DOI:
10.1039/D1RA02132J
(Paper)
RSC Adv., 2021,
11, 12802-12807
Synthesis and characterization of new fluorescent boro-β-carboline dyes†
Received
17th March 2021
, Accepted 24th March 2021
First published on 1st April 2021
Abstract
The first representatives of the new fluorescent boro-β-carboline family were synthesized by the insertion of the difluoroboranyl group into the oxaza or diaza core. The resulting compounds showed good photophysical properties with fine Stokes-shifts in the range of 38–85 nm with blue and green emission. The energetics of the excitation states and molecular orbitals of two members were investigated by quantum chemical computations suggesting effects for the improved properties of diazaborinino-carbolines over oxazaborolo-carbolines. These properties nominated this chemotype as a new fluorophore for the development of fluorescent probes. As an example, diazaborinino-carbolines were used for the specific labeling of anti-Her2 antibody trastuzumab. The fluorescent conjugate showed a high fluorophore-antibody ratio and was confirmed as a useful tool for labeling and confocal microscopy imaging of tumour cells in vitro together with the ex vivo two-photon microscopy imaging of tumour slices.
Introduction
Fluorophores or fluorescent probes are widely used tools in chemical biology for tagging proteins, cells or subcellular compartments in order to make the visualization of these species available. Although the development of synthetic fluorophores is important, Nature also gave us light absorbing and emitting tool compounds.1,2 A merged direction is the modification of natural compounds or their derivatives by turning them into efficient fluorophores. The β-carboline core is widely present in natural and synthetic biologically active compounds (Fig. 1).3 One of the most well-known families is represented by the harmala alkaloids4 found in a high number of plants. Harmaline (1), its several tryptamine analogue derivatives (2) and other serotonin (3) receptor agonists show fluorescence properties.5,6 Particularly, β-carboline (4) and methyl-β-carboline-3-carboxylate (5) are responsible for the blue fluorescent cuticle of two scorpion species. The latter (5) and its 3,4-dihydro derivative (6) can also be found in the human grey cataract.7 In addition, as close relatives, melatonin (7) analogues equipped with a difluoroboranyl group (8) were identified as fluorescent melatonin receptor agonists,8 showing a structural similarity to the widely used BODIPY (9).9
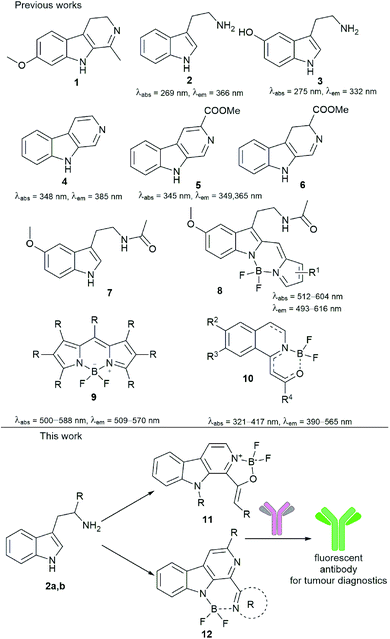 |
| Fig. 1 Biologically active and fluorescent compounds together with the aims of this work.18–20 | |
In continuation of our research on new β-carboline derivatives10–13 and new fluorescent agents,14 we aimed to synthesize β-carbolines coordinating the BF2 group either by two nitrogen atoms [like in BODIPY (9)] or a nitrogen and an oxygen [like boroisoquinolines(10)15,16] (Fig. 1). Finding a possible application for this new family of fluorophores we aimed to equip them with functionalities to label antibodies for tumour diagnostics and to investigate the conjugates in confocal and two-photon microscopy (Fig. 1).14,17
Results and discussion
After the successful synthesis of different boroisoquinolines15,16 we turned our attention to present a new fluorescent family based on the β-carboline core. Our design strategy was introducing the difluoroboranyl group between two heteroatoms (two nitrogens or a nitrogen and an oxygen) that was realized by the pyridoindole structure containing two nitrogens in rings B and C. Thus, we decided to synthesize members of two families, the oxazaborolo-carbolines (11) and the diazaborinino-carbolines (12) (Fig. 1).
