Brominated flame retardants (BFRs) in PM2.5 associated with various source sectors in southern China†
Received
21st October 2020
, Accepted 14th December 2020
First published on 16th December 2020
Abstract
The present study investigates legacy and novel brominated flame retardants (BFRs) in atmospheric PM2.5 associated with various urban source sectors in a city and electronic waste (e-waste) recycling facilities in southern China. The concentrations of polybrominated diphenyl ethers (PBDEs) and novel BFRs (∑2NBFRs) at the urban industrial park (UIP) sites varied greatly from 22.0 to 105 pg m−3 and from to 29.7 to 459 pg m−3, respectively, and higher concentrations were generally found at sites involving industrial sectors of electronics, plastics, and machinery. Their spatial variations at other urban potential source sites were small suggesting a lack of strong point emissions. The levels of PBDEs and ∑2NBFRs at the e-waste facilities (220–2356 pg m−3 and 83.6–569 pg m−3) were significantly higher and did not temporally decline, indicating that improvement in e-waste recycling techniques does not significantly reduce emissions of PBDEs. NBFRs dominated the BFRs at the urban sites (55% on average), while PBDEs were still dominant (78%) at the e-waste sites. PBDE congener profiles in PM2.5 were substantially different from those in commercial mixtures. The congener profiles as well as their correlations suggested frequent formation of lower brominated PBDEs from degradation of highly brominated congeners in this region, which became appreciable due to the reduced emissions. The significant correlations among the lower brominated congeners also reflected similar environmental behaviors due to similar physicochemical properties.
Environmental significance
We measured the BFR concentrations and compositions in PM2.5 associated with various potential source sectors in southern China. The results indicated the formation of lower brominated PBDEs from decomposition of higher congeners. Despite the application of new e-waste recycling methods, e-waste remains a significant source of BFRs in air. New e-waste recycling methods influenced the emissions, PM2.5 components, or subsequent environmental behaviors of BFRs.
|
1. Introduction
Brominated flame retardants (BFRs) are a large group of chemicals used in a variety of products or materials to prevent flaming.1 Polybrominated diphenyl ethers (PBDEs) were the most widely used BFRs; however, some PBDEs are highly persistent in the environment and bioaccumulative and may pose adverse effects to humans and wildlife. As a result, commercial pentaBDE (c-PentaBDE, containing tetra- and penta-congeners) and c-OctaBDE (containing texa- and hepta-congeners) were listed under the Stockholm Convention on Persistent Organic Pollutants (POPs) in 2009.2 The production and use of c-DecaBDE were also restricted by the Stockholm Convention in 2017, as it can form forbidden less brominated BDEs during its life cycle.3 This restriction resulted in the use of novel BFRs (NBFRs) as alternatives of PBDEs to meet the market requirement of flame retardants (FRs). For instance, decabromodiphenyl ethane (DBDPE), a replacement of commercial decaBDE (c-DecaBDE), is produced in large quantities in China.4 Subsequently, the presence of NBFRs in environmental media has been reported globally.5–9
Anthropogenic activities in urban regions are potentially significant sources of BFRs. Studies have suggested that emissions from household items are an important source of BFRs in both indoor and outdoor air, given the wide use of these chemicals in consumer goods such as furniture, electronics, and building materials.10–12 BFR concentrations in the Great Lakes atmosphere were related to the number of people within a 25 km radius of the sampling site.13,14 A recent study of legacy and emerging FRs in the urban atmosphere of Pakistan showed that higher levels are likely to associate with textile mills and garment manufacturing industries.15 In a northern city of China, Wang et al. found emissions from historical use and combustion became the important source of PBDEs after their ban.16
Increasing epidemiological research has evidenced adverse effects of fine particulate matter (PM2.5, particulate matter with diameters smaller than 2.5 micrometers) on human health in recent years.17 BFRs have a tendency to bind to fine PM due to their hydrophobic properties, which has been reported in a number of studies.18–20 Indoor PM2.5-bound BFR levels in Beijing, China were found to be significantly higher than those in outdoor counterparts; however haze pollution episodes could also contribute to higher indoor BFR levels.21 A recent study suggested that the elevated PM2.5 concentrations of PBDEs in South China were likely to associate with emissions from technical PBDEs used in manufacturing plants and e-waste recycling activities.22 Furthermore, organic compounds bound to fine particles are prone to transport long distances in the atmosphere. As a result, BFRs have been detected in air of remote and pristine regions.23–25
In most studies on urban air POPs, sampling was conducted at a certain site to represent the status of the city,16,26,27 often ignoring the possible spatial heterogeneity in emission sources. In the present study, PM2.5 was collected at various urban source sectors, which are potential emitters of BFRs into the atmosphere, in a developed city in southern China. In addition, samples from an electronic waste (e-waste) area in a rural region adjacent to this city were also obtained. The objectives are (i) to investigate the spatial distribution of PM2.5-bound BFRs in this city; (ii) to explore the possible link between the BFR concentrations and compositions and the source sectors; (iii) to understand the environmental processes that BFRs may encounter from various sources.
2. Methods
2.1 Sample collection
Urban PM2.5 samples were collected at 21 sites in Guangzhou City, an important industrial base in southern China. Among these sampling sites, 14 are industrial parks (IPs) involving industries including electrical/electronic products, chemicals, automobiles, plastics/rubber, metals, or machinery, and seven sites that were potentially impacted by specific sources were selected as follows: a residential site in an old district, a high density commercial site (new downtown area), a furnishings selling center, a leather product selling center, a wastewater treatment plant (WWTP), a resource recycling and recovery plant (RRRP), and a municipal solid waste incineration power plant (MSWIPP), respectively. The site information is given in Table S1 in the ESI.† Samples from the e-waste area were collected at four e-waste recycling parks (ERPs). This e-waste area is one of the largest e-waste sites in China, located in a rural area approximately 70 km north of Guangzhou (Fig. S1†). Primitive e-waste recycling activities have been largely restricted, and were moved to several ERPs since 2014.
