DOI:
10.1039/D0RA10513A
(Paper)
RSC Adv., 2021,
11, 13530-13536
Selective sorption of PAHs from TX100 solution by resin SP850: effects of TX100 concentrations and PAHs solubility†
Received
14th December 2020
, Accepted 2nd April 2021
First published on 12th April 2021
Abstract
Recycling of washing effluent by selective sorption using resins is a feasible method to lower the operation costs of surfactant enhanced remediation (SER). In this study, correlations capable of predicting the selective sorption removal of polycyclic aromatic hydrocarbons (PAHs) by resin SP850 from TX100 solution to recycle washing effluent in SER were developed. A negative relationship of sorption coefficients (log
Kf) of PAHs by resin SP850 with TX100 initial concentrations (log
C0,TX100) and water solubilities (log
Sw) of PAHs was observed, which indicated that solubility enhancement of PAHs in TX100 micelles was responsible for the decreasing of the selective sorption. Freundlich exponential coefficients (1/n) of PAHs were relatively constant (0.775 ± 0.012), suggesting that the sorption of PAHs by SP850 in the presence of surfactant is a surface adsorption process. The modified selectivity parameter (S*), having a relationship with log
C0,TX100 and PAHs log
Sw as well, could be employed to evaluate the efficiency of the selective sorption process and select the optimal TX100 concentration in washing effluents. For example, at the given SP850 dose of 1.0 g L−1, the optimal TX100 concentrations (CopTX100) for naphthalene, acenaphthene, phenanthrene, pyrene, anthracene and benzanthracene were about 4200, 7100, 8000, 10
000, 18
000 and 19
500 mg L−1, respectively, having a negative relationship with their log
Sw. Moreover, the CopTX100 was independent of the solid-to-solution ratio of SP850 and TX100 solution containing PAHs. These correlations would be helpful for the application of SER in contaminated soils by giving a method to quantitatively predict the selective sorption behaviors of PAHs by SP850 from TX100 solution, especially for the CopTX100, using the Sw of organic compounds and surfactant concentrations.
Introduction
Surfactant enhanced remediation (SER) has been suggested as a promising technology in removing hydrophobic organic compounds (HOCs) such as polycyclic aromatic hydrocarbons (PAHs) from contaminated soils and groundwater.1–8 However, the practical implementation of SER in contaminated soils is limited due to the increased operation costs produced by the usage of large volumes of surfactant solutions during the soil washing process and the disposal of washing effluents.9–12 Washing effluents containing high concentrations of surfactants and HOCs were obtained from soil washing by separation using centrifugation or precipitation to remove soil solids.
Sorption treatment has been regarded as a promising separation and purification method.13–16 Recycling of washing effluents by selectively sorption separating of HOCs from surfactant solutions and remaining surfactant in solutions is an economical and effective strategy to reduce the operation costs of SER.17–22 In our previous study,23 we found that resin SP850, with higher percent removal for phenanthrene from surfactant solutions and easy regeneration, was a better sorbent than activated carbon and organo-bentonite for the selective separation of phenanthrene from surfactant solutions and the recycling of washing effluents. For example, at 5000 mg L−1 TX100 solution, the removal percentage of phenanthrene from TX100 solution by 1.0 g L−1 SP850 was 82.8%, higher than that by activated carbon (65.9%) and organo-bentonite (63.3%). Moreover, a modified selectivity parameter (S*), having a parabolic relationship with surfactant equilibrium concentrations, was suggested to evaluate the efficiency of the selective sorption process for phenanthrene removal and to calculate the optimal surfactant washing concentration in washing effluents.23 However, the application of S* in predicting the selective sorption efficiency for other HOCs except for phenanthrene from surfactant solution should also be examined.
