DOI:
10.1039/D0QO01238F
(Research Article)
Org. Chem. Front., 2021,
8, 101-105
δ-Regioselective heteroarylation of free alcohols through 1,5-hydrogen-atom transfer†
Received
8th October 2020
, Accepted 11th November 2020
First published on 12th November 2020
Abstract
An efficient silver-catalyzed δ-regioselective C(sp3)–H heteroarylation of free alcohols has been developed. Various alcohols reacted with quinolines, isoquinoline, pyridines, pyrimidine, phthalazine, 4-hydroxyquinazoline, acridine, quinoxaline and pyrazine to give the corresponding C(sp2)–H alkylation products in 31–89% yields. Notably, all types (1°, 2°, and 3°) of δ-C(sp3)–H bonds in the alcohols could be regioselectively activated. This protocol provides a platform to access divergent functionalizations of alcohols and heteroaryls by forming the challenging δ-selective C(sp3)–C(sp2) bond.
Introduction
Alcohols and their derivatives serve as significant building block compounds in chemistry and pharmaceutical science.1 Many groups have developed direct C–O etherification,2 α-oxy C(sp3)–H heteroarylation,3 α-C(sp3)–H alkylation or arylation of alcohols4 and other types of functionalizations of α-oxy C(sp3)–H bonds.5 According to the bond dissociation energy,6 the C–H bonds distal to the hydroxyl group have much lower reactivity than the proximal ones. In 1960, Barton discovered that alkoxyl radicals could lead to 1,5-hydrogen atom transfer (1,5-HAT) to activate the remote C–H bond.7 However, the HAT process triggered by alkoxy radicals is still a formidable challenge, due to the strong dissociation energy of the alcoholic O–H bond (∼105 kcal mol−1) and high oxidation potential of alcoholic O–H bonds.8
Although significant challenges lie ahead,9 functionalizing remote unactivated C(sp3)–H bonds of free aliphatic alcohols has been attractive to chemists in the past few decades. A series of functionalization reactions such as amination,10 oxime cyanation,11 and mono-/di-halogenation12 have been realized on the organic synthesis stage in recent years.
Electron-deficient N-heteroaromatics play an important role in the field of bioactive molecules and drugs.13 Notably, a major breakthrough has been achieved by the Zhu group, who disclosed remote C(sp3)–H heteroarylation of free alcohols using the PhI(OTFA)2 system under visible-light catalysis (Scheme 1A).14 Chen further developed the Ru(bpy)3Cl2/PFBI-OH catalyzed δ-C(sp3)–H heteroarylation of free aliphatic alcohols (Scheme 1B).15
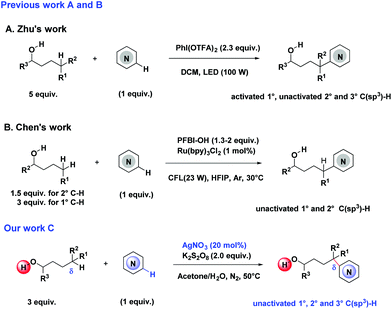 |
| Scheme 1 Remote C(sp3)–H heteroarylation of free alcohols. | |
Herein, we describe a silver-catalyzed remote C(sp3)–H (primary, secondary, and tertiary) heteroarylation of free alcohols (Scheme 1C).
Results and discussion
We initiated our research by exploring the reaction conditions for the δ-selective C(sp3)–H heteroarylation of 4-methyl quinoline (1a) with n-pentanol (2a) (Table 1). After considerable experimentation, we were pleased to obtain the heteroarylated product 3a in 79% yield with AgNO3 as the catalyst, K2S2O8 as the oxidant, and TFA as the acid in acetone/H2O (1 mL/1 mL) under a nitrogen atmosphere at 50 °C for 24 h (Table 1, entry 1). A lower yield was obtained without the application of TFA or using 1.0 equiv. of TFA (Table 1, entries 2 and 3). In order to improve the electrophilicity of 1a, other acids including TfOH, TCA, HCl, and H2SO4 were screened, but the yields showed no significant improvement (Table 1, entry 4). Furthermore, varieties of mixed solvents, such as CH3CN/H2O, DMSO/H2O, DCM/H2O, DMF/H2O and 1,4-dioxane/H2O, were examined, and acetone/H2O was found to be the best solvent (Table 1, entries 5–9). Water was vital to dissolve AgNO3 and K2S2O8 (Table 1, entry 10). Other kinds of silver salts such as Ag2CO3 and AgCOOCF3 gave 3a in moderate yields; AgCl and CuCl2 had no efficiency (Table 1, entry 11). The stoichiometries of AgNO3 and K2S2O8 also had a major influence on this reaction (Table 1, entries 12–15). Further temperature screening showed that 50 °C was the most suitable temperature for the reaction (Table 1, entry 16).
