DOI:
10.1039/D0SC01260B
(Edge Article)
Chem. Sci., 2020,
11, 6521-6526
Late-stage C(sp2)–H and C(sp3)–H glycosylation of C-aryl/alkyl glycopeptides: mechanistic insights and fluorescence labeling†‡
Received
1st March 2020
, Accepted 23rd March 2020
First published on 24th March 2020
Abstract
C(sp3)–H and C(sp2)–H glycosylations of structurally complex amino acids and peptides were accomplished through the assistance of triazole peptide-isosteres. The palladium-catalyzed peptide–saccharide conjugation provided modular access to structurally complex C-alkyl glycoamino acids, glycopeptides and C-aryl glycosides, while enabling the assembly of fluorescent-labeled glycoamino acids. The C–H activation approach represents an expedient and efficient strategy for peptide late-stage diversification in a programmable as well as chemo-, regio-, and diastereo-selective fashion.
Introduction
Glycosylation is a biological function that regulates the structure and activity of a given peptide or protein, introducing considerable structural diversity.1 The attachment of carbohydrates onto peptides can improve their metabolic stability, water solubility, and protect the peptide backbone from proteolytic attack, while playing key structural roles in numerous biological recognition processes.2 As a consequence, glycopeptides have emerged as valuable vaccine candidates and therapeutics.3 However, their chemical and enzymatic instability under physiological conditions limited the utility of O/N-glycopeptides as drugs.4 In contrast, C-glycoside – stabilized isosteres of O/N-glycosides5 – possess improved metabolic stability towards acids, bases and enzymatic hydrolysis,6 thus being promising inhibitors of cell-surface recognition events and regulators of glycoside metabolism (Fig. 1a).7 The targeted modification of biologically active peptides is an important strategy for the elucidation of structure–activity relationships (SAR). During the past decade, the direct C–H manipulation8 of a peptide side-chain mediated by transition metal catalysis has emerged as a powerful paradigm,9 with key contributions from Lavilla/Albericio,10 Chen,11 Daugulis,12 Wang,13 Shi,14 Yu,15 and Ackermann16 among others.17 However, efficient methods for the synthesis of C-alkyl/aryl glycopeptides continue to be rare,18 which contrasts with numerous examples of metal-catalyzed cross-couplings for the construction of C-aryl glycoside with two prefunctionalized substrates.19 Within our program on sustainable C–H activation,20 we herein disclose unprecedented C(sp3)/(sp2)–H glycosylations of amino acids, peptides and (hetero)arenes (Fig. 1b). Notable features of our findings include (1) internal peptide-isosteric click-triazole21 as powerful amide surrogates in various bioactive peptidomimetics, (2) modification of terminal and internal peptides by secondary C(sp3)–H alkenylation with remarkable chemo- and diastereo-selectivities, and (3) detailed mechanistic insights by experiment and computation as well as (4) versatile fluorescence labeling with structurally complex glycopeptides.
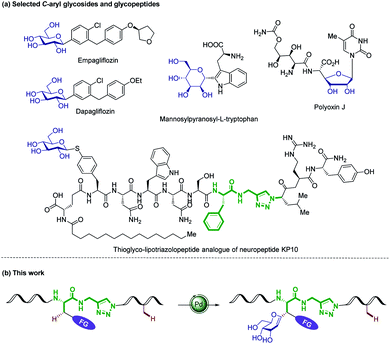 |
| Fig. 1 (a) Selected C-aryl glycosides and glycopeptides. (b) C(sp3)–H glycosylation of labeled amino acids and peptides. | |
Results and discussion
Optimization of reaction conditions
We initiated our studies by exploring reaction conditions for the challenging secondary C(sp3)–H glycosylation of triazolyldimethylmethyl (TAM) amide 1a (Table 1). Preliminary optimization indicated that 60 °C was a less suitable temperature, whilst a slight increase led to the formation of product 3a in 85% yield with Pd(TFA)2 as the catalyst, 1,4-dioxane as the solvent and AgOAc as the additive. When replacing AgOAc by other silver salts (AgTFA, Ag2CO3, and AgBF4), Ag2CO3 stood out, providing glycopeptide 3a in 95% yield (entries 3–5). Notably, further optimization indicated that 1,4-dioxane was the solvent of choice, and DCE, PhMe, or THF provided diminished or trace amounts of the desired product 3a (entries 6–8). When 8-aminoquinoline (AQ) was employed, which was independently utilized by Liu for the synthesis of C-alkyl glycoamino acids,22 the reaction also proceeded efficiently, albeit under slightly modified reaction conditions. Under otherwise identical reaction conditions the use of 2-iodo-glycals provided as of yet unsatisfactory results.
