DOI:
10.1039/D0GC03557B
(Paper)
Green Chem., 2021,
23, 405-411
Highly efficient separation of 5-hydroxymethylfurfural from imidazolium-based ionic liquids†
Received
21st October 2020
, Accepted 28th November 2020
First published on 9th December 2020
Abstract
Ionic liquids (ILs) have been widely studied as effective reaction media or catalysts for the conversion of renewable resources to 5-hydroxymethhylfurfural (5-HMF). However, the separation of 5-HMF from ILs remains a significant challenge. Thus, the aim of this work is to develop a useful guideline for the selection and design of greener solvents for efficient separation of 5-HMF from ILs by a simple solvent extraction approach. To this end, 9 kinds of low-toxic and medium boiling point organic solvents and 10 kinds of ILs typically used in biomass conversion have been evaluated for the separation of 5-HMF. The effects of the solvent hydrogen bond acceptor capacity, anion type and cationic structure of the ILs on the separation of 5-HMF from the ILs are systematically investigated and the molecular interactions among 5-HMF, ILs and the solvent contained in the mixtures have been revealed by FTIR and 1H NMR measurements. It has been found that besides a low mutual solubility with ionic liquids, an ideal solvent should have strong hydrogen bond acceptor properties. At the same time, the interactions between the anion and cation of the ILs and the hydrogen bonding interaction of the anion with 5-HMF are also very important for the separation of 5-HMF. A weak interaction of the anion with 5-HMF and a strong interaction between the anion and cation of the ILs all result in the high extraction efficiency. Under the optimized conditions, the extraction efficiency of 5-HMF from the ILs is up to 61.0%, which is a record-breaking value at the current time. This finding provides a useful guide for the selection of greener solvents for the separation of 5-HMF from ILs.
Introduction
To relieve worldwide problems related to the consumption of fossil fuel resources, great efforts have been made in the exploitation of biomass.1–4 In a biorefinery, the conversion of biomass into 5-hydroxymethylfurfural (5-HMF) has become a very important pathway due to the fact that 5-HMF is a critical raw material for the synthesis of platform chemicals, such as 2,5-dimethyfuran, levulinic acid, 2,5-furandicarboxyaldehyde and 5-hydroxymethyfuroic acid.5–8 Therefore, significant attention has been paid to the conversion of biomass into 5-HMF. Recently, ionic liquids (ILs) have provided a new chance for efficient and clean manufacture of 5-HMF from biomass on account of their ultralow volatility, high thermal stability, low melting points and strong ability to solubilize a variety of materials.9 Many investigations on the use of ILs as solvents or catalysts in the manufacture of 5-HMF have been carried out.10–17 Indeed, the application of ILs enhances the selectivity to 5-HMF and the conversion ratio. However, when the reaction is completed, the isolation of the 5-HMF product from ILs is very difficult and it still remains a great challenge.
The isolation of 5-HMF from ILs is impracticable by conventional distillation because 5-HMF can be disintegrated easily at high temperature and the boiling points of ILs are very high. Therefore, only the solvent extraction method has been applied to isolate 5-HMF from ILs. In this context, Su et al.18 used methyl isobutyl ketone (MIBK) to extract 5-HMF at 100 °C after cellulose conversion in the 1-ethyl-3-methylimidazolium chloride ([Emim]Cl) solvent. After three successive 8 h runs were conducted, more than 90% of 5-HMF was separated at 100 °C. Stark et al.19 also used MIBK to extract 5-HMF from 1-butyl-3-methylimidazolium methanesulfonate ([Bmim][CH3SO3]) at 80 °C. The extraction efficiency was only about 3%. Zhang et al.20 investigated 5-HMF separation using a model two-phase system that comprises [Bmim]Cl (the solvent phase) and MIBK (the extractive phase) with the addition of appropriate promoters (such as methanol and ethanol). It was found that the extraction efficiency of 5-HMF from [Bmim]Cl was improved by using short chain alcohol promoters. However, the separation of 5-HMF from the ILs encountered a problem of low extraction efficiency (<15%), which may limit the practical application of this strategy in industrial processes.
