DOI:
10.1039/D1RA03268B
(Paper)
RSC Adv., 2021,
11, 34820-34827
Novel potentiometric sensors for determination of ondansetron hydrochloride in pure and dosage form
Received
27th April 2021
, Accepted 7th October 2021
First published on 27th October 2021
Abstract
A new and sensitive potentiometric method has been developed and characterized for four novel sensors responsive to ondansetron hydrochloride. The potentiometric sensor method includes advancement of ondansetron hydrochloride sensors using a membrane comprised of molybdophosphoric acid (MPA) and ondansetron as an electro-active material in a polyvinylchloride (PVC) matrix membrane plasticized with di-butyl phthalate (DBPH), ortho-nitrophenyloctyl ether (O-NPOE), di-octyl phthalate (DOPH), or di-butyl phosphate (DBP). The validity of sensors in the present work has been examined, and steady and reproducible responses were obtained over the concentration ranges of 7.3 × 10−5 to 1.0 × 10−2, 6.6 × 10−6 to 1.0 × 10−2, 1.0 × 10−5 to 1.0 × 10−2, and 2.0 × 10−5 to 1.0 × 10−2 M for DBPH-, O-NPOE-, DOPH-, and DBP-ondansetron, respectively. The sensors revealed Nernstian gradients of 59.61 ± 0.50, 57.71 ± 0.23, 53.01 ± 0.14, and 53.20 ± 0.35 mV per decade individually with pH ranges of 2.5–5.5 in DBPH and 3.5–5.0 in O-NPOE electrodes, and 4.0–5.5 for both individual DOPH and DBP plasticized film-based sensors. The time responses for the sensors were 30, 32, 31, and 29 s for DBPH-, O-NPOE-, DOPH-, and DBP-ondansetron, respectively. The developed sensors also exhibited high selectivity towards ondansetron hydrochloride against different interfering species of inorganic particles with long-term stability of approximately 41, 36, 18, and multiple days for the DBPH, O-NPOE, DOPH, and DBP electrodes.
1. Introduction
The structure of ondansetron hydrochloride (ODH) is (RS)-9-methyl-3-[(2-methyl-1H-imidazole-1-yl)methyl]-1,2,3,9-tetrahydro-4H-carbazol-4-one hydrochloride dihydrate1 (Fig. 1), and it is a basic compound with a pKa of 7.7. It is a specific serotonin 5-HT3 receptor antagonist that is used to treat nausea and vomiting caused by cancer chemotherapy and radiotherapy. It has also been shown to have neuroleptic and anti-anxiety characteristics.2,3 For anti-anxiety, the normal dosage is 8–32 mg per day, and for anxiety disorder, the initial results from clinical recommendations suggested lower levels of 2–4 mg per day.3
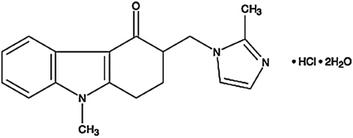 |
| Fig. 1 Structure of ondansetron hydrochloride. | |
Over time, methods have been developed for the determination of ondansetron by liquid chromatography (LC),4,5 high-performance liquid chromatography (HPLC),6,7 ion-pair chromatography,8 liquid chromatography mass spectrometry (LC-MS),9,10 capillary zone electrophoresis,11,12 flow injection atomic absorption spectroscopy, and ultraviolet (UV) spectrophotometry.13–15 Ninama et al. improved and validated a mass spectrometry method for concentrations of 1.00–100.00 ng mL−1 with a lower limit of quantitation (LLOQ) at approximately 1.00 ng mL−1, and shorter stability time for analysis of ondansetron solutions at approximately 7 h 10 min. This process is long and time consuming for routine regulatory analysis of the quality of pharmaceutical dosage forms.16 However, Farnoush et al. introduced a reliable and rapid potentiometric sensor for ondansetron with an acceptable Nernstian slope of 58 ± 0.3 mV, and the author also employed the sensor in ionic liquid solvents, such as 1-butyl-3-methylimidazolium tetrafluoroborate ([BMIM][BF4]).17 A further important issue with the use of [BMIM][BF4] is that the liquid junction potential (Ej) could possibly accrue when different solutions are used due to different mobility of ions.18
The drawbacks of the above studies have been the included number of complex processes, limited applications, and required derivatization responses for measuring ondansetron. Another challenge is that there are few existing analytical methods with sensors of sufficient sensitivity that can be used to measure ondansetron in bioequivalence studies.19 A new approach is therefore required to overcome the weaknesses of published methods and devise accurate and sensitive techniques.