Surfactant concentrations used in SER and solubilization capabilities of surfactant for HOCs are commonly varied for HOCs and largely dependent of their water solubility (Sw).24–27 For example, the molar solubilization ratios (MSR), the number of moles of compound solubilized per number of moles of micellized surfactant, of phenanthrene, naphthalene, pyrene and fluoranthene in TX100 solution were 0.111, 0.338, 0.0352 (ref. 25) and 0.092 (ref. 28), respectively. The method to calculate the optimal surfactant washing concentration for HOCs in SER using S* values should be established. Therefore, the role of surfactant concentrations and chemical solubility on the selective sorption of six PAHs by SP850 from TX100 solution were investigated in this study to develop the correlations of S* with surfactant concentrations and chemical Sw. The developed correlations could be employed to quantitatively predict an optimal surfactant concentration in washing effluents for PAHs removal by selective sorption using SP850 from surfactant solutions. Six PAHs, including naphthalene, phenanthrene, pyrene, acenaphthene, anthracene and benzanthracene, which span several orders of magnitude in Sw and commonly investigated in previous studies regarding SER technology4,10,25,29,30 were examined here. TX100 employed here because it is a typical soil washing reagent due to its great abilities in enhancing solubility of HOCs.26,31–34
Experimental
Chemicals and resin
Naphthalene (>99%) was purchased from Acros Organics Co. Phenanthrene (>98%) and pyrene (>97%) were purchased from Fluka Chemistry Co. Acenaphthene (>98%), anthracene (>99.5%) and benzanthracene (>98%) were purchased from Aladdin Chemistry Co. Selected properties of these PAHs are listed in Table 1. Nonionic surfactant, TX100 (C8H17C6H4O(OCH2CH2)9.5H), with purity of 98%, was purchased from Sigma Chemical Co. Critical micelle concentration (CMC) of TX100 was 167 mg L−1.34
Table 1 Selected properties of PAHsa
Compounds |
Formula |
MW |
Sw (mg L−1) |
log Kow (25 °C) |
λmax |
Sw and log Kow reported by Yaws.35 |
Naphthalene |
C10H8 |
128 |
31.7 |
3.30 |
219 |
Acenaphthene |
C12H10 |
154 |
3.8 |
3.92 |
226 |
Phenanthrene |
C14H10 |
178 |
1.29 |
4.46 |
250 |
Pyrene |
C16H10 |
202 |
0.135 |
4.88 |
334 |
Anthracene |
C14H10 |
178 |
0.045 |
4.45 |
254 |
Benzanthracene |
C18H12 |
228 |
0.011 |
5.90 |
277 |
Resin SP850, a styrene macroporous resin, was purchased from Rohm and Haas Company (Philadelphia, PA, USA). Before usage, the resin was extracted with ethanol at a solid/solution ratio of 1 g/5 mL for three times to remove the possible impurities, then washed by deionized water and dried in a vacuum oven at 50 °C. The Brunaer–Emmett–Teller (BET) surface area and total pore volume of SP850 obtained from N2 adsorption–desorption isotherms were 953 m2 g−1 and 1.65 cm3 g−1, respectively.
Sorption experiments
Isotherms of selected PAHs by SP850 from TX100 solution were obtained through performing a batch equilibration technique at 25 ± 1 °C, as described in our previous paper.23 SP850 was mixed with 20 mL of aqueous solutions containing TX100 and individual PAHs. Solid-to-solution ratios (m
:
v) of SP850 and solutions were controlled to achieve more than 20% removal of PAHs and TX100 by SP850. PAHs was dissolved in methanol as stock solution, and then diluted to different initial concentrations (ranged from 0.1 mg L−1 to 100 mg L−1) with the TX100 solution to simulate the soil washing effluent. The used TX100 initial concentrations in this study were 1500, 2000, 3000, 5000, 8000 and 10
000 mg L−1, respectively, all greater than 2CMC.1,23 The volume fraction of PAHs methanol in TX100 solution was controlled less than 2% to avoid the cosolvent effects. The mixtures in the vials were shaken at 150 rpm for 2 hours to reach sorption equilibrium. Then, SP850 was separated from aqueous solution by standing vials for 20 min. Equilibrium concentrations of TX100 and PAHs in the aqueous phase were determined by the reverse phase HPLC (Shimadzu, LC-20A series, XDB-C18, φ4.6 × 150 mm) with a UV-spector at a flow rate of 1 mL min−1 using mobile phase containing 90% methanol and 10% water. The UV wavelength of 224 nm is used for TX100 analysis. The UV wavelengths (λmax) for naphthalene, acenaphthene, phenanthrene, pyrene, anthracene and benzanthracene are listed in Table 1. Experiments uncertainties evaluated using PAHs and TX100 solutions without resin were less than 4% of the initial PAHs and TX100 concentrations. Therefore, the sorbed amounts of PAHs and TX100 by SP850 were calculated directly by the mass difference in initial and equilibrium solutions.
Isotherm fitting and data analysis
Freundlich model (eqn (1)), a commonly empirical formula used to fit the isotherms of organic compounds by adsorbents in the presence of surfactant,20,36,37 was employed in this study to fit the isotherms of PAHs from TX100 solution by SP850.where qe (mg g−1) is the equilibrium adsorbed concentration; Ce (mg L−1) is the equilibrium aqueous concentration; Kf [(mg g−1)/(mg L−1)1/n] is Freundlich affinity coefficient; 1/n is the Freundlich exponential coefficient.
