DOI:
10.1039/D0SC01278E
(Edge Article)
Chem. Sci., 2020,
11, 7615-7618
SET processes in Lewis acid–base reactions: the tritylation of N-heterocyclic carbenes†
Received
2nd March 2020
, Accepted 3rd April 2020
First published on 9th April 2020
Abstract
Reactions between N-heterocyclic carbenes (NHCs) and the trityl cation, [Ph3C]+, give covalent adducts of type [NHC–CPh3]+ and/or [NHC–C6H5–CPh2]+. EPR spectroscopy, UV-Vis analyses, and trapping experiments imply that adduct formation involves carbene radical cations and the trityl radical. The results demonstrate that single electron transfer (SET) processes should be considered for reaction of NHCs with oxidizing Lewis acids.
Introduction
The classification of electron-pair donors as bases and electron-pair acceptors as acids was proposed by G. N. Lewis in 1923.1 The concept gained influence in the 1940s, and today it is widely used as a systemizing tool in chemical teaching and research.2 Reactions between Lewis acids (LAs) and bases (LBs) are typically described as two-electron processes (Scheme 1a). However, the possible involvement of single electron transfer (SET) processes in reactions of this kind has been discussed early on.3 Experimental evidence for the participation of radicals was obtained for frustrated Lewis pairs (Scheme 1b).4–8 Stephan and co-workers have reported that mixtures of PMes3 and E(C6F5)3 (E = B, Al) are in equilibrium with the frustrated radical pair [Mes3P]˙+ and [E(C6F5)3]˙−.6 The former was detected by EPR spectroscopy, whereas the latter was evidenced by trapping experiments. In a follow-up study, they were able to show that the PMes3/B(C6F5)3 pair is able to cleave the O–O bond of benzoyl peroxide in a homolytic fashion.7 These results corroborate the radical reactivity of this FLP. Recently, the group of Müller described classic Lewis pair systems, for which SET pathways are also of relevance (Scheme 1c). A mixture of a phosphine and a solvent-stabilized trialkylsilylium ion was found to give radicals, even though silylphosphonium adducts can form.9 Furthermore, they were able to show that the reaction between a germylene Lewis base and B(C6F5)3 is initiated by a SET process.10 The intermediates germylene radical cation and [B(C6F5)3]˙− could be detected by EPR spectroscopy.
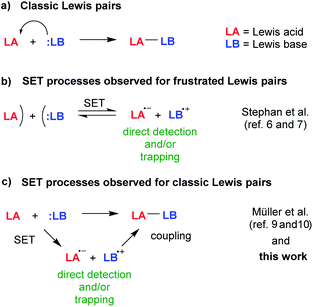 |
| Scheme 1 The formation of classic Lewis pairs via a 2-electron process (a), and SET processes observed for frustrated (b) and classic (c) Lewis pairs. | |
Below, we provide experimental evidence for the relevance of SET processes in reactions of NHCs with the Lewis acid [Ph3C][B(C6F5)4]. In particular, we show that adduct formation involves the formation of a carbene radical cation and the trityl radical.
Results and discussion
N-heterocyclic carbenes are not only important ligands in transition metal chemistry,11 but they are also used extensively as Lewis bases in main group chemistry.12 For the present study, we have investigated the reaction of NHCs with the Lewis acid [Ph3C][B(C6F5)4]. When 1,3-bis(2,6-diisopropylphenyl)imidazol-2-ylidene (IDipp, 1a) was allowed to react with the trityl salt in toluene at −40 °C, we observed the clean formation of adduct 2a (Scheme 2). The structure of 2a was established by NMR spectroscopy and by single crystal X-ray diffraction (XRD) analysis (see the ESI†). The formation of 2a was expected, since a structurally related compound was reported by the group of Stephan for the reaction of 1,3-di-tert-butylimidazol-2-ylidene (ItBu, 1b) and [Ph3C][B(C6F5)4].13
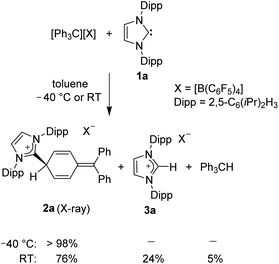 |
| Scheme 2 Reaction of IDipp (1a) with [Ph3C][B(C6F5)4] in toluene. | |
When the reaction between 1a and the trityl salt was performed in toluene at room temperature, side products were formed, as revealed by NMR spectroscopic analysis of the reaction mixture. Adduct 2a was still the main product (76%), but the imidazolium salt [IDipp-H][B(C6F5)4] (3a) was formed in 24% yield, along with a small amount of triphenylmethane, Ph3CH (∼5%).
