DOI:
10.1039/D0RA10693C
(Paper)
RSC Adv., 2021,
11, 5427-5431
Cu-catalyzed cyanomethylation of imines and α,β-alkenes with acetonitrile and its derivatives†
Received
21st December 2020
, Accepted 19th January 2021
First published on 28th January 2021
Abstract
We describe copper-catalyzed cyanomethylation of imines and α,β-alkenes with a methylnitrile source and provide an efficient route to synthesize arylacrylonitriles and β,γ-unsaturated nitriles. This method tolerates aliphatic and aromatic alkenes substituted with a variety of functional groups such as F, Cl, Br, Me, OMe, tert-Bu, NO2, NH2 and CO2H with good to excellent yields (69–98%). These systems consist of inexpensive, simple copper catalyst and acetonitrile with its derivatives (α-bromo/α-iodo-acetonitrile) and are highly applicable in the industrial production of acrylonitriles.
Introduction and importance
Acrylonitrile and cyanomethyl are versatile functional units found in many dyes, herbicides, agrochemicals, pharmaceuticals, and natural products.1 For example, β,γ-unsaturated nitriles are found in natural products such as alkanenitriles, β-amino nitriles, nitrilosids (Scheme 1).2 The biologically active ruxolitinib, alkylnitrile and acrylonitrile containing entacapone are also shown in Scheme 1.2 These β,γ-unsaturated nitriles and alkenyl nitriles are also key structural units as antifungal agents and vitamin D receptor.3 Besides, the cyano group serves as a valuable intermediate for transformation into aldehydes, amines, amides, tetrazoles, and carboxyl derivatives.2 A lot of approaches for the synthesis of β,γ-unsaturated nitriles have been progressed in recent decades.4 However, the cyanation of allyl substrates containing leaving groups such as carbonate, or ester alcohol, halide, acetate, phosphate, are frequently used in the transformation into β,γ-unsaturated nitriles.4a–f Our many efforts have been paid attention in developing non-toxic and slow-releasing cyano-methyl reagents like alkyl nitriles, especially acetonitrile. However, due to its high pKa value [pKa (CH3CN) = 31.3 in DMSO], relatively difficult to be used as a nucleophile. The catalytic C–H bond activation of acetonitrile by transition metals has rarely been explored in last decades.2b A few strategies has been reported for cyano-methylation by using acetonitrile for various substrates such as phenazines, 2,2,6,6 tetramethylpipyridine, C2-quaternary indolin-3-ones, cycloalkene, simple arenes, aryl-ketone, diarylethenes, azoles, aldehydesaliphatic amides, allylic alcohols, diazonium salts, arylacrylamides, alkenes, 1,3-dicarbonyls, benzaldehyde and coumarins substrates.5
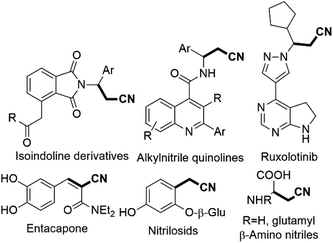 |
| Scheme 1 The natural with biologically active alkylnitriles and acrylonitriles. | |
Consequently, the reactivity of imines have been rarely explored for chiral cyanomethyl product by transfer of hydrogen atom.6 However, synthesis of phenylacrylonitriles from imines not yet explored so for (Scheme 2).7 A number of pharmaceutical reagents contain α,β-unsaturated cyanide moiety such as entacapone and rilpvirine, which can be used as anti-Parkinson's and anti-HIV agents.2
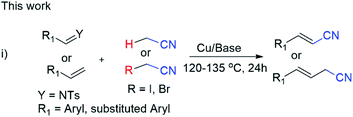 |
