DOI:
10.1039/D0RA09910D
(Paper)
RSC Adv., 2021,
11, 11732-11738
Simple and sensitive colorimetric sensors for the selective detection of Cu(II)†
Received
23rd November 2020
, Accepted 8th March 2021
First published on 23rd March 2021
Abstract
A simple, sensitive colorimetric probe for detecting Cu(II) ions with fast response has been established with a detection limit of 2.82 μM. UV-Vis spectroscopy along with metal ion response, selectivity, stoichiometry, competition was investigated. In the presence of copper(II), the UV-Vis spectrum data showed significant changes and the colorimetric detection showed a color change from colorless to yellow. After the selective binding of receptor L with Cu(II), the UV-visible absorption at 355 nm decreased dramatically, a new absorbance band appeared at 398 nm and its intensity enhanced with the increase in the amount of Cu(II). Moreover, it exhibited highly selective and sensitive recognition towards Cu(II) ions in the presence of other cations over the pH range of 7–11. The complex structure was verified by FT-IR spectroscopy, elemental analysis and quantum mechanical calculations using B3LYP/6-31G(d) to illustrate the complex formation between L and Cu(II). According to the Job plot and the quantum mechanical calculations, the stoichiometric ratio for the complex formation was proposed to be 1
:
1.
Introduction
Over the past decades, there has been considerable interest in the development of chemosensors for detecting heavy metal ions as they are harmful to the environment and important biological processes.1–7 As the third abundant element, copper(II) ion plays vital roles in numerous physiological processes. However, either excess or deficient amounts may aggravate the deterioration of vital organs and lead to the progression of complications. When humans and animals are over-exposed to Cu(II)-contaminated water, copper ions tend to accumulate, which is toxic to the environment and ecosystem, and also has an impact on the progress of Alzheimer's disease and Parkinson's disease.8–10 Therefore, numerous methods have been developed for the trace detection of Cu(II) in biological and environmental specimens, such as inductively coupled plasma mass spectrometry (ICP-MS),11,12 atomic absorption spectrometry (AAS),13,14 voltammetry,15,16 and total reflection X-ray fluorimetry (TXRF).17–20 Among the common analytical methods, most of them often require expensive instruments and sophisticated detection systems. At present, fluorescence spectroscopy is a frequently used technique for the detection of copper(II) ions in the biological and environmental media. In many cases, fluorescent sensors have limitations due to fluorescence quenching of numerous metal ions.21–26 Cu(II) also exhibits fluorescence quenching due to its intrinsic paramagnetic nature.27–29 In practical applications, it is necessary to develop a simple, convenient, and low-cost Cu(II) sensor. Therefore, the design and synthesis of colorimetric sensors for the detection of Cu(II) is very valuable.
Chemosensors for the selective detection of Cu(II) generally have structures of chelating metal ions with heteroatoms (nitrogen and sulfur), such as naphthalimide,30–32 naphthalene,33–35 anthracene,36 anthraquinone,37 quinoline,38–41 thiazole,42 and macrocyclic43–45 derivatives. However, many of them are obtained via complex synthesis procedures or require expensive materials. For example, Zhang et al.46 reported that the synthesis of a naphthalimide derivative required six steps. Therefore, developing novel chemosensors for detecting metal ions with synthesis and commercial availability are necessary. In this study, chemosensor L based on a pyridyl-isoindoline-1-one skeleton was easily obtained with good yield using simple and inexpensive starting materials, which manifested highly sensitive and selective colorimetric detection towards Cu(II) with a color change from colorless to yellow in a mixed solvent. The complex skeleton was verified by FT-IR spectroscopy, MALDI-TOF, elemental analysis and quantum mechanical calculations to illuminate the formation of the complex between Cu(II) and L; the stoichiometric ratio of 1
:
1 for complex formation and mechanism for the detection of the Cu2+ ion with L were proposed.
Experimental
All reagents were of analytical grade and used without purification. The inorganic salts of Fe2+, Ag+, Mg2+, Na+, Co2+, Ca2+, Cr3+, Cd2+, Hg2+, Ni2+, Ba2+, Zn2+, Cd2+, and Cu2+ ions and 2-pyridylaldehyde and isoindoline-1,3-dione were purchased from Chemical Reagent Company.
Absorption spectra were recorded on a TU-1901 double beam UV-Vis spectrophotometer. While 1H NMR spectra were obtained on a Bruker Avance 600 MHz NMR. CD3OD and CDCl3 were used as the solvent. FT-IR and elemental analyses were conducted on a Bruker Alpha spectrometer and Elementar Vario EL CUBE, respectively. MALDI-TOF was performed on a Bruker Autoflex speed TOF/TOF mass spectrometer using dithranol (cas: 1143-38-0) as the matrix. Quantum chemistry calculations at the density functional theory of B3LYP were used to fully optimize two molecules, and the calculations were completed in Gaussian09.