During our investigations, we were intrigued by the following observation: when 1a was added to a solution of the trityl salt (either RT or −40 °C), the color of the solution turned from yellow to purple. The purple color quickly faded away, and at the end of the reaction, a nearly colorless solution was observed (Fig. 1a).
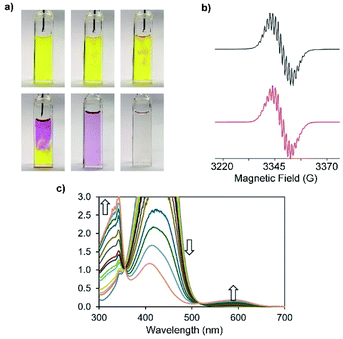 |
| Fig. 1 (a) Color of a solution of [Ph3C][B(C6F5)4] in chlorobenzene14 upon dropwise addition of a solution of IDipp; (b) experimental (black lines) and simulated (red lines) EPR spectra of the trityl radical in chlorobenzene (g = 2.0025, A(o-H) = 2.6 G, A(m-H) = 1.1 G, A(p-H) = 2.8 G),15 and (c) evolution of the UV-vis spectra of a chlorobenzene solution containing 1a and [Ph3C][B(C6F5)4]. | |
When the beginning of the reaction was followed by UV-vis spectroscopy at room temperature, one could observe a gradual decrease of the band at 438 nm, which can be attributed to the trityl cation. Simultaneously, a band 343 nm and a weaker band at 591 nm started to grow (Fig. 1c). The latter disappeared with time, and after 1 min, no significant absorption at wavelengths higher than 500 nm could be observed. This finding is in line with the fact that solutions of the products are either colorless or weakly yellow. When the pink solution was exposed to air, the bands at 343 and 591 nm disappeared nearly instantaneously (ESI, Fig. S8†).
The results imply that adduct formation proceeds via one (or more) short-lived intermediate(s). In order to examine if radicals are involved, we investigated mixtures of 1a and [Ph3C][B(C6F5)4] by EPR spectroscopy. We were able to detect a signal with a complex hyperfine coupling, which can be attributed to the trityl radical (Fig. 1b).16 The formation of [Ph3C]˙ provides an explanation for the absorption at 343 nm, because the trityl radical is known to have a band in that region.17 We propose that the purple color (broad band at 591 nm), which we observed at the beginning of the reaction, is due to [1a]˙+. This assumption is substantiated by the following control experiment: when IDipp was deliberately oxidized with [NO][SbF6] in toluene at room temperature, a short-lived purple color was also observed (for details, see ESI†). The main product of this reaction was the imidazolium salt [IDipp-H][SbF6], which is presumably formed by reaction of the intermediate [1a]˙+ with the solvent (see Fig. S14†). Taken together, the experimental data suggest that the reaction between 1a and [Ph3C][B(C6F5)4] proceeds via single electron transfer to give [Ph3C]˙ and [1a]˙+ (Scheme 3).18 At −40 °C, selective cross-coupling results in formation of adduct 2a, whereas hydrogen atoms abstraction becomes a competing pathway for reactions performed at room temperature. The carbene radical cation [1a]˙+ is more reactive than the persistent [Ph3C]˙,19 which provides an explanation that we were not able to detect the former by EPR spectroscopy.