Sampling campaigns were conducted mostly in the winter of 2015 and 2016 (January to April), with an average temperature of 17 °C. PM2.5 samples were collected on Whatman quartz fiber filters for 24 h using active large-volume air samplers (TE-6001-2.5I, Tisch Environment Inc., US) at a flow rate of 1.13 m3 min−1. At each site, the sampler was deployed for two and five consecutive days in the urban and e-waste areas, respectively, on the rooftop of a building near and downwind the sources. To avoid influence of precipitation on air concentrations, all the samples (n = 62) were obtained on dry days. The loaded filters were wrapped in aluminum foil, sealed in a small polyethylene zip bag, and stored at −20 °C until extraction.
2.2 Sample preparation and analysis
PM2.5 samples spiked with recovery standards (BDE77, BDE181, BDE205, and 13C-BDE209) were Soxhlet extracted with a 200 mL mixture of hexane and acetone (1
:
1, v/v) for 48 h. The extract was concentrated to about 1 mL, solvent-exchanged to hexane, and then purified with a solid-phase extraction cartridge (Supelclean ENVI-Florisil, 3 mL, 500 mg). Target analytes were eluted with 5 mL of hexane and subsequently 5 mL of 1
:
1 hexane
:
dichloromethane (v/v). The two fractions were mixed and concentrated to near dryness under a gentle nitrogen stream, and were then dissolved in 300 μL isooctane. Internal standards (BDE118 and BDE128) were added into the extracts before instrumental analysis. Analysis of BFRs was performed on a Shimadzu GCMS-QP 2010 Plus with an electron capture negative ionization mass spectrometer (GC-NCI-MS). A DB-XLB capillary column (30 m × 0.25 mm i.d., 0.25 μm film thickness) was used for the separation of 13 target PBDEs (BDE28, 35, 37, 49, 47, 66, 100, 99, 85, 154, 153, 138, and 183). A DB-5HT capillary column (15 m × 0.25 mm i.d., 0.10 μm) was used for the separation of eight PBDEs (BDE202, 197, 203, 196, 208, 207, 206, and 209), BTBPE, and DBDPE. The instrumental parameters that have been described elsewhere28 are given in the ESI.†
2.3 Quality control
Laboratory and travel blanks were processed routinely to control possible contamination from sample treatment in the laboratory and sampling in the field. Only a few analytes (BDE153, 208, 207, 206, and 209 and DBDPE) with minor concentrations were found in the blanks and their amounts were subtracted from those of the field samples. The average surrogate recoveries were 98.4 ± 7.7% for BDE77, 101 ± 11.9% for BDE181, 92.2 ± 10.8% for BDE205, and 110 ± 51.1% for 13C-BDE209. The method detection limits (MDLs) were assigned as ten times the signal to noise ratio, with values ranging from 0.02 to 8.91 pg m−3.
2.4 Data analysis
The Mann–Whitney rank sum test for comparing differences between data groups and the Pearson (two-tailed) or Spearman correlation analyses were performed with SigmaPlot 12.5. A confidence level of 95% was used for the statistics. Non-detectable values were replaced with ½ of the MDLs when calculating the means, medians, or difference comparison but not for correlation analysis.
3. Results and discussion
3.1 Spatial distributions of concentrations
Concentrations of total PBDEs at the seven urban sites that are potentially impacted by specific sources (named USS sites) ranged from 38.6–77.9 pg m−3 (with a mean of 56.0 pg m−3) (Table S2†). The concentrations of PBDE congeners from c-PentaBDE and c-OctaBDE products (included congeners are shown in Fig. 2), which have been banned in China for years, showed generally similar spatial distributions (Fig. 1). Both the concentrations of these two classes of PBDEs were highest at the XMK site in an old district. The higher concentration of these legacy BFRs in the old district may be due to emissions from old household products or building materials. Studies have suggested that household items are important emission sources of BFRs to outdoor air.21,29 Our early study has also found that PBDE emission from old indoor products was substantially higher than that from new houses.28 However, concentrations of c-DecaBDE congeners showed a different distribution (Fig. 1). The highest concentration was found at the MSWIPP site (67.9 pg m−3), followed by those at the furnishings selling center and RRRP.
