DOI:
10.1039/D1RA00078K
(Paper)
RSC Adv., 2021,
11, 2995-3002
Concentration-dependent supramolecular self-assembly of A1/A2-asymmetric-difunctionalized pillar[5]arene†
Received
5th January 2021
, Accepted 7th January 2021
First published on 14th January 2021
Abstract
A series of A1/A2-bromoalkoxy-and-hydroxy-difunctionalized pillar[5]arenes were synthesized by the removal of the pillar[5]arene-bearing benzyl group using catalytic hydrogenation. The difunctionalized pillar[5]arene bearing 8-bromooctoxy and benzyloxy substituents at the A1/A2 positions formed pseudo[1]rotaxane at low concentration and double-threaded supramolecular dimer at high concentration. The supramolecular self-assembly behavior has been probed with multiple methods including varying (variable) concentration 1H NMR spectroscopy, diffusion-ordered spectroscopy (DOSY), dynamic light scattering (DLS) measurements, isothermal titration calorimetry (ITC), and single-crystal X-ray analysis.
Introduction
Mechanically interlocked molecules (MIMs) such rotaxanes/pseudorotaxanes have been extensively synthesized and studied in past decades and have found many applications in the design of molecular machines,1 chemical probes2 and drug delivery.3 The reversible exchange behavior of pseudorotaxanes, which consist of macrocyclic systems with threaded linear components, plays an important role in the design and fabrication of MIMs based artificial molecular machines and supramolecular polymers.4 Thus, macrocyclic hosts such as cyclodextrins,5 calixarenes,6 cucurbiturils,7 cyclophanes,8 and more recent pillar[n]arenes9 plays an important role in construction of MIMs. Pillar[n]arenes in particular, attracted great interest for their unique structural features, versatile synthetic manipulation, and exceptional ability to selectively encapsulate different kinds of guest molecules.10,11
Pseudo[1]rotaxanes are the simplest form of threaded structures which are mechanically self-interlocked macrocyclic systems with reversible exchange behavior between threaded and open forms. Many pseudo[1]rotaxane based pillar[5]arene have been reported taking advantage of the highly symmetrical rigid structure and electron-donating cavities.9,12 For example, monofunctionalized pillar[5]arene bearing imidazolium moiety that formed stable pseudo[1]rotaxane event at high concentration has been reported.13 The concentration independent nature of self-inclusion was also shown with neutral mono-urea-functionalized pillar[5]arene, however in solid state formed double-threaded dimer.14 In contrast, the concentration dependence behavior of pseudo[1]rotaxane was demonstrated using monofunctionalized pillar[5]arene derivative containing a viologen moiety, which self-complexation at low concentration, assembled into supramolecular daisy chain polymers and eventually organogels upon the increasing of its concentration.15 More recent, concentration dependence nature between the formation of pseudo[1]rotaxane and interlocked-dimer was also been demonstrated using pillar[5]arene bearing ethylene glycol bridged pyridine, in addition to the acid/base control of the dimer between the shrinking state to extension state.16
In this work, we report the synthesis of series of A1/A2 asymmetric-difunctionalized pillar[5]arenes bearing bromoalkyl and hydroxy groups. The synthesized difunctionalized-pillar[5]arene with long 8-bromooctoxy chain and benzyloxy substituents formed two different size aggregates depending on the concentration. The concentration-dependent supramolecular transition between pseudo[1]rotaxane and double-threaded dimer was investigated by variable concentration 1H NMR spectroscopy, diffusion-ordered spectroscopy (DOSY), dynamic light scattering (DLS) measurements, isothermal titration calorimetry (ITC) and X-ray single crystal diffraction studies.
Results and discussion
Synthesis
Macrocyclic compounds with different functionality enable selective manipulations to the macrocycle frame and the synthesis of materials with various architectures. Recently, difunctionalized pillar[5]arene with hydroxy and amino groups at the A1/A2 have been synthesized by controlled de-O-methylation of permethylated pillar[5]arene using oxidation-followed-by-reduction strategy.17 Taking advantage of our previously report approach for the synthesis of hydroxylated pillar[5]arenes,11 a series of asymmetric A1/A2 difunctionalized pillar[5]arenes were synthesized. The approach involves co-cyclization of hydroquinone derivatives of 1,4-dimethoxybenzene and 1-benzyloxy-4-bromoalkoxybenzenes with paraformaldehyde in the presence of BF3·OEt2, as shown in Scheme 1. The copillararenes, Pillar-1(a–c) were isolated by silica gel column chromatography, and their corresponding hydroxylated pillar[5]arenes were obtained after removal of the benzyl protecting group by catalytic hydrogenation under mild reaction conditions using palladium on charcoal in anhydrous ethyl acetate.
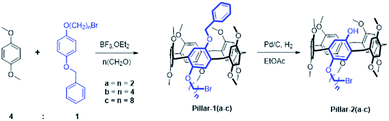 |
| Scheme 1 Synthesis of A1/A2 difunctionalized bromo-hydroxy pillar[5]arenes. | |
NMR studies
1H NMR spectra of pillar[5]arenes based on 2-bromoethoxy (Pillar-1a) and 4-bromobutoxy (Pillar-1b) at 298 K show peaks corresponding to different proton resonances (ESI†). In contrast, 1H NMR spectrum of 8-bromooctoxy based pillar[5]arene (Pillar-1c) show high upfield shift of resonances for methylene protons of the long 8-bromooctoxy arm. In spectrum of Pillar-1c at 298 K no significant upfield shift for methylene protons H1 and H2 were observed, whereas protons H4, H5, H6, H7 and H8 (0.62, −0.35, −1.35, −0.88 and 1.29 respectively) exhibit unusual high field shift which indicate these protons located in central position of pillar[5]arene cavity (Fig. 1a). The methylene protons H5 and H6 show as broad signals due to rapid movement of these groups with respect to NMR time scale. To achieve better-quality signals, the movement was reduced by lowering temperature to 233 K which resulted in higher upfield shifts of the 8-bromooctoxy substituent (Fig. 1b). The peaks assignment was based on 1H–1H–COSY experiments at 298 K and 233 K (ESI†). Further evidence of the encapsulation of the octyl chain deep inside the macrocycle cavity in solution was obtained from 2D ROSY experiment at 233 K in chloroform-d3 which shows strong correlations between all of the octyl protons H4-8 and the aromatic protons H6 as well as bridging methylene protons H12 of the copillar[5]arene unit (ESI†). Variable temperature 1H NMR experiments from −233 to 328 K in chloroform-d3 show that the octyl chain is encapsulated in the pillararene cavity in studied temperature rage (ESI†).