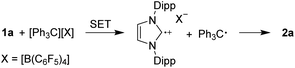 |
| Scheme 3 Formation of the Lewis acid–base adduct 2a proceeds via the radicals [Ph3C]˙ and [1a]˙+. | |
It is worth noting that NHC-based radical cations have been suggested as intermediates of reactions between NHCs and oxidants such as tetracyanoethylene,20 ferrocenium salts,20 and PhI(OTf)2.21 The products of these reactions are either imidazolium salts (formed via H atom abstraction from the solvent) or dicationic dimers (formed by homo-coupling of the NHC radical cations). In our case, cross-coupling of [Ph3C]˙ and [1a]˙+ is strongly favored over homo-coupling reactions. We have analyzed reaction mixtures by mass spectroscopy, and we were able to detect peaks for the coupling adduct 2a and the imidazolium salt 3a, but not for a dicationic dimer [(1a)2]2+.
Further evidence for the formation of radicals was obtained by trapping experiments with the hydrogen atom donors THF or Ph3SnH (Table 1). When the reaction between 1a and the trityl salt was performed in the presence of 25 vol% THF, adduct formation was impeded, and the imidazolium salt 3a was formed in 73% yield, along with equimolar amounts of Ph3CH. Similar results were observed for reactions with Ph3SnH. When a solution of Ph3SnH in toluene was added to an equimolar mixture of 1a and [Ph3C][B(C6F5)4], the dominant imidazolium-based product was 3a (42%). Furthermore, we were able to detect Ph3SnSnPh3 by 119Sn NMR spectroscopy (δ119Sn = 131.1, ESI, Fig. S16b†). The formation of the distannane is further evidence for the involvement of radicals.6,22 We noted that the starting materials (1a and the trityl salt) do also react with Ph3SnH. However, these reactions gave alternative products, and Ph3SnSnPh3 was not observed.
Table 1 Reactions of NHCs 1 with [Ph3C][B(C6F5)4] in the presence of the radical scavengers THF and Ph3SnH
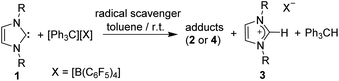
|
|
Radical scavenger |
Yield of 3a |
Yields were determined by 1H NMR spectroscopy.
|
1a (R = Dipp) |
THF (25 vol%) |
73% |
1a (R = Dipp) |
Ph3SnH (2 equiv.) |
42% |
1b (R = tBu) |
THF (25 vol%) |
79% |
1b (R = tBu) |
Ph3SnH (2 equiv.) |
87% |
1c (R = Mes) |
THF (25 vol%) |
63% |
Next, we re-examined the addition of ItBu (1b) to [Ph3C][B(C6F5)4]. When the reaction was performed in toluene at −40 °C, the known coupling product 2b13 was obtained in nearly quantitative yield. In contrast, only a small amount of 2b was formed when the reaction was carried out at room temperature in the presence of 25 vol% THF (Table 1 and ESI†). Under these conditions, the main products were the imidazolium salt [ItBu-H][B(C6F5)4] (3b, characterized also by X-ray crystallography) and Ph3CH (both 79%). Using Ph3SnH as radical scavenger, the yield of the imidazolium salt was even higher (87%). As in the case of reactions with IDipp, the dimer Ph3SnSnPh3 was detected by 119Sn NMR spectroscopy.