Synthesis of oxazaborolo-carbolines (11)
For the synthesis of oxazaborolo-carbolines we used the natural product 1-formyl-β-carboline (13) (Kumujian C) synthesized in 5 steps using literature methods with good yields (61–95%), starting from tryptamine (2).21 Then Kumujian C has been transformed to 1-(ethyl-1-ol)-β-carbolines by Grignard reaction with methyl iodide, ethyl iodide, benzyl chloride and 4-methoxy benzyl-chloride resulting in 14a–d with various yields (35–77%) (Table 1). The next step was the oxidation of 14 alcohols to ketones (15) using activated manganese(IV)-oxide (MnO2) or Dess–Martin periodinane (DMP).
Table 1 Results of the synthesis of 1-(ethyl-1-ol)-β-carbolines (14), 15 ketones and 11 boro-β-carbolines
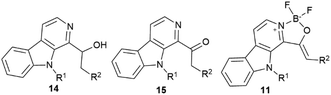
|
Entry |
Compound |
R1 |
R2 |
14 [%]a |
15 [%]a |
11 [%]a |
Isolated yields. Yields with Dess–Martin periodinane. |
1 |
a |
H |
H |
77 |
70 |
82 |
2 |
b |
H |
CH3 |
66 |
57 |
55 |
3 |
c |
H |
Ph |
73 |
46 |
— |
4 |
d |
H |
4-MeO-C6H4 |
35 |
52 |
— |
5 |
e |
CH3 |
H |
74 |
46b |
79 |
6 |
f |
CH3 |
CH3 |
72 |
50b |
92 |
In some cases, MnO2 led to significant degradation, but using DMP in milder conditions resulted in higher yields. Finally, we equipped the 15 keto-β-carbolines with the difluoroboranyl group using boron trifluoride diethyletherate in the presence of diisopropylethylamine (Scheme 1). These reactions showed good to excellent yields for the alkyl (methyl and ethyl, 15a and 15b) ketones, but in the case of benzyl derivatives (benzyl and 4-methoxybenzyl, 15c and 15d) no product was detected by HPLC-MS. Under harsher conditions (higher equivalent of BF3·OEt2, reflux) still the starting material was recovered that can be explained by the electron withdrawing character of the aromatic moiety abolishing the reactivity of the nitrogen. Next, we planned to increase the electron density for better fluorescence by the introduction of an electron donating group to the nitrogen atom of the pyrrole ring. Kumujian C (13) was methylated with MeI in the presence of sodium hydride with good yield (76%). The next borylating step leading to 11e and 11f oxazaborolo-carbolines have been performed similar to 11a and 11b and led to the products in 79% and 92% yields, respectively (Table 1).
 |
| Scheme 1 Synthesis of oxazaborolo-carbolines (11). | |
Synthesis of diazaborinino-carbolines (12)
The synthesis of diazaboranino-carbolines (12) started from tryptamine (2a) or tryptophan methyl ester (2b) (Scheme 2). In the case of 16a,b the crude product of the first Pictet–Spengler reaction was taken directly to dehydrogenation (Method A). The β-carboline core was formed with moderate yields. In the case of 16c,d the Pictet–Spengler reaction and the dehydrogenation was performed in one-pot reaction at 140 °C with a slightly longer reaction time, following the method of Singh et al. with modifications (Method B).22 Finally, the difluoroboranil group was incorporated to the structure by BF3·OEt2 in the presence of Na2CO3 at 85 °C leading to the desired heterocycles in acceptable to high yields (up to 95%) (Table 2). Notably, the imidazole-derivative (12a) gave the lowest yield that could be rationalized by its instability due to the decreased donor effect of the smaller aromatic ring to the N–B bond formation. In the final step, it was crucial to work under dry conditions. The work-up could be performed by a simple filtration through celite followed by chromatography.