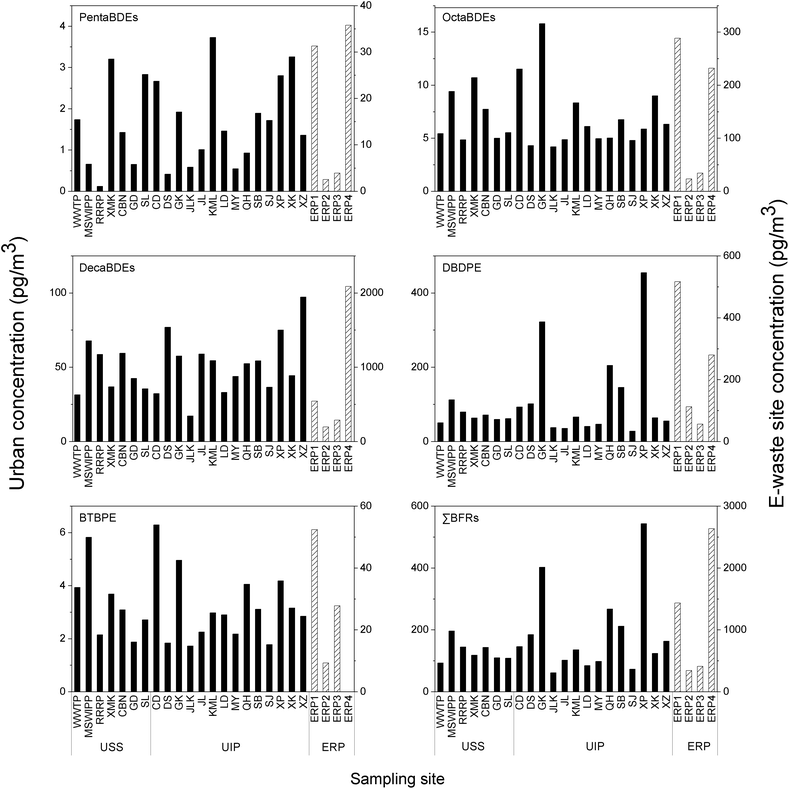 |
| Fig. 1 Mean concentrations of PM2.5-bound BFRs in the urban and e-waste areas. USS, urban specific source sites; UIP, urban industrial park sites; ERP, e-waste recycling park sites. The y-axis on the right is for ERP variables. Abbreviations for the sampling sites are listed in Table S1.† | |
At the urban industrial park (UIP) sites, concentrations of ∑PBDEs varied between 22.0 and 105 pg m−3 (with a mean of 61.2 pg m−3). Higher concentrations were found at IPs involving manufacturing household (plastic and rubber) products (XP), electronics (e.g., XP, DS, and SB) and the chemical (coating and printing ink) industry (XZ) (Fig. S2 and Table S1†). Lower concentrations were associated with IPs related to the chemical industry (JLK), textiles (SJ), and metal-product manufacturing (LD). Despite the existence of higher concentrations of PBDEs at several UIP sites, there were no statistically significant differences in the concentrations of ∑PBDEs or c-Penta-/Octa-/Deca-BDEs between USS and UIP sites.
The concentrations of ∑PBDEs at the e-waste sites (220–2356 pg m−3, mean = 941 pg m−3) were substantially higher than those from the urban sites (p = 0.002). In this e-waste area, the primitive recycling methods commonly applied in home yards in the past have generally been banned and recycling activities were moved to ERPs and formal methods were used. However, the results indicated that despite this improvement, e-waste recycling in this area remains a significant source of PBDEs in the air.
The spatial distributions of concentrations of NBFRs (DBDPE and BTBPE) are depicted in Fig. 1 and S2.† At the USS sites, ∑2NBFR concentrations were similar to those of c-DecaBDEs, with the highest levels at the MSWIPP (118 pg m−3) and lowest concentration at the WWTP (54.3 pg m−3). The presence of relatively high concentrations of both c-DecaBDEs and DBDPE at the MSWIPP and RRRP sites indicated that municipal/industrial solid waste disposal represents a significant reservoir of in-use BFRs in this city. At the UIP sites, ∑2NBFR concentrations ranged from 29.7–459 pg m−3 (with a mean of 124 pg m−3), not significantly different from those of the USS sites (with a mean of 74.6 pg m−3). The spatial distributions of ∑2NBFR at the UIP sites were different from that of c-DecaBDEs, whereas higher levels were also observed at IPs involving industrial sectors of electronics, plastics, and machinery (XP, GK, and QH). The ∑2NBFR concentrations at the ERP sites (83.6–569 pg m−3, mean = 263 pg m−3) were also significantly higher than those of the UIP sites, but the differences became much smaller than those of PBDEs (approximately 3 versus 10 folds). This result reflected that legacy compounds still constituted a significant portion of the BFRs in the air emitted from e-waste recycling at this site compared to industrial activities in the urban region. Overall, the spatial variation of total BFR concentrations at the USS sites was small, indicating a lack of strong point sources. High BFR concentrations at the urban sites were found around IPs, raising concern about the elevated BFR exposure and risk of urban residents living around the IPs in this city.
Comparison with the PM2.5-bound concentrations of BFRs in other regions in recent years is summarized in Table S3.† The urban concentrations of PBDEs and DBDPE in the present study (with a mean of 56.0 and 71.3 pg m−3) were a little higher than the levels in other Chinese cities reported by a recent study (with a mean of 35 and 50 pg m−3).22 The levels in this study were substantially lower than those in the same city reported previously (with a mean of 839 and 703 pg m−3 for PBDEs and DBDPE, respectively).27 The temporal reduction in BFR concentrations in the study city is likely due to the rigid emission control as well as effective air quality management in China in recent years.30 The more noticeable reduction for BDE209 may also be related to its foreseeable ban by the Stockholm Convention on POPs. Much higher concentrations of BDE209 (mean = 4291 pg m−3) were found in a northern city in China, but the concentrations of lower brominated PBDEs were lower (2.68 pg m−3).16 However, this reduction was not observed for BFRs in the e-waste area, where the concentrations were comparable for ∑PBDEs and even increased for DBDPE.27 This result indicated a less effective control for emissions of these chemicals from current new e-waste recycling techniques in this area. This was consistent with the high concentrations of PBDEs (20 ng m−3) found in the air inside a facility where waste printed circuit boards were formally processed in China previously.31 An average ∑35PBDE concentration of 52 pg m−3 in vapor and total particles in US cities around the Great Lakes has been reported,32 in which the average concentrations of BDE209 (11 pg m−3) and DBDPE (1.5 pg m−3) were much lower than those in this study.