Elemental analysis
Anal. calcd for C14H9CuN3O4: C, 48.49; H, 2.62; N, 12.12; found: C, 48.43; H, 2.29; N, 12.05.
Results and discussion
Receptor L was synthesized according to the literature method.47 The synthetic routes of compound L are displayed in Scheme 1. Starting from isoindoline-1,3-dione, the immediate precursor isoindolin-1-one was synthesized with a 80% yield. Upon heating a 1
:
2
:
4 mixture of isoindolin-1-one, 2-pyridylaldehyde and K2CO3 at 110 °C for 24 h in toluene, compound L was obtained in 85% yield and characterized via FT-IR and 1H NMR spectroscopy techniques.
 |
| Scheme 1 Synthesis of compound L. | |
The colorimetric selective sensing ability of receptor L with various cations in the EtOH/H2O (v/v, 4
:
1) mixed solution was determined by UV-Vis absorption spectroscopy (Fig. 1a). It was found that the absorption maximum of L was located at 355 nm in the absence of metal ions. Only after the addition of 2.0 equiv. of Cu2+, the absorption maximum of the complex L–Cu(II) red-shifted to 398 nm; however, other cations such as Co2+, Mg2+, Na+, Ca2+, Cr3+, Ba2+, Fe2+, Zn2+, Co2+, Hg2+, Ni2+, Cd2+, and Ag+ combined with L had no influence on the spectra. The new absorption band was attributed to the metal-induced intramolecular charge transfer from receptor L to the Cu2+ ion.48,49 Compared with the photograph of receptor L with various cations (Fig. 1b), the solution of L with Cu2+ ions caused a rapid and sensitive color change from colorless to yellow, which indicated that the receptor L could be used as a ‘naked-eye’ indicator for copper ions in mixed solutions. In addition, chemosensor L for Cu2+ can strongly quench the fluorescence of a fluorophore by the PET mechanism50 (Fig. 1c).
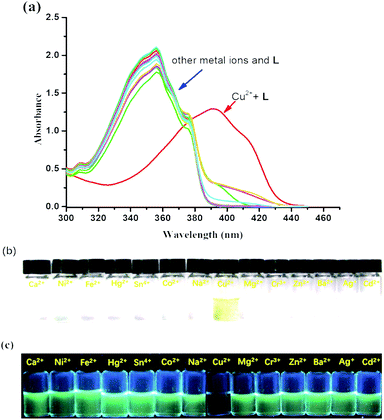 |
| Fig. 1 (a) UV-Vis spectral changes of receptor L (50 μM) after the addition of 2 equiv. of Cu2+ and 5 equiv. of other metal ions in the EtOH/H2O solution (v/v, 4 : 1). (b) The color changes of L (50 mM) upon the addition of various metal ions (5 equiv.) in the EtOH/H2O solution (v/v, 4 : 1). (c) Fluorescent changes of L (50 μM) upon the addition of various metal ions (5 equiv.) in the EtOH/H2O solution (v/v, 4 : 1). | |
The detection limit of L for the analysis of Cu(II) was investigated by UV-Vis absorption spectra of L (5.0 × 10−5 M) in an EtOH/H2O = 4
:
1 solution upon addition Cu2+ at room temperature, as shown in Fig. 2 (Fig. S1 in ESI†). With the addition of Cu2+, the absorption band at 355 nm decreased gradually, while the absorption band at 398 nm increased and reached its maximum at 1 equiv. of Cu2+. The isosbestic points at 370 nm indicated that a single species between the Cu2+ and receptor L was formed, and the maximum absorption peak of the spectrum could be redshifted by 43 nm, and the color changed from colorless to yellow. At 398 nm, the band gap with a molar extinction coefficient of 1.2 × 104 M−1 cm−1 (±10%), is not Cu-based d–d transitions due to the ligand-to-metal charge-transfer (LMCT) mechanism.51 By calculation, the detection limit of L for the analysis of Cu(II) was 2.82 μM with the linear working range from 20 μM to 45 μM, which was determined from the plot of the relative absorbance intensity I398 nm/I355 nm as a function of the concentration of Cu(II) on the basis of 3σ/k according to the literature52,53 (Fig. S2 in ESI†). Compared to other recently reported Cu(II)-sensors, the detection limit of our sensor was higher, as shown in Table S1.†54–60 Although some of the reported sensors provided better detection limits, some proceeded with the “turn-off” mode for the detection of Cu(II) and had inferior selectivity compared to that of our sensor in this study.