| Scheme 2 Our Cu-catalyzed cyanomethylation of aromatic imines and styrenes. | |
Results and discussion
For this copper-catalyzed cyanomethylation of aromatic imines with green MeCN solvent, we used (E)-N-benzylidene-4-methylbenzenesulfonamide (1a) as a model substrate (Table 1). The compound 1a was treated with Cu(OAc)2 (20 mol%) and HOAc (1.0 eq.) under N2 atmosphere at 135 °C, which gave cyano-methylated product (2a) in 10% yield (Table 1, entry 1). Moreover, the amination occurs and 4-methylbenzenesulfonamide obtained as directing auxiliary (3a) with low yield (11%) and decomposition of remaining substrate into complex mixture (Table 1, entry 1). For further week acid screening such as HCO2H and alcohols (t-BuOH, i-PrOH) were elaborated low to mild yields (10–39%) (Table 1, entries 2–4). However, strong acid (HCl) unable to produce desired product (Table 1, entry 5). To further explore the reaction parameters, a variety of boronsted bases such as KOBut, NaOBut, LiOBut and Cs2CO3 were screened. However, these bases are not suitable for reaction and gave the product (2a) in lower to medium yields (25–51%) (Table 1, entries 6–9). Importantly, Cu(OAc)2 evaluated 98% yield of phenylacrylonitrile (2a) with more than 99% of directing auxiliary (4-methylbenzenesulfonamide) in the absence of additive and base or acid (Table 1, entry 10). For further Cu catalyst optimization, a wide variety of Cu(II) catalysts such as Cu(OTf)2, Cu(ClO4)2, Cu(C2H5O2)2, CuCl2, and Cu(I) catalysts (CuI, CuBr, CuCl) were screened (Table 1, entries 11–17).
Table 1 Optimization of conditions for imine using CH3CN.a,b

|
Entry |
[Acid/base] |
[Cu(X)n] |
[Cu quant.] |
Yield 2ab (%) |
Yield 3ab (%) |
Conditions: 1a (0.2 mmol), Cu(X)n (Cu-catalysts), acid/base (1.0 eq.), N2, 135 °C, CH3CN (1.2 mL), 24 h. Isolated yield. n.d; not determined. |
1 |
HOAc |
Cu(OAc)2 |
20 mol% |
10 |
11 |
2 |
HCO2H |
Cu(OAc)2 |
20 mol% |
10 |
36 |
3 |
t-BuOH |
Cu(OAc)2 |
20 mol% |
46 |
51 |
4 |
i-PrOH |
Cu(OAc)2 |
20 mol% |
39 |
55 |
5 |
HCl |
Cu(OAc)2 |
20 mol% |
0 |
n.d |
6 |
KOtBu |
Cu(OAc)2 |
20 mol% |
51 |
60 |
7 |
NaOtBu |
Cu(OAc)2 |
20 mol% |
41 |
53 |
8 |
LiOtBu |
Cu(OAc)2 |
20 mol% |
25 |
40 |
9 |
Cs2CO3 |
Cu(OAc)2 |
20 mol% |
28 |
49 |
10 |
None |
Cu(OAc)2 |
20 mol% |
98 |
>99 |
11 |
None |
Cu(OTf)2 |
20 mol% |
31 |
47 |
12 |
None |
Cu(ClO4)2 |
20 mol% |
59 |
71 |
13 |
None |
Cu(C2H5O2)2 |
20 mol% |
68 |
79 |
14 |
None |
CuCl2 |
20 mol% |
79 |
81 |
15 |
None |
CuI |
20 mol% |
63 |
88 |
16 |
None |
CuBr |
20 mol% |
58 |
80 |
17 |
None |
CuCl |
20 mol% |
46 |
59 |
18 |
None |
Cu(OAc)2 |
5 mol% |
62 |
74 |
19 |
None |
Cu(OAc)2 |
10 mol% |
75 |
83 |
20 |
None |
Cu(OAc)2 |
15 mol% |
88 |
91 |
21 |
None |
Cu(OAc)2 |
25 mol% |
97 |
99 |
22 |
None |
Cu(OAc)2 |
30 mol% |
98 |
99 |
To our delight, these Cu(II) catalysts have good reactivity, which gave the corresponding alkenyl cyanated product (2a) in 31% to 79% yield (Table 1, entries 11–14). Moreover, copper(I) halides (I, Br and Cl) catalytic system such as CuI, CuBr and CuCl elaborated cyanomethyl products in 63%, 58% and 46% (Table 1, entries 15–17) respectively. Gratifyingly, all these copper catalysts have worse reactivity than the commercially abundant Cu(OAc)2 which produced good yield of phenylacrylonitrile (2a) product. In this context, a various quantities of Cu(OAc)2 such as 5 mol%, 10 mol%, 15 mol%, 25 mol% and 30 mol% were examined to find best quantity of Cu(OAc)2 as catalyst (Table 1, entries 18–22). Notably, the yields of desired product (2a) dramatically varied when using 5 mol%, 10 mol%, 15 mol%, 25 mol% and 30 mol% of Cu(OAc)2 (Table 1, entries 18–22). For example, 62% to 98% yields were obtained by using wide range of quantities for the Cu(OAc)2, instead of 20 mol% (Table 1, entries 18–22). After optimization, we elaborate the scope of substrate by varying the substituent on the N-benzylidene-4-methylbenzenesulfonamide (Scheme 3). A variety of N-benzylidene-4-methylbenzenesulfonamide (1a–1d) with electron-donating group such as Me, OMe, tert-butyl at para position of benzene ring afforded the corresponding desired products (2a–2d) in 75% to 98% yields (Scheme 3).
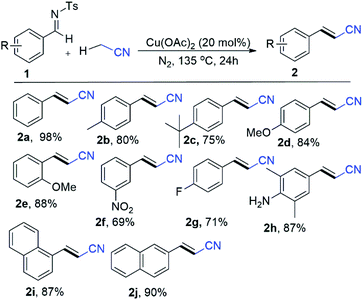 |
| Scheme 3 Substrate scope for imines using CH3CN.a,b aConditions: 1 (0.2 mmol), Cu(OAc)2 (20 mol%), N2, 135 °C, CH3CN (1.2 mL), 24 h. bIsolated yield. | |
In this context, electron-rich methoxy substituent at ortho position of benzene ring such as (E)-N-(2-methoxybenzylidene)-4-methylbenzenesulfonamide (1e) efficiently evaluated the corresponding product (2e) in 88% yields (Scheme 3). Gratifyingly, scope of substrate extended to electron sensitive electron functional groups such as nitro (1f), fluoro (1g) and free amino (1h) groups were attached to the benzene of N-benzylidene-4-methylbenzenesulfonamide, which worked well and formed aryl-alkenyl cyanated products (1f–1h) in 69%, 71% and 87% yields (Scheme 3) respectively. Delightfully, we used the 4-methyl-N-(naphthalen-1-ylmethylene)benzenesulfonamide (1i) and 2-napthalene 4-methyl-N-(naphthalen-2-ylmethylene)benzenesulfonamide (1j) for the Cu-catalyzed cyanomethylation and obtained excellent yields (87% for 1i and 90% for 1j) (Scheme 3). Additionally, our optimization shows that the reaction system was significantly improved with CuCl as a catalyst with K2CO3 as base at 120 °C for styrene derivatives as a substrate with α-haloacetonitriles (α-bromo/α-iodo-acetonitrile) as cyanomethyl source (Scheme 4). In order to elaborate the scope of substrate, a variety of styrenes were examined to get the variety of β,γ-unsaturated nitriles products (Scheme 4). The electron donating substituted styrenes such as p-1-methyl-4-vinylbenzene (4a), 1-methoxy-4-vinylbenzene (4b), 1-(tert-butyl)-4-vinylbenzene (4c), 1-methoxy-2-vinylbenzene (4d) and 4-vinyl-1,1′-biphenyl (4e) were allowed 73% to 90% yields of β,γ-unsaturated cyanated products (5a–5e) (Scheme 4). Gratifyingly, prop-1-en-2-ylbenzene (4f) underwent into desired 4-phenylpent-3-enenitrile (5f) products with 69% yields and (1
:
1) E/Z (Scheme 4). Moreover, by using α-iodo-acetonitrile as cross coupling partner of the (1-bromovinyl)benzene (4g) to form 4-bromo-4-phenylbut-3-enenitrile (5g) in 70% yield (Scheme 4). To our surprise, 2-vinylbenzoic acid (4h) gave the corresponding product (E)-2-(3-cyanoprop-1-en-1-yl)benzoic acid (5h) product with high yield (71%) (Scheme 4). Remarkably, the reaction with 1,1-diphenylethylene (4i) worked well and afforded the target product (5i) with 94% yield (Scheme 4). Delightfully, when one non-fused ring (ethene-1,1-diyldibenzene) was installed with electron donating substituent methyl (4j), and electron withdrawing substituents (bromo and chloro) for β,γ-unsaturated products (5j, 5k, 5l) in good to excellent yields (81–88%) with (1