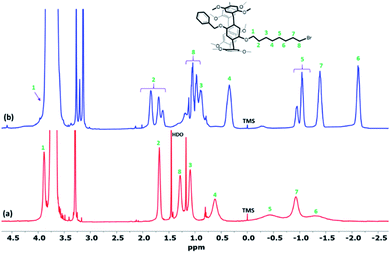 |
| Fig. 1 Partial 1H NMR spectra (600 MHz, CDCl3) of Pillar-1c at 298 K (a) and 233 K (b). | |
To investigate the inclusion behavior of the 8-bromooctoxy chain in pillar[5]arene cavity as self-inclusion or double-threaded dimer (interpenetration structure) or n-mer, 1H NMR spectra at different concentrations (1–100 mM) of Pillar-1c were measured (ESI†). The data clearly demonstrate that Pillar-1c is concentration dependent, suggesting that Pillar-1c exist in different size aggregates. Fig. 2 shows representative examples of 1H NMR spectra of Pillar-1c aromatic regions at 2- and 50 mM concentrations in chloroform-d3 at 298 K. The chemical shift data show the presence of two main size aggregates, one below the 4 mM concentration and other around the 50 mM concentrations. At low concentration, the 1H NMR concentration study implies that Pillar-1c exists predominantly as self-inclusion complex “pseudo[1]rotaxane” and at higher concentration the result indicate the existence of intermolecular complexation “double threaded dimer”, which is evidence by the unusually high chemical upfield shift observed for alkyl chain. In addition, the intermolecular threaded supramolecular formation at high concentration is indicated by the observed downfield chemical shit experienced by aromatic protons of pillaraene frame as a result of the close proximity to the de-shielding region of the adjacent pillararene system (Fig. 2). These observations are similar to results previously reported for pillar[5]arenes with long side chains.15,16 The presence of monomer to dimer in 1
:
1 ratio was estimated ca. 25 mM from a plot of concentration versus chemical shift (ESI†).
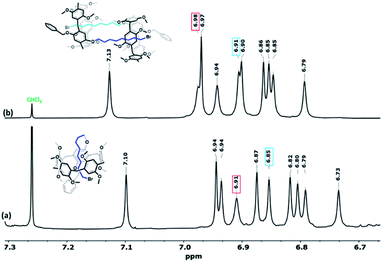 |
| Fig. 2 Partial 1H NMR spectra of pillararene aromatic frame (600 MHz, CDCl3, 298 K) of Pillar-1c at 4 mM (a) and 50 mM (b). | |
The self-assembly behavior of compound Pillar-1c at different concentrations in chloroform-d3 was further studied by two-dimensional diffusion-ordered 1H NMR (DOSY) experiments.4c,18-20 The DOSY spectrum at 2, 50 and 100 mM concentrations revealed one set of signals, manifesting only one aggregate size in solutions. A change in the diffusion coefficient (D) should be observed in the DOSY spectra because larger aggregates have larger hydrodynamic radii (R), and R is inversely proportional to D according to the Strokes–Einstein equation [D = kBT/(6πηR)], where T denotes the temperature, kB is the Boltzmann constant, and η is the dynamic viscosity of the solvent. As the concentration of Pillar-1c increased from 2 mmol L−1 to 50 mmol L−1, the value of weight-averaged diffusion coefficients (D) decreased from 1.08 × 10−9 to 5.17 × 10−10 m2 s−1 (D2/D50 = 2.01). This decrease was sufficient to suggest the change of the average dimension of aggregates with increase the concentration. The calculated hydrodynamic diameters at 2- and 50 mM are 0.76 and 1.58 nm, which correspond to monomeric and dimeric copillar[5]arene assemblies, respectively. At higher concentration of 100 mM, no significant change of the D (4.86 × 10−10 m2 s−1) was observed which indicated no further change of average dimension of the aggregates (Table 1).
Table 1 The hydrodynamic diameter calculated from DOSY experiments for Pillar-1c
Sample conc. (mM) |
log D (log m2 s−1) |
D (10−10 m2 s−1) |
Diametera (nm) |
Diameter calculated using Strokes–Einstein equation. Centered hydrodynamic diameter calculated from DLS measurement. |
2 |
−8.97 |
10.8 |
0.76 (0.72)b |
50 |
−9.29 |
5.17 |
1.58 (1.50)b |
100 |
−9.31 |
4.86 |
1.68 |
The number-averaged degree of aggregation “supramolecular assembly” could be roughly approximated using the diffusion coefficient obtained from DOSY measurements by assuming that all assemblies are hydrodynamically spherical according to the Stokes–Einstein equation [N ≈ 1/(D/Dref)3].4c The number-averaged degree of aggregation “N” is proportional to the reciprocal of the cubic root of the diffusion coefficient, where D and Dref are the diffusion coefficients of dimer and monomer, respectively. The average degree of supramolecular assembly was calculated to be 2, which corresponds to dimer formation. This simple but rough calculation confirms the presence of double threaded dimeric supramolecular assembly at high concertation. Even though the hydroxy derivative Pillar-2c show threaded 8-bromooctoxy chain as evidence from the high upfield shift in the 1H NMR, analyses were complicated by the presence of the hydroxy functional group on the cavity rim (ESI†).
DLS measurement
DLS experiments were conducted in chloroform at 298 K to investigate the supramolecular assembly of Pillar-1c at two different concentrations. DLS measurements are mainly suitable for spherical models and can only give qualitative results about supramolecular assemblies. However, the DLS measurements of the hydrodynamic diameter (Dh) show pronounced scattering intensities at the two measured concentrations (2 and 50 mM) of Pillar-1c. Fig. 3 shows histograms of the Dh distributions for the supramolecular self-assembly at the two different concentrations. In the 2 mM solution, the measured Dh distribution centered at 0.72 nm which is consistent with the Dh value of self-inclusion complex. While, the centered Dh distribution for the 50 mM solution doubled to 1.50 nm indicating the presence of double-threaded supramolecular dimer. The DLS measurements clearly indicate the supramolecular self-assembly of Pillar-1c is pronouncedly concentration dependent, which is in agreement with NMR data.