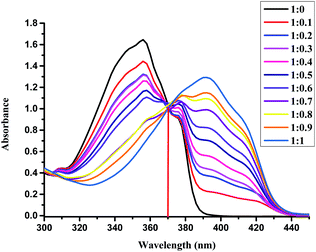 |
| Fig. 2 UV-Vis spectral changes of L (50 μM) upon the addition of Cu2+ (from 0 up to 1 equiv.) in the EtOH/H2O (4 : 1) solution at room temperature. | |
Further study of the features of L–Cu(II) interactions was obtained by 1H NMR experiments in the CD3OD solvent (Fig. 3). By hydrogen spectrum analysis, the chemical shift of the complex L–Cu(II) changed greatly after the addition of copper ions. Compared with 1H NMR before and after the addition of copper ions, the N–H chemical shift at 11.18 ppm became weak, and the signal of C–H also slightly became broader. It is suggested that nitrogen in secondary amides might be involved in the binding of Cu2+, which was consistent with the previous reports.61,62 The stoichiometry of L was obtained by the Job's plot. The results showed that when the molar ratio was 0.5, and the absorbance band of L–Cu(II) complex reached the maximum, which indicated that the stoichiometric ratio of the complexes between L to Cu2+ ions was 1
:
1 (Fig. 4 and S3 in ESI†). In order to research the effects of other metal ions on the complex of Cu(II)–L, competitive experiments were conducted by the addition of copper ions (1 equiv.) to the solution of L with other metal ions (5 equiv. of Na+, Co2+, Mg2+, Ca2+, Ba2+, Cr3+, Fe2+, Co2+, Hg2+, Ni2+, Zn2+, Cd2+, and Ag+). As shown in Fig. 5 (Fig. S4 in ESI†), compared with the addition of Cu(II) to the solution of L, the presence of competing metal ions did not cause any distinct change in UV-Vis absorption spectra. Thus, this displayed the stronger binding capacity of L towards Cu(II), and simple receptor L could be used as a preferential selective colorimetric sensor for Cu(II) in the presence of most interfering ions.
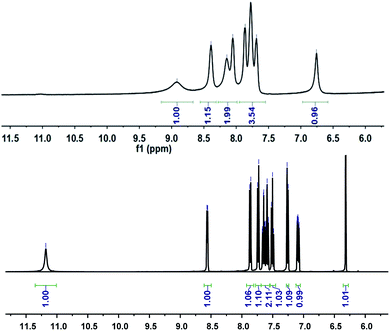 |
| Fig. 3 1H NMR spectrum of L and L with Cu2+ (ratio 1 : 1). | |
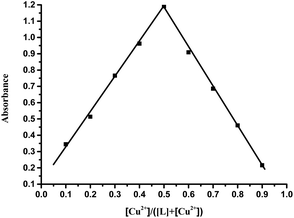 |
| Fig. 4 Job plot for the binding of L with Cu2+. Absorbance at 398 nm was plotted as a function of the molar ratio [Cu2+]/([L] + [Cu2+]). The total concentration of Cu2+ with receptor L was 5.0 × 10−5 M. | |
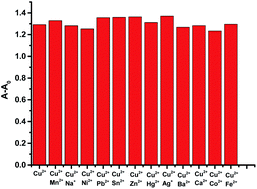 |
| Fig. 5 UV-Vis selectivity–competition study of receptor L with added M(II) salts (5.0 equiv.), followed by the addition of 1.0 equiv. of Cu(II). [L] = 5.0 × 10−5 M, absorbance changes monitored at 398 nm. Relative intensity of L (0.01 mM) in presence of various metal ions in the EtOH/H2O solution, detection wavelength: 398 nm. | |
We next investigated the effects of pH on the response of complex L towards Cu(II) ions. It was clear that the absorption spectrum of the ligand was almost unchanged and the proposed L–Cu(II) sensor could be applied successfully over the pH range 7–11 (Fig. S5 in ESI†). With pH greater than 11, the precipitation of Cu(OH)2 likely occurred in these conditions.63,64 With pH less than 7, due to the protonation process, the absorption peak of the complex L–Cu2+ at 398 nm decreases accordingly. In addition, to determine the repeatability and reproducibility of the L–Cu(II) complex, a reversibility experiment was carried out with the addition of EDTA, which had a stronger binding ability towards Cu2+. It can be seen that with the addition of Cu2+ to L the maximum absorption band appeared at 398 nm, as shown in Fig. 6 (Fig. S6 in ESI†). After the addition of EDTA, the maximum absorption band of the complex appeared at 355 nm, and further addition of Cu2+ restored the location of the maximum absorption peak at 398 nm.