:
0.17 and 1
:
0.1) E/Z respectively. Additionally, both non-fused rings of ethene-1,1-diyldibenzene installed with electron donating substituent methyl (4m), methoxy (4n), and electron withdrawing substituents bromo (4o) and chloro (4p) have low impact on the reaction efficiency, resulting β,γ-unsaturated products (5m to 5p) with good to excellent yields (78–88%). Moreover, the product 3-(10,11-dihydro-5H-dibenzo[a,d][7]annulen-5-ylidene)propanenitrile (5q), mainly found in the biological active compounds, could synthesize in our reaction system by allowing cyanomethyl functionalization through a cross-coupling of 5-methylene-10,11-dihydro-5H-dibenzo[a,d][7]annulene (4q) and α-bromo-acetonitrile with 94% yield (Scheme 4).
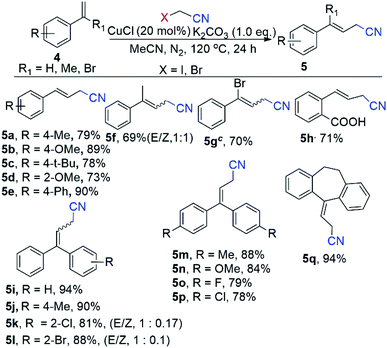 |
| Scheme 4 Substrate scope for styrenes using CH3CN derivatives.a,b aConditions: 4 (0.2 mmol), CuCl (20 mol%), K2CO3 (1.0 eq.), bromoacetonitrile (2.0 eq.), N2, 135 °C, CH3CN (1.2 mL), 24 h. bIsolated yield. ciodoacetonitrile (2.0 eq.). | |
On the basis of reported mechanistic studies8,9 we examined this reaction and propose a possible pathway of reaction as described in Scheme 5. Accordingly, the C(sp3)–H activation of acetonitrile was possibly promoted by copper species (Scheme 5a).10 Firstly, cyano of acetonitrile could coordinates with Cu species and speculate that the acetonitrile deprotonated via capture of proton by imine substrate (1) to generate nucleophile of acetonitrile (Scheme 5a). Further, it can coordinates with proposed 6a and produces 6b possible species (Scheme 5a). Moreover, 3a (methylbenzenesulfonamide) and 2a (phenylacrylonitrile) could be formed by dehydrogenation and recovered proton transferred to imine (Scheme 5a).
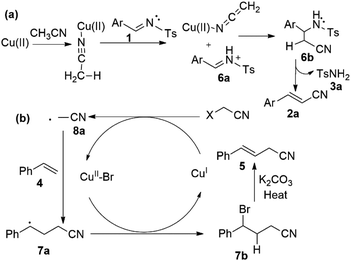 |
| Scheme 5 Proposed mechanism for Cu-catalyzed cyanomethylation of imines and styrenes. | |
Similarly, bromo-acetonitrile activated by copper metal into radical species 8a (Scheme 5b). Consequently, substrate (4) was converted into 7a with Cu(I) species through single electron transfer (SET), was observed by adding 2 equivalent of TEMPO, which abstract a radical hydrogen to form TEMPOH. In addition, TEMPOCH2CN was isolated and confirmed by NMR and spectra was mentioned in ESI.†
Radical of acetonitrile (8a) coupled with substrate (4) and generated intermediate 7a (Scheme 5b). Additionally, this 7a could converted into 7b intermediate by bromide transfer from Cu(II)–Br species (Scheme 5b). This kind of intermediate 7b–1 confirmed by NMR spectroscopy though performing the reaction using 1-chloro-3-vinylbenzene (4r) as substrate under our standard conditions and isolated 4-bromo-4-(3-chlorophenyl)butanenitrile (7b–1) (ESI).† The Cu(I) completed catalytic cycle and intermediate 7b or 7b–1 underwent elimination of proton and gave β,γ-unsaturated cyanomethylated product (5) in the presence of K2CO3 (Scheme 5b). Currently, further mechanstic study is ongoing in our laboratory.