3.2 Compositions
∑2NBFRs accounted for, on average, 57% and 58% of the total BFRs at the USS and UIP sites, respectively, indicating a dominance of NBFRs in this city. In the e-waste area, ∑2NBFRs constituted only 25% on average of the BFRs. For individual BFRs, BDE209 and its commercial replacement DBDPE were the most abundant chemicals in both the urban and e-waste areas together accounting for 90.6 ± 4.6% and 85.7 ± 8.0%, respectively. However, differences in their ratios were observed. The median DBDPE/BDE209 ratios were 1.8 (0.9–4.0) and 2.1 (0.4–7.3) at the USS and UIP sites, respectively, indicating more emissions of DBDPE from the UIPs than other urban sites. This is in line with the fact that DBDPE is one of the most extensive current-use BFRs in China.4 However, in the e-waste area, BDE209 still exceeded DBDPE in most of the samples, with a median ratio of 0.33. Compared to those measured previously in the same region,27 the ratios in the urban area (median = 1.1) were not statistically significantly different, whereas the ratios in the e-waste area (median = 0.10) were significantly lower (p < 0.001, Mann–Whitney Rank Sum Test). This result suggests a noticeable shift from c-DecaBDE-containing to novel DBDPE-containing obsolete products or an association with the transformation of recycling techniques (from primitive recycling to controlled ERPs) in this area.
Fig. 2 displays the congener profiles of lower brominated PBDEs, typically components of c-PentaBDE products. The profiles from both urban and e-waste sites were quite different from those in the commercial products, in which BDE47 and 99 were dominant (with approximate proportions of 37% and 42%, respectively).33 Although alterations in profiles have been observed in the environment in the study region in previous studies, BDE47 and 99 remained dominant.27,34–36 Also considering the detection frequencies of BDE47 and 99 in the samples, we speculated that at least some of these congeners were derived from degradation of highly brominated congeners, or these congeners were subjected to degradation, which exerts an appreciable influence on the compositions when the emissions are weak. Aerobic and anaerobic biodegradation and photo-degradation of PBDEs have been demonstrated previously,37,38 and generally, the degradation of lower brominated PBDEs is faster.38 Likewise, congener profiles of PBDEs that are components of the c-OctaBDE product also underwent significant alterations in the urban region. In most of the samples, the contributions of BDE183 considerably decreased (only 20% on average) and those of BDE203 and 196 substantially increased (35% and 38%) relative to their proportions in the commercial products. However, in the e-waste area, the profiles of c-OctaBDE resembled that in the commercial products, indicating that dismantling e-waste containing the c-OctaBDE mixture was their primary source. This was not unexpected given that the main use of the c-OctaBDE product was in housings/casings of electrical and electronic equipment.39 For these lower brominated PBDEs in the urban region, one exception is the old district site where the profiles were similar to those in the c-Penta- and c-OctaBDE products, respectively. At this site, the highest concentrations of both c-Penta- and c-OctaBDE congeners were also found; thus there was likely a stronger emission than that of the degradation source of these legacy PBDEs near this site. In the old downtown area, there is greater likelihood of emissions from old household products or building materials. Deca- and nonaBDEs are the main components of the c-DecaBDE products. The average ratios of decaBDE/(decaBDE + nonaBDEs) were 0.71 and 0.78 at the USS and UIP sites, respectively. The ratios were significantly lower than those reported in the commercial products (0.90–0.97)33 and those in the air in this city in previous studies (with mean of 0.96 and 0.88).27,28 This declining trend in the ratios was probably a result of degradation of decaBDE and reduction in emissions due to its ban in 2017.40 It is a little surprising that the ratios at the ERP sites (mean = 0.80) were also lower than previous values at this site, with means of 0.88 and 0.85.27,41 However, there was not a temporal declining trend in the ratios. The compositions of these highly brominated BDEs at this e-waste site could be affected not only by the reduced emission (due to replacement of novel BFRs in the obsoleting electrical and electronic equipment) and photo-/bio-degradation, but also by the processing techniques of e-waste. It has been implied that BFRs in e-waste may be subjected to thermal decomposition during the processing.