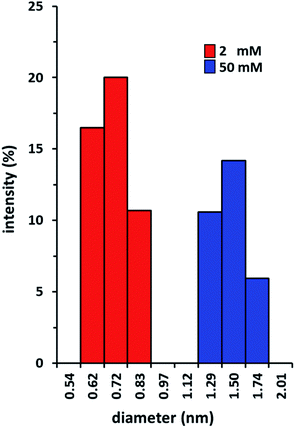 |
| Fig. 3 Hydrodynamic radii distributions of Pillar-1c in chloroform at two different concentrations (2, and 50 mM). | |
ITC studies
The ITC technique has been successfully employed to study the reversible noncovalent interactions of the supramolecular assemblies in solution.21–25 Recently, we reported self-assembled linear supramolecular polymer based on pillar[5]arene driven by guest halogen–halogen interactions,4c the supramolecular assembly in solution was investigated by dilution ITC experiments which involve sequential injections of concentrated solution of supramolecular system into a cell containing pure solvent. Similarly, the concentration-dependent double threaded supramolecular dimer was studied by dilution ITC experiments. At high concentration (50 mM), the dimer is present predominately and the exchanged heat is a measure of the dissociated dimer and it is characteristic of the dissociation event. When the concentration is sufficiently low, there is no further disassembly of the supramolecular dimer and only the heat of dilution remains.
The ITC dilution data for the dissociation of Pillar-1c dimer into chloroform at 2 and 50 mM are shown in Fig. 4. A plot of heat rate (μJ s−1) against time (min) shows a series of peaks where the area under the endothermic heat peaks gives the enthalpy (ΔH) of dissociation (Fig. 4a). At high concertation (50 mM), the integrated heat data fit to a dimer dissociation model with positive value of ΔHdiss = 11.53 kJ mol−1 and dissociation equilibrium constant of Kdiss = 2.27 × 10−2 M (Fig. 4b). In addition, the positive value of Gibbs free energy (ΔG = 11.42 kJ mol−1) obtained from ITC dilution experiment indicate the formation of doubl-threaded supramolecular dimer at high concentration is a spontaneous process. In contrast, ITC dilution experiments at low concentration (Pillar-1c at 2 mM), no significant dimer dissociation was observed as evidence of the endothermic heat pulses (Fig. 4a). The ITC experiment results are in agreement with data obtained from DOSY experiments and DLS measurements.
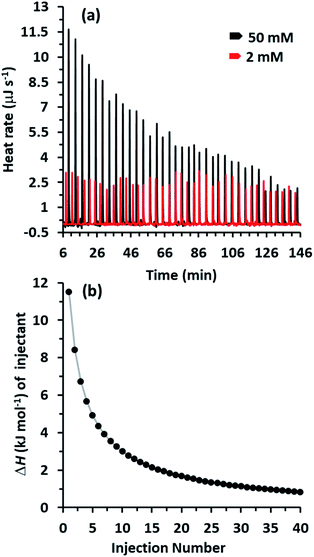 |
| Fig. 4 ITC dilution raw heats for sequential injection in chloroform at 298 K for 2 (red) and 50 (black) mM of Pillar-1c (a), and the integrated heat data for Pillar-1c at 50 mM fitted to a dimer dissociation model (b). | |
Crystallography
Single crystals of Pillar-1a suitable for X-ray diffraction studies were grown by slow vapor diffusion of hexanes into CH2Cl2/1-bromooctane solutions (10/1 v/v), whereas X-ray single crystal structures of pillar[5]arene Pillar-(1b) were obtained by the slow evaporation method using dichloroethane Single crystals of Pillar-1c suitable for X-ray diffraction studies were grown by slow vapor diffusion of acetone into chloroform solution. No suitable crystals of the hydroxy-pillar[5]arene derivatives (Pillar 2a–c) could be obtained for X-ray analysis. For Pillar-1a, the 1-Bromooctane molecules were included in the pillar[5] arene cavity and the pillararenes are aligned in a face-to-edge manner in the crystal network (ESI†). These host–guest complexes were stabilized by C–H⋯π and C–H⋯O interactions. The asymmetric unit of Pillar-1b crystal is observed to be half of the molecular structure and it was complicated by positional disorder for both benzyloxy and 1-bromobutoxy substituents. The crystal refinement was carried out by assigning half occupancy for constituent atoms of two fragments. Since the occupancy of the substituents are half at each site, the completed structure has both substituents (benzyloxy and 1-bromobutoxy) at both of the pillararene rim (ESI†).
The crystal structure of Pillar-1c shows the formation of a supramolecular double-threaded dimer where the bromo-octyl chains is threaded inside the cavity of the adjacent pillar[5]arene (Fig. 5). The formation of interlocked structure in the solid state were previously reported with mono-urea-functionalized pillar[5]arene and copillar[5]arene with long bromodceyl group in the solid state.14,26 The dimer formation is induced by C–H⋯π and C–H⋯O interactions between the octyl chain and the host. The encapsulation characteristics demonstrated in the solid state by the single-crystal X-ray diffraction technique agree with the results obtained from 1H NMR concentration experiments, DOSY and DLS measurements which indicate the formation of a supramolecular double-threaded dimer or [c2]daisy chain type structure at high concertation.
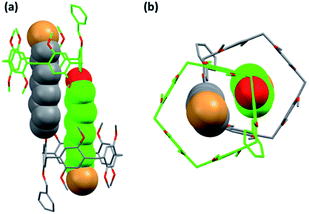 |
| Fig. 5 Supramolecular double-threaded dimer of Pillar-1c. (a) The top view, and (b) the side view. Red, grey (or green) and yellow denote oxygen, carbon and bromine, respectively. Hydrogen atoms are omitted for clarity. | |
Experimental
Materials and methods
Nuclear Magnetic Resonance (NMR) spectroscopy measurements were carried out by Bruker Avance II 600 MHz (Germany), spectrometer. Electron impact ionization (EI) mass spectrometry was performed using Thermo Scientific DFS High Resolution GC/MS (Germany) mass spectrometer. Electro-spary ionization in high resolution mode was done using Waters Xevo G2-S Qtof, (Germany) LC MS/MS mass spectrometer. The single crystal data analysis was made by R-AXIS RAPID II, Rigaku, (Japan) diffractometer. The data were collected at 150 K (Oxford cryosystems, UK). Flash column chromatography was performed using silica gel (Silica gel 60, 40–60 mesh ASTM, EMD Millipore, Merck KGaA, Germany). 4-Benzyloxyphenol was synthesized according to literature procedure.11 DMF, acetonitrile and dichloroethane were distilled before use. All other reagents and solvents were of reagent grade purity and used without further purification.