In principle, to effectively improve the separation efficiency of 5-HMF by solvent extraction, the following three issues must be clarified: (i) what is the prevailing interaction between 5-HMF and ILs, (ii) how the IL structures affect the separation efficiency, and (iii) what is the most efficient extracting solvent that can weaken or even break the interaction between 5-HMF and ILs to achieve the separation purpose. Among these three issues, the prevailing interactions between 5-HMF and ILs have been investigated by us in our recent publications.9,21 It has been shown that the prevailing interactions of ILs with 5-HMF are attributed to the strong hydrogen bonds between 5-HMF and the anions of the ILs. Nevertheless, the other two issues have not been better understood. Therefore, in this work, the effects of the IL structures and extracting solvent properties on the separation of 5-HMF are systematically investigated by partition experiments, Fourier transform infrared (FTIR) and 1H nuclear magnetic resonance (1H NMR) spectroscopy. Based on these results, the optimal selection of a highly efficient extracting solvent and the “best” IL for the separation of 5-HMF has been achieved.
Results and discussion
The effect of extraction conditions on the separation of 5-HMF
In order to determine the optimal operating conditions for the separation of 5-HMF from the ILs, we investigated the effects of the system temperature, initial concentrations of 5-HMF in the ILs and extraction time on the extraction efficiency of 5-HMF. As an example, Fig. 1(a) shows the results for the separation of 5-HMF from a representative ionic liquid 1-butyl-3-methylimidazolium methyl sulfonate ([Bmim][CH3OSO3]) used in the biomass conversion by using tetrahydrofuran (THF) as the extracting solvent at different temperatures. The extraction percentage was found to have only a slight change in the temperature range of 20–40 °C. In addition, for the other ILs investigated in this work, the same trend was observed (as shown in Fig. S1†). This temperature insensitivity allows us to perform the separation process at room temperature. Fig. 1(b) and Fig. S2† describe the changes in the extraction efficiency of 5-HMF with different initial concentrations in the ILs ranging from 60 to 300 mg mL−1. It is clearly noted that the extraction percentage of 5-HMF did not vary appreciably with the increasing initial concentration of 5-HMF in the ILs. Furthermore, the effect of the extraction time on the extraction percentage was investigated at 25 °C. It was shown that the extraction efficiency of 5-HMF remained almost unchanged after 40 min under stirring. Therefore, the extraction experiments were completed under the following conditions: initial 5-HMF concentration of 60 mg mL−1, 25 °C and a contact time of 40 min throughout the investigation.
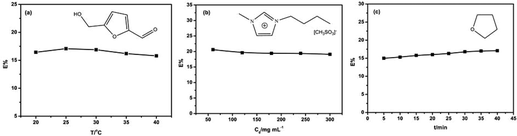 |
| Fig. 1 The effect of system temperature (a), initial concentrations of 5-HMF in the ILs (b) and extraction time (c) on the extraction efficiency of 5-HMF from [Bmim][CH3OSO3] by using THF as the extracting solvent. The inset charts in panels (a)–(c) show the molecular structures of 5-HMF, [Bmim][CH3OSO3], and THF, respectively. | |
The effect of the extracting solvent properties on the separation of 5-HMF
In principle, to effectively improve the extraction efficiency of 5-HMF, an ideal extracting solvent should have the following two characteristics: (i) it should not be miscible with ILs but must have strong interaction with 5-HMF; and (ii) its boiling point should not be high to avoid side reactions such as thermal decomposition and polymerization of 5-HMF during the separation of 5-HMF from an extracting solvent by simple distillation. Therefore, [Bmim][CH3OSO3] and a wide range of medium boiling point extracting solvents, including methanol (MeOH), ethanol (EtOH), butanol (BuOH), butyl acetate (BuOAc), succinic acid diethylester (SADE), methyl