 |
| Fig. 6 The cycle of L–Cu2+ and L + Cu2+ + EDTA. | |
Quantum chemistry calculations at the optimized geometries of the free L and the L–Cu2+ complexes were conducted with the density functional theory of B3LYP to fully optimize the molecule, with a mix of basis sets composed of the split valence double-ζ (DZ) basis set 6-31G(d) for C, O, N, and H atoms, and effective core potential LANL2DZ for the Cu atom. DFT calculations indicated that the HOMO and LUMO of L were delocalized in the whole π-moiety and there was little difference between them (Fig. S7†). The band gap between HOMO (−5.7 eV) and LUMO (−2.0 eV) of L was calculated as 3.70 eV. On the other hand, the HOMO orbital electrons of L–Cu(II) were uniformly distributed in the π-moiety of the molecule and the LUMO orbital electrons of L–Cu(II) mainly delocalized around the nitrogen and oxygen atoms and copper ions. In addition, the band gap between HOMO (−2.98 eV) and LUMO (−2.33 eV) of the L–Cu(II) complex was decreased to 0.65 eV. The smaller energy led to a large-scale spectral redshift, which met the requirement for colorimetric detection from colorless to yellow.
To explain a mechanism for the detection of Cu2+ ions with L, the FT-IR spectroscopy, MALDI-TOF, and elemental analysis were carried out. The FT-IR spectrum analysis showed the N–H stretching band at 3241 cm−1, and C
O stretching band at 1705 cm−1 in compound L. For compound L–Cu(II), it presented the C
N stretching band at 1599 cm−1, and no N–H and C
O stretching bands. Elemental analysis showed that the molecular formula of the compound L–Cu(II) is C14H9CuN3O4, and the molecular formula obtained was complex L–Cu(II) combined with an anionic nitrate. From the analysis of MALDI-TOF, the cluster peaks at 568.838 and 567.829 corresponded to [L + Cu2+ + H+] (calcd = 569.008), [L + Cu2+] (calcd = 568.002), which indicated that the complex L–Cu(II) has a bimolecular chelating center [L–Cu2+–L–Cu2+]. The binding mode of compound L with Cu2+ supported by the quantum chemistry calculation at the optimized geometries is shown in the Fig. 7. Based on the results from IR, elemental analysis, 1H NMR titration studies and quantum chemistry calculation, the proposed binding mode of ligand L with copper ions is through two nitrogen atoms and oxygen atom of carbonyl groups connected to adjacent molecules, as shown in Scheme 2.
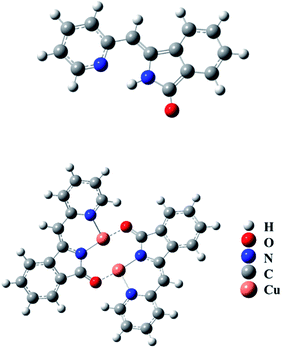 |
| Fig. 7 The structure of L and L–Cu2+ complexes optimized with density functional theory of B3LYP. | |
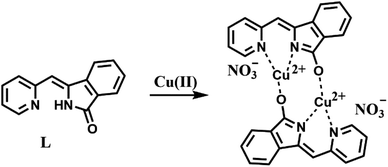 |
| Scheme 2 Proposed mode of complexation between L and Cu2+. | |
Conclusions
In conclusion, we provided a chemosensor sensor L with high yield by using simple starting materials, which manifested highly sensitive and selective colorimetric detection towards Cu(II) with a color change from colorless to yellow. The experimental results displayed the stoichiometric ratio of 1
:
1 for complex formation were consistent with the quantum chemistry calculations.
Conflicts of interest
There are no conflicts to declare.
Acknowledgements
M. F. L. thanks Weifang University for a PhD fellowship and Weifang Sci-tech Development Programme (2019ZJ1034, 2019GX019, 2020GX009).
References
- A. Tigrerosa and J. Portilla, Recent progress in chemosensors based on pyrazole derivatives, RSC Adv., 2020, 10, 19693–19712 RSC.
- P. Grandjean, P. Weihe, R. F. White and F. Debes, Cognitive Performance of Children Prenatally Exposed to ‘‘Safe’’ Levels of Methylmercury, Environ. Res., 1998, 77, 165–172 CrossRef PubMed.
- B. High, D. Bruce and M. M. Richter, Determining copper ions in water using electrochemiluminescence, Anal. Chim. Acta, 2001, 449, 17–22 CrossRef.
- L. Tapia, M. Suazo, C. Hodar, V. Cambiazo and M. Gonzalez, Copper exposure modifies the content and distribution of trace metals in mammalian cultured cells, BioMetals, 2003, 16, 169–174 CrossRef.
- D. J. Waggoner, T. B. Bartnikas and J. D. Gitlin, The role of copper in neurodegenerative disease, Neurobiol. Dis., 1999, 6, 221–230, DOI:10.1006/nbdi.1999.0250.