Thus, the overall goal of the current study was to improve a new version of potentiometric polymer matrix membrane sensors so that they were sufficiently accurate to measure trace amounts of ondansetron. The sensors successfully measured ondansetron in a bioequivalence pharmaceutical form, which was achieved using polyvinylchloride (PVC) as a matrix membrane plasticized with electro-active materials acting as ion pair complexes with either dibutyl phthalate, o-nitrophenyl octylether, di-octyl phthalate, or di-butyl phosphate in molybdophosphoric acid ondansetron.
2. Experimental
2.1. Chemical preparations
All analytical reagents were prepared in deionised water. Molybdophosphoric acid (MPA) and tetrahydrofuran (THF) were purchased from Aldrich and used without further purification. Polyvinylchloride (PVC) Breon S110/10 was purchased from B.P. Chemical UK, Ltd, whilst o-nitrophenyl octyl ether, di-butyl phthalate, di-octyl phthalate, and di-butyl phosphate were purchased from Fluka and BDH. Pure ondansetron hydrochloride was supplied by the State Company of Drug Industries and Medical Appliances (IRAQ-SDI, Samara). The formulated ondansetron hydrochloride tablets used were Zofran, 4 mg, manufactured in Singapore, and Zofran, 8 mg, manufactured in Egypt.
2.2. Instruments and analytical conditions
All electrochemical measurements were carried out at room temperature (25 ± 1 °C) with a digital pH meter (WTW model PH720, Germany) utilizing an MPA-ondansetron PVC matrix membrane sensor with calomel reference electrodes (Gallenkamp, USA). A reference electrode of glass/Ag AgCl was constructed for pH estimates. The ion-selective electrode contained an inner solution of 0.01 M of ondansetron solution.
2.3. Preparation of the PVC sensor membrane
The ion pair system was formed by 100 mL of 1.0 × 10−1 M ODH solution and 100 mL of 1.0 × 10−1 M aqueous MPA. The resulting precipitate was filtered and washed with distilled water, and then left to dry. The film (membrane) was prepared by dissolving 0.170 mg of powdered PVC, 0.40 mg of plasticizer, and 0.040 mg of the ion pair in 7 mL THF. The solvent was added to a Petri dish (7 cm in diameter), and then covered with filter paper and allowed to gradually evaporate at room temperature. A punched-out circular piece of film (membrane) was connected to a polyethylene tube with a diameter of approximately 8 mm in an electrode construction.20,21 A 1.0 × 10−2 M ODH solution was used as the interior solution in the Ag/AgCl reference electrode. The constructed electrode was formulated and soaked for 24 h in 1.0 × 10−2 M ODH solution, with subsequent storage in a similar solution. The electrochemical cell for the ion-selective electrode (ISE) system used in this study is shown in Fig. 2.
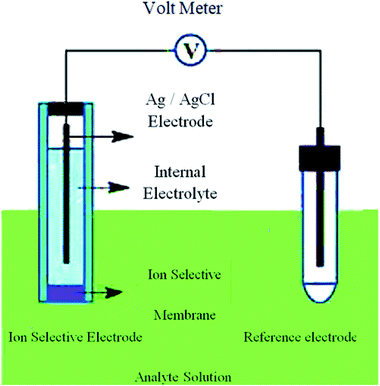 |
| Fig. 2 Ion-selective electrodes in an electro-analytical cell. | |
2.4. Calibration of the sensors
The standardization of the sensors was tested by immersing the sensor into 50 mL-beakers containing 1.0 × 10−6 to 1.0 × 10−2 M ondansetron solutions. The potential was verified by plotting as a function of the logarithm of the concentration of ondansetron. The associated calibration curve was subsequently used to determine unknown ondansetron concentrations.
