DOI:
10.1039/D1RA04130D
(Paper)
RSC Adv., 2021,
11, 25207-25210
Cerium photocatalyzed radical smiles rearrangement of 2-aryloxybenzoic acids†
Received
27th May 2021
, Accepted 15th July 2021
First published on 21st July 2021
Abstract
We report herein a cerium photocatalyzed aryl migration from an aryl ether to a carboxylic acid group through radical-Smiles rearrangement. This operationally simple protocol utilizes inexpensive CeCl3 as a photocatalyst and converted a variety of 2-aryloxybenzoic acids into aryl-2-hydroxybenzoates in good yields.
Introduction
In synthetic organic chemistry, rearrangement reactions offer a unique path to atom-economic synthesis.1 In this vein, the Smiles rearrangement and its varients2 have been found widespread synthetic applications in organic synthesis originally through an intramolecular nucleophilic aromatic ipso substitution.3 Typically the aromatic substrates are activated by electron-withdrawing groups at the ortho or para positions. Initially these reactions were explored in ionic reaction conditions and later was transposed into radical chemistry by Speckamp.4 However, the importance of radical-Smiles rearrangement has been realized recently.5 The radical Smiles rearrangement allows not only the formal migration of aryl and other unsaturated C–C bonds but is also capable of breaking various C(sp2)–X (X = S, O, N, C) bonds.6 Different from ionic reaction conditions, the presence of electron-withdrawing groups is not essential in radical Smiles rearrangement.
Various strategies have been developed for aryl-migration.6 Notably, aryl migration from an aryl ether through C–O bond cleavage to form a carboxyl ester is rare. In 1955 DeTar and Hlynsky first observed this migration through thermal decomposition of 2-phenoxybenzoyl peroxide.7 In 1972 Yang and co-workers reported the first example of radical Smiles rearrangement of 2-aryloxybenzoic acids using UV-irradiation (λ > 280 nm) as a promoter.8 In 2016, Hossian and Jana reported silver-catalyzed carboxyl radical-assisted 1,5-aryl migration from 2-aryloxy-benzoic acids at 130 °C in the presence of stoichiometric amounts of oxidant and base to afford aryl-2-hydroxybenzoates (Fig. 1).9
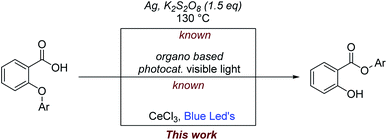 |
| Fig. 1 Known reports for 1,5-aryl migration of 2-aryloxybenzoic acids. | |
In recent years, visible light driven photocatalysis has emerged as a sustainable synthetic tool in organic chemistry to generate a variety of radical entities from organic molecules.10 However, the employment of expensive and toxic metal catalysts (Ru and Ir) in photoredox catalysis is a major concern especially for the synthesis of pharmaceutical compounds, particularly on a large scale. Therefore, the use of cheaper and more sustainable photocatalysts is a good alternative.11 In 2017, independently two groups reported visible light driven efficient aryl migration from an aryl ether to a carboxylic acid group to form an ester.12 This reaction was catalyzed by perylenediimide (PDI) and the Fukuzumi photocatalyst (Mes-Acr+ = PC) and displays a broad substrate scope. Very recently Ye and co-workers13 reported dual N-heterocyclic carbene/photo-catalyzed aryl migration of 2-aryloxy benzaldehydes via in situ formation of 2-aryloxy benzoic acids which further participate in radical smiles rearrangement. However, all the reported methods require stoichiometric amounts of oxidant or expensive photocatalysts. An operationally simple and inexpensive method for the efficient aryl migration of 2-aryloxy benzoic acids is still desirable. Although recently, it was found that employing inexpensive chemicals in the visible light photocatalysis is a robust alternative to generate carbon radicals under mild reaction conditions.14 In particular CeCl3 was recently discovered to be a ligand-to-metal charge transfer (LMCT) activation manifold to trigger the generation of oxygen centered radicals from alcohols and carboxylic acids.14 In continuation of our research interest on visible light driven cerium photocatalysis,15 we herein report a mild protocol for the 1,5-aryl migration of 2-aryloxy benzoic acids using 10 mol% CeCl3.
Results and discussions
At first, we initiated our study utilizing 2-phenoxybenzoic acid (1a) as a model substrate and (NH4)2S2O8 as an oxidant. A variety of reaction parameters were tested during the optimization of the reaction (Table 1). We were pleased to find that the efficient aryl migration from an aryl ether to a carboxylic acid group takes place at 35 °C, upon continuous irradiation with blue LEDs (455 nm) under cerium photocatalysis. Employing 10 mol% CeCl3 as the photocatalyst and 20 mol% (NH4)2S2O8 as an oxidant in the presence of NaHCO3 (10 mol%) in EtOAc gave compound 2a in 75% isolated yield after 30 h (Table 1, entry 1). The reaction using CeCl3·7H2O and (nBu4N)2CeIVCl6 as a photocatalyst slightly reduced the yield of the reaction (Table 1, entry 2 and 3), while the conversion to 2a decreased upon use of Ce(SO4)2·4H2O (Table 1, entry 4). When NaHCO3 was replaced by Cs2CO3, 2a was afforded in 40% yield (Table 1, entry 5), while other bases such as Na2CO3, K3PO4 led to drastic reduction in the yield (Table 1, entry 6–7). The reaction was performed in absence of base afforded 2a in 25% yield (Table 1, entry 8). The reaction worked with similar efficiency in CH3CN (Table 1, entry 9), while other solvents such as THF, DCM and dioxane afforded 2a in moderate yield (Table 1, entry 10–12). Employing O2 balloon instead of (NH4)2S2O8 afford 2a in 68% (Table 1, entry 13).16 Also, it was observed that the yields were less reproducible and varied about 15% upon using air as an oxidant (Table 1, entry 14). Irradiation with green LEDs did not lead to any product formation. Additionally, controlled experiments indicated that catalytic amount of the cerium salt, oxidant and light irradiation were necessary for the reaction to occur (Table 1, entries 16 and 17).