- H. Jiang, D. Tang, Z. Li, J. Li, H. Liu, Q. Meng, Q. Han and X. Liu, A dual-channel chemosensor based on 8-hydroxyquinoline for fluorescent detection of Hg2+ and colorimetric recognition of Cu2+, Spectrochim. Acta, Part A, 2020, 243, 118784, DOI:10.1016/j.saa.2020.118784.
- H. B. Wang, H. Y. Bai, Y. S. Wang, T. Gan and Y. M. Liu, Highly selective fluorimetric and colorimetric sensing of mercury(II) by exploiting the self-assembly-induced emission of 4-chlorothiophenol capped copper nanoclusters, Microchim. Acta, 2020, 187, 185, DOI:10.1007/s00604-020-4158-2.
- D. Strausak, J. F. Mercer, H. H. Dieter, W. Stremmel and G. Multhaup, Copper in disorders with neurological symptoms: Alzheimer's, Menkes, and Wilson diseases, Brain Res. Bull., 2001, 55, 175–185, DOI:10.1016/S0361-9230(01)00454-3.
- E. Gaggelli, H. Kozlowski, D. Valensin and G. Valensin, Copper homeostasis and neurodegenerative disorders (Alzheimer's, prion, and Parkinson's diseases and amyotrophic lateral sclerosis), Chem. Rev., 2006, 37, 1995–2044, DOI:10.1002/chin.200637260.
- G. Multhaup, A. Schlicksupp, L. Hesse, D. Beher, T. Ruppert, C. L. Masters and K. Beyreuther, The amyloid precursor protein of Alzheimer's disease in the reduction of copper(II) to copper(I), Science, 1996, 271, 1406–1409 CrossRef PubMed.
- J. S. Becker, M. V. Zoriy, C. Pickhardt, N. Palomero-Gallagher and K. Zilles, Imaging of Copper, Zinc, and Other Elements in Thin Section of Human Brain Samples (Hippocampus) by Laser Ablation Inductively Coupled Plasma Mass Spectrometry, Anal. Chem., 2005, 77, 3208–3216, DOI:10.1021/ac040184q.
- J. S. Becker, A. Matusch, C. Depboylu, J. Dobrowolska and M. V. Zoriy, Quantitative imaging of selenium, copper, and zinc in thin sections of biological tissues (slugs-genus arion) measured by laser ablation inductively coupled plasma mass spectrometry, Anal. Chem., 2007, 79, 6047–6080, DOI:10.1021/ac0700528.
- N. Pourreza and R. Hoveizavi, Simultaneous preconcentration of Cu, Fe and Pb as methylthymol blue complexes on naphthalene adsorbent and flame atomic absorption determination, Anal. Chim. Acta, 2005, 549, 124–128, DOI:10.1016/j.aca.2005.06.037.
- A. P. S. Gonzales, M. A. Firmino, C. S. Nomura, F. R. P. Rocha, P. V. Oliveira and I. Gaubeur, Peat as a natural solid-phase for copper preconcentration and determination in a multicommuted flow system coupled to flame atomic absorption spectrometry, Anal. Chim. Acta, 2009, 636, 198–204, DOI:10.1016/j.aca.2009.01.047.
- V. Beni, V. I. Ogrutsov, N. V. Bakunin, D. W. M. Arrigan and M. Hill, Development of a portable electroanalytical system for the stripping voltammetry of metals: determination of copper in acetic acid soil extracts, Anal. Chim. Acta, 2005, 552, 190–200, DOI:10.1016/j.aca.2005.07.058.
- A. A. Ensafi, T. Khayamian, A. Benvidi and E. Mirmomtaz, Simultaneous determination of copper, lead and cadmium by cathodic adsorptive stripping voltammetry using artificial neural network, Anal. Chim. Acta, 2006, 561, 225–232, DOI:10.1016/j.aca.2006.01.015.
- V. K. Shukla, S. Dhara and N. L. Mishra, Total reflection X-ray fluorescence spectrometric determination of ultra-trace uranium in natural water samples using a dispersive liquid–liquid micro-extraction method, J. Anal. At. Spectrom., 2020, 35, 1632–1640, 10.1039/D0JA00039F.
- A. V. Garmay, K. V. Oskolok, O. V. Monogarova and N. V. Alov, Total reflection X-ray fluorescence analysis of highly mineralized water samples using relative intensities and scattered radiation, Spectrochim. Acta, Part B, 2019, 152, 74–83, DOI:10.1016/j.sab.2018.12.011.
- J. Otero-RomanÍ, A. Moreda-Piñeiro, A. Bermejo-Barrera and P. Bermejo-Barrera, Evaluation of commercial C18 cartridges for trace elements solid phase extraction from seawater followed by inductively coupled plasma-optical emission spectrometry determination, Anal. Chim. Acta, 2005, 536, 213–218, DOI:10.1016/j.aca.2004.12.046.