2.5. Determination of the selectivity coefficient
The selectivity coefficient (KpotA,B) was determined for the different electrodes by applying the matched potential method (MPM). In the MPM method, different interfering ions are added to a reference solution until the same electrode potential is recorded as that with a particular activity of elementary ions in a separate experiment. The KMPM assumption was measured to calculate the selectivity coefficient for each interference ion from the ratio of the primary ion to interfering ion activity, as indicated in eqn (1) below:where the activity of primary solution a2 and a1 is the activity of the reference solution, and aB denotes the interfering concentration that causes the same ΔE.19
3. Results and discussion
3.1. Characterization of the sensor
We devised a specific method of preparing plasticized PVC matrix membrane sensors using MPA-ODH with suitable ionophores. Four types of ion-selective plasticizer membranes were formed to increase the diffusional mobility of the analysts and electroactive complex. A detailed standard protocol was investigated for applying the electrochemical technique to assess the ondansetron sensors. The response to the Nernst equation with respect to the potential-defining spaces in the solution was also considered. In this study, the sensor was activated before use by soaking in ondansetron solution for approximately two days. A series of 7.3 × 10−5 to 1.0 × 10−2, 6.6 × 10−6 to 1.0 × 10−2, 1.0 × 10−5 to 1.0 × 10−2, and 2.0 × 10−5 to 1.0 × 10−2 M solutions for DBPH-, O-NPOE-, DOPH-, and DBP-ondansetron anodes was prepared to study the response of the electrode as a function of nominal Nernstian behavior that remained similar to the standard conditions.19
The data in Table 1 show an accurate response and high reproducibility for the O-NPOE, DBPH, DOPH, and DBP films. The slope of the graph was 59.61 ± 0.50 for DBPH, while the other slopes slightly decreased to 57.71 ± 0.23, 53.01 ± 0.14, and 53.20 ± 0.35 mV per decade for DBPH, DOPH, and DBP, respectively. It is clear that the four types provide a linear response with a satisfactory lifetime. These sensors could, however, provide an analytical signal for measuring ondansetron hydrochloride.
Table 1 The value of parameters for ondansetron hydrochloride-selective electrodes
Parameters |
Electrode 1 DBPH |
Electrode 2 NPOE |
Electrode 3 DOPH |
Electrode 4 DBP |
Slope (mV per decade) |
59.61 ± 0.50 and |
57.71 ± 0.23 |
53.01 ± 0.14 |
53.20 ± 0.35 |
Limit of detection (M) |
3.00 × 10−6 |
2.53 × 10−6 |
4.50 × 10−6 |
8.10 × 10−6 |
Correlation coefficient |
0.9911 |
0.9985 |
0.9915 |
0.9962 |
Linear range (mol L−1) |
7.3 × 10−5 to 1.0 × 10−2 |
6.6 × 10−6 to 1.0 × 10−2 |
1.0 × 10−5 to 1.0 × 10−2 |
2.0 × 10−5 to 1.0 × 10−2 |
pH range |
2.5–5.5 |
3.5–5.0 |
4.0–5.5 |
4.0–5.5 |
Time (s) |
30 |
32 |
31 |
29 |
Regression equation, Y = mX + b |
Y = 25.884 ln(x) + 314.1 |
Y = 25.0594 ln(x) + 303.2 |
Y = 23.018 ln(x) + 294 |
Y = 23.1044 ln(x) + 290.7 |
Lifetime (day) |
41 |
36 |
18 |
20 |
3.2. Effect of time