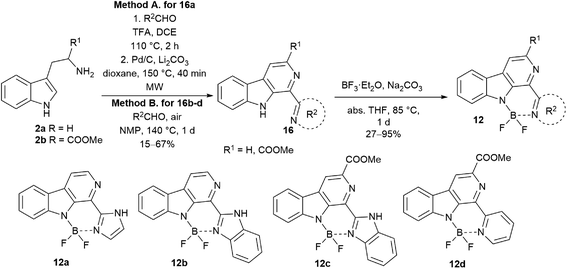 |
| Scheme 2 Synthesis of diazaborinino-carbolines (12a–d). | |
Table 2 Results of the synthesis of compounds 16a–d and 12a–d
Entry |
Compound |
R1 |
R2 |
16 [%]a |
12 [%]a |
Isolated yields. Imidazole-2-yl. Benzimidazole-2y-l. Pyridine-2-yl. Method B was applied. |
1 |
a |
H |
Imb |
49 |
27 |
2 |
b |
H |
Bimc |
33e |
95 |
3 |
c |
COOMe |
Bimc |
67e |
63 |
4 |
d |
COOMe |
Pyd |
15e |
74 |
Photophysical properties
After the synthesis of the borocarbolines (11a,b,e,f and 12a–d), we have investigated the absorption, excitation and emission spectra, molar absorption coefficients, quantum yields and brightness. Specific wavelengths are given in Table 3. The absorbance maxima are usually located in the range of near UV (363–396 nm for compounds 11a,b,e,f and 12a), but three derivatives goes up to low-vis (405–414 nm for compounds 12b–d). The emission maxima are found from blue light to the beginning of green light of the visible spectra (417–489 nm). The oxazaborolo-carbolines (11a,b,e,f) are shifted hypsochromic direction if we compare it with the diazaborinino-carbolines (12a–d). The Stokes shifts are usually in a range of 60 to 85 nm that can be considered as relatively large compared to the widely used BODIPY dyes (6–32 nm).
Table 3 Photophysical properties of oxazaborolo- and diazaborinino carbolines
# |
Compound |
R1 |
R2 |
λabsmax [nm] |
λexcmax [nm] |
λemmax [nm] |
Δλ [nm] |
ε (λabsmax) [M−1 cm−1] |
ΦF [−] |
B [M−1 cm−1] |
Imidazole-2-yl. Benzimidazole-2y-l. Pyridine-2-yl. |
1 |
11a |
H |
H |
375 |
378 |
450 |
75 |
506 |
0.49 |
248 |
2 |
11b |
H |
Me |
379 |
376 |
417 |
38 |
11 700 |
0.21 |
2460 |
3 |
11e |
Me |
H |
377 |
377 |
445 |
68 |
5150 |
0.0062 |
32 |
4 |
11f |
Me |
Me |
363 |
378 |
435 |
72 |
11 700 |
0.27 |
3160 |
5 |
12a |
H |
Ima |
396 |
380 |
472 |
76 |
3750 |
0.40 |
1500 |
6 |
12b |
H |
Bimb |
414 |
398 |
484 |
70 |
8860 |
0.45 |
3990 |
7 |
12c |
COOMe |
Bimb |
405 |
393 |
465 |
60 |
9320 |
0.47 |
4380 |
8 |
12d |
COOMe |
Pyc |
407 |
404 |
489 |
85 |
23 500 |
0.27 |
6360 |
The molar absorbance coefficient is generally lower for oxazaborolo-carbolines than the diazaborinino carbolines, and the same phenomenon can be observed regarding the quantum yields resulting in usually moderate brightness values. Outstanding compound is 12d borocarboline with good brightness (B = 6360 M−1 cm−1) and the largest Stokes shift (85 nm). We hypothesize that the lower flexibility of the core and less enhanced push–pull system are behind the weaker photophysical properties of oxazaborolo compounds.