iso-butylketone (MIBK), methyl tert-butylether (MTBE), tetrahydrofuran (THF), and ethyl acetate (EtOAc), were selected for systematic evaluation of the separation performance of 5-HMF from the ILs at 25 °C. Based on the CHEM21 solvent selection guide,22 solvents can be divided into three categories (recommended, problematic or hazardous), and the solvents selected here are ranked as “recommended” or “problematic” and can be used for scaling-up except for methyl tert-butylether which is ranked as “hazardous”. That is, methanol, ethanol, butanol, butyl acetate, methyl iso-butylketone, tetrahydrofuran, and ethyl acetate are all greener solvents. It was found that among the solvents studied here, those with the hydrogen bond donor property (methanol, ethanol, and butanol) have high solubility in ILs, and are not applicable as extracting solvents for liquid–liquid separation. The other solvents with the hydrogen bond acceptor property (butyl acetate, succinic acid diethylester, methyl iso-butylketone, methyl tert-butylether, tetrahydrofuran, and ethyl acetate) could form biphase systems with ILs to extract 5-HMF. The 5-HMF extraction efficiencies of different extracting solvents at 25 °C are shown in Fig. 2(a) and Fig. S3 and S4.† It is clear that the extraction efficiency in [Bmim][CH3OSO3] decreased in the order THF (17.1%) > MIBK (14.1%) > SADE (10.7%) > EtOAc (8.6%) > BuOAc (7.3%) > MTBE (3.3%). In addition, the β values (i.e., the hydrogen bond acceptor strength) of these extracting solvents decreased in the sequence THF (0.55) > MIBK (0.48) > SADE (0.46) > EtOAc (0.45) > BuOAc (0.43).23,24 Interestingly, the order of the extraction efficiency is approximately in agreement with that of the β values. This result indicates that the extracting solvent with a higher β value (stronger hydrogen bond acceptor ability) possesses a higher extraction capacity for 5-HMF. For example, THF has the highest β value, and its extraction efficiency for 5-HMF is also maximal. In addition, the partition coefficients (K) of 5-HMF between the extracting solvent phase and the IL phase are also determined, and the relationship between the K values and β values of the extracting solvents is evaluated. An approximately linear relationship between the K values and β values is found (shown in Fig. 2(b)).
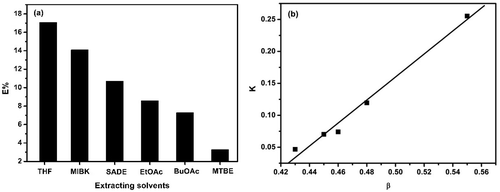 |
| Fig. 2 (a) The extraction efficiency of 5-HMF from [Bmim][CH3OSO3] for different extracting solvents at 25 °C, (b) the partition coefficients of 5-HMF between the extracting solvent phase and [Bmim][CH3OSO3] phase as a linear function of β-values of the extracting solvents. | |
In order to understand the variation in the extraction efficiency with β values of the extracting solvents at the molecular level, FTIR and 1H NMR spectroscopy were used to study the interaction of 5-HMF with the extracting solvents (Fig. S5–S10†). Fig. 3(a) shows the change in the wavenumber of the O–H stretching, Δν(O–H), which is defined as the difference between the wavenumber of the O–H stretching vibration of 5-HMF in the mixtures (extracting solvents and 5-HMF) and that of neat 5-HMF, for different systems as a function of the mole fraction of any given extracting solvent. It was found that the Δν(O–H) values were all positive and increased with the increasing solvent content added, which indicated that with the continuous addition of the extracting solvent, the intermolecular hydrogen bonding interactions among the 5-HMF molecules were gradually broken,25 resulting in the blue-shift of the O–H stretching vibration. Furthermore, the value of Δν(O–H) decreased in the sequence THF > MIBK > SADE > EtOAc > BuOAc > MTBE, suggesting that the capacity of the extracting solvents to destroy the intermolecular hydrogen bonding interactions of 5-HMF itself decreased in this order. Namely, the hydrogen bonding interactions of the extracting solvents with 5-HMF also decreased in this sequence.