The feasibility and efficiency of the selective sorption process for HOCs removal from surfactant solutions were evaluated by the modified selectivity parameter (S*, in eqn (2))
|
S* = (log Kf)/(Kf,TX100)
| (2) |
where
Kf [(mg g
−1)/(mg L
−1)
1/n] is the sorption coefficient of PAHs by resin from surfactant solutions, which obtained from the Freundlich model fitted isotherms of PAHs by SP850 from TX100 solution.
Kf,TX100 (L g
−1) is the sorption coefficient of TX100 by resin. In our previous study,
23 the distribution coefficient (
Kd) was used to calculate the
S* values.
Kf and
Kd are both the ratio of sorption amounts of PAHs on SP850 to equilibrium concentrations of PAHs in surfactant solutions. However,
Kd values would change with the variation of PAHs concentrations when the isotherms of PAHs were nonlinear. While the
Kf values would keep constant and were independent of the concentrations of PAHs,
i.e.,
Kf values could normalize the sorption ratio per unit mass when the equilibrium concentrations of solutions reached the unit value. Therefore,
Kf had a wider application scope than
Kd and was used in this study to calculate the
S* values. Higher
S* value indicates more feasibility and better efficiency for the removing HOCs and the recovering surfactant solution by selection sorption. Standard deviation (SD) between experimental data and the correlations was calculated using
eqn (3),
|
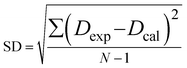 | (3) |
where
Dexp and
Dcal are data determined from experiment and calculation, respectively;
N is the number of data points.
Results and discussion
Isotherms of PAHs, including naphthalene, acenaphthene, phenanthrene, pyrene, anthracene and benzanthracene, by SP850 from TX100 solutions were nonlinear and fitted well by Freundlich model (Fig. S1 and Table S1†), as indicated by correlation coefficient values (R2) close to 1 and the significance of F test (P < 0.001) (Table S1†). The fitted parameters (i.e., Kf and 1/n) of Freundlich model for isotherms of PAHs by SP850 from TX100 solution are listed in Table S1.† Negative relationships of PAHs sorption coefficients (log
Kf) by SP850 with TX100 equilibrium concentrations (log
Ce,TX100) were observed (Fig. 1a and Table S2†), indicating that higher TX100 equilibrium concentrations will decrease the sorption of PAHs by SP850. These negative relationships should be attributed to the solubility enhancement of PAHs into TX100 micelles in solution, as has been suggested widely in the previous studies.25,29,38–40 For example, the apparent solute soil-water distribution coefficients decreased with increasing Ce,TX100 at Ce,TX100 greater than 2CMC,10,39 which was consistent with the above observation. At Ce,TX100 > 2CMC, sorption of TX100 on SP850 reached a saturation value (788 mg g−1).23 Therefore, no more PAHs can be competitived by the adsorbed TX100 micelles. However, more TX100 micelles formed in water once more TX100 were added, which can as an effective partitioning medium to decrease PAHs sorption by solubility enhancement. These negative relationships were independent of the solid-to-solution ratio of resin SP850 and TX100 solution containing PAHs. For example, at given SP850 doses of 0.5, 1.5, 2.0 and 2.5 g L−1, the phenanthrene sorption coefficients by SP850 from TX100 solution reported in our previous study,23 were followed into the observed negatively relationship (Fig. 1b).
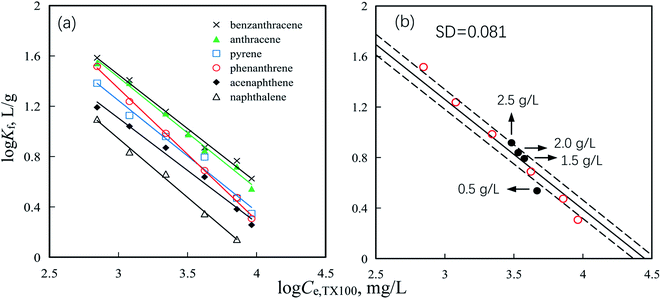 |
| Fig. 1 Correlations of PAHs sorption coefficient (log Kf) by SP850 with TX100 equilibrium concentrations (log Ce,TX100) (a) and that of phenanthrene at various SP850 dose (b). Solid lines are the fitted lines. Dashed lines indicate the SD values from the fit line. Data of phenanthrene at 0.5, 1.5, 2.0 and 2.5 g L−1 SP850 were from ref. 23. | |
At a given TX100 initial concentration, PAHs sorption coefficient (log
Kf) was also negatively correlated with PAHs solubility (eqn (4), Fig. 2 and Table S3†).
|
log Kf = α × log Sw + β
| (4) |
where
α and
β are the slope and intercept, respectively. The observed negative relationship between log
Kf and log
Sw was in agreement with the previous observations, for example, the sorption coefficients of PAHs by soils from TX100 solution at a given surfactant concentration correlated inversely with
Sw of organic compounds
41 and decreased with the increasing
Sw of pesticides.