Conclusion
We report copper catalyzed cyanomethylation of imines and α,β-alkenes with acetonitrile (MeCN) and its derivatives for the synthesis of arylacrylonitriles and β,γ-unsaturated nitriles. Moreover, considering the importance of acrylonitrile and β,γ-unsaturated nitriles, this protocol has potential in the industrial production. This method could tolerates broad scope of substrate with substitution of variety of functional groups led good to excellent yields (69–98%). These aromatic acrylonitriles and β,γ-unsaturated nitriles has application in organic reactions and medicinal chemistry which founded in biologically active products.
Conflicts of interest
There are no conflicts to declare.
Notes and references
-
(a) A. Kleemann, J. Engel, B. Kutscher and D. Reichert, Pharmaceutical Substance: Synthesis, Patents, Applications, Georg Thieme, Stuttgart, Germany, 4th edn, 2001 Search PubMed;
(b) R. C. Larock, in Comprehensive organic transformations: a guide to functional group preparations, Wiley-VCH, Weinheim, Germany, 1989, pp. 819–995 Search PubMed.
-
(a) P. Anbarasan, T. Schareina and M. Beller, Chem. Soc. Rev., 2011, 40, 5049 RSC;
(b) M. S. Ahmad, I. N. Pulidindi and C. Li, New J. Chem., 2020, 44, 17177 RSC.
-
(a) M. Murakami, T. Kato and T. Mukaiyama, Chem. Lett., 1987, 1167 CrossRef CAS;
(b) Y. Hayashi and T. Mukaiyama, Chem. Lett., 1987, 1811 CrossRef CAS;
(c) S. Araki, K. Minami and Y. Butsugan, Bull. Chem. Soc. Jpn., 1981, 54, 629 CrossRef CAS;
(d) K. Otaka, D. Oohira and S. Okada, PCT Int. Appl., 2002 Search PubMed WO 2002090320 A2, .
-
(a) D. Munemori, H. Tsuji, K. Uchida, T. Suzuki, K. Isa, M. Minakawa and M. Kawatsura, Synthesis, 2014, 46, 2747 CrossRef;
(b) A. J. Grenning and J. A. Tunge, J. Am. Chem. Soc., 2011, 133, 14785 CrossRef CAS PubMed;
(c) M. N. Soltani Rad, A. Khalafi-Nezhad, S. Behrouz and M. A. Faghihi, Tetrahedron Lett., 2007, 48, 6779 CrossRef CAS;
(d) S. H. Yoneda and T. Kurihara, J. Org. Chem., 1991, 56, 1827 CrossRef;
(e) Y. Tsuji, N. Yamada and S. Tanaka, J. Org. Chem., 1993, 58, 16 CrossRef CAS;
(f) N. W. M. Michel, A. D. M. Jeanneret, H. Kim and S. A. L. Rousseaux, J. Org. Chem., 2018, 83, 11860 CrossRef CAS PubMed;
(g) B. Gao, Y. Xie, L. Yang and H. Huang, Org. Biomol. Chem., 2016, 14, 2399 RSC;
(h) G. Rong, J. Mao, Y. Zheng, R. Yao and X. Xu, Chem. Commun., 2015, 51, 13822 RSC;
(i) F. M. Irudayanathan and S. Lee, Org. Lett., 2017, 19, 2318 CrossRef CAS PubMed;
(j) Y. Amako, S. Arai and A. Nishida, Org. Biomol. Chem., 2017, 15, 1612 RSC;
(k) L. Bini, C. Müller, J. Wilting, S. A. L. von Chrzanowski and D. Vogt, J. Am. Chem. Soc., 2007, 129, 12622 CrossRef CAS PubMed;
(l) H. R. Hoveyda and M. Vézina, Org. Lett., 2005, 7, 2113 CrossRef CAS PubMed;
(m) J. M. Concellón, H. Rodríguez-Solla, C. Simal, D. Santos and N. R. Paz, Org. Lett., 2008, 10, 4549 CrossRef PubMed;
(n) R. Oda, T. Kawabata and S. Tanimoto, Tetrahedron Lett., 1964, 25, 1653 CrossRef.