- Y. Liu, P. Liang and L. Guo, Nanometer titanium dioxide immobilized on silica gel as sorbent for preconcentration of metal ions prior to their determination by inductively coupled plasma atomic emission spectrometry, Talanta, 2005, 68, 25–30, DOI:10.1016/j.talanta.2005.04.035.
- A. Mokhir, D. P. Herten and R. Kraemer, Fluorescent sensor for Cu2+ with a tunable emission wavelength, Inorg. Chem., 2005, 44, 5661–5666, DOI:10.1021/ic050362d.
- J. K. Choi, S. H. Kim, J. Y. Yoon, K. H. Lee, R. A. Bartsch and J. S. Kim, A PCT-Based, Pyrene-Armed Calix[4]crown Fluoroionophore, J. Org. Chem., 2006, 71, 8011–8015, DOI:10.1021/jo060981j.
- Y. J. Mei, P. A. Bentley and W. A. Wang, Selective and sensitive chemosensor for Cu2+ based on 8-hydroxyquinoline, Tetrahedron Lett., 2006, 47, 2447–2449, DOI:10.1016/j.tetlet.2006.01.091.
- S. M. Park, M. H. Kim, J. I. Choe, K. T. No and S. K. Chang, Cyclams Bearing Diametrically Disubstituted Pyrenes as Cu2+- and Hg2+-Selective Fluoroionophores, J. Org. Chem., 2007, 72, 3550–3553, DOI:10.1021/jo062516s.
- J. Xie, M. Ménand, S. Maisonneuve and R. Métivier, Synthesis of Bispyrenyl Sugar-Aza-Crown Ethers as New Fluorescent Molecular Sensors for Cu(II), J. Org. Chem., 2007, 72, 5980–5985, DOI:10.1021/jo070315y.
- Y. Q. Weng, F. Yue, Y. R. Zhong and B. H. Ye, A Copper(II) Ion-Selective On–Off-Type Fluoroionophore Based on Zinc Porphyrin Dipyridylamino, Inorg. Chem., 2007, 46, 7749–7755, DOI:10.1021/ic061709v.
- A. W. Varnes, R. B. Dodson and E. L. Wehry, Interactions of transition-metal ions with photoexcited states of flavines. Fluorescence quenching studies, J. Am. Chem. Soc., 1972, 94, 946–950, DOI:10.1021/ja00758a037.
- J. A. Kemlo and T. M. Shepherd, Quenching of excited singlet states by metal ions, Chem. Phys. Lett., 1977, 47, 158–162, DOI:10.1016/0009-2614(77)85329-3.
- R. Bergonzi, L. Fabbrizzi, M. Licchelli and C. Mangano, Molecular switches of fluorescence operating through metal centred redox couples, Coord. Chem. Rev., 1998, 170, 31–46 CrossRef.
- N. Wanichacheva, N. Prapawattanapol, V. L. Sanghiran, K. Grudpan and A. Petsom, Hg2+-induced self assembly of a naphthalimide derivative by selective ‘‘turn-on’’ monomer/excimer emissions, J. Lumin., 2013, 134, 686–690, DOI:10.1016/j.jlumin.2012.07.009.
- H. Cheng and Y. Qian, Synthesis and intramolecular FRET of perylene diimide naphthalimide dendrons, Dyes Pigm., 2015, 112, 317–326, DOI:10.1016/j.dyepig.2014.07.005.
- M. Dodangeh, K. Gharanjig and M. Arami, A novel Ag+ cation sensor based on polyamidoamine dendrimer modified with 1,8-naphthalimide derivatives, Spectrochim. Acta, Part A, 2016, 154, 207–214, DOI:10.1016/j.saa.2015.09.031.
- S. Goswami, D. Sen, N. K. Das, S. Goswami, D. Sen and N. K. Das, A New Highly Selective, Ratiometric and Colorimetric Fluorescence
Sensor for Cu2+ with a Remarkable Red Shift in Absorption and Emission Spectra Based on Internal Charge Transfer, Org. Lett., 2010, 12, 856–859, DOI:10.1021/ol9029066.
- J. Dessingiou, J. K. Khedkar and C. P. Rao, Examining School Principals' Moral Behaviors and Teachers' Organizational Alienation Levels with CHAD Analysis, J. Chem. Sci., 2014, 126, 1135–1141, DOI:10.1007/s12039-014-0648-2.
- M. Q. Wang, K. Li, J. T. Hou, M. Y. Wu, Z. Huang and X. Q. Yu, BINOL-based fluorescent sensor for recognition of Cu(II) and sulfide anion in water, J. Org. Chem., 2012, 77, 8350–8354, DOI:10.1021/jo301196m.