Preparation of single crystals for X-ray diffraction
The single crystal diffraction data were collected on an R-AXIS RAPID diffractometer (Rigaku, Japan) at 150 K using the Crystal-Clear software package. The structures were solved by direct methods and expanded using Fourier techniques. The structure refinement was performed using SHELXL-2017/1. All non-hydrogen atoms were refined anisotropically, and hydrogen atoms were placed at calculated positions and refined using the riding model. The crystallographic data for the structure reported in this paper have been deposited at the Cambridge Crystallographic Data Centre as a supplementary publication (CCDC 2000224–2000226).
DLS measurements
Samples of the Pillar-1c at different concentrations (2 and 50 mM in CHCl3) were analyzed on a Zetasizer Nano Range, Malvern PANalytical (UK) at 298 K. All DLS measurements were performed at a scattering angle of 90°. Sample solutions were prepared by filtering each component solution through a 0.2 μm polytetrafluoroethylene (PTFE) syringe filter into a clean scintillation vial.
ITC measurements
All ITC studies were carried out on an Affinity ITC, TA Instruments (USA). The data were analyzed using NanoAnalyze (Version 3.10.0). In the ITC dimer-dissociation experiments, a 10 mM solution of the self-assembled Pillar-1c system in CHCl3 was placed in a syringe and automatically titrated as 2 μL per injection at a time interval of 240 s into a reaction cell loaded with pure CHCl3 (300 μL). The dissociation of the self-assembled aggregates was accompanied by non-constant heat signals along with a constant heat of dilution. A control experiment was performed using 2 mM Pillar-1c chloroform solution under similar experimental conditions against pure CHCl3. All the titrations were conducted at 298 K.
Synthesis of 1-benzyloxy-4-bromoalkoxybenzenes (1–4)
1-(Benzyloxy)-4-(2-bromoethoxy)benzene (1). 4-Benzyloxyphenol (2 g, 10 mmol) was dissolved in dry DMF (30 mL) at room temperature, and anhydrous potassium carbonate (2.76 g; 20 mmol) was added. Then 1,2-dibroethane (1.20 mL, 14 mmol) was added, and the mixture was heated at 80 °C overnight. The product was precipitated in ice cold water and purified by column chromatography using dichloromethane/hexane mixture (65
:
35 v/v). Yield 2.4 g, 78%. 1H NMR (600 MHz, CDCl3) δ: 3.63 (t, J = 6.0 and J = 6.6 Hz, 2H), 4.26 (t, J = 6.0 and J = 6.6 Hz, 2H), 5.04 (s, 2H), 6.87 (m, 2H), 6.92 (m, 2H), 7.33 (m, 1H), 7.40 (m, 2H), 7.44 (m, 2H). 13C NMR (150 MHz, CDCl3), δ: 29.5, 69.0, 70.9, 116.1, 116.3, 127.7, 128.1, 128.8, 137.4, 152.6, 153.8. HRMS: (m/z): calcd for [M+]: 306.0250 (for C15H15O2Br); found 306.0250.
1-(Benzyloxy)-4-(4-bromobutoxy)benzene (2). 4-Benzyloxyphenol (2 g, 10 mmol) was dissolved in dry DMF (30 mL) at room temperature, and anhydrous potassium carbonate (2.76 g; 20 mmol) was added. Then 1,4-dibromobutane (1.96 mL, 14 mmol) was added, and the mixture was heated at 80 °C overnight. The product was precipitated in ice cold water and purified by column chromatography using dichloromethane/hexane mixture (65
:
35 v/v). Yield 3.1 g, 92%. 1H NMR (600 MHz, CDCl3) δ: 1.94 (m, 2H) 2.09 (m, 2H), 3.51 (t, J = 6.6 and J = 6.6 Hz, 2H), 3.97 (t, J = 6.6 and J = 6.0 Hz, 2H), 5.04 (s, 2H), 6.85 (m, 2H), 6.92 (m, 2H), 7.34 (m, 1H), 7.40 (m, 2H), 7.43 (m, 2H). 13C NMR (150 MHz, CDCl3), δ: 28.2, 29.7, 33.7, 67.7, 70.9, 71.0, 115.6, 116.1, 116.1, 127.7, 128.1, 128.8, 137.5, 153.3, 153.5. HRMS: (m/z): calcd for [M+]: 334.0563 (for C17H19O2Br); found 334.0564.
1-(Benzyloxy)-4-(8-bromooctoxy)benzene (3). 4-Benzyloxyphenol (2 g, 10 mmol) was dissolved in dry DMF (30 mL) at room temperature, and anhydrous potassium carbonate (2.76 g; 20 mmol) was added. Then 1,8-dibromooctane (2.64 mL, 14 mmol) was added, and the mixture was heated at 80 °C overnight. The product was precipitated in ice cold water and purified by column chromatography using dichloromethane/hexane mixture (60
:
40 v/v). Yield 3.4 g, 85%. 1H NMR (600 MHz, CDCl3) δ: 1.38 (m, 4H), 1.47 (m, 4H), 1.77 (m, 2H), 1.87 (m, 2H), 3.42 (m, 2H), 3.92 (t, J = 7.2 and J = 6.6 Hz, 2H), 5.04 (s, 2H), 6.85 (m, 2H), 6.93 (m, 2H), 7.34 (m, 1H), 7.39 (m, 2H), 7.44 (m, 2H). 13C NMR (150 MHz, CDCl3), δ: 25.8, 26.1, 28.2, 28.3, 28.8, 28.8, 28.9, 29.0, 29.2, 29.4, 29.5, 32.9, 33.0, 34.1, 34.2, 68.1, 68.7, 70.9, 115.6, 116.0, 127.7, 128.1, 128.7, 137.5, 153.1, 153.7. HRMS: (m/z): calcd for [M+]: 390.1189 (for C21H27O2Br); found 390.1189.