42 Organic compounds with larger
Sw have higher molar solubilization ratio (MSR) in TX100 solution
3,25,43 to partition into the surfactant micelles in solution, and thus have lower sorption coefficients by SP850. For example, the sorption coefficient of naphthalene with water solubilities of 31.7 mg L
−1 by SP850 at TX100 solution with initial concentration of 5000 mg L
−1 is 2.22 L g
−1, lower than that of pyrene (6.26 L g
−1) having lower water solubility of 0.135 mg L
−1 (Table S1
†). The
α values in
eqn (4) obtained by plotting log
Kf against log
Sw are almost constant (Table S3
†) with a mean value of −0.154 ± 0.030 (
Fig. 3a) and independent on the TX100 concentrations. The absolute values of
α for resin SP850 are smaller than that for Ca-montmorillonite (0.277 ± 0.060),
42 implying that the negative influence of
Sw on PAHs sorption (
i.e., solubility enhancement effect) was less significant for SP850 than that by Ca-montmorillonite. Intercepts of
eqn (4) (
β, L g
−1) were the sorption coefficients of PAHs by SP850 (log
Kf) from TX100 with chemical solubilities closing to 1. A negatively relationship between
β (data listed in Table S3
†) and log
Ce,TX100 was observed (
Fig. 3b and
eqn (5)), further indicated the accuracy of the negative relationships of log
Kf and log
Ce,TX100 (
Fig. 1a and Table S2
†). In our previous study,
23 a positively linear relationship between log
Ce,TX100 and TX100 initial concentrations (log
C0,TX100) was observed at
Ce,TX100 higher than 2CMC (
eqn (6)), where the sorption of TX100 on SP850 reached a saturated value of 788 mg g
−1. Combining
eqn (4)–(6), therefore, the relationship of PAHs sorption coefficient (log
Kf) by SP850 with TX100 initial concentrations (log
C0,TX100) and PAHs solubilities (log
Sw) could be established (
eqn (7)), which could be used to quantitatively estimate the sorption of organic compounds by SP850 from TX100 solution. The smaller absolute coefficient of log
Sw (0.145) than that of log
C0,TX100 (1.20) in
eqn (7), implying that the negative effect of
Sw on
Kf is much weaker than that of
C0,TX100. The calculated log
Kf (log
Kfcal) values of 6 PAHs using
eqn (7), are well agreed with the experimental log
Kf values (log
Kfexp) (Fig. S2
†), which derived from Freundlich model fitted isotherms of PAHs by SP850 from TX100 solution.
|
β = −0.927(±0.032)log Ce,TX100 + 3.99(±0.11), R2 = 0.995, F = 822, P < 0.001, N = 6
| (5) |
|
log Ce,TX100 = 1.33(±0.06)log C0,TX100 − 1.33(±0.20), R2 = 0.992, F = 588, P < 0.001, N = 7
| (6) |
|
log Kf = −1.20(±0.04)log C0,TX100 − 0.145(±0.011)log Sw + 5.12(±0.16), R2 = 0.964, F = 450, P < 0.001, N = 37
| (7) |
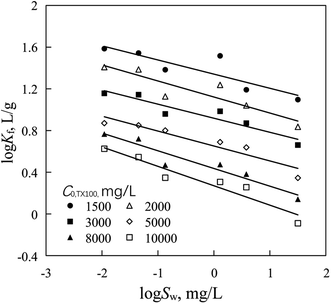 |
| Fig. 2 Correlations of PAHs sorption coefficient (log Kf) with water solubility (log Sw) of PAHs at given TX100 initial concentrations (C0,TX100). | |
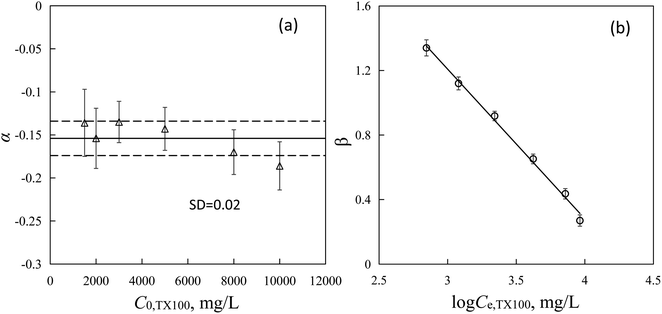 |
| Fig. 3 Values of α with TX100 initial concentrations (a) and linear relationship of β values with TX100 equilibrium concentrations (Ce,TX100) (b). Dashed lines indicate the SD value from the reference line. | |
Freundlich model fitted 1/n is a parameter to describe the non-linearity of isotherms.20,36 For SP850, 1/n values of 6 PAHs by SP850 at given TX100 initial concentrations were relatively constant, with a mean value of 0.775 ± 0.012 (Fig. S3†), which was smaller than that of organo-layered double hydroxide (organo-LDH with 1/n ≈ 1.0)20 and organo-bentonite with 1/n ≈ 1.0.10 This indicating that the sorption of PAHs by SP850 in the presence of surfactant is a surface adsorption process, while that by the organo-LDH and organo-bentonite is a partition-dominated process.