Table 1 Optimization of reaction conditionsa

|
Entry |
Deviation from the standard conditions |
Yieldb (%) |
Reaction conditions: 1a (0.3 mmol), 2a (0.9 mmol), AgNO3 (20 mol%), K2S2O8 (2.0 equiv.), TFA (2.0 equiv.), acetone/H2O (1 mL/1 mL), stirred at 50 °C under N2 (1 atm) for 24 h.
Isolated yields based on 1a.
|
1 |
None |
79 |
2 |
Without TFA |
59 |
3 |
1.0 equiv. of TFA |
67 |
4 |
TfOH, TCA, HCl, or H2SO4 as the acid |
73, 67, trace, 67 |
5 |
CH3CN/H2O as the solvent |
45 |
6 |
DMSO/H2O as the solvent |
49 |
7 |
DCM/H2O as the solvent |
64 |
8 |
DMF/H2O as the solvent |
44 |
9 |
1,4-Dioxane/H2O as the solvent |
47 |
10 |
Acetone as the solvent |
N.R. |
11 |
AgCl, Ag2CO3, AgCOOCF3, or CuCl2 as the catalyst |
N.R., 67, 75, N.R. |
12 |
Without AgNO3 |
N.R. |
13 |
Without K2S2O8 |
N.R. |
14 |
1.5 equiv. of K2S2O8 |
60 |
15 |
10 mol% of AgNO3 |
61 |
16 |
25 °C, 70 °C |
50, 70 |
With the optimized reaction conditions established, we began to investigate the generality of the protocol (Scheme 2). At first, diverse electron-deficient N-heteroaromatics were tested under the standard conditions. Quinolines with para-substituents such as an electron-donating group (Me) or electron-withdrawing groups (Br, Cl) gave the desired alkylation products 3a, 3b, 3c and 3d in 51–79% yields. Pleasingly, the aldehyde group was tolerated in this oxidant system (3e). Quinolines with ortho-substitutions such as Me, OMe, Cl and CO2Et gave 3f, 3g, 3h and 3i in 57–86% yields. Unsubstituted quinoline could react with alcohols, giving a mixture of ortho and para substitution products (3j, o/p = 1
:
1). Isoquinoline reacted with n-pentanol to give a 1-alkylated product (3k). Moreover, 4-CH3 and 4-CN substituted pyridine, 2-chloropyrimidine and phthalazine only afforded mono-functionalization products in moderate yields, along with some unreacted materials (3l, 3m, 3n and 3o). Other N-heteroaromatics such as 4-hydroxyquinazoline and acridine gave a 2-substituted product (3p) and a 9-substituted product (3q), respectively. Additionally, 2-chloroquinoxaline and 2,3,5-trimethylpyrazine could be converted into the corresponding products both in 81% yields (3r, 3s). Unfortunately, when 4-hydroxyquinoline, benzothiazole and benzoxazole were used, we failed to obtain the corresponding Minisci-type products (3t, 3u, and 3v). In general, this protocol indicated a broad scope of heteroaryls.