Table 1 Optimization of C(sp3)–H glycosylationa
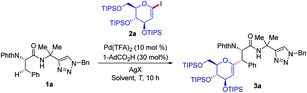
|
Entry |
[Ag] |
Solvents |
T/°C |
Yieldb/% |
Reaction conditions: 1a (0.10 mmol), 2a (0.15 mmol), Pd(TFA)2 (10 mol%), Ag2CO3 (0.20 mmol), 1,4-dioxane (0.5 mL) at 80 °C for 10 h.
Yield of isolated products.
|
1 |
AgOAc |
1,4-Dioxane |
60 |
49 |
2 |
AgOAc |
1,4-Dioxane |
80 |
85 |
3 |
AgTFA |
1,4-Dioxane |
80 |
Trace |
4
|
Ag
2
CO
3
|
1,4-Dioxane
|
80
|
95
|
5 |
AgBF4 |
1,4-Dioxane |
80 |
Trace |
6 |
Ag2CO3 |
DCE |
80 |
45 |
7 |
Ag2CO3 |
PhMe |
80 |
28 |
8 |
Ag2CO3 |
THF |
80 |
<5% |
Substrate scope
With the optimized reaction conditions for the challenging C(sp3)–H glycosylation in hand, we subsequently examined its versatility. Phenylalanine derivatives 1 bearing a variety of functional groups, such as esters or aldehydes, were well tolerated, leading to the formation of products 3a–e with high diasteroselectivities (Scheme 1a). Besides the substrate 2a, the reaction of rhamnose-derived glycal 2b also occurred efficiently. In addition, primary C(sp3)–H bonds of deuterated or non-deuterated Ala-TAM [D3]-1f and 1f were likewise converted, giving product 3g and 3h in 88% and 93% yields respectively, without racemization of the stereogenic centers (see Pages S46–S47 of the ESI‡). The optimized glycosylation proved amenable to substrate 1h with an N-aryl substituent. Similarly, we also proved the reactivity of substrates 1h–j with 8-amino quinoline as a terminal auxiliary. We were pleased to find that glycosylation of primary and secondary C(sp3)–H bonds of alanine, phenylalanine, glutamic acid and even lysine proved to viable. Interestingly, when the reaction temperature was raised to 100 °C, 1,3-diene 3j′ was isolated because of the elimination of the OTIPS group.
 |
| Scheme 1 Scope of glycosylation with TAM and AQ as auxiliaries. (a) 100 °C. | |
When deuterated L-α-aminobutyramide [D3]-1l was employed as the substrate, deuterium-labeled glycopeptide 3p was obtained.
Due to the unique role of C-aryl glycosides as privileged glycomimetics,19a we next probed the TAM-assisted C(sp2)–H glycosylation by palladium catalysis (Scheme 2). Hence, arenes 4a–c with electron-donating or electron-withdrawing substituents were compatible, furnishing the desired products 5a–5c in 92% to 97% yields. It was found that the heteroarenes furan and thiophene were likewise tolerated by the versatile palladium catalyst to deliver conjugate saccharides. Moreover, the fluorescent label pyrene could be successively attached to the glycoside 5f.
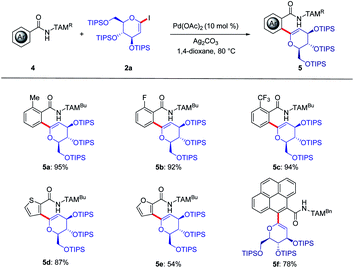 |
| Scheme 2 Scope of C(sp2)–H glycosylation to C-aryl glycosides. | |
Next, we studied the bio-conjugation to form versatile glycopeptides and the hybrids derived thereof (Scheme 3). Various terminal peptides and peptide-natural product hybrids were employed, and late-stage modified peptides 7a–g were obtained by C–H glycosylation. Thereby, the possibilities for accessing structurally complex peptides for drug discovery were showcased.