Time response is an important factor for use in ion-selective electrodes, and it is usually dependent on the time required for the system to reach equilibrium. An interesting observation was the stability of the potential, and chemical equilibrium in 10−3 M ondansetron solution resulted in 30, 32, 31, and 29 seconds for sensors O-NPOE, DBPH, DOPH, and DBP, respectively. The response of the electrode can disrupt the non-linearity, and this error could decrease the efficiency of the electrode. In this case, the potential at equilibrium was less stable, and the sensor could display uncertainty in the linear response to the Nernst equation. In some cases, it was found that uncertainty was recorded for potentiometric titration of the order of potential measurements, and these differences were approximately ±5 mV e.m.f.20 In a study for measuring metoclopramide hydrochloride by PVC using a similar apparatus, the authors noted that the PVC membrane produced linear responses to the Nernst equation, while the day-to-day reproducibility of the sensor showed a decrease in the potential measurements of approximately ±0.5 mV for the same solutions.20
The data in Fig. 3 show that the change in the potential for the four electrodes in different concentrations of ondansetron solutions is in satisfactory agreement with the Nernst equation. However, the four electrodes gave slight deviations in analytical signals above 0.01 M. Previously, it has been shown that accurate measurements above 0.01 M could be clearly obtained when the reaction mixture was heated to 60 °C. This was due to reaching the optimum reaction time for the charge-transfer complexation to occur between ondansetron base as an n-electron donor with chloranil as a π-acceptor to produce a colored complex.21 However, the quantitation of ODH in all four prepared electrodes at a concentration range lower than 10−5 M could not be feasible without a fitting of accumulation of transport ions, which would minimize the ion exchange of the ion-pair with competing endogenous ions.22
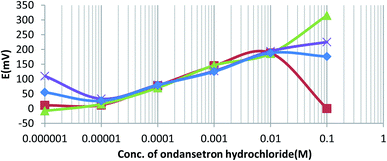 |
| Fig. 3 Potentiometric response of ondansetron PVC matrix membrane sensors. | |
All prepared sensors were in good agreement with the life expectancy values of 41, 36, 18, and 20 days for the O-NPOE-, DBPH-, DOPH-, and DBP-based membrane sensors, respectively. Therefore, in most cases, it is assumed that the differences between the responses of the four membranes are dependent on the filtering of the ion pair from the membranes.6
Fig. 4a and b shows the characterization of ondansetron with ion pair and pure drug formulation. The graph is split into two parts, and they indicate wavelength measurements of the ion pair productions. The data are similar to those expected from the previous study showing the functional groups for ondansetron with an ion pair. The Fourier transform infrared (FTIR) spectroscopy results for ondansetron show intense bands at 3483.41 cm−1, 2910.11 cm−1, and 1638.7 cm−1, which correspond to the presence of the NH group, C–C-aromatic group, and C–C-aliphatic group, respectively.23
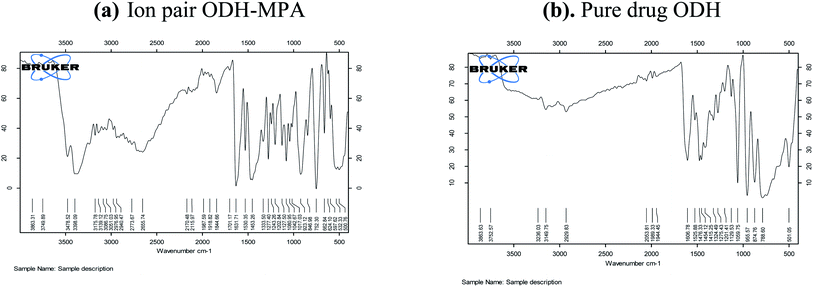 |
| Fig. 4 FTIR spectrum of (a) ion pair ODH-MPA and (b) pure drug ODH. | |
3.3. Effect of pH
The performances of the membranes in electrodes mostly depend on the potentiometric responses. One of the important factors is the presence of hydrogen ion. To further understand the potentiometric responses of the O-NPOE-, DBPH-, DOPH-, and DBP-based membrane sensors, they were tested with the standard addition of 10−3 M ondansetron solution in a pH range of 1.2–12.4, as shown in Table 2. An adjustment of pH was achieved using diluted solutions of either sodium hydroxide or hydrochloric acid. Fig. 5 shows that the pH of 2.5–5.5 for DBPH, 3.5–5.5 for NPOE, and 4.0–5.5 for DOPH and DBP did not significantly affect the sensor response. A much slower response of the membrane was obtained at pH < 2.00. This could be attributed to competitive binding of hydrogen ion at the surface of the membrane, while at pH > 6, the electrode function remained unaltered with a slight change in pH due to progressive precipitation of high alkali solution.24
