27, 1024–1034 CrossRef CAS.
- C. Xie, J. Xu, J. Tang, S. A. Baig and X. Xu, Comparison of Phosphorus Determination Methods by Ion Chromatography and Molybdenum Blue Methods, Commun. Soil Sci. Plant Anal., 2013, 44, 2535–2545 CrossRef CAS.
- J. Murphy and J. P. Riley, A modified single solution method for the determination of phosphate in natural waters, Anal. Chim. Acta, 1962, 27, 31–36 CrossRef CAS.
- L. Ma, X. Jiang, G. Liu, L. Yao, W. Liu, Y. Pan and Y. Zuo, Environmental factors and microbial diversity and abundance jointly regulate soil nitrogen and carbon biogeochemical processes in Tibetan wetlands, Environ. Sci. Technol., 2020, 54, 3267–3277 CrossRef CAS.
- K. H. Das Panna, Mycorrhizal colonization and distribution of arbuscular mycorrhizal fungi associated with Michelia champaca L. under plantation system in northeast India, J. For. Res., 2010, 21, 137–142 CrossRef.
- M. Krüger, C. Krüger, C. Walker, H. Stockinger and A. Schüssler, Phylogenetic reference data for systematics and phylotaxonomy of arbuscular mycorrhizal fungi from phylum to species level, New Phytol., 2012, 193, 970 CrossRef.
- S. E. Smith and F. David Read, Mycorrhizal Symbiosis, 3rd edn, 2008 Search PubMed.
- J. P. Gai, P. Christie, X. B. Cai, J. Q. Fan, J. L. Zhang, G. Feng and X. L. Li, Occurrence and distribution of arbuscular mycorrhizal fungal species in three types of grassland community of the Tibetan Plateau, Ecol. Res., 2009, 24, 1345–1350 CrossRef.
- Y. Liu, J. He, G. Shi, L. An, M. Opik and H. Feng, Diverse communities of arbuscular mycorrhizal fungi inhabit sites with very high altitude in Tibet Plateau, FEMS Microbiol. Ecol., 2011, 78, 355–365 CrossRef CAS.
- J. P. Gai, H. Tian, F. Y. Yang, P. Christie, X. L. Li and J. N. Klironomos, Arbuscular mycorrhizal fungal diversity along a Tibetan elevation gradient, Pedobiologia, 2012, 55, 145–151 CrossRef.
- X. L. Li, J. Gai, X. Cai, X. Li, P. Christie, F. Zhang and J. Zhang, Molecular diversity of arbuscular mycorrhizal fungi associated with two co-occurring perennial plant species on a Tibetan altitudinal gradient, Mycorrhiza, 2014, 24, 95–107 CrossRef.
- M. A. Lugo, M. A. Ferrero, E. Menoyo, M. C. Estevez, F. Sineriz and A. M. Anton, Arbuscular Mycorrhizal Fungi and Rhizospheric Bacteria Diversity Along an Altitudinal Gradient in South American Puna Grassland, Microb. Ecol., 2008, 55, 705–713 CrossRef CAS.
- M. H. Khan, M. K. Meghvansi, R. Gupta and V. Veer, Elemental stoichiometry indicates predominant influence of potassium and phosphorus limitation on arbuscular mycorrhizal symbiosis in acidic soil at high altitude, J. Plant Physiol., 2015, 189, 105–112 CrossRef CAS.
- M. H. Khan, M. K. Meghvansi, R. Gupta, V. Veer, L. Singh and M. C. Kalita, Foliar spray with vermiwash modifies the Arbuscular mycorrhizal dependency and nutrient stoichiometry of Bhut Jolokia (Capsicum assamicum), PLoS One, 2014, 9, e92318 CrossRef.
- R. Kjøller and K. E. Clemmensen, Belowground ectomycorrhizal fungal communities respond to liming in three southern Swedish coniferous forest stands, For. Ecol. Manage., 2009, 257, 2217–2225 CrossRef.
- S. Chen, W. Jin, A. Liu, S. Zhang, D. Liu, F. Wang, X. Lin and C. He, Arbuscular mycorrhizal fungi (AMF) increase growth and secondary metabolism in cucumber subjected to low temperature stress, Sci. Hortic., 2013, 160, 222–229 CrossRef CAS.
- M. E. Gavito, P. A. Olsson, H. Rouhier, A. Medina-Peñafiel, I. Jakobsen, A. Bago and C. Azcón-Aguilar, Temperature constraints on the growth and functioning of root organ cultures with arbuscular mycorrhizal fungi, New Phytol., 2005, 168, 179–188 CrossRef CAS.
- H. K. Gamage and S. M. S. Ashton, Effects of Light and Fertilization on Arbuscular Mycorrhizal Colonization and Growth of Tropical Rain-Forest Syzygium Tree Seedlings, J. Trop. Ecol., 2004, 20, 525–534 CrossRef.
- R. A. Lankau and R. N. Nodurft, An exotic invader drives the evolution of plant traits that determine mycorrhizal fungal diversity in a native competitor, Mol. Ecol., 2013, 22, 5472–5485 CrossRef.