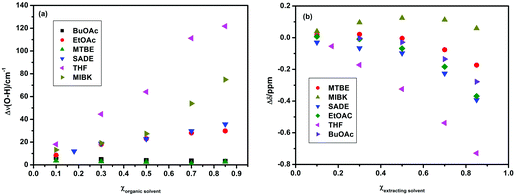 |
| Fig. 3 Variation of Δν(O–H) in the FTIR spectrum (a) and Δδ values in the NMR spectrum (b) for the O–H group of 5-HMF as a function of the mole fraction of the extracting solvent in the mixtures of extracting solvents and 5-HMF. | |
Fig. 3(b) shows the variation in the Δδ values for the proton of the O–H group in the mixtures of extracting solvents and 5-HMF. Here, for the sake of convenience, the difference in the chemical shift between the proton of O–H of 5-HMF in the mixtures and that in neat 5-HMF, (Δδ), was used to analyze the interactions of 5-HMF with the solvents. It was found that the Δδ values were all negative, except in the mixture containing MIBK, with the addition of the extracting solvent, indicating that the addition of the extracting solvent reduced the intermolecular hydrogen bonding interactions of 5-HMF itself. This result supports the conclusion derived from FTIR investigations. On the other hand, breaking or weakening of the X–H⋯Y hydrogen bond would cause a change in the chemical shift of the proton. Therefore, a larger Δδ value suggests a greater ability to reduce the intermolecular hydrogen bonding interactions of 5-HMF. Based on this mechanism, it is clear from Fig. 3(b) that the capability of the extracting solvent to weaken the intermolecular hydrogen bonding interactions of 5-HMF decreased in the sequence THF > MIBK > SADE > EtOAc > BuOAc > MTBE, which is in agreement with the order derived from the FTIR spectral results. Therefore, the order of the capability of extracting solvents to weaken the intermolecular hydrogen bonds of 5-HMF was in line with the sequence of the extraction efficiency. These results indicate that the extracting solvent with a stronger hydrogen bond acceptor ability (a higher β value) has a stronger interaction with 5-HMF (as a hydrogen bond donor), resulting in the higher extraction efficiency, which is more suitable for the extraction of 5-HMF from ILs.
The effect of the anionic structure of the ILs on the separation of 5-HMF
Under the optimized conditions, the extraction experiments of 5-HMF from the ILs with the same cation ([Bmim]+) but different anions ([CH3OSO3]−, [CH3SO3]−, [NO3]−, [BF4]−, and Cl−) were carried out by using THF. The 5-HMF extraction efficiencies of different ILs are shown in Fig. 4. It can be seen that the values decreased in the sequence [Bmim][BF4] (22.3%) > [Bmim][CH3SO3] (19.4%) > [Bmim][CH3OSO3] (17.1%) > [Bmim][NO3] (16.3%) > [Bmim]Cl (15.1%). Interestingly, this sequence is reversed to that of the β values of the ILs.9 These results indicate that the strong hydrogen bonding interactions between the anions of the ILs and 5-HMF result in the difficulty of separation of 5-HMF from the ILs, and the IL that has weaker hydrogen bonding with 5-HMF has a higher extraction efficiency of 5-HMF. Importantly, at 25 °C the single step extraction efficiency of 5-HMF from [Bmim][CH3SO3] by using THF (19.4%) was about 6.5 times that of 5-HMF extracted by MIBK at 80 °C (3%) being reported in the literature.19 In addition, for complete separation of 5-HMF from the ILs, successive runs were conducted. It was found that more than 95% of 5-HMF was separated after ten successive runs (shown in Fig. S11–S15†).