Table 2 pH range for ondansetron hydrochloride electrodes
Electrode no. |
Composition |
Range of pH |
1 |
ODH-MPA-DBPH |
2.5–5.5 |
2 |
ODH-MPA-NPOE |
3.5–5.0 |
3 |
ODH-MPA-DOPH |
4.0–5.5 |
4 |
ODH-MPA-DBP |
4.0–5.5 |
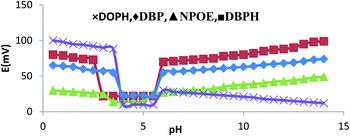 |
| Fig. 5 pH range for ODH electrodes in 10−3 M ondansetron hydrochloride solution. | |
3.4. Effect of interfering ions
Having shown that the presence of ion pairs in the membrane increases the selectivity of the electrode and enables quantification of the ondansetron solutions, the next step was to examine the influences in different inorganic anions. The selectivity coefficients (kpotODH,B) are representative of the activity ratio of primary and interfering ions. They are also an essential parameter that refers to the operability of the electrodes, as it is generally known that high selectivity values refer to the high free ions that confer a smaller effect than the other interfering ions in the solution. Table 3 shows that the results are logical given the acceptable selectivity for ondansetron solutions in the presence of numerous inorganic anions.25,26
Table 3 Selectivity coefficient value for different interfering ions using (ODH-MPA) electrodes
KA,B calculation for 10−3 M ODH solution |
Ion interference |
Electrode 1 |
Electrode 2 |
Electrode 3 |
Electrode 4 |
Li1+ |
2.0954 × 10−2 |
4.9168 × 10−2 |
9.1845 × 10−2 |
6.0186 × 10−2 |
Na1+ |
6.9934 × 10−2 |
5.6210 × 10−2 |
7.9220 × 10−2 |
5.3314 × 10−2 |
K1+ |
3.3789 × 10−2 |
1.1897 × 10−2 |
6.6543 × 10−2 |
8.0267 × 10−2 |
Ca2+ |
8.5077 × 10−3 |
8.4398 × 10−3 |
8.0300 × 10−3 |
1.1125 × 10−3 |
Mg2+ |
5.8124 × 10−3 |
5.9855 × 10−3 |
2.2346 × 10−3 |
3.4676 × 10−3 |
Zn2+ |
1.6589 × 10−3 |
4.5783 × 10−3 |
7.7865 × 10−3 |
3.5654 × 10−3 |
Cr3+ |
4.4532 × 10−4 |
6.8754 × 10−4 |
7.7589 × 10−4 |
3.5435 × 10−4 |
Fe3+ |
5.6330 × 10−4 |
7.6540 × 10−4 |
5.1034 × 10−4 |
2.0147 × 10−4 |
Al3+ |
9.1044 × 10−4 |
6.0366 × 10−4 |
6.9305 × 10−4 |
8.0509 × 10−4 |
3.5. Measuring the pharmaceutical form of ondansetron
Tests were performed to ensure that the electrodes were valid for measuring ondansetron. All electrodes underwent the direct, standard (SAM), and multi-standard potentiometric method (SAMS) to determine the concentration of 10−4 M and 10−3 M ondansetron solutions for all electrodes. These data are shown in Table 6, and in all cases, it was assumed that there was considerably high precision and linearity with a low detection limit for all values obtained using the potentiometric titration method for all plasticizer-based membranes, and they emphasize the improved performances of the electrodes, as summarized in Tables 4–7. The performances of the prepared membrane electrodes were similar to those previously mentioned in the literature. In the same context, a study by Nigović examined the electrochemical behavior of ondansetron on the multi-walled carbon nanotubes of a Nafion polymer composite in a glassy carbon electrode.27 The quantification of ondansetron concentrations in coated tablets was reported as electrochemical data.
Table 4 Potentiometric methods for ODH-MPA-DBPH electrodes in 10−3 and 10−4 M ondansetron solutions
Sample |