Computed properties
The best members of each family were chosen for quantum chemical investigations. The optimal geometry conformers of compounds 11f and 12d were taken in S0 and S1 states, and transition energies were calculated. These calculations showed only small difference between the excitation and relaxation energy values (Fig. 2), just like in the energy of the band gap between the HOMO and LUMO orbitals (5.72 eV for 11f and 5.48 eV for 12d). The trends were the same, the less energy was in correspondence with the lower energy gap. Studying the change in the HOMO and LUMO energies upon excitation, it could be seen that for both compounds the LUMO level decreased similarly by 30–31 kJ mol−1, while the HOMO increased by 11f with 31.9 kJ mol−1, and by 12d with 16.1 kJ mol−1. These data suggest that the better fluorescence properties of 12d might be caused by the lower energy excited state, the lower HOMO–LUMO band gap and the lower excited state HOMO energy difference. Visualizing the molecular orbitals, many similar patterns could be observed between the HOMO and LUMO of 11f and 12d because of the common carboline core (Fig. 2). Interestingly, however, the participation of BF2 in the HOMO was larger in the structure of 12d while quite different conjugations could be observed in HOMO-1 orbital. This suggests that the vinylene group next to the oxazaborolidine ring has different effect than the pyridine ring connected to the carboline core. The vinylene group participates in the highest occupied orbitals and it is more distant from the BF2 group, while the pyiridine is directly connected participating only in HOMO. The atomic charges and the electron density was also investigated (Fig. 2). The partial negative charges are lower around BF2 of 12d, the electron density is more homogenous than that for 11f. In the latter the vinylene group acts as a borderline between the negatively and positively charged parts of 11f.
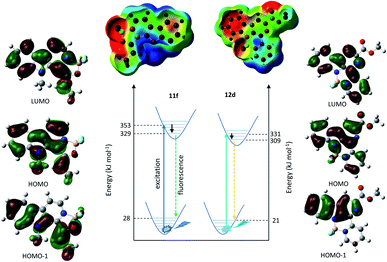 |
| Fig. 2 Schematic representation of the excitation and fluorescence with computed energetics together with the visualized molecular orbitals, atomic charges and electrostatic potential (ESP) surface for 11f and 12d. On the ESP surface charges change from red (negative) to blue (positive). The atomic charges change from red (negative) to green (positive). | |
Effects of solvents and pH
Next, the performance of the best of each fluorescent carboline families (11f and 12d) were investigated in different solvents. This solvent screen showed that the wavelength and intensity of emission of 11f shifts in batochromic direction with the increasing polarity of the solvent (e.g. in acetonitrile, EtOH or water, Fig. 3a). The intensity is the greatest in acetonitrile, but in water we still have decent emission, and the emission maximum is above 450 nm. In the case of 12d the intensity decreased as we headed towards more polar solvents, but except of water the emission maximum did not change significantly (Fig. 3b). Notably, in water, the emission maxima shifted above 500 nm. The fluorescence of 12d did not change significantly in the pH range of 4.4–8.4 (Fig. S9†).
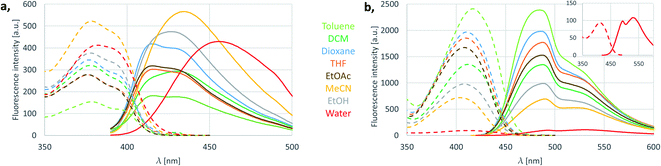 |
| Fig. 3 Solvent effect on excitation and emission spectra of 11f oxazaborolo-carboline (a) and 12d diazaborinino-carboline (b). | |
Stability
Finally, to prove the ability for biological application, we have investigated the photostability of 11f and 12d in acetonitrile, excited with UV light (8 W, 366 nm). The half of the original fluorescence intensity was reached after 1 h irradiation by 11f, but on the contrary, that did not decrease significantly after 1 h for 12d (Fig. 4). We also followed the changes in 10% serum containing DMEM cell media and found 12d stabile over 24 hours (Fig. S10†). In addition, 12d did not show cytotoxicity and neither anti-proliferative activity by the treatment of MRC-5 human lung fibroblast cell line for 24 h and 72 h, respectively (Tables S1 and S2, Fig. S11 and S12†).