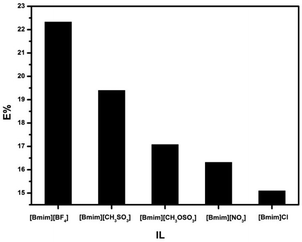 |
| Fig. 4 The extraction efficiency of 5-HMF from the ILs with different anions by using THF at 25 °C. | |
The effect of the cationic structure of the ILs on the separation of 5-HMF
The 5-HMF extraction efficiencies of the ILs with different cations ([Amim][NO3], [Pmim][NO3], [Hemim][NO3], and [Cpmim][NO3]) under the optimized conditions by using THF solvent are shown in Fig. 5. It was found that the extraction efficiency of 5-HMF decreased in the sequence [Hemim][NO3] (44.2%) > [Cpmim][NO3] (34.5%) > [Pmim][NO3] (28.5%) > [Amim][NO3] (20.4%). Notably, the extraction efficiency of 5-HMF from [Hemim][NO3] was up to 44.2% when one step extraction was used. To understand the mechanism of the cationic structure in the separation of 5-HMF, 1H NMR and FTIR spectroscopy were used (Fig. S16–19†). Fig. 6(a) exhibits a change in the O–H stretching vibration, Δν(O–H), of 5-HMF in the mixtures (5-HMF and ILs) relative to that in neat ILs as a function of the IL mole fraction. We found that for a given composition, the Δν(O–H) value of 5-HMF in different mixtures increased in the order [Hemim][NO3] < [Cpmim][NO3] < [Pmim][NO3] < [Amim][NO3], while the interaction strength of the cation with the anion of the ILs increased in the order [Amim][NO3] < [Pmim][NO3] < [Cpmim][NO3] < [Hemim][NO3].26 These results indicate that although the hydrogen bonding interaction between the O–H group of 5-HMF and [NO3]− of the ILs was much stronger than that between the cation and the O–H group, the cation can affect the O–H stretching vibration of 5-HMF by its interaction with the anion.
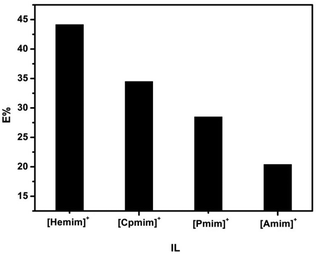 |
| Fig. 5 The extraction efficiency of 5-HMF from the [NO3]−-based ILs with different cations at 25 °C. | |
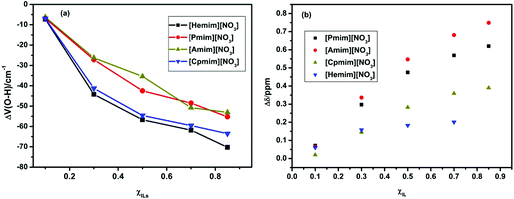 |
| Fig. 6 The change of Δν(O–H) in the FTIR spectrum (a) and Δδ values in the NMR spectrum (b) for the O–H group of 5-HMF as a function of the mole fraction of the ILs in different mixtures of 5-HMF and ILs. | |
Fig. 6(b) shows the change in the Δδ values for the proton of the O–H group in various mixtures of ILs and 5-HMF as a function of the IL mole fraction. It was shown that the Δδ values in these mixtures were all positive and increased with the increase of the IL mole fraction. As mentioned above, the O–H group of 5-HMF can afford a proton to form a hydrogen bond with the anion of the ILs. Such a hydrogen bond formation would lead to a change in the chemical shift of the proton of the O–H group. These results indicate that the intensity of the hydrogen bond formed by the O–H group of 5-HMF with the anion of the ILs was stronger than those formed by 5-HMF itself, thus resulting in a decrease in the electron density for the proton of the O–H group in the IL and 5-HMF mixtures and the downfield chemical shifts. In addition, the Δδ values decreased in the order [Amim][NO3] > [Pmim][NO3] > [Cpmim][NO3] > [Hemim][NO3]. Therefore, the stronger interactions between the cation and anion of the ILs cause the strength of the interaction between the anion and O–H group of 5-HMF to decrease, leading to a smaller change in the Δδ value. This result confirmed the conclusion derived from the FTIR spectral results. Therefore, it is clear that the stronger interaction of the cation with the anion of the ILs reduces the hydrogen bonding interaction between 5-HMF and the anion of the ILs, making the separation of 5-HMF easier.