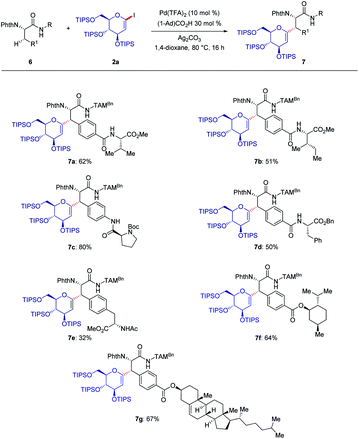 |
| Scheme 3 Scope of glycosylation of terminal peptides and hybrids. | |
Mechanistic studies
As shown in Fig. 2, a minor deuterium kinetic isotope effect (KIE) with deuterated and non-deuterated Ala-TAM [D3]-1f and 1f (kH/kD = 1.0) indicated that the C(sp3)–H bond cleavage is not likely a kinetically relevant step of the palladium catalysis. In order to gain further insights into the mechanism of the palladium-catalyzed C–H glycosylation DFT calculations were hence performed at the ωB97X-D/6-311++G(d,p), SDD(Pd, I, Ag) + SMD(1,4-dioxane)//ωB97X-D/6-31G(d), LANL2DZ(Pd, I, Ag) level of theory.23 The calculated barrier for the initial C–H activation is 19.4 kcal mol−1 (see Fig. S1 of the ESI‡), which is in good agreement with our previous studies.16f Subsequent to the C–H activation, the dissociation of acetic acid and association of substrate 2a lead to the intermediate I1′, which could be further stabilized by Ag2CO3 to afford the stable intermediate I1.24 This process is highly exergonic by 36.8 kcal mol−1, thus making the step irreversible. In I1 as shown in Fig. 3, C–I bond cleavage occurs with the assistance of silver via the transition state TS1–2 to afford the oxidized palladium(IV) intermediate I2, with a barrier of 19.3 kcal mol−1 with respect to I1. In this transition state, it is possible to observe attractive dispersive interactions between the imide of the substrate and Ag2CO3, which subsequently becomes evident by the bond distances between both moieties as shown in Fig. 4. This could be further confirmed by visualizing the NCI (non-covalent interactions) plot. The reaction continues with the reductive elimination via the transition state TS2–3 with a barrier of 18.8 kcal mol−1 to finalize the C–C formation process, followed by subsequent protonation to release the desired product.
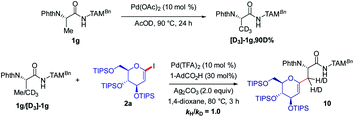 |
| Fig. 2 H/D exchange and KIE experiments. | |
 |
| Fig. 3 Calculated Gibbs free energy profiles for the oxidative addition and reductive elimination steps in kcal mol−1 at the ωB97X-D/6-311++G(d,p), SDD(Pd, I, Ag) + SMD(1,4-dioxane)//ωB97X-D/6-31G(d), LANL2DZ(Pd, I, Ag) level of theory. | |
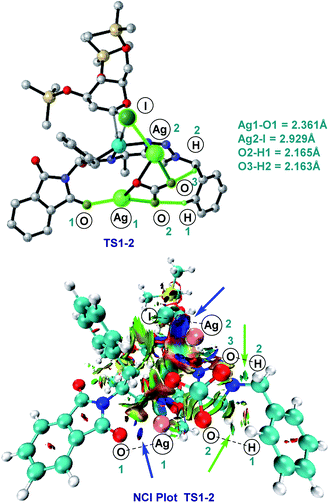 |
| Fig. 4 The 3D structures and the non-covalent interactions visualized through NCI-plots of the transition state TS1–2 (strong and weak attractive interactions are given in blue and green, respectively, while red corresponds to strong repulsive interactions). | |
In order to illustrate the robustness of our C(sp3)–H activation, we explored the unprecedented late-stage glycosylation of internal peptides (Scheme 4). With the sterically congested gem-disubstituted substrates 8a–8e various peptides 9a–9e were converted into value-added glycopeptides under exceedingly mild reaction conditions. When unprotected peptide Phth-Ala-Tzl-Tyr-OMe was employed, only trace amounts of product 9f were observed. Here, the peptidomimetic Tzl scaffold set the stage for expedient site-selective peptide late-stage glycosylations.
 |
| Scheme 4 Scope of glycosylation of terminal peptides. | |
Intrigued by the unique potential of BODIPYs as bio-compatible fluorescent probes,16e,25 glycosylation of different BODIPY labeled amino acids was explored for our C(sp3)–H activation process (Scheme 5). Hence, the C–H activation enabled the unprecedented preparation of BODIPY labeled glycoamino acids (11a–11e).
 |
| Scheme 5 Scope of BODIPY labeled glycoamino acids. | |
Conclusion
In summary, we have reported a versatile C(sp3)–H/C(sp2)–H glycosylation strategy enabled by peptide isosteric click-triazoles. Thus, the de novo synthesis of structurally complex C-alkyl glycoamino acids, glycopeptides and C-aryl glycosides was achieved by palladium-catalyzed C(sp3)–H/C(sp2)–H activation with excellent levels of regio-, chemo- and diastereoselectivities. The synthetic utility of our strategy was reflected inter alia by the assembly of BODIPY fluorescent labeled glycoamino acids and the racemization-free late-stage diversification of structurally complex molecules. Our approach holds major potential for the preparation of C-alkyl and C-aryl glycosyl amino acid building blocks for their subsequent use in glycopeptide assembly and molecular labeling.
Conflicts of interest
There are no conflicts to declare.