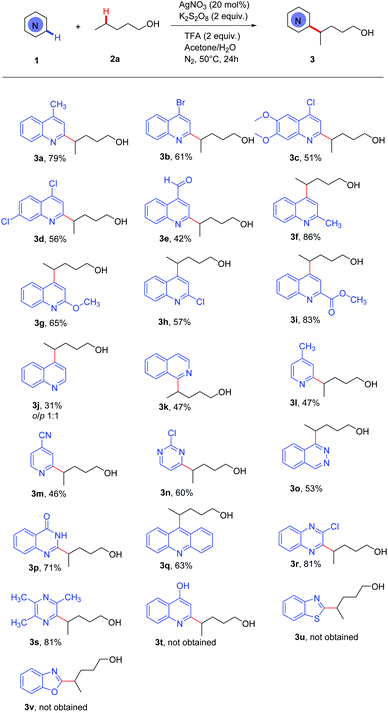 |
| Scheme 2 Scope of N-heteroarenes. Reaction conditions: 1 (0.3 mmol), 2a (0.9 mmol), AgNO3 (20 mol%), K2S2O8 (2.0 equiv.), TFA (2.0 equiv.), acetone/H2O (1 mL/1 mL), stirred at 50 °C under N2 (1 atm) for 24 h, isolated yields. | |
In addition, diverse classes of alcohols were investigated (Scheme 3). In most cases, alcohols reacted with 2-methylquinoline well to afford the Minisci-type products in moderate to excellent yields. The unactivated primary C(sp3)–H bond in 1-butanol was readily transformed, delivering the product 3w in 61% yield. Other simple aliphatic primary alcohols containing secondary δ-C(sp3)–H bonds were suitable substrates, and the corresponding products were obtained in 73–89% yields (3x, 3y, 3z). Notably, the tertiary δ-C(sp3)–H bond with significant steric hindrance could also be regioselectively activated to obtain the product (3aa). Besides, secondary alcohols were applicable to generate alkoxy radicals to obtain the heteroaryl products (3ab, 3ac, 3ad). The reaction with cyclic C(sp3)–H bonds proceeded smoothly (3ae). Remarkably, the 1,5-HAT exclusively occurred even in the presence of the more reactive allylic and benzylic C–H bonds (3af and 3ag), showing excellent regioselective control. 4-Phenyl-1-butanol, containing the benzylic C–H bond, was oxidized to 4-hydroxy-1-phenylbutan-1-one in this oxidation system. Additionally, the substrates containing ester and amide groups did not degrade the activity (3ag and 3ah). The C(sp3)–H bonds adjacent to the oxygen atom were also readily functionalized, giving 3ai in 45% yield. Unfortunately, when tertiary alcohol 2-methylpentan-2-ol was used, only 4-methylquinoline was recovered.
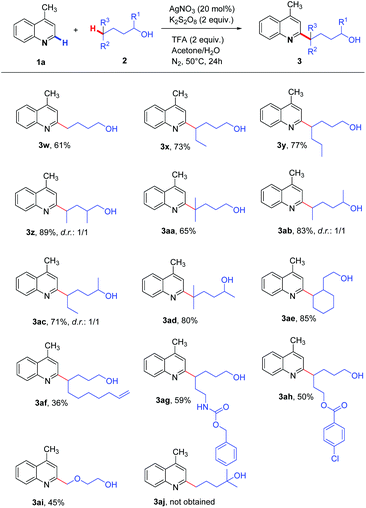 |
| Scheme 3 Scope of alcohols. Reaction conditions: 1a (0.3 mmol), 2 (0.9 mmol), AgNO3 (20 mol%), K2S2O8 (2.0 equiv.), TFA (2.0 equiv.), acetone/H2O (1 mL/1 mL), stirred at 50 °C under N2 (1 atm) for 24 h, isolated yields. | |
Then we explored the reaction efficiency on a larger scale using 1.15 g of 1f, and 3f was isolated in 80% yield (Scheme 4).
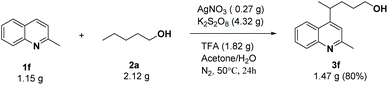 |
| Scheme 4 Scale-up of the reaction. | |
A series of control experiments were conducted to investigate the preliminary mechanism. No desired product 3a was detected when the radical scavenger 2,2,6,6-tetramethyl-1-piperidinyloxy (TEMPO) or 2,6-di-tert-butyl-4-methylphenol (BHT) was added to the reaction mixture under the standard conditions (Scheme 5a and b). Then, we replaced 1-butanol with di-n-butyl ether and discovered that the transformation was entirely inhibited (Scheme 5c). The above experiments indicated that this kind of silver-catalyzed reaction might start from an oxygen radical.