Similar results were obtained for reactions of IMes (1c) with [Ph3C][B(C6F5)4] in the presence of the radical scavenger THF: the main products were the imidazolium salt 3c and Ph3CH (Table 1), indicating an initial SET process between the carbene and the trityl salt.23 However, reactions performed in toluene at −40 °C gave a surprising result. The expected adduct 2c was only formed in 18% yield. The main product (71%) was the isomeric coupling product 4, with a C–C bond to the central carbon atom of the trityl group (Scheme 4). The formation of 4 can be explained by the reduced steric demand of the mesityl wingtip groups.24
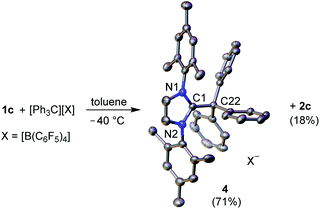 |
| Scheme 4 Reaction of IMes (1c) with [Ph3C][B(C6F5)4] in toluene. The graphic representation of the cation of 4 is based on a crystallographic analysis. The yields were determined by 1H NMR spectroscopy. | |
Conclusions
To conclude, we have investigated the formation of adducts between N-heterocyclic carbenes and the trityl cation, a mildly oxidizing Lewis acid.23 EPR spectroscopy, UV-vis analyses, and trapping experiments indicate that the reactions proceed via an initial SET step, giving highly reactive carbene radical cations and the trityl radical. The adducts are then formed by selective cross-coupling. So far, there is scarce experimental evidence for the involvement of radical processes in Lewis acid–base reactions, in particular for systems which form stable acid–base adducts.4,6–10 The results demonstrate that SET chemistry is a viable option for reactions of NHC Lewis bases with oxidizing Lewis acids. For the present study, we have focused on the trityl cation, but our findings should be considered for reactions of NHCs with oxidizing metal ions/complexes.25
Conflicts of interest
There are no conflicts to declare.
Acknowledgements
The work was supported by the Swiss National Science Foundation, and by the École Polytechnique Fédérale de Lausanne (EPFL). We thank Dr Euro Solari for handling and mounting the sensitive single crystals.
References
-
G. N. Lewis, Valence and the Structure of Atoms and Molecules, Chemical Catalog Com., New York, 1923 Search PubMed.
-
(a) W. B. Jensen, Chem. Rev., 1978, 78, 1–22 CrossRef CAS;
(b)
S. E. Denmark and G. L. Beutner, Principles, Definitions, Terminology, and Orbital Analysis of Lewis Base-Lewis Acid Interactions Leading to Catalysis, in Lewis Base Catalysis in Organic Synthesis, ed. E. Vedejs and S. E. Denmark, Wiley-VCH, Weinhein, 2016, ch. 2, pp. 31–54 Search PubMed.
-
(a) S. S. Shaik, J. Am. Chem. Soc., 1981, 103, 3692–3701 CrossRef CAS;
(b) A. Pross, Acc. Chem. Res., 1985, 18, 212–219 CrossRef CAS;
(c) S. S. Shaik, Acta Chem. Scand., 1990, 44, 205–221 CrossRef CAS;
(d) W. E. Piers, A. J. Marwitz and L. G. Mercier, Inorg. Chem., 2011, 50, 12252–12262 CrossRef CAS PubMed.
-
(a) L. L. Liu and D. W. Stephan, Chem. Soc. Rev., 2019, 48, 3454–3463 RSC;
(b) A. R. Jupp and D. W. Stephan, Trends Chem., 2019, 1, 35–48 CrossRef.
- For general reviews about FLPs, see:
(a) F.-G. Fontaine and D. W. Stephan, Philos. Trans. R. Soc., A, 2017, 375, 20170004 CrossRef PubMed;
(b) D. W. Stephan, J. Am. Chem. Soc., 2015, 137, 10018–10032 CrossRef CAS PubMed;
(c) D. W. Stephan and G. Erker, Angew. Chem., Int. Ed., 2015, 54, 6400–6441 CrossRef CAS PubMed.
- L. Liu, L. L. Cao, Y. Shao, G. Ménard and D. W. Stephan, Chem, 2017, 3, 259–267 CAS.
- L. L. Liu, L. L. Cao, D. Zhu, J. Zhou and D. W. Stephan, Chem. Commun., 2018, 54, 7431–7434 RSC.