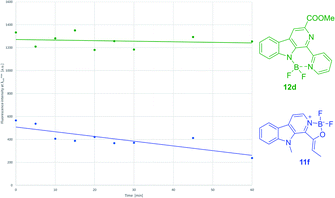 |
| Fig. 4 Photostability of 11f and 12d carbolines. | |
These results show that both compounds are promising candidates for chemical biology applications, considering that the excitation during imaging procedures usually does not take more than dozens of minutes. Due to its easy coupling and beneficial photophysical properties we selected 12d diazaborinino-carboline for antibody labelling experiments. Since antibodies are target cancer cells selectively, and deliver their fluorescent23 or cytotoxic payloads24 specifically, we have conjugated 12d to the human IgG trastuzumab creating a potential diagnostical tool for imaging Her2 positive tumour cells (Scheme 3a).25
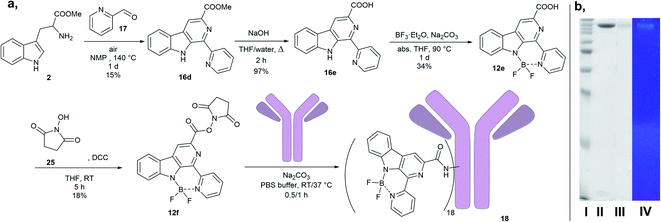 |
| Scheme 3 (a) Synthesis of 12f dye with NHS ester group and antibody-fluorophore conjugate 18; (b) SDS-PAGE in non-reducing gel: (I) molecular weight protein marker (kDa), (II) trastuzumab, (III) 18, (IV) 18 under 366 nm light. | |
Synthesis and biological evaluation of trastuzumab conjugate 18
We hydrolysed 16d ester with sodium hydroxide, followed by the incorporation of the difluoroboranil group. Then carboxylic acid 12e was transformed to 12f NHS ester. Reverse-phase chromatography was necessary for purification that decreased the isolated yields unexpectedly after full conversion. The NHS-ester (12f) was able to acylate the free lysines on the antibody (Scheme 3a) leading to a conjugate (18).26 The conjugate was analysed by SDS-PAGE to confirm the attachment of the fluorophores (Scheme 3b), and the fluorophore-antibody ratio (FAR) was determined by UV-VIS absorbance measurement to be FAR = 18.
Having the antibody-dye conjugate in hand, three types of tumour cell lines were treated (Fig. 5A). The animals were anaesthetized, and the tumours were resected and fixed. The 100 μm thick slices were treated with 18 for 24 h. No specific signal could be detected in cells of the epithelial human breast cancer cell line MDA-MB-231 negative for Her2 by confocal microscopy (Fig. 5A left). The cell membrane of the OVCAR-8, an ovarian carcinoma cell line slightly positive for Her2, tumour cells emitted a slight signal (Fig. 5A middle), whereas SKOV-3 an ovarian cell line expressing high amounts of Her2 showed a stronger signal (Fig. 5A right). For the two photon imaging tumours were resected from the anaesthetised animals, and 200 μm thick slices were cut. The living tumour tissue was incubated with 18 at 35 °C for 1 h. The fluorescent image demonstrated that 18 antibody was bound to the cells of the SKOV-3 tumours (Fig. 5B).