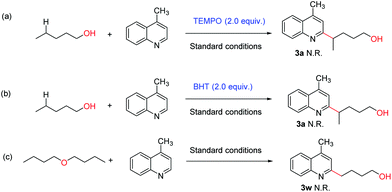 |
| Scheme 5 Control experiment. | |
We thus postulated a plausible mechanism based on the results of our group and others16 (Scheme 6). At first, alcohol 2 coordinated with AgII, which was oxidized from AgI by K2S2O8, to form intermediate 4. Then, intermediate 4 was activated to form alkoxyl radical 5, generating AgI. Subsequently, the N-heteroaromatic salt 6 trapped 7, which was triggered by 5 through 1,5-HAT, generating the radical cation 8. After a sulfate radical anion obtained a hydrogen atom from 8, the alkylated product 3 was ultimately formed.
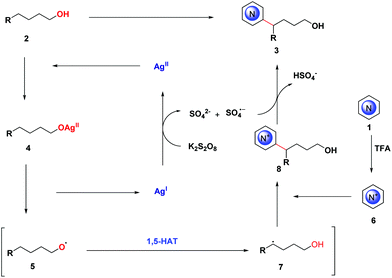 |
| Scheme 6 Postulated mechanism. | |
Conclusions
In summary, a general protocol for direct silver-catalyzed δ-regioselective C(sp3)–H heteroarylation of free alcohols through 1,5-hydrogen atom transfer has been developed. This simple catalytic system is suitable for a diverse range of N-heteroarenes and alcohols under mild reaction conditions. In particular, activated and unactivated (1°, 2°, and 3°) δ-C(sp3)–H bonds of alcohols can be regioselectively converted into heteroarylated adducts. Furthermore, it may be worthwhile to provide a reasonable method for medicinal synthesis in the near future.
Conflicts of interest
There are no conflicts of interest to declare.
Acknowledgements
We are grateful to the Natural Science Foundation of China (No. 21776254) and Natural Science Foundation of Zhejiang Province (No. LQ 20B060006) for financial help.
Notes and references
-
(a) H. T. Luk, C. Mondelli, D. C. Ferre, J. A. Stewart and J. Perez-Ramirez, Status and Prospects in Higher Alcohols Synthesis from Syngas, Chem. Soc. Rev., 2017, 46, 1358–1426 RSC;
(b) T. Hille, T. Irrgang and R. Kempe, Synthesis of meta-Functionalized Pyridines by Selective Dehydrogenative Heterocondensation of β- and γ- Amino Alcohols, Angew. Chem., Int. Ed., 2017, 56, 371–374 CrossRef CAS.
-
(a) J. Zhou, P. Zhou, T. Zhao, Q. Ren and J. Li, (Thio)etherification of Quinoxalinones under Visible-Light Photoredox Catalysis, Adv. Synth. Catal., 2019, 361, 5371–5382 CrossRef CAS;
(b) X. Jiang, L. Yang, Z. Ye, X. Du, L. Fang, Y. Zhu, K. Chen, J. Li and C. Yu, Electrosynthesis of C3 Alkoxylated Quinoxaline-2(1H)-ones through Dehydrogenative C-H/O-H Cross-Coupling, Eur. J. Org. Chem., 2020, 1687–1694 CrossRef CAS.
-
(a) S. Ambala, T. Thatikonda and S. Sharma, Cross-dehydrogenative Coupling of α-C(sp3)–H of Ethers/alkanes with C(sp2)–H of Heteroarenes under Metal-free Conditions, Org. Biomol. Chem., 2015, 13, 11341–11350 RSC;
(b) J. Jin and D. W. MacMillan, Direct α–Arylation of Ethers through the Combination of Photoredox–Mediated C-H Functionalization and the Minisci Reaction, Angew. Chem., Int. Ed., 2015, 54, 1565–1569 CrossRef CAS;
(c) S. Devari and B. A. Shah, Visible Light-Promoted C–H Functionalization of Ethers and Electron-Deficient Arenes, Chem. Commun., 2016, 52, 1490–1493 RSC;
(d) L. Niu, J. Liu, X. A. Liang, S. Wang and A. Lei, Visible Light-Induced Direct α C–H Functionalization of Alcohols, Nat. Commun., 2019, 10, 467 CrossRef.