Potentiometric methods for ODH-MPA-DBPH electrodes in 10−3 and 10−4 M ondansetron solutions |
Direct |
SAM |
SAMS |
Titration |
RSD represents the average of five different determinations. |
1 × 10−3 (M) |
0.9596 × 10−3 |
0.9762 × 10−3 |
0.9817 × 10−3 |
0.9833 × 10−3 |
Relative standard deviation (RSD)a (%) |
1.28 |
1.59 |
— |
— |
Recovery (rec) (%) |
95.96 |
97.62 |
98.17 |
98.33 |
Relative error (RE) (%) |
−4.04 |
−2.38 |
−1.90 |
−1.67 |
1 × 10−4 M |
0.96 50 × 10−4 |
0.9790 × 10−4 |
0.9798 × 10−4 |
0.9848 × 10−4 |
RSDa (%) |
3.12 |
0.46 |
— |
— |
Recovery (%) |
96.50 |
97.90 |
97.98 |
98.48 |
Relative error (%) |
−3.12 |
−2.10 |
−2.02 |
−1.52 |
Table 5 Potentiometric methods for ODH-MPA-NPOE electrodes in 10−3 and 10−4 M ondansetron solutions
Sample |
Potentiometric methods for ODH-MPA-NPOE electrodes in 10−3 and 10−4 M ondansetron solutions |
Direct |
SAM |
SAMS |
Titration |
1 × 10−3 |
0.9533 × 10−3 |
0.9870 × 10−3 |
0.9814 × 10−3 |
0.9839 × 10−3 |
RSD (%) |
1.64 |
1.06 |
— |
— |
Rec (%) |
95.33 |
98.70 |
98.14 |
98.39 |
RE (%) |
−4.67 |
−1.30 |
−1.86 |
−1.61 |
1 × 10−4 |
0.9482 × 10−4 |
0.9817 × 10−4 |
0.9860 × 10−4 |
0.9877 × 10−4 |
RSD (%) |
4.92 |
1.36 |
— |
— |
Rec (%) |
94.82 |
98.17 |
98.60 |
98.77 |
RE (%) |
−5.18 |
−1.83 |
−1.40 |
−1.23 |
Table 6 Potentiometric methods for ODH-MPA-DOPH electrodes in 10−3 and 10−4 M of ondansetron solutions
Sample |
Potentiometric methods for ODH-MPA-DOPH electrodes in 10−3 and 10−4 M ondansetron solutions |
Direct |
SAM |
SAMS |
Titration |
1 × 10−3 |
0.9538 × 10−3 |
0.9767 × 10−3 |
0.9733 × 10−3 |
0.98100 × 10−3 |
RSD (%) |
3.72 |
1.62 |
— |
— |
Rec (%) |
95.38 |
97.67 |
97.33 |
98.10 |
RE (%) |
−4.62 |
−1.62 |
−2.67 |
−1.90 |
1 × 10−4 |
0.9582 × 10−4 |
0.9841 × 10−4 |
0.9630 × 10−4 |
0.9857 × 10−4 |
RSD (%) |
3.24 |
1.04 |
— |
— |
Rec (%) |
95.82 |
98.41 |
96.30 |
98.57 |
RE (%) |
−4.18 |
−1.59 |
−3.70 |
−1.34 |
Table 7 Results for potentiometric methods for ODH-MPA-DOP electrodes in 10−3 and 10−4 M ondansetron solutions
Sample |
Potentiometric methods for ODH-MPA-DOP electrodes in 10−3 and 10−4 M ondansetron solutions |
Direct |
SAM |
SAMS |
Titration |
1 × 10−3 |
0.9687 × 10−3 |
0.9818 × 10−3 |
0.9704 × 10−3 |
0.9823 × 10−3 |
RSD (%) |
3.13 |
1.07 |
— |
— |
Rec (%) |
96.87 |
98.18 |
97.04 |
98.23 |
RE (%) |
−3.13 |
−1.82 |
−2.96 |
−1.77 |
1 × 10−4 |
0.9721 × 10−4 |
0.9680 × 10−4 |
0.9852 × 10−4 |
0.9855 × 10−4 |
RSD (%) |
2.14 |
2.48 |
— |
— |
Rec (%) |
97.21 |
96.80 |
98.52 |
98.55 |
RE (%) |
−2.14 |
−3.2 |
−1.48 |
−1.45 |
Recovery studies of drugs in serum samples were performed using the standard addition method, with 98.77% recovery of ondansetron as protonated drug molecules on a Nafion surface matrix.27 Another comparable study by Ninama et al. measured ODH concentration using the solid-phase extraction chromatography-mass spectrometry method.16 The work reported that the percent accuracy of samples prepared with different biological matrixes was found to be 91.88%, and wet extract stability was found to be 43 h 30 min at 5 ± 3 °C.16
For the current method, the recovery measurements for prepared electrodes were approximately 97.90% using the standard addition method. The precision and accuracy of the method expressed as recovery% was found to be approximately 2.1%.9 The results obtained were within the acceptable range and were higher than those observed in the biological matrix.16 The values are also in accordance with the effective accuracy measurements of ODH solutions, as shown in Table 4.