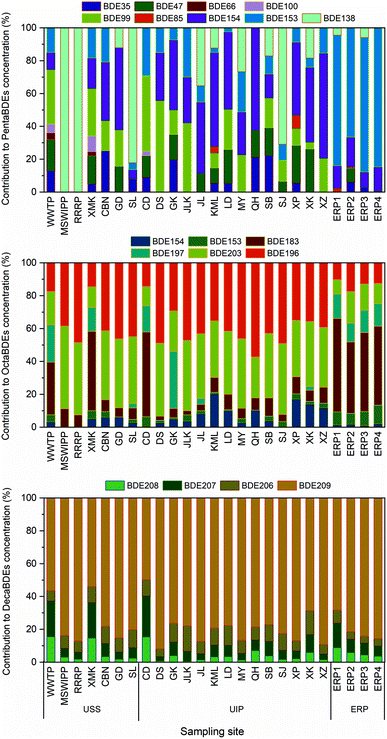 |
| Fig. 2 Compositions of PBDEs in PM2.5 in the urban and e-waste areas. USS, urban specific source sites; UIP, urban industrial park sites; ERP, e-waste recycling park sites. Abbreviations for the sampling sites are listed in Table S1.† | |
3.3 Correlation analysis
Correlation analysis was conducted to understand the relationships between the BFRs and explore their environmental processes or sources. Generally, moderate but significant correlations were found among the major lower brominated BDE congeners (BDE47, 99, 154, and 153) (r > 0.324, p < 0.04, Fig. S3†), although c-PentaBDE may not be their main origin as mentioned above. The significant correlations may also be attributed to the similarity in their physicochemical properties (i.e., similar environmental behaviors). Furthermore, these congeners all had significant correlations with BDE183 (r > 0.315, p < 0.048), a typical congener of the c-OctaBDE product (36–47%), and BDE207 and 208 (r > 0.330, p < 0.037), which are minor congeners of the c-DecaBDE product (0.07–4%).33 In particular, BDE47 and 99 had strong correlations with these three congeners (r > 0.499, p < 0.001). This result may further indicate the formation of these lower brominated BDEs from highly brominated PBDEs. Surprisingly, BDE207 and 208 (components of c-DecaBDE) showed no correlations with BDE209, whereas BDE206, 203, and 196 were significantly correlated with it (r > 0.438, p < 0.005). BDE206 is the major congener next to BDE209, but BDE203 and 196 are minor components in c-DecaBDE mixtures. The relationships of nona- and octa-BDE with BDE209 suggest a high likelihood that some of these congeners were degradation products of decaBDE and some were subjected to further degradation. A recent study simulating stepwise photolytic debromination pathways of BDE209 by sunlight with an analogue Markov Chain Monte Carlo algorithm also found that BDE206 has higher accumulation and BDE207 has the higher depletion in producing octa products, although the two congeners had comparable yields from BDE209.42 There was a significant correlation between DBDPE and BTBPE (r = 0.502, p = 0.001) and moderate correlation between DBDPE and BDE209 (r = 0.347, p = 0.028). BTBPE was significantly correlated with BDE183, 207, and 208 (r > 0.723, p < 0.001). A possible explanation is that BTBPE is a replacement of an c-OctaBDE mixture.43
Correlations between BFRs and other pollutants including organophosphorus esters (OPEs) and polycyclic aromatic hydrocarbons (PAHs) in these samples44 were also analyzed. OPEs are a class of flame retardants and plasticizers and widely used in many products like BFRs.45 PAHs are well-known organic pollutants emitted from various combustion or thermal treatment sources.46 In the urban region, BFRs (mostly highly brominated c-DecaBDEs and DBDPE) displayed significant correlations with triphenyl phosphate (TPHP) (r > 0.428, p < 0.006, Fig. S2†), an OPE that is documented as an alternative for the c-DecaBDE mixture extensively applied in electronic and electrical products.47 The majority of BFRs (except for BDE209, DBDPE, and a few other BDEs) derived from c-PentaBDE or c-OctaBDE mixtures were moderately correlated with tris(2-ethylhexyl) phosphate (TEHP). TEHP is used in polyvinyl chloride (PVC), cellulose, rubber, and polyurethane foam,44 in which these PBDEs could be used before their ban.48 Other OPEs had rarely significant correlations with BFRs. The findings indicated that application is an important factor determining the relationships between PBDEs and OPEs in the environment.
There were significant correlations between most of the BFRs (except for BDE206, and BDE209, and DBDPE) and most of the PAHs, particularly for 4- and 5-ring compounds. The association with PAHs in this region did not necessarily point to predominant combustion sources for these BFRs, but similarity in the environmental processes in the air. These lower brominated BFRs and high-ring PAHs have closer physicochemical properties (e.g., KOA, octanol-air partition coefficient) (Table S4†). Particle/gas partitioning plays an important role in the occurrence of semivolatile compounds (e.g., PAHs and lower brominated BFRs) in particles.49 The partitioning is considered to be controlled by absorption into organic matter and/or adsorption onto black carbon within the particle, and KOA has been used to describe the partitioning.50,51 The correlations between these BFRs and OC and EC contents in PM further support this hypothesis.
In the e-waste area, strong correlations were observed among nearly all the BFRs (Fig. S3†), indicating a common emission from e-waste recycling. BFRs also showed good correlations with several OPEs including TPhP, TEHP, tricresyl phosphate (TMPP), and 2-ethylhexyl diphenyl phosphate (EHDPP). For BDE209, however, good correlation with only TPhP was found. This may be a hint of decomposition of BDE209 disturbing the relationship. Unlike those in the urban region, most BFRs were moderately correlated with some of the PAHs including three to five-ring compounds. It is surprising that BFRs were not correlated with OC, but were partly correlated with EC. This finding differed from the previous observations in this e-waste area that BFRs were closely associated with OC and EC.27 PM2.5 samples in this study were obtained near a site where e-waste was processed using primitive techniques (e.g., burning), while samples in the present study were obtained near ERPs using controlled formal methods. This difference could account for their associations with OC and EC. The results suggest that moving to ERPs and application of formal recycling methods affect the emissions, PM2.5-bound components, or subsequent environmental behaviors of BFRs.
4. Conclusion
The present study investigates the occurrence of PM2.5-bound legacy and two novel BFRs associated with various source sectors in a Chinese city. The banned lower brominated BFRs showed considerably low concentrations and NBFRs were dominant. BFR concentrations at industrial parks were highly spatially heterogeneous, depending on the industrial sectors. Although e-waste recycling activities have been moved to industrial parks, they remain a significant source of BFRs (dominated by PBDEs). We observed congener profiles of PBDEs substantially different from those in the commercial mixtures and in previous environments in this region. The PBDE profiles and their correlations suggest that these PBDEs were partly formed from decomposition of highly brominated congeners in the urban region. The correlations for the e-waste sites suggest that the transformation of e-waste recycling methods affects the emissions, PM2.5 components, or subsequent environmental behaviors of BFRs. A limitation of this study is the small sample size in the urban area. Another is lack of information on gaseous phase BFRs, including the levels, sources, and environmental fate.