- S. Y. Zhang, Y. Q. Tu, C. A. Fan, F. M. Zhang and L. Shi, Iron–Catalyzed C(sp3)-C(sp3) Bond Formation through C(sp3)-H Functionalization: A Cross–Coupling Reaction of Alcohols with Alkenes, Angew. Chem., Int. Ed., 2009, 48, 8761–8765 CrossRef CAS.
-
(a) Y. Obora, S. Hatanaka and Y. Ishii, Iridium-Catalyzed Coupling Reaction of Primary Alcohols with 1-Aryl-1-propynes Leading to Secondary Homoallylic Alcohols, Org. Lett., 2009, 11, 3510–3513 CrossRef CAS;
(b) S. Y. Zhang, F. M. Zhang and Y. Q. Tu, Direct sp3 α-C–H Activation and Functionalization of Alcohol and Ether, Chem. Soc. Rev., 2011, 40, 1937–1949 RSC;
(c) J. K. Cheng and T. P. Loh, Copper- and Cobalt-Catalyzed Direct Coupling of sp3 α-Carbon of Alcohols with Alkenes and Hydroperoxides, J. Am. Chem. Soc., 2015, 137, 42–45 CrossRef CAS;
(d) W. Zhang, N. X. Wang, C. B. Bai, Y. J. Wang, X. W. Lan, Y. Xing, Y. H. Li and J. L. Wen, Manganese-Mediated Coupling Reaction of Vinylarenes and Aliphatic Alcohols, Sci. Rep., 2015, 5, 15250 CrossRef;
(e) S. W. Kim, W. Zhang and M. J. Krische, Catalytic Enantioselective Carbonyl Allylation and Propargylation via Alcohol-Mediated Hydrogen Transfer: Merging the Chemistry of Grignard and Sabatier, Acc. Chem. Res., 2017, 50, 2371–2380 CrossRef CAS;
(f) X. Zheng, T. Zhong, L. Zhang, J. Chen, Z. Chen, X. Jiang and C. Yu, Radical–Triggered Cyclization of Methylthio–Substituted Alkynones: Synthesis of Diverse 3-Alkylthiochromones, Eur. J. Org. Chem., 2020, 4534–4541 CrossRef CAS.
- S. J. Blanksby and G. B. Ellison, Bond Dissociation Energies of Organic Molecules, Acc. Chem. Res., 2003, 36, 255–263 CrossRef CAS.
- D. H. R. Barton, J. M. Beaton, L. E. Geller and M. M. Pechet, A New Photochemical Reaction, J. Am. Chem. Soc., 1960, 82, 2640–2641 CrossRef CAS.
- X. S. Xue, P. Ji, B. Zhou and J. P. Cheng, The Essential Role of Bond Energetics in C–H Activation/Functionalization, Chem. Rev., 2017, 117, 8622–8648 CrossRef CAS.
-
(a) J. Robertson, J. Pillai and R. K. Lush, Radical Translocation Reactions in Synthesis, Chem. Soc. Rev., 2001, 30, 94–103 RSC;
(b) T. Newhouse and P. S. Baran, If C-H Bonds Could Talk: Selective C-H Bond Oxidation, Angew. Chem., Int. Ed., 2011, 50, 3362–3374 CrossRef CAS;
(c) J. Yamaguchi, A. D. Yamaguchi and K. Itami, C-H Bond Functionalization: Emerging Synthetic Tools for Natural Products and Pharmaceuticals, Angew. Chem., Int. Ed., 2012, 51, 8960–9009 CrossRef CAS;
(d) X. Q. Hu, J. R. Chen and W. J. Xiao, Controllable Remote C–H Bond Functionalization by Visible–Light Photocatalysis, Angew. Chem., Int. Ed., 2017, 56, 1960–1962 CrossRef CAS.