- D. J. Ballhorn, B. S. Younginger and S. Kautz, An aboveground pathogen inhibits belowground rhizobia and arbuscular mycorrhizal fungi in Phaseolus vulgaris, BMC Plant Biol., 2014, 14, 321 CrossRef.
- Z. Zhang, X. Song, X. Lu and Z. Xue, Ecological stoichiometry of carbon, nitrogen, and phosphorus in estuarine wetland soils: influences of vegetation coverage, plant communities, geomorphology, and seawalls, J. Soils Sediments, 2013, 13, 1043–1051 CrossRef CAS.
- J. R. Mayor, N. J. Sanders, A. T. Classen, R. D. Bardgett, J.-C. Clement, A. Fajardo, S. Lavorel, M. K. Sundqvist, M. Bahn and C. Chisholm, Elevation alters ecosystem properties across temperate treelines globally, Nature, 2017, 542, 91–95 CrossRef CAS.
- S. Güsewell, N : P ratios in terrestrial plants: variation and functional significance, New Phytol., 2004, 164, 243–266 CrossRef.
- W. Koerselman and A. F. M. Meuleman, The vegetation N:P ratio : a new tool to detect the nature of nutrient limitation, J. Appl. Ecol., 1996, 33, 1441–1450 CrossRef.
- M. Govindarajulu, P. Pfeffer, H. Jin, J. Abubaker, D. Douds, J. Allen, H. Bucking, P. Lammers and Y. Shachar-Hill, Nitrogen transfer in the arbuscular mycorrhizal symbiosis, Nature, 2005, 435, 819–823 CrossRef CAS.
- F. T. D. Vries, J. W. V. Groenigen, E. Hoffland and J. Bloem, Nitrogen losses from two grassland soils with different fungal biomass, Soil Biol. Biochem., 2011, 43, 997–1005 CrossRef.
- X. X. Wang, X. Wang, Y. Sun, Y. Cheng, S. Liu, X. Chen, G. Feng and T. W. Kuyper, Arbuscular Mycorrhizal Fungi Negatively Affect Nitrogen Acquisition and Grain Yield of Maize in a N Deficient Soil, Front. Microbiol., 2018, 9, 418 CrossRef.
- M. Friede, S. Unger, L. Heuer, R. Stammes and W. Beyschlag, Nitrogen limitation impairs plant control over the arbuscular mycorrhizal symbiosis in response to phosphorus and shading in two European sand dune species, Plant Ecol., 2018, 219, 17–29 CrossRef.
- G. D. Batten and I. F. Wardlaw, Senescence and grain development in wheat plants grown with contrasting phosphorus regimes, Funct. Plant Biol., 1987, 14, 253–265 CrossRef.
- E. C. H. E. T. Lucassen, R. Bobbink, A. J. P. Smolders, P. J. M. V. Der Ven, L. P. M. Lamers and J. G. M. Roelofs, Interactive effects of low pH and high ammonium levels responsible for the decline of Cirsium dissectum (L.) Hill, Plant Ecol., 2003, 165, 45–52 CrossRef.
- S. E. Smith and F. A. Smith, Roles of arbuscular mycorrhizas in plant nutrition and growth: new paradigms from cellular to ecosystem scales, Annu. Rev. Plant Biol., 2011, 62, 227–250 CrossRef CAS.
- M. H. Miller, T. P. Mcgonigle and H. D. Addy, Functional Ecology of Vesicular Arbuscular Mycorrhizas as Influenced by Phosphate Fertilization and Tillage in an Agricultural Ecosystem, Crit. Rev. Biotechnol., 1995, 15, 241–255 CrossRef.
- B. Zhang, S. Li, S. Chen, T. Ren and X. Han, Arbuscular mycorrhizal fungi regulate soil respiration and its response to precipitation change in a semiarid steppe, Sci. Rep., 2016, 6, 19990 CrossRef CAS.
- S. D. Veresoglou, L. J. Shaw, J. E. Hooker and R. Sen, Arbuscular mycorrhizal modulation of diazotrophic and denitrifying microbial communities in the (mycor)rhizosphere of Plantago lanceolata, Soil Biol. Biochem., 2012, 53, 78–81 CrossRef CAS.
- N. C. Johnson, G. W. Wilson, J. A. Wilson, R. M. Miller and M. A. Bowker, Mycorrhizal phenotypes and the Law of the Minimum, New Phytol., 2015, 205, 1473–1484 CrossRef CAS.
- J. A. White and I. Charvat, The mycorrhizal status of an emergent aquatic, Lythrum salicaria L., at different levels of phosphorus availability, Mycorrhiza, 1999, 9, 191–197 CrossRef CAS.
- K. J. Dietz and T. Pfannschmidt, Novel regulators in photosynthetic redox control of plant metabolism and gene expression, Plant Physiol., 2011, 155, 1477–1485 CrossRef CAS.
- F. Battini, M. Grønlund, M. Agnolucci, M. Giovannetti and I. Jakobsen, Facilitation of phosphorus uptake in maize plants by mycorrhizosphere bacteria, Sci. Rep., 2017, 7, 4686 CrossRef.
Footnote |
† Electronic supplementary information (ESI) available. See DOI: 10.1039/d0ra08185j |
|
This journal is © The Royal Society of Chemistry 2020 |
Click here to see how this site uses Cookies. View our privacy policy here.