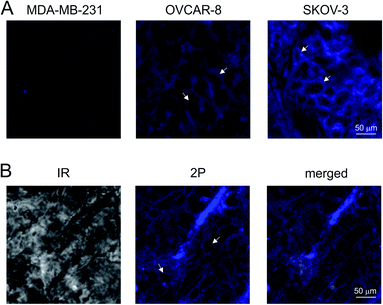 |
| Fig. 5 (A) Confocal images showing the BK-bound trastuzumab antibody (18) on MDA-MB-231 (left), OVCAR-8 (middle) and SKOV-3 (right) tumour sections. Note that only the cell membrane is marked, therefore cells appear as circles (white arrows). Scale bar: 100 μm. (B) Two-photon microscopy images of living tumour tissue incubated with the BK-bound trastuzumab antibody. Infrared image (IR, left) shows the tumour tissue, fluorescent image (middle) demonstrates that cells of the SKOV-3 tumours bound the antibody. Note that the fluorescent image is coloured artificially. Connective tissue surrounding the groups of tumour cells are autofluorescent. Scale bar: 50 μm. | |
Ethical statement
All animal experiments will be conducted following standards and procedures approved by the Animal Care and Use Committee of the National Institute of Oncology, Budapest (license number: PEI/001/2574-6/2015).
Conclusions
We have synthesized new families of boro-β-carbolines including oxazaborolo-carbolines and the diazaborinino-carbolines. Their photophysical analysis showed that the compounds can be excited in the 370–405 nm range, and emitting up to 489 nm. Stokes-shifts are greater than those of the BODIPY derivatives indicating their potential in chemical biology applications. Brightness data are acceptable and photostability was impressive by the best member of the diazaborinino-carbolines. The energetics of excitation states and molecular orbitals of two representatives were investigated by quantum chemical computations suggesting effects for the improved properties of diazaborinino-carbolines over oxazaborolo-carbolines. The best diazaborinino-carboline (12d) was further functionalized to an NHS-ester (12f) that was successfully used for antibody labelling and visualizing Her2 positive tumour cells in confocal and two-photon microscopy.
Conflicts of interest
There are no conflicts to declare.
Acknowledgements
Supported by the 2018-1.3.1-VKE-2018-00032 and Hungarian Thematic Excellence Programme TKP2020-NKA-26 grant of the National Office of Science, Innovation and Technology (NKFIH). We are grateful for the support of NKFIH PD124598 and ÚNKP-19-3-I-BME-408 New National Excellence Program of the Ministry for Innovation and Technology. The authors acknowledge the supportive work of Krisztina Németh.
Notes and references
- A. J. Singh, A. P. Gorka, H. R. Bokesch, A. Wamiru, B. R. O'Keefe, M. J. Schnermann and K. R. Gustafson, J. Nat. Prod., 2018, 81, 2750–2755 CrossRef CAS PubMed.
- R. Duval and C. Duplais, Nat. Prod. Rep., 2017, 34, 161–193 RSC.
- E. V. Costa, M. L. B. Pinheiro, C. M. Xavier, J. R. A. Silva, A. C. F. Amaral, A. D. L. Souza, A. Barison, F. R. Campos, A. G. Ferreira, G. M. C. Machado and L. L. P. Leon, J. Nat. Prod., 2006, 69, 292–294 CrossRef CAS PubMed.
- J. S. Glasby, Encyclopedia of the Alkaloids, Springer US, Boston, MA, 1983 Search PubMed.
- R. A. Glennon, M. Dukat, B. Grella, S.-S. Hong, L. Costantino, M. Teitler, C. Smith, C. Egan, K. Davis and M. V. Mattson, Drug Alcohol Depend., 2000, 60, 121–132 CrossRef CAS.
- J. Hidalgo, C. Carmona, M. Balón and M. A. Muñoz, Pharm. Weekbl., Sci. Ed., 1990, 12, 142–144 CrossRef CAS PubMed.
- S. J. Stachel, S. A. Stockwell and D. L. Van Vranken, Chem. Biol., 1999, 6, 531–539 CrossRef CAS PubMed.
- J. Thireau, J. Marteaux, P. Delagrange, F. Lefoulon, L. Dufourny, G. Guillaumet and F. Suzenet, ACS Med. Chem. Lett., 2014, 5, 158–161 CrossRef CAS PubMed.