Acknowledgements
Generous support by the DFG (Gottfried-Wilhelm-Leibniz award to L. A. and SPP1807), the CSC (fellowship to J. W.) and the Onassis Foundation (fellowship to N. K.) is gratefully acknowledged.
Notes and references
-
(a) J. N. deGruyter, L. R. Malins and P. S. Baran, Biochemistry, 2017, 56, 3863–3873 CrossRef CAS PubMed;
(b) L. Krasnova and C.-H. Wong, Annu. Rev. Biochem., 2016, 85, 599–630 CrossRef CAS PubMed;
(c) S. J. Danishefsky and J. R. Allen, Angew. Chem., Int. Ed., 2000, 39, 836–863 CrossRef CAS.
-
(a) B. Lepenies and P. H. Seeberger, Nat. Biotechnol., 2014, 32, 443–445 CrossRef CAS PubMed;
(b) O. Werdelin, M. Meldal and T. Jensen, Proc. Natl. Acad. Sci. U. S. A., 2002, 99, 9611–9613 CrossRef CAS PubMed;
(c) A. Varki, Glycobiology, 1993, 3, 97–130 CrossRef CAS PubMed;
(d) P. Sears and C. H. Wong, Cell. Mol. Life Sci., 1998, 54, 223–252 CrossRef CAS PubMed;
(e) C.-H. Wong, J. Org. Chem., 2005, 70, 4219–4225 CrossRef CAS PubMed.
- M.-L. Hecht, P. Stallforth, D. V. Silva, A. Adibekian and P. H. Seeberger, Curr. Opin. Chem. Biol., 2009, 13, 354–359 CrossRef CAS PubMed.
-
(a) P. H. Seeberger and D. B. Werz, Nature, 2007, 446, 1046–1051 CrossRef CAS PubMed;
(b) D. C. Koester, A. Holkenbrink and D. B. Werz, Synthesis, 2010, 2010, 3217–3242 CrossRef.
-
(a) R. J. Payne and C.-H. Wong, Chem. Commun., 2010, 46, 21–43 RSC;
(b) M. J. McKay and H. M. Nguyen, ACS Catal., 2012, 2, 1563–1595 CrossRef CAS PubMed;
(c) M. A. Bonache, F. Nuti, A. Le Chevalier Isaad, F. Real-Fernández, M. Chelli, P. Rovero and A. M. Papini, Tetrahedron Lett., 2009, 50, 4151–4153 CrossRef CAS;
(d) M. R. Pratt and C. R. Bertozzi, Chem. Soc. Rev., 2005, 34, 58–68 RSC;
(e) Z. Guo and N. Shao, Med. Res. Rev., 2005, 25, 655–678 CrossRef CAS PubMed;
(f) B. Wang, Y. Liu, R. Jiao, Y. Feng, Q. Li, C. Chen, L. Liu, G. He and G. Chen, J. Am. Chem. Soc., 2016, 138, 3926–3932 CrossRef CAS PubMed;
(g) H. Herzner, T. Reipen, M. Schultz and H. Kunz, Chem. Rev., 2000, 100, 4495–4538 CrossRef CAS PubMed;
(h) J. Rodriguez, S. O'Neill and M. A. Walczak, Nat. Prod. Rep., 2018, 35, 220–229 RSC;
(i) D. P. Gamblin, E. M. Scanlan and B. G. Davis, Chem. Rev., 2009, 109, 131–163 CrossRef CAS PubMed;
(j) Y. Dai, B. Tian, H. Chen and Q. Zhang, ACS Catal., 2019, 9, 2909–2915 CrossRef CAS.
- A. Dondoni and A. Marra, Chem. Rev., 2000, 100, 4395–4422 CrossRef CAS PubMed.
-
(a) J. W. Dennis, I. R. Nabi and M. Demetriou, Cell, 2009, 139, 1229–1241 CrossRef PubMed;
(b) C. R. Bertozzi and L. L. Kiessling, Science, 2001, 291, 2357–2364 CrossRef CAS PubMed;
(c) B. Imperiali, Acc. Chem. Res., 1997, 30, 452–459 CrossRef CAS;
(d) D. Montoir, M. Amoura, Z. E. A. Ababsa, T. M. Vishwanatha, E. Yen-Pon, V. Robert, M. Beltramo, V. Piller, M. Alami, V. Aucagne and S. Messaoudi, Chem. Sci., 2018, 9, 8753–8759 RSC.