The modified selectivity parameter (S*), i.e., the ratio of log
Kf to Kf,TX100, was suggested to evaluate the efficiency of the selective sorption process and optimize the surfactant concentration for SER.23 Kf,TX100, the ratio of the adsorbed amount of TX100 on SP850 to Ce,TX100, has a negative relationship with Ce,TX100 at Ce,surf > 2CMC (eqn (8)), where the sorption amount of TX100 reached the saturation.23 According to eqn (2) and (6)–(8), S* was related to log
C0,TX100 and log
Sw of PAHs. Moreover, the concentrations of surfactants and the properties of PAHs could both affect the selective sorption of PAHs by adsorbents from surfactant solutions,9–11 i.e., log
C0,TX100 and log
Sw of PAHs are the predominant factors accounting for the selective sorption of PAHs by SP850 from TX100 solution. Therefore, multiple linear regression can be conducted between S* and these two parameters to establish a novel relationship of S* with log
C0,TX100 and log
Sw of PAHs (eqn (9)). The calculated S* values
using eqn (9) are in agreement well with the experimental S* values
(Fig. 4), suggesting the feasibility of eqn (9) for S* predicting. Compared to the relationship of S* with Ce,TX100 observed in the previous study,23 by replacing Ce,TX100 with C0,TX100 and adding a new parameter, log
Sw, this novel relationship is more sufficient and convenient to quantitative predict the selective sorption behaviors of PAHs by resin SP850 from TX100 solution and guide the application of SER in contaminated soil.
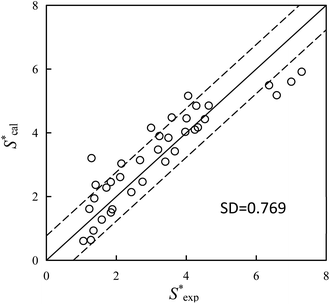 |
| Fig. 4 Calculated S* values using eqn (9) versus the experimental S* values . Solid lines is the reference line, y = x, indicating that the values equal to the values. Dashed lines indicate the SD values from the reference lines. | |
By selective sorption removal of PAHs from washing effluents using resin SP850, washing effluents containing surfactants can be reused to wash contaminated soil to lower the operation costs of SER. Therefore, for soil washing, the used surfactant concentrations in SER should be high enough to form large quantitative micelles for solubility enhancement of HOCs and decrease the sorption loss of surfactant in soil.1,34,41 However, the higher surfactant concentrations would lead to higher spend and lower removal percentages of HOCs by adsorbents.10,41,42 Thus, selecting a suitable surfactant concentration is important for the application of SER technology. To select the optimal TX100 concentrations (CopTX100) for PAHs in SER, the relationships between S* and Ce,TX100, observed for phenanthrene,23 were also observed for other PAHs (Fig. 5a). Values of S* for PAHs increased firstly, and then decreased with increasing Ce,TX100 (Fig. 5a). Solid lines represent S* values fitted by the parabolic equation, i.e., S* = ACe,TX1002 + BCe,TX100, established in our previous study,23 can well describe the increasing and the followed decreasing of S* with increasing Ce,TX100 (Fig. 5a and Table S4†). A and B are the coefficients of the quadratic and primary terms of the equation, respectively. For the parabolic equation, there was a maximum S* value, which could be obtained at Ce,TX100 = −B/(2A), implying that Ce,TX100 = −B/(2A) could be employed to calculate the CopTX100 in washing effluents for the application of SER. For example, at the given SP850 dose of 1.0 g L−1, the CopTX100 for naphthalene, acenaphthene, phenanthrene, pyrene, anthracene and benzanthracene were about 4200, 7100, 8000, 10
000, 18
000 and 19
500 mg L−1, respectively (Fig. 5a and Table S4†). Moreover, a negative relationship of log
CopTX100 with log