Conflicts of interest
There are no conflicts of interest to declare.
Acknowledgements
This study was financially supported by the National Key R&D Program of China (2017YFC0212000), the Guangzhou Science and Technology Program (No. 201707020033), the National Science Foundation of China (No. 41771530), the Guangdong Foundation for Program of Science and Technology Research (No. 2017B030314057), and the State Key Laboratory of Organic Geochemistry, GIGCAS (No. SKLOG202001).
References
- A. Salamova, M. H. Hermanson and R. A. Hites, Organophosphate and halogenated flame retardants in atmospheric particles from a European Arctic site, Environ. Sci. Technol., 2014, 48, 6133–6140 CrossRef CAS.
-
Stockholm Convention on Persistent Organic Pollutants (2009), Adoption of amendments to annexes A, B, and C. Report #C.N.524.2009, TREATIES-4, http://chm.pops.int/Implementation/IndustrialPOPs/BDEs/Additionalresources/tabid/5376/Default.aspx, accessed September, 2020 Search PubMed.
-
Stockholm Convention on Persistent Organic Pollutants 2010, Debromination of brominated flame retardants (UNEP/POPS/POPRC.6/INF/20), http://chm.pops.int/TheConvention/POPsReviewCommittee/Meetings/POPRC6/POPRC6Documents/tabid/783/Default.aspx, accessed September, 2020 Search PubMed.
- A. Covaci, S. Harrad, M. A. Abdallah, N. Ali, R. J. Law, D. Herzke and C. A. de Wit, Novel brominated flame retardants: A review of their analysis, environmental fate and behaviour, Environ. Int., 2011, 37, 532–556 CrossRef CAS.
- P. Wang, Q. Zhang, Y. Li, J. Matsiko, Y. Zhang and G. Jiang, Airborne persistent toxic substances (PTSs) in China: Occurrence and its implication associated with air pollution, Environ. Sci.: Process Impacts, 2017, 19, 983–999 CAS.
- T. J. McGrath, A. Kolobaric, E. Lee and B. O. Clarke, Brominated flame retardants (BFRs) in Western Australian biosolids and implications for land application, Chemosphere, 2020, 260, 127601 CrossRef CAS.
- J. Ma, X. Li, S. Ma, X. Zhang, G. Li and Y. Yu, Temporal trends of “old” and “new” persistent halogenated organic pollutants in fish from the third largest freshwater lake in China during 2011–2018 and the associated health risks, Environ. Pollut., 2020, 267, 115497 CrossRef CAS.
- Y. Hao, W. Meng, Y. Li, X. Han, H. Lu, P. Wang, R. Yang, Q. Zhang and G. Jiang, Concentrations and distribution of novel brominated flame retardants in the atmosphere and soil of Ny-Alesund and London Island, Svalbard, Arctic, J. Environ. Sci. (China), 2020, 97, 180–185 CrossRef.
- J. Q. Guo, Y. F. Li, L. Y. Liu, C. Y. Huo, Y. Sun, W. L. Ma, Z. F. Zhang and Y. F. Li, Occurrence and partitioning of brominated flame retardants (BFRs) in indoor air and dust: A 15-month case study in a test home, Environ. Sci. Pollut. Res., 2020, 27, 35126–35136 CrossRef CAS.
- I. C. Yadav, N. L. Devi, A. Kumar, J. Li and G. Zhang, Airborne brominated, chlorinated and organophosphate ester flame retardants inside the buildings of the Indian state of Bihar: Exploration of source and human exposure, Ecotoxicol. Environ. Saf., 2020, 191, 110212 CrossRef CAS.
- Y. Li, L. Chen, D. M. Ngoc, Y. P. Duan, Z. B. Lu, Z. H. Wen and X. Z. Meng, Polybrominated diphenyl ethers (PBDEs) in PM2.5, PM10, TSP and gas phase in office environment in Shanghai, China: Occurrence and human exposure, PLos One, 2015, 10, e0119144 CrossRef.
- X. Zhang, M. L. Diamond, M. Robson and S. Harrad, Sources, emissions, and fate of polybrominated diphenyl ethers and polychlorinated biphenyls indoors in Toronto, Canada, Environ. Sci. Technol., 2011, 45, 3268–3274 CrossRef CAS.
- Y. Ma, A. Salamova, M. Venier and R. A. Hites, Has the phase-out of PBDEs affected their atmospheric levels? Trends of PBDEs and their replacements in the Great Lakes atmosphere, Environ. Sci. Technol., 2013, 47, 11457–11464 CrossRef CAS.
- L.-Y. Liu, M. Venier, A. Salamova and R. A. Hites, A novel flame retardant in the Great Lakes atmosphere: 3,3′,5,5′-Tetrabromobisphenol A bis(2,3-dibromopropyl) ether, Environ. Sci. Technol. Lett., 2016, 3, 194–199 CAS.
- J. H. Syed, M. Iqbal, K. Breivik, M. J. I. Chaudhry, M. Shahnawaz, Z. Abbas, J. Nasir, S. H. H. Rizvi, M. M. Taqi, J. Li and G. Zhang, Legacy and emerging flame retardants (FRs) in the urban atmosphere of Pakistan: Diurnal variations, gas-particle partitioning and human
health exposure, Sci. Total Environ., 2020, 743, 140874 CrossRef CAS.
- Y. Wang, Y. Zhang, F. Tan, Y. Yang, Z. Qu, J. Kvasnicka and J. Chen, Characteristics of halogenated flame retardants in the atmosphere of Dalian, China, Atmos. Environ., 2020, 223, 117219 CrossRef CAS.