-
(a) S. Rej, Y. Ano and N. Chatani, Chem. Rev., 2020, 120, 1788–1887 CrossRef CAS PubMed;
(b) L. Ackermann, Acc. Chem. Res., 2020, 53, 84–104 CrossRef CAS PubMed;
(c) J. He, M. Wasa, K. S. L. Chan, Q. Shao and J.-Q. Yu, Chem. Rev., 2017, 117, 8754–8786 CrossRef CAS PubMed;
(d) Z. Dong, Z. Ren, S. J. Thompson, Y. Xu and G. Dong, Chem. Rev., 2017, 117, 9333–9403 CrossRef CAS PubMed;
(e) B. Ye and N. Cramer, Acc. Chem. Res., 2015, 48, 1308–1318 CrossRef CAS PubMed;
(f) G. Rouquet and N. Chatani, Angew. Chem., Int. Ed., 2013, 52, 11726–11743 CrossRef CAS PubMed;
(g) L. McMurray, F. O'Hara and M. J. Gaunt, Chem. Soc. Rev., 2011, 40, 1885–1898 RSC;
(h) C. S. Yeung and V. M. Dong, Chem. Rev., 2011, 111, 1215–1292 CrossRef CAS PubMed;
(i) O. Daugulis, H.-Q. Do and D. Shabashov, Acc. Chem. Res., 2009, 42, 1074–1086 CrossRef CAS PubMed;
(j) L. Ackermann, R. Vicente and A. R. Kapdi, Angew. Chem., Int. Ed., 2009, 48, 9792–9826 CrossRef CAS PubMed.
-
(a) W. Wang, M. M. Lorion, J. Shah, A. R. Kapdi and L. Ackermann, Angew. Chem., Int. Ed., 2018, 57, 14700–14717 CrossRef CAS PubMed;
(b) M. Jbara, S. K. Maity and A. Brik, Angew. Chem., Int. Ed., 2017, 56, 10644–10655 CrossRef CAS PubMed;
(c) A. F. M. Noisier and M. A. Brimble, Chem. Rev., 2014, 114, 8775–8806 CrossRef CAS PubMed.
-
(a) A. F. M. Noisier, J. García, I. A. Ionuţ and F. Albericio, Angew. Chem., Int. Ed., 2017, 56, 314–318 CrossRef CAS PubMed;
(b) R. Subiros-Funosas, L. Mendive-Tapia, J. Sot, J. D. Pound, N. Barth, Y. Varela, F. M. Goñi, M. Paterson, C. D. Gregory, F. Albericio, I. Dransfield, R. Lavilla and M. Vendrell, Chem. Commun., 2017, 53, 945–948 RSC;
(c) L. Mendive-Tapia, S. Preciado, J. García, R. Ramón, N. Kielland, F. Albericio and R. Lavilla, Nat. Commun., 2015, 6, 7160 CrossRef PubMed;
(d) S. Preciado, L. Mendive-Tapia, F. Albericio and R. Lavilla, J. Org. Chem., 2013, 78, 8129–8135 CrossRef CAS PubMed;
(e) J. Ruiz-Rodríguez, F. Albericio and R. Lavilla, Chem.–Eur. J., 2010, 16, 1124–1127 CrossRef PubMed.
-
(a) B. Li, X. Li, B. Han, Z. Chen, X. Zhang, G. He and G. Chen, J. Am. Chem. Soc., 2019, 141, 9401–9407 CrossRef PubMed;
(b) X. Chen, F. Ye, X. Luo, X. Liu, J. Zhao, S. Wang, Q. Zhou, G. Chen and P. Wang, J. Am. Chem. Soc., 2019, 141, 18230–18237 CrossRef CAS PubMed;
(c) X. Zhang, G. Lu, M. Sun, M. Mahankali, Y. Ma, M. Zhang, W. Hua, Y. Hu, Q. Wang, J. Chen, G. He, X. Qi, W. Shen, P. Liu and G. Chen, Nat. Chem., 2018, 10, 540–548 CrossRef CAS PubMed;
(d) B. Wang, X. Wu, R. Jiao, S. Y. Zhang, W. A. Nack, G. He and G. Chen, Org. Chem. Front., 2015, 2, 1318–1321 RSC;
(e) B. Wang, W. A. Nack, G. He, S.-Y. Zhang and G. Chen, Chem. Sci., 2014, 5, 3952–3957 RSC;
(f) B. Wang, C. Lu, S.-Y. Zhang, G. He, W. A. Nack and G. Chen, Org. Lett., 2014, 16, 6260–6263 CrossRef CAS PubMed;
(g) G. He, S.-Y. Zhang, W. A. Nack, R. Pearson, J. Rabb-Lynch and G. Chen, Org. Lett., 2014, 16, 6488–6491 CrossRef CAS PubMed;
(h) S.-Y. Zhang, Q. Li, G. He, W. A. Nack and G. Chen, J. Am. Chem. Soc., 2013, 135, 12135–12141 CrossRef CAS PubMed;
(i) G. He and G. Chen, Angew. Chem., Int. Ed., 2011, 50, 5192–5196 CrossRef CAS PubMed.