Synthesis of A1/A2-benzyloxybromoalkoxy difunctionalized-pillar[5]arenes 1(a–c)
Pillar-1(a). Paraformaldehyde (1.5 g, 50 mmol) was added to a solution of 1,4-dimethoxybenzene (2.2 g, 16.0 mmol) and 1-benzyloxy-4-(2-bromoethoxy)benzene (306 mg, 1 mmol) in dry dichloromethane (120 mL) under nitrogen atmosphere. Boron trifluoride diethyl etherate (BF3·OEt2), (2.0 mL, 16 mmol) was then added to the solution and the mixture was stirred at 30 °C for 1 h. After this time, methanol (50 mL) was poured into the reaction mixture and the solution was concentrated then dissolved in dichloromethane (100 mL). The resulting organic solution was washed with aqueous NaHCO3 (2 × 50 mL) and H2O (50 mL), then dried over anhydrous Na2SO4, concentrated under reduced pressure, and subjected to purification by silica gel chromatography (dichloromethane/hexanes (75
:
25 v/v)) to give Pillar-1(a) as a white solid (240 mg, 26%), 1H NMR (600 MHz, CDCl3) δ: 3.37 (s, 3H), 3.55 (t, J = 6.0, 6.6, 2H), 3.61 (s, 3H), 3.69 (s, 3H), 3.72 (m, 15H), 3.79 (s, 2H). 3.82 (m, 4H), 3.85 (d, J = 8.4, 4H) 4.15 (t, J = 6.0, 6.6, 2H), 4.97 (s, 2H), 6.72 (s, 1H), 6.80 (m, 3H), 6.82 (s, 1H), 6.85 (m, 4H), 6.93 (s, 1H), 7.36 (m, 3H), 7.43 (m, 2H). 13C NMR (150 MHz, CDCl3), δ: 29.5, 29.8, 29.8, 30.1,30.2, 53.2, 55.6, 55.8, 55.9, 56.0, 56.2, 69.0, 70.5, 114.1, 114.1, 114.2, 114.2, 114.3, 115.1, 116.0, 127.4, 127.8, 127.9, 128.3, 128.4, 128.4, 128.5, 128.5, 128.6, 128.6, 128.8, 129.3, 138.1, 149.4, 150.8, 150.9, 150.9, 151.0. HRMS: (m/z): calcd for [M+]: 918.2973 (for C52H55O10Br); found 918.2971.
Pillar-1(b). Paraformaldehyde (1.5 g, 50 mmol) was added to a solution of 1,4-dimethoxybenzene (2.2 g, 16.0 mmol) and 1-benzyloxy-4-(4-bromobutoxy)benzene (335 mg, 1 mmol) in dry dichloromethane (120 mL) under nitrogen atmosphere. Boron trifluoride diethyl etherate (BF3·OEt2), (2.0 mL, 16 mmol) was then added to the solution and the mixture was stirred at 30 °C for 1 h. After this time, methanol (50 mL) was poured into the reaction mixture and the solution was concentrated then dissolved in dichloromethane (100 mL). The resulting organic solution was washed with aqueous NaHCO3 (2 × 50 mL) and H2O (50 mL), then dried over anhydrous Na2SO4, concentrated under reduced pressure, and subjected to purification by silica gel chromatography (dichloromethane/hexanes (65
:
35 v/v)) to give Pillar-1(b) as white solid (330 mg, 35%). 1H NMR (600 MHz, CDCl3) δ: 1.76 (s, 4H), 3.04 (s, 2H) 3.32 (s, 3H), 3.63 (d, J = 8.4, 6H), 3.69 (m, 13H), 3.78 (s, 2H), 3.81 (s, 8H), 3.87 (s, 2H), 4.91 (s, 2H), 6.72 (s, 2H), 6.81 (m, 5H), 6.85 (s, 1H), 6.87 (s, 1H), 6.88 (s, 1H), 7.34 (m, 3H), 7.40 (m, 2H). 13C NMR (150 MHz, CDCl3), δ: 28.6, 29.7, 29.8, 30.0, 30.3, 33.6, 55.5, 55.9, 56.0, 56.1, 56.2, 56.3, 56.4, 56.4, 67.6, 70.6, 114.1, 114.2, 114.2, 114.3, 114.3, 114.4, 115.2, 115.3, 127.4, 127.8, 128.2, 128.4, 128.4, 128.4, 128.4, 128.5, 128.5, 128.6, 128.6, 128.6, 138.2, 150.2, 150.3, 150.9, 150.9, 150.9, 151.0. HRMS: (m/z): calcd for [M + H2O]: 964.3397 (for C54H59O11Br); found 964.3345.
Pillar-1(c). Paraformaldehyde (1.5 g, 50 mmol) was added to a solution of 1,4-dimethoxybenzene (2.21 g, 16.0 mmol) and 1-benzyloxy-4-(n-bromo)octyloxy benzene (391 mg, 1 mmol) in dry dichloromethane (120 mL) under nitrogen atmosphere. Boron trifluoride diethyl etherate (BF3·OEt2), (2.0 mL, 16 mmol) was then added to the solution and the mixture was stirred at 30 °C for 1 h. After this time, methanol (50 mL) was poured into the reaction mixture and the solution was concentrated then dissolved in dichloromethane (100 mL). The resulting organic solution was washed with aqueous NaHCO3 (2 × 50 mL) and H2O (50 mL), then dried over anhydrous Na2SO4, concentrated under reduced pressure, and subjected to purification by silica gel chromatography (dichloromethane/hexanes (65
:
35 v/v)) to give Pillar-1(c) as a white solid (410 mg, 41%).1H NMR (600 MHz, CDCl3) δ: −1.35 (s, 2H), −0.90 (s, 2H), −0.45 (s, 2H), 0.67 (s, 2H), 1.18 (s, 2H), 1.34 (s, 2H), 1.80 (s, 2H), 3.36 (s, 3H), 3.85 (m, 31H), 3.98 (s, 2H), 5.01 (s, 2H), 6.79 (s, 1H), 6.86 (m, 3H), 6.92 (m, 2H), 6.95 (s, 1H), 6.99 (m, 2H), 7.16 (s, 1H), 7.36 (m, 1H), 7.45 (t, J = 7.8, 7.2, 2H), 7.63 (d, J = 7.3, 2H). 13C NMR (150 MHz, CDCl3), δ: 26.8, 27.5, 29.1, 29.3, 29.6, 29.9, 34.0, 55.1, 55.4, 55.4, 55.5, 55.5, 55.5, 55.8, 70.0, 113.1, 113.1, 113.2, 113.3, 113.6, 114.1, 114.4, 127.8, 127.9, 128.1, 128.2, 128.4, 128.6, 128.8, 138.3, 149.5, 149.6, 150.2, 150.2, 150.3, 150.3, 150.5, 150.7, 150.8. HRMS: (m/z): calcd for [M+]: 1002.3913 (for C58H67O10Br); found 1002.3912.