- A. Hu, J. J. Guo, H. Pan, H. Tang, Z. Gao and Z. Zuo, δ-Selective Functionalization of Alkanols Enabled by Visible-Light-Induced Ligand-to-Metal Charge Transfer, J. Am. Chem. Soc., 2018, 140, 1612–1616 CrossRef CAS.
- Y. Zhu, K. Huang, J. Pan, X. Qiu, X. Luo, Q. Qin, J. Wei, X. Wen, L. Zhang and N. Jiao, Silver-Catalyzed Remote Csp3-H Functionalization of Aliphatic Alcohols, Nat. Commun., 2018, 9, 2625 CrossRef.
- A. N. Herron, D. Liu, G. Xia and J. Q. Yu, δ-C–H Mono- and Dihalogenation of Alcohols, J. Am. Chem. Soc., 2020, 142, 2766–2770 CrossRef CAS.
-
(a) A. Fournet, R. Mahieux, M. A. Fakhfakh, X. Franck, R. Hocquemiller and B. Figadère, Substituted Quinolines Induce Inhibition of Proliferation of HTLV-1 Infected Cells, Bioorg. Med. Chem. Lett., 2003, 13, 891–894 CrossRef CAS;
(b) M. A. J. Duncton, Minisci Reactions: Versatile CH-Functionalizations for Medicinal Chemists, MedChemComm, 2011, 2, 1135–1161 RSC;
(c) Y. Q. Hu, C. Gao, S. Zhang, L. Xu, Z. Xu, L. S. Feng, X. Wu and F. Zhao, Quinoline Hybrids and Their Antiplasmodial and Antimalarial Activities, Eur. J. Med. Chem., 2017, 139, 22–47 CrossRef CAS;
(d) J. Zhou, Z. Li, Z. Sun, Q. Ren, Q. Zhang, H. Li and J. Li, Electrochemically C–H/S–H Oxidative Cross-Coupling between Quinoxalin-2(1H)-ones and Thiols for the Synthesis of 3-Thioquinoxalinones, J. Org. Chem., 2020, 85, 4365–4372 CrossRef CAS;
(e) J. Zhou, Y. Zou, P. Zhou, Z. Chen and J. Li, Copper-Catalyzed Versatile C(sp3)–H Arylation: Synthetic Scope and Regioselectivity Investigations, Org. Chem. Front., 2019, 6, 1594–1598 RSC.
-
(a) X. Wu, H. Zhang, N. Tang, Z. Wu, D. Wang, M. Ji, Y. Xu, M. Wang and C. Zhu, Metal-Free Alcohol-Directed Regioselective Heteroarylation of Remote Unactivated C(sp3)–H Bonds, Nat. Commun., 2018, 9, 3343 CrossRef.
- G. X. Li, X. Hu, G. He and G. Chen, Photoredox-Mediated Remote C(sp3)–H Heteroarylation of Free Alcohols, Chem. Sci., 2019, 10, 688–693 RSC.
-
(a) F. Q. Huang, Y. W. Wang, J. G. Sun, J. Xie, L. W. Qi and B. Zhang, Silver-Mediated Oxidative Trifluoromethylthiolation of Cycloalkanols by C–C Bond Cleavage: a Regioselective Approach to Distally Trifluoromethylthiolated Ketones, RSC Adv., 2016, 6, 52710–52714 RSC;
(b) B. Xu, D. Wang, Y. Hu and Q. Shen, Silver-Catalyzed Ring-Opening Difluoromethylthiolation/trifluoromethylthiolation of Cycloalkanols with PhSO2SCF2H or PhSO2SCF3, Org. Chem. Front., 2018, 5, 1462–1465 RSC.
Footnote |
† Electronic supplementary information (ESI) available: Experimental procedures, characterization data, NMR spectra. See DOI: 10.1039/d0qo01238f |
|
This journal is © the Partner Organisations 2021 |
Click here to see how this site uses Cookies. View our privacy policy here.