- L. D. Tran and O. Daugulis, Angew. Chem., Int. Ed., 2012, 51, 5188–5191 CrossRef CAS PubMed.
-
(a) J. Tan, J. Wu, S. Liu, H. Yao and H. Wang, Sci. Adv., 2019, 5, eaaw0323 CrossRef CAS PubMed;
(b) Q. Luo, Y. Tao, W. Sheng, J. Lu and H. Wang, Nat. Commun., 2019, 10, 142 CrossRef PubMed;
(c) J. Lu, J. Li, Y. Wu, X. Fang, J. Zhu and H. Wang, Org. Lett., 2019, 21, 4676–4679 CrossRef CAS PubMed;
(d) S. Chen, B. Xu, E. Chen, J. Wang, J. Lu, S. Donadio, H. Ge and H. Wang, Proc. Natl. Acad. Sci. U. S. A., 2019, 116, 2533–2538 CrossRef CAS PubMed;
(e) J. Tang, H. Chen, Y. He, W. Sheng, Q. Bai and H. Wang, Nat. Commun., 2018, 9, 3383 CrossRef PubMed;
(f) Z. Bai, C. Cai, Z. Yu and H. Wang, Angew. Chem., Int. Ed., 2018, 57, 13912–13916 CrossRef CAS PubMed;
(g) J. Tang, Y. He, H. Chen, W. Sheng and H. Wang, Chem. Sci., 2017, 8, 4565–4570 RSC.
-
(a) L. Liu, Y.-H. Liu and B.-F. Shi, Chem. Sci., 2020, 11, 290–294 RSC;
(b) B.-B. Zhan, J. Fan, L. Jin and B.-F. Shi, ACS Catal., 2019, 9, 3298–3303 CrossRef CAS;
(c) B.-B. Zhan, Y. Li, J.-W. Xu, X.-L. Nie, J. Fan, L. Jin and B.-F. Shi, Angew. Chem., Int. Ed., 2018, 57, 5858–5862 CrossRef CAS PubMed;
(d) S. Q. Zhang, H. Z. Jiang, J. W. Xu and B. F. Shi, Chemistry, 2015, 21, 3264–3270 CrossRef PubMed;
(e) Q. Zhang, X. S. Yin, K. Chen, S. Q. Zhang and B. F. Shi, J. Am. Chem. Soc., 2015, 137, 8219–8226 CrossRef CAS PubMed;
(f) K. Chen, S. Q. Zhang, J. W. Xu, F. Hu and B. F. Shi, Chem. Commun., 2014, 50, 13924–13927 RSC;
(g) K. Chen and B. F. Shi, Angew. Chem., Int. Ed., 2014, 53, 11950–11954 CrossRef CAS PubMed;
(h) Q. Zhang, K. Chen, W. Rao, Y. Zhang, F. J. Chen and B. F. Shi, Angew. Chem., Int. Ed., 2013, 52, 13588–13592 CrossRef CAS PubMed;
(i) K. Chen, F. Hu, S.-Q. Zhang and B.-F. Shi, Chem. Sci., 2013, 4, 3906 RSC.
-
(a) T. Liu, J. X. Qiao, M. A. Poss and J.-Q. Yu, Angew. Chem., Int. Ed., 2017, 56, 10924–10927 CrossRef CAS PubMed;
(b) G. Chen, T. Shigenari, P. Jain, Z. Zhang, Z. Jin, J. He, S. Li, C. Mapelli, M. M. Miller, M. A. Poss, P. M. Scola, K.-S. Yeung and J.-Q. Yu, J. Am. Chem. Soc., 2015, 137, 3338–3351 CrossRef CAS PubMed;
(c) J. He, S. Li, Y. Deng, H. Fu, B. N. Laforteza, J. E. Spangler, A. Homs and J.-Q. Yu, Science, 2014, 343, 1216–1220 CrossRef CAS PubMed;
(d) W. Gong, G. Zhang, T. Liu, R. Giri and J.-Q. Yu, J. Am. Chem. Soc., 2014, 136, 16940–16946 CrossRef CAS PubMed;
(e) L. Chu, K.-J. Xiao and J.-Q. Yu, Science, 2014, 346, 451–455 CrossRef CAS PubMed.