The optimal IL for the separation of 5-HMF
Based on the above results, it was found that among the studied solvents and ILs, THF was the optimal solvent (Fig. 2), [BF4]− was the optimal anion (Fig. 4), and [Hemim]+ was the optimal cation (Fig. 5). Therefore, we speculate that [Hemim][BF4] is the “best” IL from which 5-HMF can be separated. To verify this deduction, we performed the extraction experiment of 5-HMF from [Hemim][BF4] by using THF at 25 °C. We were surprised to find that the single step extraction efficiency of 5-HMF was 61.0%, which is a record-breaking value so far in this field (the highest value reported in the literature is 15.1%
20). Furthermore, successive runs were conducted to examine the cumulative separation of 5-HMF from the ILs. More than 99% of 5-HMF could be separated after three successive runs (Fig. S20†), and only about 2 h was needed for this separation process. In addition, it should be emphasized that although the anion [BF4]− may be slightly hydrolyzed in water at higher temperature, it is stable in an organic environment at room temperature. Thus, [Hemim][BF4] is preferentially recommended as the solvent in the IL-based biomass conversion to 5-HMF.
Conclusions
In summary, the effects of the anion type, cationic structure and organic solvent hydrogen bond acceptor properties on the separation of 5-HMF from the ILs were analyzed systematically. It was found that an ideal extracting solvent should have a low mutual solubility with ionic liquids and strong hydrogen bond acceptor ability. Among the solvents studied, THF was the best extracting solvent. Furthermore, the anion of the ILs played an important role in the separation of 5-HMF. The weaker the hydrogen bonding interaction of the anion with 5-HMF, the higher the extraction efficiency of 5-HMF from the ILs. The cation also impacted the separation of 5-HMF from the ILs through its interaction with the anion. The stronger interactions between the cation and the anion of the ILs led to a decrease in the interaction strength of the anion with the O–H group of 5-HMF, thus resulting in the increased extraction efficiency of 5-HMF. Under the optimized conditions, the one step extraction efficiency of 5-HMF was up to 61.0%, which is the record-breaking value at this time.
Meanwhile, because of its medium boiling point, THF could readily be recovered from 5-HMF by simple distillation, and then the ILs can be easily recycled. Based on the CHEM21 solvent selection guide, THF is a greener solvent. Therefore, this study represents a breakthrough in the separation of 5-HMF from ILs, and also provides a useful guideline for the selection of an optimal extracting solvent for use in such an important separation process.