Sw of PAHs was observed (Fig. 5b and eqn (10)), which could be also attributed to the higher MSR of PAHs with larger Sw in TX100 solution.25,43 With eqn (10), one can directly calculate the optimal TX100 concentrations in washing effluents from the Sw of organic compounds. In addition, the optimal TX100 concentrations were independent of the solid-to-solution ratio of resin SP850 and TX100 solution containing PAHs. For example, at given SP850 doses of 0.5, 1.0, 1.5 and 2.0 g L−1, the similar parabolic relationships of S* for phenanthrene by SP850 with Ce,TX100 were obtained (Fig. S4† and eqn (11)), suggesting that the relationship of S* with Ce,TX100 was irrelevant to the SP850 dose. Therefore, at various SP850 doses, the optimal TX100 concentrations for the selective removal of phenanthrene by SP850 were all 7767 mg L−1 (Fig. S4†). The negative relationships of log
Kf ∼ Ce,TX100 and Kf,TX100 ∼ Ce,TX100 were both independent of the solid-to-solution ratio of resin SP850 and TX100 solution containing PAHs at Ce,surf > 2CMC (Fig. 1b and eqn (8)) which could result in this phenomenon.
|
Kf,TX100 = 788(±7) × Ce,TX100−1.00(±0.00), R2 = 0.999, F = 2.42 × 106, P < 0.001, N = 8
| (8) |
|
S* = −1.49(±1.98)(log C0,TX100)2 + 15.0(±14.2)log C0,TX100 − 0.693(±0.112)log Sw − 31.6(±25.4), R2 = 0.805, F = 70.1, P < 0.001, N = 37
| (9) |
|
log CopTX100 = −0.182(±0.029)log Sw + 3.94(±0.04), R2 = 0.908, F = 39.3, P = 0.003, N = 6
| (10) |
|
S* = −4.59(±0.21) × 10−8 Ce,TX1002 + 7.13(±0.32) × 10−4 Ce,TX100 + 1.22(±0.10), R2 = 0.948, F = 2.69 × 103, P < 0.001, N = 28
| (11) |
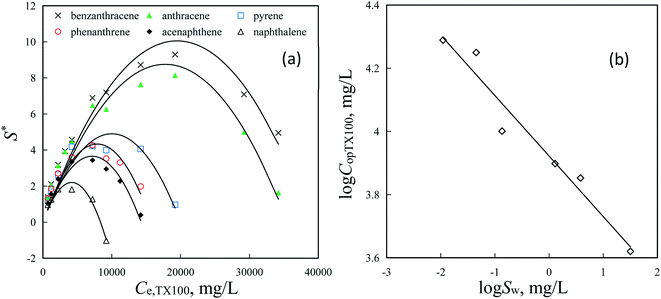 |
| Fig. 5 Correlations between the modified selectivity parameters (S*) of PAHs by SP850 (1.0 g L−1) and TX100 equilibrium concentrations (Ce,TX100) (a) and the correlation of the optimal TX100 washing concentrations (log CopTX100) with water solubility (log Sw) of PAHs (b). Solid lines in plots (a) and (b) are fitted by the Parabolic equation and linear equation, respectively. | |
Conclusions
Effects of TX100 concentrations and PAHs solubilities on the selective sorption of PAHs by resin SP850 from TX100 solution were investigated in this study. A negative relationship of sorption coefficients (log
Kf) of PAHs, including naphthalene, acenaphthene, phenanthrene, pyrene, anthracene and benzanthracene, by resin SP850 with TX100 initial concentrations (log
C0,TX100) and water solubilities (log
Sw) of PAHs was developed, which could be attributed to the solubility enhancement of PAHs in TX100 micelles. The modified selectivity parameter (S*), having a relationship with log
C0,TX100 and log
Sw of PAHs as well. Compared to the relationship of S* with just TX100 equilibrium concentrations (Ce,TX100) observed in the previous study,23 by replacing Ce,TX100 with C0,TX100 and adding a new parameter, log
Sw, this novel relationship is more sufficient and convenient to quantitative predict the selective removal of PAHs to recycle washing effluent in SER and gives new insights into the S* values. Moreover, S* values, having a parabolic equation with Ce,TX100, can be employed to evaluate the optimal TX100 concentration (log
CopTX100) in washing effluents for PAHs in SER. For example, at the given SP850 dose of 1.0 g L−1, the CopTX100 for naphthalene, acenaphthene, phenanthrene, pyrene, anthracene and benzanthracene were about 4200, 7100, 8000, 10
000, 18
000 and 19
500 mg L−1, respectively. The optimal TX100 concentrations for the selective removal of PAHs by SP850 had a negative relationship with log
Sw of PAHs and were independent of the solid-to-solution ratio of SP850 and TX100 solution containing PAHs. These established correlations can be employed to quantitatively estimate the selective sorption behaviors of PAHs by SP850 from TX100 solution, especially for the CopTX100, using the log
Sw of organic compounds and TX100 concentrations for recycling TX100 solution in SER. In addition, more experiments such as selective sorption of HOCs from washing effluents containing mixture surfactants by resins are in urgent needed to be established for more feasible applications of resins and the modified selectivity parameter.