-
(a) A. Schischko, N. Kaplaneris, T. Rogge, G. Sirvinskaite, J. Son and L. Ackermann, Nat. Commun., 2019, 10, 3553 CrossRef PubMed;
(b) M. M. Lorion, N. Kaplaneris, J. Son, R. Kuniyil and L. Ackermann, Angew. Chem., Int. Ed., 2019, 58, 1684–1688 CrossRef CAS PubMed;
(c) N. Kaplaneris, T. Rogge, R. Yin, H. Wang, G. Sirvinskaite and L. Ackermann, Angew. Chem., Int. Ed., 2019, 58, 3476–3480 CrossRef CAS PubMed;
(d) W. Wang, M. M. Lorion, O. Martinazzoli and L. Ackermann, Angew. Chem., Int. Ed., 2018, 57, 10554–10558 CrossRef CAS PubMed;
(e) A. Schischko, H. Ren, N. Kaplaneris and L. Ackermann, Angew. Chem., Int. Ed., 2017, 56, 1576–1580 CrossRef CAS PubMed;
(f) Y. Zhu, M. Bauer and L. Ackermann, Chem.–Eur. J., 2015, 21, 9980–9983 CrossRef CAS PubMed;
(g) Y. Zhu, M. Bauer, J. Ploog and L. Ackermann, Chem.–Eur. J., 2014, 20, 13099–13102 CrossRef CAS PubMed.
-
(a) X. Wang, S. Niu, L. Xu, C. Zhang, L. Meng, X. Zhang and D. Ma, Org. Lett., 2017, 19, 246–249 CrossRef CAS PubMed;
(b) A. J. Reay, L. A. Hammarback, J. T. W. Bray, T. Sheridan, D. Turnbull, A. C. Whitwood and I. J. S. Fairlamb, ACS Catal., 2017, 7, 5174–5179 CrossRef CAS PubMed;
(c) T. J. Osberger, D. C. Rogness, J. T. Kohrt, A. F. Stepan and M. C. White, Nature, 2016, 537, 214–219 CrossRef CAS PubMed;
(d) B. Mondal, B. Roy and U. Kazmaier, J. Org. Chem., 2016, 81, 11646–11655 CrossRef CAS PubMed;
(e) B. V. S. Reddy, L. R. Reddy and E. J. Corey, Org. Lett., 2006, 8, 3391–3394 CrossRef CAS PubMed.
-
(a) Q. Wang, S. An, Z. Deng, W. Zhu, Z. Huang, G. He and G. Chen, Nat. Catal., 2019, 2, 793–800 CrossRef CAS;
(b) N. Probst, G. Grelier, S. Dahaoui, M. Alami, V. Gandon and S. Messaoudi, ACS Catal., 2018, 8, 7781–7786 CrossRef CAS;
(c) M. Liu, Y. Niu, Y.-F. Wu and X.-S. Ye, Org. Lett., 2016, 18, 1836–1839 CrossRef CAS PubMed.
-
(a) Y. Yang and B. Yu, Chem. Rev., 2017, 117, 12281–12356 CrossRef CAS PubMed;
(b) X. Li and J. Zhu, Eur. J. Org. Chem., 2016, 2016, 4724–4767 CrossRef CAS;
(c) Y. Dai, B. Tian, H. Chen and Q. Zhang, ACS Catal., 2019, 9, 2909–2915 CrossRef CAS;
(d) J. Liu and H. Gong, Org. Lett., 2018, 20, 7991–7995 CrossRef CAS PubMed;
(e) F. Zhu, J. Rodriguez, S. O'Neill and M. A. Walczak, ACS Cent. Sci., 2018, 4, 1652–1662 CrossRef CAS PubMed;
(f) F. Zhu, J. Rodriguez, T. Yang, I. Kevlishvili, E. Miller, D. Yi, S. O'Neill, M. J. Rourke, P. Liu and M. A. Walczak, J. Am. Chem. Soc., 2017, 139, 17908–17922 CrossRef CAS PubMed;
(g) L. Adak, S. Kawamura, G. Toma, T. Takenaka, K. Isozaki, H. Takaya, A. Orita, H. C. Li, T. K. M. Shing and M. Nakamura, J. Am. Chem. Soc., 2017, 139, 10693–10701 CrossRef CAS PubMed;
(h) F. Zhu, M. J. Rourke, T. Yang, J. Rodriguez and M. A. Walczak, J. Am. Chem. Soc., 2016, 138, 12049–12052 CrossRef CAS PubMed;
(i) J. Zeng, J. Ma, S. Xiang, S. Cai and X.-W. Liu, Angew. Chem., Int. Ed., 2013, 52, 5134–5137 CrossRef CAS PubMed;
(j) L. Nicolas, P. Angibaud, I. Stansfield, P. Bonnet, L. Meerpoel, S. Reymond and J. Cossy, Angew. Chem., Int. Ed., 2012, 51, 11101–11104 CrossRef CAS PubMed;
(k) H. Gong and M. R. Gagné, J. Am. Chem. Soc., 2008, 130, 12177–12183 CrossRef CAS PubMed.