Experimental
Materials
5-Hydroxymethylfural (99 wt%), methanol (99.5 wt%), ethanol (99.5 wt%), butanol (99.5 wt%), butyl acetate (BuOAc, 99 wt%), succinic acid diethylester (SADE, 99 wt%), methyl-iso-butylketone (MIBK, 98.5 wt%), methyl-tert-butylether (MTBE, 99.8 wt%), tetrahydrofuran (THF, 99 wt%), and ethyl acetate (EtOAc, 99 wt%) were all purchased from Aladdin Industrial Co. Ltd. 1-Butyl-3-methylimidazolium nitrate ([Bmim][NO3], 98.5 wt%), 1-butyl-3-methylimidazolium methyl sulfonate ([Bmim][CH3OSO3], 99 wt%), 1-butyl-3-methylimidazolium tetrafluoroborate ([Bmim][BF4], 99 wt%) and 1-hydroxyethyl-3-methylimidazolium tetrafluoroborate ([Hemim][BF4], 99 wt%) were all purchased from Green Chemistry and Catalytic Center, Lanzhou Institute of Chemical Physics. 1-Allyl-3-methylimidazolium nitrate ([Amim][NO3], 99 wt%), 1-propyl-3-methylimidazolium nitrate ([Pmim][NO3], 99 wt%), 1-hydroxyethyl-3-methylimidazolium nitrate ([Hemim][NO3], 99 wt%), and 1-nitrile propyl-3-methylimidazolium nitrate ([Cpmim][NO3], 99 wt%) were bought from Shanghai Cheng Jie Chemical Co. Ltd. All these chemicals were used as received and the structures of these ILs are shown in Fig. S21.†
Spectroscopic analysis
Mid-IR spectra were obtained at 25 °C with a PerkinElmer Spectrum 400 FTIR spectrometer (PerkinElmer, USA) that was equipped with a deuterated L-ananine-doped triglycine sulfate (DLaTGS) detector and a Golden Gate single-reflection diamond ATR. Purge gas of dehumidified air was supplied to the instrument to wipe off water vapor. The distinguishability of the instrument was 4 cm−1 and 16 scans were performed for each measurement. The 1H NMR spectra of 5-HMF in the ILs or extracting solvents were obtained at 25 °C using an Avance-400 NMR spectrometer (Bruker, Switzerland) operating at 400.13 MHz. An internal standard tube containing D2O and TMS was used, and the chemical shifts were expressed in parts per million downfield from TMS.
Extraction experiments
Typically, 0.024 g of 5-HMF, 0.5 g of IL, and 1 mL of extracting solvent were added to a glass vial with scale marks and mixed for 40 min to accomplish equilibrium by using a thermostat oscillator (Jinghong Instrument Factory, Shanghai, China). The glass vial was equipped with a water circulating jacket, and the temperature was controlled within ±0.1 °C by using a DC-2006 water thermostat (Shanghai Hengping Instrument Factory, China). After stopping the shaking process, the phase separation quickly occurred. Then, the volumes of the extractive and raffinate phases were recorded on the basis of the scale marks on the wall of the vial, and the samples with specified volumes from the two phases were collected for analysis by using a pipette. The concentration of 5-HMF was determined using an ultraviolet-visible spectrophotometer (Shanghai Lengguang Technology Co. Ltd, China) at a wavelength of 283 nm. The partition coefficient (K) of 5-HMF was defined as the ratio of the equilibrium concentration of 5-HMF in the extracting solvent phase and IL phase and can be calculated using the equation:where Cb and Ct are the equilibrium concentrations (mol L−1) of 5-HMF in the extracting solvent phase and IL phase, respectively.
The extraction efficiency of 5-HMF was computed using the equation
where m is the initial mass of the added 5-HMF and Vb is the volume of the extracting solvent phase.
Conflicts of interest
There are no conflicts to declare.
Acknowledgements
This work was supported by the National Natural Science Foundation of China (No. 21573060 and U1704251), the National Key Research and Development Program of China (No. 2017YFA0403101), the Natural Science Foundation of Henan (No. 202300410220) and the 111 Project (No. D17007).
Notes and references
- P. Anbarasan, Z. C. Baer, S. Sreekumar, E. Gross, J. B. Binder, H. W. Blanch, D. S. Clark and F. D. Toste, Nature, 2012, 491, 235–237 CrossRef CAS PubMed.
- J. B. Binder and R. T. Raines, J. Am. Chem. Soc., 2009, 131, 1979–1985 CrossRef CAS PubMed.
- C. H. Zhou, X. Xia, C. X. Lin, D. S. Tong and J. Beltramini, Chem. Soc. Rev., 2011, 40, 5588–5617 RSC.
- P. Gallezot, Chem. Soc. Rev., 2012, 41, 1538–1558 RSC.