Author contributions
Yaxiong Zeng: methodology, investigation, formal analysis, writing – original draft. Ming Zhang: writing – review & editing. Daohui Lin: conceptualization. Kun Yang: conceptualization, supervision, writing – review & editing, funding acquisition.
Conflicts of interest
There are no conflicts to declare.
Acknowledgements
This work was supported partly by the National Key Research and Development Program of China (2017YFA0207001), the National Natural Science Foundation of China (21621005, 21777138), and the Key Research and Development Program of Zhejiang Province (2019C03105 and 2020C03011).
Notes and references
- C. C. West and J. H. Harwell, Environ. Sci. Technol., 1992, 26, 2324–2330 CrossRef CAS.
- C. N. Mulligan, R. N. Yong and B. F. Gibbs, Eng. Geol., 2001, 60, 371–380 CrossRef.
- S. Paria, Adv. Colloid Interface Sci., 2008, 138, 24–58 CrossRef CAS PubMed.
- S. Kuppusamy, P. Thavamani, K. Venkateswarlu, Y. B. Lee, R. Naidu and M. Megharaj, Chemosphere, 2017, 168, 944–968 CrossRef CAS PubMed.
- M. Cheng, G. M. Zeng, D. L. Huang, C. P. Yang, C. Lai, C. Zhang and Y. Liu, Chem. Eng. J., 2017, 314, 98–113 CrossRef CAS.
- X. X. Bai, Y. Wang, X. Zheng, K. M. Zhu, A. H. Long, X. G. Wu and H. Zhang, Chem. Eng. J., 2019, 369, 1014–1023 CrossRef CAS.
- F. A. Bezza and E. M. N. Chirwa, Chem. Eng. J., 2017, 309, 563–576 CrossRef CAS.
- S. Lamichhane, K. C. Bal Krishna and R. Sarukkalige, J. Environ. Manage., 2017, 199, 46–61 CrossRef CAS PubMed.
- C. K. Ahn, S. H. Woo and J. M. Park, Chem. Eng. J., 2010, 158, 115–119 CrossRef CAS.
- W. J. Zhou, X. H. Wang, C. P. Chen and L. Z. Zhu, Chem. Eng. J., 2013, 233, 251–257 CrossRef CAS.
- H. L. Li, R. H. Qu, C. Li, W. L. Guo, X. M. Han, F. He, Y. B. Ma and B. S. Xing, Bioresour. Technol., 2014, 163, 193–198 CrossRef CAS PubMed.
- X. Zheng, H. Lin, Y. F. Tao and H. Zhang, Chemosphere, 2018, 208, 951–959 CrossRef CAS PubMed.
- M. Kumar, A. K. Singh and M. Sikandar, Heliyon, 2020, 6, e03321 CrossRef PubMed.
- M. Kumar, A. K. Singh and M. Sikandar, Appl. Water Sci., 2018, 8, 225 CrossRef.
- F. Schuricht, E. S. Borovinskaya and W. Reschetilowski, J. Environ. Sci., 2017, 54, 160–170 CrossRef CAS PubMed.
- A. A. Siyal, M. R. Shamsuddin, A. Low and N. E. Rabat, J. Environ. Manage., 2020, 254, 109797 CrossRef CAS PubMed.
- D. A. Sabatini, R. C. Knox and J. H. Harwell, Environ. Res. Brief., 1996, EPA/625/R-95-005 Search PubMed.