Synthesis of A1/A2-benzyloxybromoalkoxy difunctionalized-pillar[5]arenes 2(a–c)
Pillar-2(a). To a solution of the starting material Pillar-1(a) (100 mg, 0.11 mmol) in anhydrous ethyl acetate (30 mL) was added Pd/C (20 mg). The reaction mixture was stirred at room temperature under an atmosphere of hydrogen for 24 h in the hydrogenation chamber. The catalyst was filtered through Celite. The Celite pad was washed with ethyl acetate (30 mL × 2). The combined filtrate was concentrated under reduced pressure. The residue was purified by silica gel column chromatography (99
:
1 v/v, CH2Cl2/methanol) to afford the desired product Pillar-2(a) as a white solid (yield 83 mg, 92%). 1H NMR (600 MHz, CDCl3) δ: 3.53 (s, 3H), 3.62 (m, 15H), 3.73 (m, 6H), 3.81 (m, 12H), 4.19 (t, J = 6.0, J = 6.6, 2H), 6.62 (d, J = 5.4, 2H), 6.65 (s, 1H), 6.69 (m, 2H), 6.75 (m, 4H), 6.90 (s, 1H). 13C NMR (150 MHz, CDCl3), δ: 28.2, 29.0, 29.6, 29.8, 29.9, 30.0, 30.0, 30.1, 30.3, 31.1, 32.1 55.6, 55.9, 56.0, 56.1, 56.2, 56.2, 56.3, 56.4, 56.6, 69.9, 113.2, 114.0, 114.0, 114.3, 114.5, 114.6, 114.7, 114.7, 114.8, 115.5, 119.2, 123.7, 125.5, 126.8, 128.0, 128.3, 128.5, 128.5, 128.6, 128.6, 129.5, 129.7, 131.1, 133.6, 148.6, 148.8, 149.5, 150.0, 151.0, 151.0, 151.1, 151.1, 151.2, 151.3, 152.1. HRMS: (m/z): calcd for [M + H2O]: 846.2615 (for C45H51O11Br); found 846.2672.
Pillar-2(b). To a solution of the starting material Pillar-1(b) (100 mg, 0.11 mmol) in anhydrous ethyl acetate (30 mL) was added Pd/C (20 mg). The reaction mixture was stirred at room temperature under an atmosphere of hydrogen for 24 h in the hydrogenation chamber. The catalyst was filtered through Celite. The Celite pad was washed with ethyl acetate (30 mL × 2). The combined filtrate was concentrated under reduced pressure. The residue was purified by silica gel column chromatography (99
:
1 v/v, CH2Cl2/methanol) to afford the desired product Pillar-2(b) as a white solid (yield 81 mg, 90%).1H NMR (600 MHz, CDCl3) δ: 1.70 (s, 2H), 3.14 (S, 2H), 3.54 (s, 3H), 3.62 (m, 4H), 3.65 (m, 10H), 3.76 (m, 11H), 3.81 (m, 10H), 6.69 (m, 5H), 6.79 (s, 2H), 6.84 (m, 1H), 6.88 (s, 1H), 6.93 (s, 1H). 13C NMR (150 MHz, CDCl3) δ: 28.2, 28.5, 28.8, 29.2, 29.6, 29.6, 29.9, 30.3, 31.1, 33.5, 55.6, 55.9, 56.0, 56.1, 56.1, 56.2, 56.4, 56.6, 68.0, 113.3, 113.8, 113.9, 114.2, 114.3, 114.4, 114.5, 114.7, 119.1, 123.6, 125.3, 127.1, 128.0, 128.0, 128.3, 128.4, 128.5, 128.5, 128.8, 129.5, 129.6, 130.0, 133.6, 146.7, 147.7, 148.8, 150.3, 150.9, 150.9, 150.9, 151.0, 151.0, 151.1, 151.2, 151.2, 152.0. HRMS: (m/z): calcd for [M + H2O]: 874.2928 (for C47H55O11Br); found 874.2988.
Pillar-2(c). To a solution of the starting material Pillar-1(c) (100 mg, 0.11 mmol) in anhydrous ethyl acetate (30 mL) was added Pd/C (20 mg). The reaction mixture was stirred at room temperature under an atmosphere of hydrogen for 24 h in the hydrogenation chamber. The catalyst was filtered through Celite. The Celite pad was washed with ethyl acetate (30 mL × 2). The combined filtrate was concentrated under reduced pressure. The residue was purified by silica gel column chromatography (99
:
1 v/v, CH2Cl2/methanol) to afford the desired product Pillar-2(c) as a white solid (yield 85 mg, 93%).1H NMR (600 MHz, CDCl3) δ: −0.09 (s, 2H), 0.28 (s, 2H) 0.38 (s, 2H), 0.97 (s, 2H), 1.29 (m, 2H), 1.75 (m, 2H), 2.24 (s, 2H), 3.68 (s, 3H), 3.71 (m, 14H) 3.79 (m, 14H), 3.84 (s, 3H), 3.95 (m, 2H), 6.65 (s, 1H), 6.71 (s, 1H), 6.78 (m, 3H), 6.82 (m, 4H), 6.93 (s, 1H). 13C NMR (150 MHz, CDCl3), δ: 25.2, 27.2, 27.8, 28.7, 28.9, 29.1, 29.6, 29.8, 29.9, 31.0, 32,1, 34.0, 55.7, 55.8, 55.8, 55.8, 55.9, 55.9, 56.0, 56.3, 68.5, 113.0, 113.6, 113.8, 113.8, 113.9, 113.9, 113.9, 114.0, 114.2, 114.4, 119.4, 125.7, 127.3, 127.9, 128.2, 128.3, 128.3, 128.4, 128.5, 129.3, 129.9, 147.2, 149.0, 149.8, 150.7, 150.8, 150.8, 150.9, 150.9, 151.6. HRMS: (m/z): calcd for [M+]: 912.3443 (for C51H61O10Br); found 912.3448.
Conclusions
In summary, A1/A2-difunctionalized pillar[5]arenes bearing bromoalkoxy and hydroxy substituents were synthesized by the co-cyclization of hydroquinone derivatives followed by the removal of the benzyl protected group using catalytic hydrogenation over palladium on charcoal in anhydrous ethyl acetate. The 8-bromooctoxy substituent in the pillar[5]arene rim (Pillar-1c) undergo self-inclusion as evidence by the unusual high upfield proton chemical shift. 1HNMR concentration experiments demonstrated that the inclusion behavior of long chain 8-bromooctoxy substituent is concentration-dependent, suggesting the presence of two main size aggregates. The formation of pseudo[1]rotaxane and double-threaded supramolecular dimer at low and high concentrations respectively was confirmed by single-crystal X-ray diffraction analysis, DOSY, DLS and ITC measurements. Currently further studies and modifications of the A1/A2-bromoalkyloxy-hydroxy-functionalized pillar[5]arenes host to develop new supramolecular systems and functional materials are underway in our laboratories.