-
(a) M. Masui, K. Yamagata, C. Ueda and H. Ohmori, J. Chem. Soc., Chem. Commun., 1985, 272 RSC;
(b) R. S. Hiriksan, S. Nanjundian and G. S. Virendra, J. Chem. Soc., Chem. Commun., 1990, 1603 Search PubMed;
(c) T. Yamashita, M. Yasuda, M. Watanabe, R. Kojima, K. Tanabe and K. Shima, J. Org. Chem., 1996, 61, 6438 CrossRef CAS PubMed;
(d) T. Michida and Y. Yamaoka, Chem. Pharm. Bull., 1998, 46, 207 CrossRef CAS;
(e) P. Kisanga, M. Dale, D. S. Bosco and V. John, J. Org. Chem., 1999, 64, 3090 CrossRef CAS PubMed;
(f) G.-W. Wang, A.-X. Zhou, J.-J. Wang, R.-B. Hu and S.-D. Yang, Org. Lett., 2013, 15, 5270 CrossRef CAS PubMed;
(g) H. Yoshida, Y. Fujimura, H. Yuzawa, J. Kumagai and T. Yoshida, Chem. Commun., 2013, 49, 3793 RSC;
(h) S. Chakraborty, Y. J. Patel, J. A. Krause and H. Guan, Angew. Chem., Int. Ed., 2013, 52, 7523 CrossRef CAS PubMed;
(i) J. Shen, D. Yang, Y. Liu, S. Qin, J. Zhang, J. Sun, C. Liu, C. Liu, X. Zhao, C. Chu and R. Liu, Org. Lett., 2014, 16, 350 CrossRef CAS PubMed;
(j) J. Li, Z. Wang, N. Wu, G. Gao and J. You, Chem. Commun., 2014, 50, 15049 RSC;
(k) A. Bunescu, Q. Wang and J. Zhu, Angew. Chem., Int. Ed., 2015, 54, 1 CrossRef PubMed;
(l) J. B. Smith and A. J. M. Miller, Organometallics, 2015, 34, 4669 CrossRef CAS;
(m) C. Pan, H. Zhang and C. Zhu, Org. Biomol. Chem., 2015, 13, 361 RSC;
(n) X.-Q. Chu, X.-P. Xu, H. Meng, Y. Zi and S.-J. Ji, Org. Chem. Front., 2015, 2, 216 RSC;
(o) X.-Q. Chu, X.-P. Xu, H. Meng and S.-J. Ji, RSC Adv., 2015, 5, 67829 RSC;
(p) J. Zhang, W. Wu, X. Ji and S. Cao, RSC Adv., 2015, 5, 20562 RSC;
(q) Z. Qin, X. Huang, J. Wang and Y. Pan, RSC Adv., 2015, 6, 522 Search PubMed;
(r) C. Wang, Y. Li, M. Gong, Q. Wu, J. Zhang, J. K. Kim, M. Huang and Y. Wu, Org. Lett., 2016, 18, 4151 CrossRef CAS PubMed;
(s) Y. Yu, S. Zhuang, P. Liu and P. Sun, J. Org. Chem., 2016, 81, 11489 CrossRef CAS PubMed;
(t) W. Zhang, S. Yang and Z. Shen, Adv. Synth. Catal., 2016, 358, 2392 CrossRef CAS;
(u) Y. Liu, K. Yang and H. Ge, Chem. Sci., 2016, 7, 2804 RSC;
(v) Z. Deng, X. Peng, P. Huang, L. Jiang, D. Ye and L. Liu, Org. Biomol. Chem., 2017, 15, 442 RSC;
(w) H. Su, L. Wang, H. Rao and H. Xu, Org. Lett., 2017, 19, 2226 CrossRef CAS PubMed;
(x) E. Wada, T. Takeuchi, Y. Fujimura, A. Tyagi, T. Kato and H. Yoshida, Catal. Sci. Technol., 2017, 7, 2457 RSC;
(y) W. Xuesong, R. Jan and Vy M. Dong, Angew. Chem., Int. Ed., 2017, 56, 11589 CrossRef PubMed;
(z) A. Bunescu, T. M. Ha, Q. Wang and J. Zhu, Angew. Chem., Int. Ed., 2017, 56, 10555 CrossRef CAS PubMed;
(a
a) D. A. Culkin and J. F. Hartwig, Acc. Chem. Res., 2003, 36, 234 CrossRef CAS PubMed;