- C. Trellu, E. Mousset, Y. Pechaud, D. Huguenot, E. D. van Hullebusch, G. Esposito and M. A. Oturan, J. Hazard. Mater., 2016, 306, 149–174 CrossRef CAS PubMed.
- F. Gharibzadeh, R. R. Kalantary, S. Nasseri, A. Esrafili and A. Azari, Sep. Purif. Technol., 2016, 168, 248–256 CrossRef CAS.
- M. Zhang, C. Zhao, J. Y. Li, L. H. Xu, F. Wei, D. Y. Hou, B. Sarkar and Y. S. Ok, J. Hazard. Mater., 2019, 373, 678–686 CrossRef CAS PubMed.
- C. K. Ahn, S. H. Woo and J. M. Park, Chem. Eng. J., 2010, 158, 115–119 CrossRef CAS.
- J. Z. Wan, L. N. Chai, X. H. Lu, Y. S. Lin and S. T. Zhang, J. Hazard. Mater., 2011, 189, 458–464 CrossRef CAS PubMed.
- Y. X. Zeng, M. Zhang, D. H. Lin and K. Yang, Chem. Eng. J., 2020, 388, 124191 CrossRef CAS.
- K. U. Din, M. Shafi, P. A. Bhat and A. A. Dar, J. Hazard. Mater., 2009, 167, 575–581 CrossRef PubMed.
- D. A. Edwards, R. G. Luthy and Z. B. Liu, Environ. Sci. Technol., 1991, 25, 127–133 CrossRef CAS.
- X. J. Yang, G. N. Lu, R. Wang, Y. Y. Xie, C. L. Guo, X. Y. Yi and Z. Dan, Chem. Eng. J., 2015, 274, 84–93 CrossRef CAS.
- X. H. Mao, R. Jiang, W. Xiao and J. G. Yu, J. Hazard. Mater., 2015, 285, 419–435 CrossRef CAS PubMed.
- D. J. L. Prak and P. H. Pritchard, Water Res., 2002, 36, 3463–3472 CrossRef CAS PubMed.
- D. A. Edwards, Z. Liu and R. G. Luthy, J. Environ. Eng., 1994, 120, 5–22 CrossRef CAS.
- L. Z. Zhu and W. J. Zhou, Environ. Pollut., 2008, 152, 130–137 CrossRef CAS PubMed.
- J. H. Harwell, D. A. Sabatini and R. C. Knox, Colloids Surf., A, 1999, 151, 255–268 CrossRef CAS.
- J. J. Deitsch and J. A. Smith, Environ. Sci. Technol., 1995, 29, 1069–1080 CrossRef CAS PubMed.
- K. Yang, L. Z. Zhu and B. W. Zhao, J. Colloid Interface Sci., 2005, 291, 59–66 CrossRef CAS PubMed.
- K. Yang, L. Z. Zhu and B. S. Xing, Environ. Sci. Technol., 2006, 40, 4274–4280 CrossRef CAS PubMed.
- C. L. Yaws, Chemical Properties Handbook, McGraw-Hill, Beijing, 1999 Search PubMed.
- C. K. Ahn, Y. M. Kim, S. H. Woo and J. M. Park, J. Hazard. Mater., 2008, 154, 153–160 CrossRef CAS PubMed.
- J. F. Liu, J. J. Chen, L. Jiang and X. Yin, J. Ind. Eng. Chem., 2014, 20, 616–623 CrossRef CAS.
- D. E. Kile and C. T. Chiou, Environ. Sci. Technol., 1989, 23, 832–838 CrossRef CAS.
- S. Sun, W. P. Inskeep and S. A. Boyd, Environ. Sci. Technol., 1995, 29, 903–913 CrossRef CAS PubMed.
- J. F. Liu, Y. S. Zhang, X. J. Sun and W. L. Hu, Environ. Earth Sci., 2016, 75, 1453 CrossRef.
- W. J. Zhou and L. Z. Zhu, Chemosphere, 2005, 60, 1237–1245 CrossRef CAS.
- J. F. Lee, P. M. Liao, C. C. Kuo, H. T. Yang and C. T. Chiou, J. Colloid Interface Sci., 2000, 22, 445–452 CrossRef PubMed.
- L. Z. Zhu and S. L. Feng, Chemosphere, 2003, 53, 459–467 CrossRef CAS PubMed.
Footnote |
† Electronic supplementary information (ESI) available: Supplementary figures and tables. See DOI: 10.1039/d0ra10513a |
|
This journal is © The Royal Society of Chemistry 2021 |
Click here to see how this site uses Cookies. View our privacy policy here.