- For the formation of a transient frustrated radical pair from a mixture of PR3, Al(C6F5)3 and N2O, see: G. Ménard, J. A. Hatnean, H. J. Cowley, A. J. Lough, J. M. Rawson and D. W. Stephan, J. Am. Chem. Soc., 2013, 135, 6446–6449 CrossRef PubMed.
- A. Merk, H. Großekappenberg, M. Schmidtmann, M.-P. Luecke, C. Lorent, M. Driess, M. Oestreich, H. F. T. Klare and T. Müller, Angew. Chem., Int. Ed., 2018, 57, 15267–15271 CrossRef CAS PubMed.
- Z. Dong, H. H. Cramer, M. Schmidtmann, L. A. Paul, O. Siewert and T. Müller, J. Am. Chem. Soc., 2018, 140, 15419–15424 CrossRef CAS PubMed.
- For selected review articles, see:
(a) A. A. Danopoulos, T. Simler and P. Braunstein, Chem. Rev., 2019, 119, 3730–3961 CrossRef CAS PubMed;
(b) E. Peris, Chem. Rev., 2018, 118, 9988–10031 CrossRef CAS PubMed;
(c) D. Munz, Organometallics, 2018, 37, 275–289 CrossRef CAS;
(d) M. N. Hopkinson, C. Richter, M. Schedler and F. Glorius, Nature, 2014, 510, 485–496 CrossRef CAS PubMed;
(e) L. Meres and M. Albrecht, Chem. Soc. Rev., 2010, 39, 1903–1912 RSC;
(f) F. E. Hahn and M. C. Jahnke, Angew. Chem., Int. Ed., 2008, 47, 3122–3127 CrossRef CAS PubMed;
(g) W. A. Herrmann, Angew. Chem., Int. Ed., 2002, 41, 1290–1309 CrossRef CAS.
-
(a) A. Doddi, M. Peters and M. Tamm, Chem. Rev., 2019, 119, 6994–7112 CrossRef CAS PubMed;
(b) V. Nesterov, D. Reiter, P. Bag, P. Frisch, R. Holzner, A. Porzelt and S. Inoue, Chem. Rev., 2018, 118, 9678–9842 CrossRef CAS PubMed;
(c) C. E. Willans, Organomet. Chem., 2010, 36, 1–28 CAS;
(d) N. Kuhn and A. Al-Sheikh, Coord. Chem. Rev., 2005, 249, 829–857 CrossRef CAS;
(e) C. J. Carmalt and A. H. Cowley, Adv. Inorg. Chem., 2000, 50, 1–32 CrossRef CAS.
- P. A. Chase, A. L. Gille, T. M. Gilbert and D. W. Stephan, Dalton Trans., 2009, 7179–7188 RSC.
- In order to facilitate dissolution of the starting materials and the products, clorobenzene was used as solvent for the EPR and UV-vis measurements, and for the reactions, which were photographed. Similar color changes were observed in toluene.
- For the details of simulated parameters, see ESI, Fig. S6.†.
-
(a) W. P. Neumann, W. Uzick and A. K. Zarkadis, J. Am. Chem. Soc., 1986, 108, 3762–3770 CrossRef CAS;
(b) D. B. Chesnut and G. J. Sloan, J. Chem. Phys., 1960, 33, 637–638 CrossRef CAS.
-
(a) S. Fukuzumi, T. Kitano and M. Ishikawa, J. Am. Chem. Soc., 1990, 112, 5631–5632 CrossRef CAS;
(b) T. L. Chu and S. I. Weissman, J. Chem. Phys., 1954, 22, 21–25 CrossRef CAS.