- H. G. Lee, K. B. Kim, G. J. Park, Y. J. Na, H. Y. Jo, S. A. Lee and C. Kim, An anthracene-based fluorescent sensor for sequential detection of zinc and copper ions, Inorg. Chem. Commun., 2014, 39, 61–65, DOI:10.1016/j.inoche.2013.10.049.
- K. Ghosh and D. Kar, Anthraquinone coupled benzothiazole-based receptor for selective sensing of Cu2+, J. Inclusion Phenom. Macrocyclic Chem., 2013, 77, 67–74, DOI:10.1007/s10847-012-0217-6.
- C. Zhou, N. Xiao and Y. Li, Simple quinoline-based “turn-on” fluorescent sensor for imaging copper(II) in living cells, Can. J. Chem., 2014, 92, 1092–1097, DOI:10.1139/cjc-2014-0011.
- C. Zhou, Y. Song, N. Xiao, Y. P. Li and J. Y. Xu, A Novel Highly Sensitive and Selective Fluorescent Sensor for Imaging Copper(II) in Living Cells, J. Fluoresc., 2014, 4, 33614–33618, 10.1039/c4ra03908d.
- L. Tang, P. Zhou, Z. Huang, J. Zhao and M. Cai, New application of 2-(4-N-phenyl-3-thiosemicarbazone)-8-hydroxyquinoline as a sensor for relay recognition of Cu2+ and sulfide in aqueous solution, Bull. Korean Chem. Soc., 2013, 34, 2905–2908, DOI:10.5012/bkcs.2013.34.10.2905.
- J. Jiang, H. Jiang, X. Tang, L. Yang, W. Dou, W. Liu, R. Fang and W. Liu, An efficient sensor for Zn2+ and Cu2+ based on different binding modes, Dalton Trans., 2011, 40, 6367–6370, 10.1039/c1dt10144g.
- X. Wu, Z. Guo, Y. Wu, S. Zhu, T. D. James and W. Zhu, Near-Infrared Colorimetric and Fluorescent Cu2+ Sensors Based on Indoline–Benzothiadiazole Derivatives via Formation of Radical Cations, ACS Appl. Mater. Interfaces, 2013, 5, 12215–12220, DOI:10.1021/am404491f.
- X. Yang, X. Liu, K. Shen, C. Zhu and Y. Cheng, A Chiral Perazamacrocyclic Fluorescent Sensor for Cascade Recognition of Cu(II) and the Unmodified r-Amino Acids in Protic Solutions, Org. Lett., 2011, 13, 3510–3513, DOI:10.1021/ol2013268.
- P. N. Basa and A. G. Sykes, Differential sensing of Zn(II) and Cu(II) via two independent mechanisms, J. Org. Chem., 2012, 77, 8428–8434, DOI:10.1021/jo301193n.
- P. Singh, L. S. Mittal, S. Kumar, G. Bhargava and S. Kumar, Perylene Diimide Appended with 8-Hydroxyquinoline for Ratiometric Detection of Cu2+ Ions and Metal Displacement Driven ‘‘Turn on’’ Cyanide Sensing, J. Fluoresc., 2014, 24, 909–915, DOI:10.1007/s10895-014-1371-6.
- X. H. Zhao, Q. J. Ma, X. B. Zhang, B. Huang, Q. Jiang, J. Zhang, G. L. Shen and R. Q. Yu, A highly selective fluorescent sensor for Cu2+ based on a covalently immobilized naphthalimide derivative, Anal. Sci., 2010, 26, 585–590, DOI:10.2116/analsci.26.585.
- Y. Zhou, Y. Xiao, D. Li, M. Fu and X. Qian, Novel Fluorescent Fluorine-Boron Complexes: Synthesis, Crystal Structure, Photoluminescence, and Electrochemistry Properties, J. Org. Chem., 2008, 73, 1571–1574, DOI:10.1021/jo702265x.
- M. M. AboAly, B. A. El Sayed and A. M. Hassan, Vibrational spectra of benzaldazine and salicylaldazine complexes with Zn(II) and Fe(II) ions, Spectrosc. Lett., 2002, 35, 337, DOI:10.1081/SL-120005670.
- R. Nishiyabu and P. Anzenbacher, 1,3-Indane-based chromogenic calixpyrroles with push-pull chromophores: synthesis and anion sensing, Org. Lett., 2006, 8, 359–362, DOI:10.1021/ol0521782.
- B. Khan, M. R. Shah, D. Ahmed, M. Rabnawaz, I. Anis, S. Afridi, T. Makhmoor and M. N. Tahir, Synthesis, characterization and Cu2+ triggered selective fluorescence quenching of bis-calix[4]arene tetra-triazole macrocycle, J. Hazard. Mater., 2016, 309, 97–106 CrossRef PubMed.