- L. Jin, X. Luo, P. Fu and X. Li, Airborne particulate matter pollution in urban China: A chemical mixture perspective from sources to impacts, Natl. Sci. Rev., 2017, 4, 593–610 CrossRef CAS.
- Y. Li, T. Lin, F. Wang, T. Ji and Z. Guo, Seasonal variation of polybrominated diphenyl ethers in PM2.5 aerosols over the East China Sea, Chemosphere, 2015, 119, 675–681 CrossRef CAS.
- A. de la Torre, B. Barbas, P. Sanz, I. Navarro, B. Artinano and M. A. Martinez, Traditional and novel halogenated flame retardants in urban ambient air: Gas-particle partitioning, size distribution and health implications, Sci. Total Environ., 2018, 630, 154–163 CrossRef CAS.
- Y. F. Li, L. N. Qiao, N. Q. Ren, E. Sverko, D. Mackay and R. W. Macdonald, Decabrominated diphenyl ethers (BDE-209) in Chinese and global air: Levels, gas/particle partitioning, and long-range transport: Is long-range transport of BDE-209 really governed by the movement of particles?, Environ. Sci. Technol., 2017, 51, 1035–1042 CrossRef CAS.
- D. Wang, P. Wang, Y. Zhu, R. Yang, W. Zhang, J. Matsiko, W. Meng, P. Zuo, Y. Li, Q. Zhang and G. Jiang, Seasonal variation and human exposure assessment of legacy and novel brominated flame retardants in PM2.5 in different microenvironments in Beijing, China, Ecotoxicol, Environ. Saf., 2019, 173, 526–534 CAS.
- D. Liu, T. Lin, K. Shen, J. Li, Z. Yu and G. Zhang, Occurrence and concentrations of halogenated flame retardants in the atmospheric fine particles in Chinese cities, Environ. Sci. Technol., 2016, 50, 9846–9854 CrossRef CAS.
- M. D. Mulder, A. Heil, P. Kukučka, J. Kuta, P. Přibylová, R. Prokeš and G. Lammel, Long-range atmospheric transport of PAHs, PCBs and PBDEs to the central and eastern Mediterranean and changes of PCB and PBDE congener patterns in summer 2010, Atmos. Environ., 2015, 111, 51–59 CrossRef CAS.
- C. L. Davie-Martin, K. J. Hageman, Y. P. Chin, B. J. Nistor and H. Hung, Concentrations, gas-particle distributions, and source indicator analysis of brominated flame retardants in air at Toolik Lake, Arctic Alaska, Environ. Sci.: Process Impacts, 2016, 18, 1274–1284 CAS.
- M. A. Khairy, J. L. Luek, R. Dickhut and R. Lohmann, Levels, sources and chemical fate of persistent organic pollutants in the atmosphere and snow along the western Antarctic Peninsula, Environ. Pollut., 2016, 216, 304–313 CrossRef CAS.
- J. Li, X. Liu, L. L. Yu, G. Zhang, X. D. Li, C. S. Lee and H. T. Lin, Comparing polybrominated diphenyl ethers (PBDEs) in airborne particles in Guangzhou and Hong Kong: Sources, seasonal variations and inland outflow, J. Environ. Monit., 2009, 11, 1185–1191 RSC.
- N. Ding, S. J. Chen, T. Wang, T. Wang and B. X. Mai, Halogenated flame retardants (HFRs) and water-soluble ions (WSIs) in fine particulate matter (PM2.5) in three regions of South China, Environ. Pollut., 2018, 238, 823–832 CrossRef CAS.
- N. Ding, T. Wang, S. J. Chen, M. Yu, Z. C. Zhu, M. Tian, X. J. Luo and B. X. Mai, Brominated flame retardants (BFRs) in indoor and outdoor air in a community in Guangzhou, a megacity of southern China, Environ. Pollut., 2016, 212, 457–463 CAS.
- F. Wong, C. A. de Wit and S. R. Newton, Concentrations and variability of organophosphate esters, halogenated flame retardants, and polybrominated diphenyl ethers in indoor and outdoor air in Stockholm, Sweden, Environ. Pollut., 2018, 240, 514–522 CrossRef CAS.
- X. Lu, S. Zhang, J. Xing, Y. Wang, W. Chen, D. Ding, Y. Wu, S. Wang, L. Duan and J. Hao, Progress of air pollution control in China and its challenges and opportunities in the ecological civilization era, Engineering, 2020, 6, 1423–1431 CrossRef.
- J. Guo, A. Ji, J. Wang, O. A. Ogunseitan and Z. Xu, Emission characteristics and exposure assessment of particulate matter and polybrominated diphenyl ethers (PBDEs) from waste printed circuit boards de-soldering, Sci. Total Environ., 2019, 662, 530–536 CrossRef CAS.
- J. Castro-Jimenez, B. Barhoumi, A. Paluselli, M. Tedetti, B. Jimenez, J. Munoz-Arnanz, H. Wortham, M. Ridha Driss and R. Sempere, Occurrence, loading, and exposure of atmospheric particle-bound POPs at the African and European edges of the Western Mediterranean Sea, Environ. Sci. Technol., 2017, 51, 13180–13189 CrossRef CAS.
- M. L. GUARDIA, R. HALE and E. NHARVEY, Detailed polybrominated diphenyl ether (PBDE) congener composition of the widely used penta-, octa-, and deca-PBDE technical flame-retardant mixtures, Environ. Sci. Technol., 2006, 40, 6247–6254 CrossRef.