- For SET processes in NHC-catalyzed reactions, see for example:
(a) B.-S. Li, Y. Wang, R. S. J. Proctor, Y. Zhang, R. D. Webster, S. Yang, B. Song and Y. R. Chi, Nat. Commun., 2016, 7, 12933–12940 CrossRef PubMed;
(b) K. Zhao and D. Enders, Angew. Chem., Int. Ed., 2017, 56, 3754–3756 CrossRef CAS PubMed;
(c) Y. Wang, X. Wu and Y. R. Chi, Chem. Commun., 2017, 53, 11952–11955 RSC;
(d) Y. Wang, Y. Du, X. Huang, X. Wu, Y. Zhang, S. Yang and Y. R. Chi, Org. Lett., 2017, 19, 632–635 CrossRef CAS PubMed;
(e) T. Ishii, Y. Kakeno, K. Nago and H. Ohamiya, J. Am. Chem. Soc., 2019, 141, 3854–3858 CrossRef CAS PubMed;
(f) V. Regnier, E. A. Romero, F. Molton, R. Jazzar, G. Bertrand and D. Martin, J. Am. Chem. Soc., 2019, 141, 1109–1117 CrossRef CAS PubMed;
(g) R. Song and Y. R. Chi, Angew. Chem., Int. Ed., 2019, 58, 8628–8630 CrossRef CAS PubMed.
-
(a) D. Leifert and A. Studer, Angew. Chem., Int. Ed., 2020, 59, 74–108 CrossRef CAS PubMed;
(b) K. Kato and A. Osuka, Angew. Chem., Int. Ed., 2019, 58, 8978–8986 CrossRef CAS PubMed.
- T. Ramnial, I. McKenzie, B. Gorodetsky, E. M. W. Tsang and J. A. C. Clyburne, Chem. Commun., 2004, 1054–1055 RSC.
- T. P. Pell, S. A. Couchman, S. Ibrahim, D. J. D. Wilson, B. J. Smith, P. J. Barnard and J. L. Dutton, Inorg. Chem., 2012, 51, 13034–13040 CrossRef CAS PubMed.
- Y. Kim, L. L. Liu and D. W. Stephan, Chem.–Eur. J., 2019, 25, 7110–7113 CrossRef CAS PubMed.
- Based on the published potentials for the IMes/IMes˙+ and Ph3C˙/Ph3C+ redox pairs, the SET process is thermodynamically favored. See: N. G. Connelly and W. E. Geiger, Chem. Rev., 1996, 96, 877–910 CrossRef CAS PubMed and ref. 20.
-
(a) A. Gómez-Suárez, D. J. Nelson and S. P. Nolan, Chem. Commun., 2017, 53, 2650–2660 RSC;
(b) T. Dröge and F. Glorius, Angew. Chem., Int. Ed., 2010, 49, 6940–6952 CrossRef PubMed.
- For example, it is known that (NHC)CuX2 complexes with non-chelating carbene ligands are difficult to access. This difficulty could be related to SET processes between the NHC ligand and Cu(II). For reports on (NHC)CuX2 complexes, see:
(a) N. Ségaud, J. McMaster, G. van Koten and M. Albrecht, Inorg. Chem., 2019, 58, 16047–16058 CrossRef PubMed;
(b) J. Cheng, L. Wang, P. Wang and L. Deng, Chem. Rev., 2018, 118, 9930–9987 CrossRef CAS PubMed;
(c) B. R. M. Lake, A. Ariafard and C. E. Willans, Chem.–Eur. J., 2014, 20, 12729–12733 CrossRef CAS PubMed;
(d) E. L. Kolychev, V. V. Shuntikov, V. N. Khrustalev, A. A. Bush and M. S. Nechaev, Dalton Trans., 2011, 40, 3074–3076 RSC;
(e) B.-L. Lin, P. Kang and T. D. P. Stack, Organometallics, 2010, 29, 3683–3685 CrossRef CAS PubMed.
Footnote |
† Electronic supplementary information (ESI) available: Containing synthetic procedures and experimental details. CCDC 1980460–1980462 and 1980595. For ESI and crystallographic data in CIF or other electronic format see DOI: 10.1039/d0sc01278e |
|
This journal is © The Royal Society of Chemistry 2020 |
Click here to see how this site uses Cookies. View our privacy policy here.