Conflicts of interest
There are no conflicts to declare.
Acknowledgements
The support received from the Kuwait Foundation of Advancement of Science (KFAS), made available through research grant no. PR17-14SC-07, and the facilities of the RSPU (grant no. GS01/01, GS01/03, and GS03/08) are gratefully acknowledged.
Notes and references
-
(a) D. A. Leigh, Genesis of the Nanomachines: The 2016 Nobel Prize in Chemistry, Angew. Chem., Int. Ed., 2016, 55, 14506–14508 CrossRef CAS;
(b) J. F. Stoddart, The Master of Chemical Topology, Chem. Soc. Rev., 2009, 38, 1521–1529 RSC.
- S. F. M. van Dongen, S. Cantekin, J. Elemans, A. E. Rowan and R. J. M. Nolte, Functional Interlocked Systems, Chem. Soc. Rev., 2014, 43, 99–122 RSC.
- N. Pairault, R. Barat, I. Tranoy-Opalinski, B. Renoux, M. Thomas and S. Papot, Rotaxane-Based Architectures for Biological Applications, C. R. Chim., 2016, 19, 103–112 CrossRef CAS.
-
(a) L. Brunsveld, B. J. B. Folmer, E. W. Meijer and R. P. Sijbesma, Supramolecular Polymers, Chem. Rev., 2001, 101, 4071–4098 CrossRef CAS;
(b) L. Yang, X. Tan, Z. Wang and X. Zhang, Supramolecular Polymers: Historical Development, Preparation, Characterization, and Functions, Chem. Rev., 2015, 115, 7196–7239 CrossRef CAS;
(c) T. Ogoshi, T. Yamagishi and Y. Nakamoto, Pillar-Shaped Macrocyclic Hosts Pillar[n]arenes: New Key Players for Supramolecular Chemistry, Chem. Rev., 2016, 116, 7937–8002 CrossRef CAS;
(d) T. F. Al-Azemi and M. Vinodh, Pillar[5]arene-Based Self-Assembled Linear Supramolecular Polymer Driven by Guest Halogen–Halogen Interactions in Solid and Solution States, Polym. Chem., 2020, 11, 3305–3312 RSC.
- Y. Kazuhiro, M. Atsuhisa, T. Yoshinori, Y. Hiroyasu and H. Akira, Switching Between Supramolecular Dimer and Nonthreaded Supramolecular Self-Assembly of Stilbene Amide-α-Cyclodextrin by Photoirradiation, Org. Lett., 2010, 12(6), 1284–1286 CrossRef.
- G. Giuseppe, N. Anna, P. Andrea, P. Melchiorre, P. Ilenia, P. Sebastiano, G. Domenico, M. Angela, C. Yoram and S. Sarit, Self-Assembly Dynamics of Modular Homoditopic Bis-calix[5]arenes and Long-Chain α,ω-Alkanediyldiammonium Components, J. Org. Chem., 2008, 73(18), 7280–7289 CrossRef.
- S. J. Barrow, S. Kasera, M. J. Rowland, J. del Barrio and O. A. Scherman, Cucurbituril-Based Molecular Recognition, Chem. Rev., 2015, 115, 12320–12406 CrossRef CAS.
- E. J. Dale, N. A. Vermeulen, M. Juríček, J. C. Barnes, R. M. Young, M. R. Wasielewski and J. F. Stoddart, Supramolecular Explorations: Exhibiting the Extent of Extended Cationic Cyclophanes, Acc. Chem. Res., 2016, 49, 262–273 CrossRef CAS.
- T. Ogoshi, K. Demachi, K. Kitajima and T. Yamagishi, Monofunctionalized Pillar[5]arenes: Synthesis and Supramolecular Structure, Chem. Commun., 2011, 47, 7164–7166 RSC.
-
(a) T. Ogoshi, S. Kanai, S. Fujinami, T. A. Yamagishi and Y. Nakamoto, para-Bridged Symmetrical Pillar[5]arenes: Their Lewis Acid Catalyzed Synthesis and Host–Guest Property, J. Am. Chem. Soc., 2008, 130, 5022–5023 CrossRef CAS;
(b) Z. Zhang, Y. Luo, J. Chen, S. Dong, Y. Yu, Z. Ma and F. Huang, Formation of Linear Supramolecular Polymers That is Driven by C–H⋯π Interactions in Solution and in the Solid State, Angew. Chem., Int. Ed., 2011, 50, 1397–1401 CrossRef CAS;
(c) Y. Wu, J. Zhou, E. Li, M. Wang, K. Jie, H. Zhu and F. Huang, Selective Separation of Methylfuran and Dimethylfuran by Nonporous Adaptive Crystals of Pillararenes, J. Am. Chem. Soc., 2020, 142, 19722–19730 CrossRef CAS;
(d) Y. Zhou, K. Jie, R. Zhao, E. Li and F. Huang, Highly Selective Removal of Trace Isomers by Nonporous Adaptive Pillararene Crystals for Chlorobutane Purification, J. Am. Chem. Soc., 2020, 142, 6957–6961 CrossRef CAS;
(e) X. Sheng, E. Li, Y. Zhou, R. Zhao, W. Zhu and F. Huang, Separation of 2-Chloropyridine/3-Chloropyridine by Nonporous Adaptive Crystals of Pillararenes with Different Substituents and Cavity Sizes, J. Am. Chem. Soc., 2020, 142, 6360–6364 CrossRef CAS;
(f) M. Wang, J. Zhou, E. Li, Y. Zhou, Q. Li and F. Huang, Separation of Monochlorotoluene Isomers by Nonporous Adaptive Crystals of Perethylated Pillar[5]arene and Pillar[6]arene, J. Am. Chem. Soc., 2019, 141, 17102–17106 CrossRef CAS.