(a
b) J. You and J. G. Verkade, Angew. Chem., Int. Ed., 2003, 42, 5051 (Angew. Chem., Int. Ed., 2003, 115, 5205) CrossRef CAS PubMed;
(a
c) Y. Suto, N. Kumagai, S. Matsunaga, M. Kanai and M. Shibasaki, Org. Lett., 2003, 5, 3147 CrossRef CAS PubMed;
(a
d) N. Kumagai, S. Matsunaga and M. Shibasaki, J. Am. Chem. Soc., 2004, 126, 13632 CrossRef CAS PubMed;
(a
e) Y. Suto, R. Tsuji, M. Kanai and M. Shibasaki, Org. Lett., 2005, 7, 3757 CrossRef CAS PubMed;
(a
f) T. Wu, X. Mu and G. Liu, Angew. Chem., Int. Ed., 2011, 50, 12578 (Angew. Chem., Int. Ed., 2011, 123, 12786) CrossRef CAS PubMed;
(a
g) Y. Kawato, N. Kumagai and M. Shibasaki, Chem. Commun., 2013, 49, 11227 RSC;
(a
h) D. S. Kumar, V. Ganesh, N. Kumagai and M. Shibasaki, Chem. –Eur. J., 2014, 20, 15637 CrossRef;
(a
i) D. Sureshkumar, V. Ganesh, N. Kumagai and M. Shibasaki, Chem. –Eur. J., 2014, 20, 15723 CrossRef CAS PubMed;
(a
j) A. Goto, K. Endo, Y. Ukai, S. Irle and S. Saito, Chem. Commun., 2008, 2212 RSC;
(a
k) A. Goto, H. Naka, R. Noyori and S. Saito, Chem. –Asian J., 2011, 6, 1740 CrossRef CAS PubMed.
-
(a) N. Abermil, G. Masson and J. Zhu, J. Am. Chem. Soc., 2008, 130, 12596 CrossRef CAS PubMed;
(b) N. Abermil, G. Masson and J. Zhu, Org. Lett., 2009, 11, 4648 CrossRef CAS PubMed;
(c) N. Abermil, G. Masson and J. Zhu, Adv. Synth. Catal., 2010, 352, 656 CrossRef CAS;
(d) S. Kawahara, A. Nakano, T. Esumi, Y. Iwabuchi and S. Hatakeyama, Org. Lett., 2003, 5, 3103 CrossRef CAS PubMed;
(e) K. Matsui, S. Takizawa and H. Sasai, J. Am. Chem. Soc., 2005, 127, 3680 CrossRef CAS PubMed;
(f) S. Takizawa, N. Inoue, S. Hirata and H. Sasai, Angew. Chem., Int. Ed., 2010, 49, 9725 (Angew. Chem., 2010, 122, 9919) CrossRef CAS PubMed;
(g) I. T. Raheem and E. N. Jacobsen, Adv. Synth. Catal., 2005, 347, 1701 CrossRef CAS;
(h) M. S. Taylor and E. N. Jacobsen, Angew. Chem., Int. Ed., 2006, 45, 1520 (Angew. Chem., 2006, 118, 1550) CrossRef CAS PubMed;
(i) K. Hyodo, S. Nakamura and N. Shibata, Angew. Chem., Int. Ed., 2012, 51, 10337 (Angew. Chem., 2012, 124, 10483) CrossRef CAS PubMed;
(j) T. Yukawa, B. Seelig, Y. Xu, H. Morimoto, S. Matsunaga, A. Berkessel and M. Shibasaki, J. Am. Chem. Soc., 2010, 132, 11988 CrossRef CAS PubMed;
(k) Y. L. Shi and M. Shi, Adv. Synth. Catal., 2007, 349, 2129 CrossRef CAS;
(l) R. Gausepohl, P. Buskens, J. Kleinen, A. Bruckmann, C. W. Lehmann, J. Klankermayer and W. Leitner, Angew. Chem., Int. Ed., 2006, 45, 3555 (Angew. Chem., 2006, 118, 3635) CrossRef;
(m) S. Čhalov, P. Dziedzic, A. Cjrdova and J. Veselý, Adv. Synth. Catal., 2011, 353, 1906 Search PubMed.