- E. J. Song, J. Kang, G. R. You, G. J. Park, Y. Kim, S. J. Kim and C. Kim, A single molecule that acts as a fluorescence sensor for zinc and cadmium and a colorimetric sensor for cobalt, Dalton Trans., 2013, 42, 15514–15520, 10.1039/c3dt51635k.
- P. Venkatesan and S.-P. Wu, A turn-on fluorescent pyrene-based chemosensor for Cu(II) with live cell application, RSC Adv., 2015, 5, 42591–42596 RSC.
- B. Zhang, H. Liu, F. Wu, G. F. Hao, Y. Chen, C. Tan, Y. Tan and Y. Jiang, A dual-response quinoline-based fluorescent sensor for the detection of copper(II) and Iron(III) ions in aqueous medium, Sens. Actuators, B, 2017, 243, 765–774 CrossRef.
- A. Ganguly, S. Ghosh, S. Kar and N. Guchhait, Selective fluorescence sensing of Cu(II) and Zn(II) using a simple Schiff base ligand: naked eye detection and elucidation of photoinduced electron transfer (PET) mechanism, Spectrochim. Acta, Part A, 2015, 143, 72–80 CrossRef PubMed.
- H. Lia, X. Suna, T. Zheng, Z. Xu, Y. Song and X. Gua, Coumarin-based multifunctional chemosensor for arginine/lysine and Cu2+/Al3+ ions and its Cu2+ complex as colorimetric and fluorescent sensor for biothiols, Sens. Actuators, B, 2019, 279, 400–409, DOI:10.1016/j.snb.2018.10.017.
- Q. Dai, C. Gao, Y. Liu, H. Liu, B. Xiao, C. Chen, J. Chen, Z. Yuan and Y. Jiang, Highly sensitive and selective “naked eye” sensing of Cu(II) by a novel acridine-based sensor both in aqueous solution and on the test kit, Tetrahedron, 2018, 74, 6459–6464, DOI:10.1016/j.tet.2018.09.027.
- R. Hong, W. Ping, L. Fei, J. Li and L. Dawei, Visual colorimetric and fluorescence turn-on probe for Cu(II) ion based on coordination and catalyzed oxidative cyclization of ortho amino azobenzene, Inorg. Chim. Acta, 2019, 487, 234–239, DOI:10.1016/j.ica.2018.12.023.
- N. Mergua and V. K. Gupta, Novel colorimetric detection probe for copper(II) ions based on a Schiff base, Sens. Actuators, B, 2015, 210, 408–417, DOI:10.1016/j.snb.2014.12.130.
- Y. B. Wagh, A. Kuwar, S. K. Sahoo, J. Galluccic and D. S. Dalal, Highly selective fluorimetric sensor for Cu2+ and Hg2+ using a benzothiazole-based receptor in semi-aqueous media and molecular docking studies, RSC Adv., 2015, 5, 45528–45534, 10.1039/C5RA03146J.
- S. J. Ranee, G. Sivaraman, A. M. Pushpalatha and S. Muthusubramanian, Quinoline based sensors for bivalent copper ions in living cells, Sens. Actuators, B, 2018, 255, 630–637, DOI:10.1016/j.snb.2017.08.111.
- H. Seo, M. An, B. Y. Kim, J. H. Choi, A. Helal and H. S. Kim, Highly selective fluorescent probe for sequential recognition of copper(II) and iodide ions, Tetrahedron, 2017, 73, 4684–4691, DOI:10.1016/j.tet.2017.06.034.
- X. Zhu, Q. Lin, J. C. Lou, T. T. Lu, Y. M. Zhang and T. B. Wei, Colorimetric probes designed to provide high sensitivity and single selectivity for CN- in aqueous solution, New J. Chem., 2015, 39, 7206–7210, 10.1039/c5nj01158b.
- L. M. Berreau, S. Mahapatra, J. A. Halfen, V. G. Young and W. B. Tolman, Independent Synthesis and Structural Characterization of a Mononuclear Copper-Hydroxide Complex Previously
Assigned as a Copper-Superoxide Species, Inorg. Chem., 1996, 35, 6339–6342 CrossRef.
- J. J. Lee, Y. W. Choi, G. R. You, S. Y. Lee and C. Kim, A phthalazine-based two-in-one chromogenic receptor for detecting Co(2+) and Cu(2+) in an aqueous environment, Dalton Trans., 2015, 44, 13305–13312 RSC.
Footnote |
† Electronic supplementary information (ESI) available. See DOI: 10.1039/d0ra09910d |
|
This journal is © The Royal Society of Chemistry 2021 |
Click here to see how this site uses Cookies. View our privacy policy here.