- S. J. Chen, A. H. Feng, M. J. He, M. Y. Chen, X. J. Luo and B. X. Mai, Current levels and composition profiles of PBDEs and alternative flame retardants in surface sediments from the Pearl River Delta, southern China: Comparison with historical data, Sci. Total Environ., 2013, 444, 205–211 CrossRef CAS.
- W. J. Deng, J. S. Zheng, X. H. Bi, J. M. Fu and M. H. Wong, Distribution of PBDEs in air particles from an electronic waste recycling site compared with Guangzhou and Hong Kong, South China, Environ. Int., 2007, 33, 1063–1069 CrossRef CAS.
- J. Li, T. Lin, S.-H. Pan, Y. Xu, X. Liu, G. Zhang and X.-D. Li, Carbonaceous matter and PBDEs on indoor/outdoor glass window surfaces in Guangzhou and Hong Kong, South China, Atmos. Environ., 2010, 44, 3254–3260 CrossRef CAS.
- Y. Pan, D. C. W. Tsang, Y. Wang, Y. Li and X. Yang, The photodegradation of polybrominated diphenyl ethers (PBDEs) in various environmental matrices: Kinetics and mechanisms, Chem. Eng. J., 2016, 297, 74–96 CrossRef CAS.
- C. Zhao, M. Yan, H. Zhong, Z. Liu, L. Shi, M. Chen, G. Zeng, B. Song, B. Shao and H. Feng, Biodegradation of polybrominated diphenyl ethers and strategies for acceleration: A review, Int. Biodeter. Biodegr., 2018, 129, 23–32 CrossRef CAS.
-
European Union risk assessment report. Diphenyl ether, octabromo derivative, https://www.chemeurope.com/en/encyclopedia/Octabromodiphenyl_ether.html#_ref-EU2003_2/, accessed September, 2020.
-
Stockholm Convention on Persistent Organic Pollutants, 2017, The 16 new POPs, an introduction to the chemicals added to the Stockholm Convention as persistent organic pollutants by the Conference of the Parties, http://chm.pops.int/TheConvention/ThePOPs/TheNewPOPs/tabid/2511/Default.aspx, accessed September, 2020.
- M. Tian, S. J. Chen, J. Wang, X. B. Zheng, X. J. Luo and B. X. Mai, Brominated flame retardants in the atmosphere of e-waste and rural sites in southern China: Seasonal variation, temperature dependence, and gas-particle partitioning, Environ. Sci. Technol., 2011, 45, 8819–8825 CrossRef CAS.
- Y. Zou, E. R. Christensen, W. Zheng, H. Wei and A. Li, Estimating stepwise debromination pathways of polybrominated diphenyl ethers with an analogue Markov Chain Monte Carlo algorithm, Chemosphere, 2014, 114, 187–194 CrossRef CAS.
- Y. Meng, H. Zhang, Y. Qiu, X. Du, D. Niu, Z. Lu, Z. Zhu, J. Zhao and D. Yin, Seasonal distribution, gas–particle partitioning and inhalation exposure of brominated flame retardants (BFRs) in gas and particle phases, Environ. Sci. Eur., 2019, 31, 61 CrossRef.
- T. Wang, N. Ding, T. Wang, S. J. Chen, X. J. Luo and B. X. Mai, Organophosphorus esters (OPEs) in PM2.5 in urban and e-waste recycling regions in southern China: Concentrations, sources, and emissions, Environ. Res., 2018, 167, 437–444 CrossRef CAS.
- A. Moller, R. Sturm, Z. Xie, M. Cai, J. He and R. Ebinghaus, Organophosphorus flame retardants and plasticizers in airborne particles over the Northern Pacific and Indian Ocean toward the polar regions: Evidence for global occurrence, Environ. Sci. Technol., 2012, 46, 3127–3134 CrossRef.
- K. Slezakova, D. Castro, C. Delerue-Matos, M. d. C. Alvim-Ferraz, S. Morais and M. d. C. Pereira, Impact of vehicular traffic emissions on particulate-bound PAHs: Levels and associated health risks, Atmos. Res., 2013, 127, 141–147 CrossRef CAS.
-
An alternatives assessment for the flame retardant decabromodiphenyl ether (DecaBDE)
https://www.epa.gov/assessing-and-managing-chemicals-under-tsca/alternatives-assessment-flame-retardant, accessed September, 2020 Search PubMed.
-
Stockholm Convention on Persistent Organic Pollutants 2010, Technical review of the implications of recycling commercial pentabromodiphenyl ether and commercial octabromodiphenyl ether (UNEP/POPS/POPRC.6/2), http://chm.pops.int/TheConvention/POPsReviewCommittee/Meetings/POPRC6/POPRC6Documents/tabid/783/Default.aspx, accessed September, 2020 Search PubMed.
- K.-U. GOSS and R. P. SCHWARZENBACH, Gas/solid and gas/liquid partitioning of organic compounds: Critical evaluation of the interpretation of equilibrium constants, Environ. Sci. Technol., 1998, 32, 1–53 CrossRef.
- J. Dachs and S. J. Eisenreich, Adsorption onto aerosol soot carbon dominates gas-particle partitioning of polycyclic aromatic hydrocarbons, Environ. Sci. Technol., 2000, 34, 690–3697 Search PubMed.
- A. Finizio, D. Mackay, T. Bidleman and T. Harner, Octanol-air partition coefficient as a predictor of partitioning of semi-volatile organic chemicals to aerosols, Atmos. Environ., 1997, 31, 2289–2296 CrossRef CAS.
Footnote |
† Electronic supplementary information (ESI) available. See DOI: 10.1039/d0em00443j |
|
This journal is © The Royal Society of Chemistry 2021 |
Click here to see how this site uses Cookies. View our privacy policy here.