Additional tests were performed after the application of the improved electrodes in pure solutions of ondansetron. The plasticized PVC matrix membranes were used to measure pharmaceutical preparations, and the data are shown in Table 8. It was noted that all electrodes were able to adequately measure the concentration of ondansetron, and these data indicate that the proposed potentiometric method is applicable for quality control of medicinal preparations.28
Table 8 Application of ODH electrodes to measure ondansetron dosage
Direct potentiometric methods at 1.00 × 10−3 M ODH |
Zofran tablet – ondansetron hydrochloride, 8 mg |
Electrode 1 |
Electrode 2 |
Electrode 3 |
Electrode 4 |
Concentration of ODH (M) |
0.9761 × 10−3 |
9.7443 × 10−3 |
9.6535 × 10−3 |
9.5815 × 10−3 |
Rec (%) |
97.61 |
97.44 |
96.53 |
95.81 |
RE (%) |
−2.39 |
−2.56 |
−3.47 |
−4.19 |
![[thin space (1/6-em)]](https://www.rsc.org/images/entities/char_2009.gif) |
Zofran tablet – ondansetron hydrochloride, 4 mg |
Electrode 1 |
Electrode 2 |
Electrode 3 |
Electrode 4 |
Concentration of ODH (M) |
9.8007 × 10−3 |
9.6739 × 10−3 |
9.7123 × 10−3 |
9.6908 × 10−3 |
Rec (%) |
98.00 |
96.73 |
97.12 |
96.90 |
RE (%) |
−2.00 |
−3.27 |
−2.88 |
−3.10 |
![[thin space (1/6-em)]](https://www.rsc.org/images/entities/char_2009.gif) |
Zofran tablet – ondansetron hydrochloride, 4 mg |
Reference method21 |
Rec (%) |
99.42 |
RE (%) |
0.61 |
4. Conclusion
This work has demonstrated the importance of PVC sensors in potentiometric applications for measurement of ondansetron in two pharmaceutical formulations. The ODH-MPA-DBPH membrane sensor was more optimal than the other prepared electrodes with respect to Nernstian response, reproducibility, and sensitivity. The results of this investigation enable the direct prediction of ondansetron. Our data indicate that polymeric membrane sensors are unique for characterizations at wide ranges of pH (from 2.5 to 5.5). Our methodology was also found to be an important design process because a selective response toward ondansetron in the presence of all the expected interfering ions was exhibited.
Conflicts of interest
There are no conflicts to declare.
References
- R. Asad, I. A. Subhan, K. Aslam and A. Rehman, Application of certain π-acceptors for the spectrophotometric determination of ondansetron in pharmaceutical formulations, Anal. Chem., 2007, 6(2), 43–47 Search PubMed.
- British pharmacopoeia, Version 17, Copyright by, London, 2012.
- British Pharmacopoeia, Her Majesty, Stationery Office, London, 2003, 2.
- S. Mujbaile, P. Prasad and S. Wate, Simultaneous estimation of Ondansetron and Pantoprazole in solid dosage form by first derivative spectroscopy method, Tablet 4, no. 40, 2012, 100–117.
- M. Zaabal, M. Doulache, N. K. Bakirhan, S. Kaddour, B. Saidat and S. A. Ozkan, A facile strategy for construction of sensor for detection of ondansetron and investigation of its redox behavior and thermodynamic parameters, Electroanalysis, 2019, 31(7), 1279–1290 CrossRef CAS.
- S. Jadhav, R. Kharat, A. Ansari and A. Tamboli, Estimation of Ondansetron Hydrochloride in bulk and formulation by second order derivative area under curve UV-Spectrophotometric Methods, PharmaTutor, 2015, 3(8), 42–46 Search PubMed.
- S. N. Gayatri, V. M. N. Biju and A. M. Starvin, Determination of Ondansetron by Spectrofluorimetry: Application to Forced Degradation Study, Pharmaceuticals and Human Plasma, J. Fluoresc., 2019, 29(1), 203–209 CrossRef CAS PubMed.
- R. Kalaichelvi, B. Madhava Rao, S. Manikanta, G. Gopinath, M. Usha, D. Venkata Ramana, D. Srinivasa Rao and E. Jayachandran, UV spectrophotometric method for determination of ondansetron hydrochloride in pure and its formulation, Int. J. Pharm. Pharm. Sci., 2012, 4(4), 151–152 CAS.
- P. V. Colthup, C. C. Felgate, J. L. Palmer and N. L. Scully, Determination of ondansetron in plasma and its pharmacokinetics in the young and elderly, J. Pharm. Sci., 1991, 80(9), 868–871 CrossRef CAS PubMed.
- A. M. I. Mohamed and N. A.-L. Mohamed, Development and validation of RP-HPLC method for simultaneous determination of ondansetron hydrochloride and granisetron hydrochloride in their admixtures with pantoprazole sodium, Thai J. Pharm. Sci., 2020, 44(2), 82–90 CAS.
- A. Anilkumar, Formulation of Ondansetron HCl Matrix Tablets with Microenvironmental pH Modifier for Improved Dissolution and Bioavailability under Hypochlorhydria, Asian J. Pharm., 2016, 10(3), 188–197 CAS.
- Z. Dedania, R. Dedania, V. Karkhanis, G. Vidya Sagar, M. Baldania and N. R. Sheth, RP-HPLC method for simultaneous estimation of omeprazole and ondansetron in combined dosage forms, Asian J. Res. Chem., 2009, 2(2), 108–111 CAS.