- R. J. van Putten, J. C. van der Waal, E. de Jong, C. B. Rasrendra, H. J. Heeres and J. G. de Vries, Chem. Rev., 2013, 113, 1499–1597 CrossRef CAS PubMed.
- T. Wang, M. W. Nolte and B. H. Shanks, Green Chem., 2014, 16, 548–572 RSC.
- Y. Roman-Leshkov, C. J. Barrett, Z. Y. Liu and J. A. Dumesic, Nature, 2007, 447, 982–985 CrossRef CAS.
- Y. Chen and T. Mu, Green Energy Environ., 2019, 4, 95–115 CrossRef.
- H. Wang, S. Liu, Y. Zhao, H. Zhang and J. Wang, ACS Sustainable Chem. Eng., 2016, 4, 6712–6721 CrossRef CAS.
- H. Zhao, J. E. Holladay, H. Brown and Z. C. Zhang, Science, 2007, 316, 1597–1600 CrossRef CAS PubMed.
- S. Zhang, J. Sun, X. Zhang and J. Wang, Chem. Soc. Rev., 2014, 43, 7838–7869 RSC.
- Z. Zhang, J. Song and B. Han, Chem. Rev., 2017, 117, 6834–6880 CrossRef CAS.
- S. Hu, Z. Zhang, J. Song, Y. Zhou and B. Han, Green Chem., 2009, 11, 1746–1749 RSC.
- T. Stahlberg, M. G. Sorensen and A. Riisager, Green Chem., 2010, 12, 321–325 RSC.
- Z. H. Zhang, Q. Wang, H. B. Xie, W. J. Liu and Z. K. Zhao, ChemSusChem, 2011, 4, 131–138 CrossRef CAS PubMed.
- B. Liu, Z. H. Zhang and Z. K. Zhao, Chem. Eng. J., 2013, 215–216, 517–521 CrossRef CAS.
- M. X. Tan, L. Zhao and Y. G. Zhang, Biomass Bioenergy, 2011, 35, 1367–1370 CrossRef CAS.
- Y. Su, H. M. Brown, X. Huang, X. Zhou, J. E. Amonette and Z. C. Zhang, Appl. Catal., A, 2009, 361, 117–122 CrossRef CAS.
- A. Stark, B. Ondruschka, D. H. Zaitsau and S. P. Verevkin, J. Chem. Eng. Data, 2012, 57, 2985–2991 CrossRef CAS.
- J. Zhou, T. Huang, Y. Zhao, Z. Xia, Z. Xu, S. Jia, J. Wang and Z. C. Zhang, Ind. Eng. Chem. Res., 2015, 54, 7977–7983 CrossRef CAS.
- H. Wang, J. Cui, H. Li, Y. Zhao and J. Wang, J. Mol. Struct., 2019, 1179, 57–64 CrossRef CAS.
- D. Prat, A. Wells, J. Hayler, H. Sneddon, C. R. McElroy, S. Abou-Shehadad and P. J. Dunne, Green Chem., 2016, 18, 288–296 RSC.
- A. Stark, B. Ondruschka, D. H. Zaitsau and S. P. Verevkin, J. Chem. Eng. Data, 2012, 57, 2985–2991 CrossRef CAS.
- M. J. Kamlet, J. M. Abboud, M. H. Abraham and R. W. Taft, J. Org. Chem., 1983, 48, 2877–2991 CrossRef CAS.
- L. Q. Zhang, Z. Xu, Y. Wang and H. Li, J. Phys. Chem. B, 2008, 112, 6411–6419 CrossRef CAS PubMed.
- H. Wang, M. Liu, Y. Zhao, X. Xuan, Y. Zhao and J. Wang, Sci. China: Chem., 2017, 60, 970–978 CrossRef CAS.
Footnote |
† Electronic supplementary information (ESI) available. See DOI: 10.1039/d0gc03557b |
|
This journal is © The Royal Society of Chemistry 2021 |
Click here to see how this site uses Cookies. View our privacy policy here.