- A. Loudet and K. Burgess, Chem. Rev., 2007, 107, 4891–4932 CrossRef CAS PubMed.
- M. Milen, P. Abranyi-Balogh, Z. Mucsi, A. Dancso, D. Frigyes, L. Pongo and G. Keglevich, Curr. Org. Chem., 2013, 17, 1894–1902 CrossRef CAS.
- M. Milen, P. Abranyi-Balogh, Z. Mucsi, A. Dancso, T. Kortvelyesi and G. Keglevich, Curr. Org. Chem., 2011, 15, 1811–1825 CrossRef CAS.
- P. Ábrányi-Balogh, T. Földesi, A. Grün, B. Volk, G. Keglevich and M. Milen, Tetrahedron Lett., 2016, 57, 1953–1957 CrossRef.
- M. Milen, P. Slégel, P. Keglevich, G. Keglevich, G. Simig and B. Volk, Tetrahedron Lett., 2015, 56, 5697–5700 CrossRef CAS.
- L. Petri, P. A. Szijj, Á. Kelemen, T. Imre, Á. Gömöry, M. T. W. Lee, K. Hegedűs, P. Ábrányi-Balogh, V. Chudasama and G. M. Keserű, RSC Adv., 2020, 10, 14928–14936 RSC.
- D. Sóvári, A. Kormos, O. Demeter, A. Dancsó, G. M. Keserű, M. Milen and P. Ábrányi-Balogh, RSC Adv., 2018, 8, 38598–38605 RSC.
- D. Sóvári, G. M. Keserű and P. Ábrányi-Balogh, Materials, 2020, 13, 199 CrossRef PubMed.
- J. M. Warram, E. de Boer, A. G. Sorace, T. K. Chung, H. Kim, R. G. Pleijhuis, G. M. van Dam and E. L. Rosenthal, Cancer Metastasis Rev., 2014, 33, 809–822 CrossRef CAS PubMed.
- A. Chattopadhyay, R. Rukmini and S. Mukherjee, Biophys. J., 1996, 71, 1952–1960 CrossRef CAS PubMed.
- D. Reyman, M. J. Tapia, C. Carcedo and M. H. Viñas, Biophys. Chem., 2003, 104, 683–696 CrossRef CAS PubMed.
- A. Mallick, P. Das and N. Chattopadhyay, J. Photochem. Photobiol., C, 2010, 11, 62–72 CrossRef CAS.
- T. Szabó, V. Hazai, B. Volk, G. Simig and M. Milen, Tetrahedron Lett., 2019, 60, 1471–1475 CrossRef.
- V. Kumar, D. Singh, A. K. Paul, R. Shrivastava and V. Singh, New J. Chem., 2019, 43, 18304–18315 RSC.
- J. M. Warram, E. de Boer, A. G. Sorace, T. K. Chung, H. Kim, R. G. Pleijhuis, G. M. van Dam and E. L. Rosenthal, Cancer Metastasis Rev., 2014, 33, 809–822 CrossRef CAS PubMed.
- P. Khongorzul, C. J. Ling, F. U. Khan, A. U. Ihsan and J. Zhang, Mol. Cancer Res., 2020, 18, 3–19 CrossRef CAS PubMed.
- T. A. D. Smith, Br. J. Radiol., 2010, 83, 638–644 CrossRef CAS PubMed.
- C. Martin, G. Brachet, C. Colas, E. Allard-Vannier, C. Kizlik-Masson, C. Esnault, R. Respaud, C. Denevault-Sabourin, I. Chourpa, V. Gouilleux-Gruart, M.-C. Viaud-Massuard and N. Joubert, Pharmaceuticals, 2019, 12, 176 CrossRef CAS PubMed.
Footnote |
† Electronic supplementary information (ESI) available: For experimental procedures and compound characterization. See DOI: 10.1039/d1ra02132j |
|
This journal is © The Royal Society of Chemistry 2021 |
Click here to see how this site uses Cookies. View our privacy policy here.