-
(a) -F. W. Xiao, V.-L. Chloé, L. B. Bray and D. Christophe, Tetrahedron, 2009, 65, 7380 CrossRef;
(b) D.-G. María and T. C. Brian, Tetrahedron, 2011, 67, 7901 CrossRef;
(c) Z. C. Jessica, Y. Wenzhi, T. H. Brian, K. L. Charles and W. Masayuki, Angew. Chem., Int. Ed., 2016, 55, 13877 CrossRef PubMed;
(d) C. Hao, Z. Yu, Z. Dong, X. Jinyi and L. Hong, Chem. Commun., 2014, 50, 14771 RSC;
(e) K. G. Manas, D. Subhomoy, D. Kalpataru and K. Amit, Org. Biomol. Chem., 2015, 13, 9042 RSC;
(f) F. Mar, C. C. Ana, F. Alberto and A. Jos, Chem.–Eur. J., 2018, 24, 3117 CrossRef PubMed;
(g) Z. Abdolkarim, R. M.-Z. Ahmad, H. Alireza, P. Abolfath, K.-N. Ali and H. B. Mohammad, Synth. Commun., 2009, 39, 3156 CrossRef.
- For selected reviews on CDC reactions, see:
(a) Z. Li, D. S. Bohle and C.-J. Li, Proc. Natl. Acad. Sci. U. S. A., 2006, 103, 8928 CrossRef CAS PubMed;
(b) C.-J. Li, Acc. Chem. Res., 2009, 42, 335 CrossRef CAS PubMed;
(c) S. A. Girard, T. Knauber and C.-J. Li, Angew. Chem., 2014, 126, 76 (Angew. Chem., Int. Ed., 2014, 53, 74) CrossRef.
- For mechanistic studies, see:
(a) E. Boess, D. Sureshkumar, A. Sud, C. Wirtz, C. Fars and M. Klussmann, J. Am. Chem. Soc., 2011, 133, 8106 CrossRef CAS PubMed;
(b) E. Boess, C. Schmitz and M. Klussmann, J. Am. Chem. Soc., 2012, 134, 5317 CrossRef CAS PubMed;
(c) A. Gogoi, S. Guin, S. K. Rout and B. K. Patel, Org. Lett., 2013, 15, 1802 CrossRef CAS PubMed;
(d) A. Gogoi, A. Modi, S. Guin, S. K. Rout, D. Das and B. K. Patel, Chem. Commun., 2014, 50, 10445 RSC.
- R. Lpez and C. Palomo, Angew. Chem., 2015, 127, 13366 (Angew. Chem., Int. Ed., 2015, 54, 13170) CrossRef.
Footnote |
† Electronic supplementary information (ESI) available. See DOI: 10.1039/d0ra10693c |
|
This journal is © The Royal Society of Chemistry 2021 |
Click here to see how this site uses Cookies. View our privacy policy here.