-
(a) P. Gandeepan, T. Müller, D. Zell, G. Cera, S. Warratz and L. Ackermann, Chem. Rev., 2019, 119, 2192–2452 CrossRef CAS PubMed;
(b) L. Ackermann, Acc. Chem. Res., 2014, 47, 281–295 CrossRef CAS PubMed;
(c) L. Ackermann, Chem. Rev., 2011, 111, 1315–1345 CrossRef CAS PubMed.
-
(a) H. C. Kolb and K. B. Sharpless, Drug Discovery Today, 2003, 8, 1128–1137 CrossRef CAS;
(b) C. W. Tornøe, C. Christensen and M. Meldal, J. Org. Chem., 2002, 67, 3057–3064 CrossRef PubMed;
(c) H. C. Kolb, M. G. Finn and K. B. Sharpless, Angew. Chem., Int. Ed., 2001, 40, 2004–2021 CrossRef CAS.
- During the preparation of our manuscript, Liu independently reported on a modification of simple amino acids with 8-aminoquinoline assistance:
(a) Y. Liu, Y. Wang, W. Dai, W. Huang, Y. Li and H. Liu, Angew. Chem., Int. Ed., 2020, 59, 3491–3494 CrossRef CAS PubMed;
(b) Q. Wang, S. An, Z. Deng, W. Zhu, Z. Huang, G. He and G. Chen, Nat. Catal., 2019, 2, 793–800 CrossRef CAS;
(c) For previous manganese catalysis, see: W. Wang, P. Subramanian, O. Martinazzoli, J. Wu and L. Ackermann, Chem.–Eur. J., 2019, 25, 10585–10589 CrossRef CAS PubMed.
- For detailed information, see the ESI.‡.
- For previous reports on the stabilization of intermediates by complexation of silver, see:
(a) S. Guin, P. Dolui, X. Zhang, S. Paul, V. K. Singh, S. Pradhan, H. B. Chandrashekar, S. S. Anjana, R. S. Paton and D. Maiti, Angew. Chem., Int. Ed., 2019, 58, 5633–5638 CrossRef CAS PubMed;
(b) I. Funes-Ardoiz and F. Maseras, Chem.–Eur. J., 2018, 24, 12383–12388 CrossRef CAS PubMed;
(c) Y.-F. Yang, G. Chen, X. Hong, J.-Q. Yu and K. N. Houk, J. Am. Chem. Soc., 2017, 139, 8514–8521 CrossRef CAS PubMed;
(d) P. Xiao, H. Yuan, J. Liu, Y. Zheng, X. Bi and J. Zhang, ACS Catal., 2015, 5, 6177–6184 CrossRef CAS;
(e) Y.-F. Yang, G.-J. Cheng, P. Liu, D. Leow, T.-Y. Sun, P. Chen, X. Zhang, J.-Q. Yu, Y.-D. Wu and K. N. Houk, J. Am. Chem. Soc., 2014, 136, 344–355 CrossRef CAS PubMed.
- For representative references, see:
(a) M. Virelli, W. Wang, R. Kuniyil, J. Wu, G. Zanoni, A. Fernandez, J. Scott, M. Vendrell and L. Ackermann, Chem.–Eur. J., 2019, 25, 12712–12718 CrossRef CAS PubMed;
(b) M. Bauer, W. Wang, M. M. Lorion, C. Dong and L. Ackermann, Angew. Chem., Int. Ed., 2018, 57, 203–207 CrossRef CAS PubMed;
(c) L. Mendive-Tapia, R. Subiros-Funosas, C. Zhao, F. Albericio, N. D. Read, R. Lavilla and M. Vendrell, Nat. Protoc., 2017, 12, 1588–1619 CrossRef CAS PubMed;
(d) A. Fernandez, M. Vermeren, D. Humphries, R. Subiros-Funosas, N. Barth, L. Campana, A. MacKinnon, Y. Feng and M. Vendrell, ACS Cent. Sci., 2017, 3, 995–1005 CrossRef CAS PubMed;
(e) L. Mendive-Tapia, C. Zhao, A. R. Akram, S. Preciado, F. Albericio, M. Lee, A. Serrels, N. Kielland, N. D. Read, R. Lavilla and M. Vendrell, Nat. Commun., 2016, 7, 10940 CrossRef CAS PubMed;
(f) A. Vázquez-Romero, N. Kielland, M. J. Arévalo, S. Preciado, R. J. Mellanby, Y. Feng, R. Lavilla and M. Vendrell, J. Am. Chem. Soc., 2013, 135, 16018–16021 CrossRef PubMed , and cited references.
Footnotes |
† Dedicated to the memory of Prof. Kilian Muñiz. |
‡ Electronic supplementary information (ESI) available. See DOI: 10.1039/d0sc01260b |
§ These authors contributed equally. |
|
This journal is © The Royal Society of Chemistry 2020 |
Click here to see how this site uses Cookies. View our privacy policy here.