-
(a) T. F. Al-Azemi, M. Vinodh, F. H. Alipour and A. A. Mohamod, Constitutional Isomers of Pentahydroxy-Functionalized Pillar[5]arenes: Synthesis, Characterization, and Crystal Structures, J. Org. Chem., 2017, 82, 10945–10952 CrossRef CAS;
(b) T. F. Al-Azemi, A. A. Mohamod, M. Vinodh and F. H. Alipour, A New Approach for the Synthesis of Mono- and A1/A2-Dihydroxy-Substituted Pillar[5]arenes and Their Complexation with Alkyl Alcohols in Solution and in the Solid State, Org. Chem. Front., 2018, 5, 10–18 RSC;
(c) T. F. Al-Azemi, M. Vinodh, F. H. Alipour and A. A. Mohamod, Chiral Discrimination of 2-Heptlyaminium Salt by Planar-Chiral Monohydroxy-Functionalized Pillar[5]arenes, Org. Chem. Front., 2019, 6, 603–610 RSC.
-
(a) Y. H. Cui, R. Deng, Z. Li, X. S. Du, Q. Jia, X. H. Wang, C. Y. Wang, K. Meguellati and Y. W. Yang, Pillar[5]arene Pseudo[1]rotaxane-Based Redox-Responsive Supramolecular Vesicles for Controlled Drug Release, Mater. Chem. Front., 2019, 3, 1427–1432 RSC;
(b) J. F. Chen, X. Liu, J. F. Ma, B. B. Han, J. D. Ding, Q. Lin, H. Yao, Y. M. Zhang and T. B. Wei, A Pillar[5]arene-Based Multiple-Stimuli Responsive Metal-Organic Gel Was Constructed for Facile Removal of Mercury Ions, Soft Matter, 2017, 13(30), 5214–5218 RSC;
(c) T. B. Wei, J. F. Chen, X. B. Cheng, H. Li, B. B. Han, H. Yao, Y. M. Zhang and Q. Lin, Construction of Stimuli-Responsive Supramolecular Gel via Bispillar[5]arene-Based Multiple Interactions, Polym. Chem., 2017, 8, 2005–2009 RSC;
(d) X. Wu, L. Gao, J. Sun, X. Y. Hu and L. Wang, Stable Pillar[5]arene-Based Pseudo[1]rotaxanes Formed in Polar Solution, Chin. Chem. Lett., 2016, 27(11), 1655–1660 CrossRef CAS;
(e) Z. Zhang, C. Han, G. Yu and F. Huang, A solvent-driven molecular spring, Chem. Sci., 2012, 3, 3026–3031 RSC.
- C.-L. Sun, J.-F. Xu, Y.-Z. Chen, L.-Y. Niu, L.-Z. Wu, C.-H. Tung and Q.-Z. Yang, Monofunctionalized Pillar[5]arene-Based Stable [1]pseudorotaxane, Chin. Chem. Lett., 2015, 26, 843–846 CrossRef CAS.
- M. Ni, X.-Y. Hu, J. Jiang and L. Wang, The Self-Complexation of Mono-Urea-Functionalized Pillar[5]arenes with Abnormal Urea Behaviors, Chem. Commun., 2014, 50, 1317–1319 RSC.
- N. L. Strutt, H. C. Zhang, M. A. Giesener, J. Y. Lei and J. F. Stoddart, A Self-Complexing and Self-Assembling Pillar[5]arene, Chem. Commun., 2012, 48, 1647–1649 RSC.
- Q. Zhao, Y. Chen, B. Sun, C. Qian, M. Cheng, J. Jiang, C. Lin and L. Wang, Pillar[5]arene Based Pseudo[1]rotaxane Operating as Acid/Base-Controllable Two State Molecular Shuttle, Eur. J. Org. Chem., 2019, 21, 3396–3400 CrossRef.
- C. Han, D. Zhao, Z. Lü, F. Zhan, L. Zhang, S. Dong and L. Jin, Synthesis of a Difunctionalized Pillar[5]arene with Hydroxyl and Amino Groups at A1/A2
Positions, Eur. J. Org. Chem., 2019, 14, 2508–2512 CrossRef.
- C. S. Johnson, Diffusion Ordered Nuclear Magnetic Resonance Spectroscopy: Principles and Applications, Prog. Nucl. Magn. Reson. Spectrosc., 1999, 34(3–4), 203–256 CrossRef CAS.
- Y. Cohen, L. Avram and L. Frish, Diffusion NMR Spectroscopy in Supramolecular and Combinatorial Chemistry: An Old Parameter—New Insights, Angew. Chem., Int. Ed., 2005, 44(4), 520–554 CrossRef CAS.
- K. P. Wang, D. S. Guo, H. X. Zhao and Y. Liu, Synthesis of Doubly Ethyl-Bridged Bis(p-sulfonatocalix[4]arene) and its Supramolecular Polymerization with Viologen Dimer, Chem.–Eur. J., 2014, 20(14), 4023–4031 CrossRef CAS.
- A. Arnaud and L. Bouteiller, Isothermal Titration Calorimetry of Supramolecular Polymers, Langmuir, 2004, 20(16), 6858–6863 CrossRef CAS.
- P. P. N. Syamala, B. Soberats, D. Görl, S. Gekle and F. Würthner, Thermodynamic Insights into the Entropically Driven Self-assembly of Amphiphilic Dyes in Water, Chem. Sci., 2019, 10(40), 9358–9366 RSC.
- P. Dey, P. Rajdev, P. Pramanik and S. Ghosh, Specific Supramolecular Interaction Regulated Entropically Favorable Assembly of Amphiphilic Macromolecules, Macromolecules, 2018, 51(14), 5182–5190 CrossRef CAS.
- J. Matern, Y. Dorca, L. Sánchez and G. Fernández, Revising Complex Supramolecular Polymerization under Kinetic and Thermodynamic Control, Angew. Chem., Int. Ed., 2019, 58(47), 16730–16740 CrossRef CAS.
- V. H. S. Tellini, A. Jover, J. C. García, L. Galantini, F. Meijide and J. V. Tato, Thermodynamics of Formation of Host–Guest Supramolecular Polymers, J. Am. Chem. Soc., 2006, 128(17), 5728–5734 CrossRef CAS.
- Z. Zhang, G. Yu, C. Han, J. Liu, X. Ding, Y. Yu and F. Huang, Formation of a Cyclic Dimer Containing Two Mirror Image Monomers in the Solid State Controlled by van der Waals Forces, Org. Lett., 2011, 13, 4818–4821 CrossRef CAS.
Footnote |
† Electronic supplementary information (ESI) available. CCDC 2000224–2000226. For ESI and crystallographic data in CIF or other electronic format see DOI: 10.1039/d1ra00078k |
|
This journal is © The Royal Society of Chemistry 2021 |
Click here to see how this site uses Cookies. View our privacy policy here.