- F. C. Chen, L.-H. Wang, J. Guo, X.-Y. Shi and B.-X. Fang, Simultaneous determination of dexamethasone, ondansetron, granisetron, tropisetron, and azasetron in infusion samples by HPLC with DAD detection, J. Anal. Methods Chem., 2017, 2017, 1–6 CrossRef PubMed.
- F. Faridbod, F. Mizani, M. R. Ganjali and P. Norouzi, Determination of ondansetron hydrochloride by a liquid membrane potentiometric sensor based on room temperature ionic liquids, Int. J. Electrochem. Sci., 2013, 8(8), 10461–10472 CAS.
- S. S. M. Hassan, W. H. Mahmoud, M. A. F. Elmosallamy and M. H. Almarzooyi, Determination of diclofenac in pharmaceutical preparations using a novel PVC membrane sensor, Int. J. Pharm. Sci., 2003, 58(no. 1), 29–31 CAS.
- G. Ninama, R. Patel, M. Patel and G. Shah, Solid phase extraction liquid chromatography mass spectrometry method with electrospray ionization for the determination of Ondansetron in human plasma: Development and validation consideration, Arabian J. Chem., 2017, 10, S3135–S3141 CrossRef CAS.
- F. Faridbod, F. Mizani, M. R. Ganjali and P. Norouzi, Determination of ondansetron hydrochloride by a liquid membrane potentiometric sensor based on room temperature ionic liquids, Int. J. Electrochem. Sci., 2013, 8(8), 10461–10472 CAS.
- A. P. Abbott, S. S. M. Alabdullah, A. Y. M. Al-Murshedi and K. S. Ryder, Brønsted acidity in deep eutectic solvents and ionic liquids, Faraday Discuss., 2017, 206, 365–377 RSC.
- A. Craggs, G. J. Moody and J. D. R. Thomas, PVC matrix membrane ion-selective electrodes. Construction
and laboratory experiments, J. Chem. Educ., 1974, 51(8), 541 CrossRef CAS.
- G. A. E. Mostafa, PVC matrix membrane sensor for potentiometric determination of metoclopramide hydrochloride in some pharmaceutical formulations, J. Pharm. Biomed. Anal., 2003, 31(3), 515–521 CrossRef CAS PubMed.
- A. Raza, A. S. Ijaz and U. Rasheed, Spectrophotometric determination of ondansetron hydrochloride in pharmaceutical bulk and dosage forms, J. Chin. Chem. Soc., 2007, 54(1), 223–227 CrossRef CAS.
- J. M. Miller, A. Dahan, D. Gupta, S. Varghese and G. L. Amidon, "Quasi-equilibrium analysis of the ion-pair mediated membrane transport of low-permeability drugs, J. Controlled Release, 2009, 137(1), 31–37 CrossRef CAS PubMed.
- S. Mulagada and S. Rao Baratam, Design and evaluation of ondansetron fast disintegrating tablets using natural polymers and modified starches as super disintegrants for the enhancement of dissolution, J. Young Pharm., 2017, 9(4), 519 CrossRef CAS.
- M. A. F. Elmosalamy, G. J. Moody, J. D. R. Thomas and S. M. H. Saad, Poly(Vinyl Chloride) Matrix Membrane Electrodes Responsive to Thiocyanate, Perchlorate and Periodate, Anal. Lett., 1987, 20(10), 1541–1555 CrossRef CAS.
- R. Jain and R. Sharma, Cathodic adsorptive stripping voltammetry of an anti-emetic agent Granisetron in pharmaceutical formulation and biological matrix, J. Pharm. Anal., 2012, 2(6), 443–449 CrossRef CAS PubMed.
- A. M. Abass, Preparation and Application of Tetracycline Hydrochloride Liquid membrane Electrodes, Al-Nahrain Journal of Science, 2018, 21(2), 73–80 Search PubMed.
- B. Nigović, M. Sadiković and M. Sertić, Multi-walled carbon nanotubes/Nafion composite film modified electrode as a sensor for simultaneous determination of ondansetron and morphine, Talanta, 2014, 122, 187–194 CrossRef PubMed.
- H. A. Mohammed and A. M. Abass, Poly(vinyl chloride) matrix membrane sensors for the quantification of cyclizine hydrochloride in pure and pharmaceutical preparations, in AIP Conference Proceedings, AIP Publishing LLC, 2020, vol. 2213, no. 1, p. 020034 Search PubMed.
|
This journal is © The Royal Society of Chemistry 2021 |