Table 1 Optimization of the reaction conditions. 1a (0.2 mmol), CeCl3 (10 mol%), EtOAc (2 ml) at 35 °C, 455 nm blue LED for 24 h
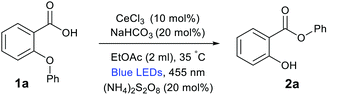
|
Entry |
Deviation from standard conditions |
2aa (%) |
NMR yields using trimethoxy benzene as internal standard. Isolated yield. |
1 |
None |
80(75)b |
2 |
CeCl3·7H2O instead of CeCl3 |
68 |
3 |
(nBu4N)2CeCl6 instead of CeCl3 |
60 |
4 |
Ce(SO4)2·4H2O instead of CeCl3 |
35 |
5 |
Cs2CO3 instead of NaHCO3 |
40 |
6 |
Na2CO3 instead of NaHCO3 |
10 |
7 |
K3PO4 instead of NaHCO3 |
23 |
8 |
With out NaHCO3 |
25 |
9 |
CH3CN instead of EtOAc |
75 |
10 |
THF instead of EtOAc |
33 |
11 |
DCM instead of EtOAc |
23 |
12 |
Dioxane instead of EtOAc |
50 |
13 |
O2 balloon instead of (NH4)2S2O8 |
68 |
14 |
Air instead of (NH4)2S2O8 |
55–70 |
15 |
Green Led's (530 nm) |
0 |
16 |
Without light |
0 |
17 |
With out CeCl3 |
Trace |
With the optimized reaction conditions in hand, we evaluated the substrate scope of the reaction with diverse substituted 2-aryloxybenzoic acids, which were prepared through known literature protocols. As shown in Scheme 1, a broad range of 2-aryloxybenzoic acids were reacted in our reaction conditions afforded corresponding aryl-2-hydroxybenzoate derivatives in good yields. First, the electronic variation in the para-position of the Ar2 ring was studied. The results indicated that electron-donating and withdrawing substituents such as Me (1b), methoxy (1c), tert-butyl (1d), phenyl (1e), fluoro (1f), chloro (1g) and bromo (1h) were all well tolerated, giving aryl-2-hydroxybenzoates (2b–2h) in 65–85% yield. Next, the electronic variation in the ortho and meta-substitution of the Ar2 ring was investigated. Electron donating (Me, OMe) and withdrawing groups (Cl, Br, I, COCH3) provided the corresponding aryl-2-hydroxybenzoates (2i–2p) in moderate to excellent yields (Scheme 1). Meanwhile employing ortho/meta substituted aryloxy benzoic acids (1q, 1r) afforded the products (2q, 2r) in good yields. Interestingly, substrate derived from 2-naphthol (1s) also furnished the migratory product (2s) in 60% yield. Next the substitution on Ar1 ring was studied. Diverse electron donating and withdrawing groups provided the corresponding aryl-2-hydroxybenzoates (2t–2ac) in moderate to good yields (Scheme 1). Unfortunately, the migratory event of the corresponding thioether (1ad) and aryl amine (1ae) failed in our reaction conditions. Further to demonstrate the potential application of our methodology, a gram-scale synthesis of acetyl free Guacetisal was carried out in our cerium photocatalysis. Performing the reaction with 4.1 mmol portion of 1j, it could be converted to 2j in 55% yield. This result indicated that the cerium photocatalyzed radical smiles rearrangement had great potential in practical organic synthesis.
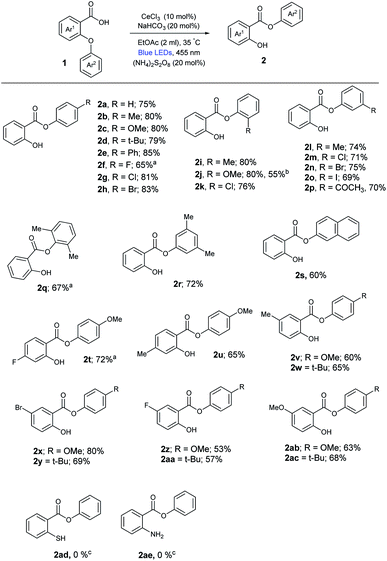 |
| Scheme 1 Cerium photocatalyzed 1,5-aryl migration of 2-aryloxybenzoic acids. Reaction conditions as given in Table 1 (entry 1). Isolated yields, average of at least two independent runs. a30 h reaction time. b80 h reaction time. c36 h reaction time. | |
The efficiency of our cerium photocatalyzed 1,5-aryl migration of 2-aryloxybenzoic acids prompted us to conduct some preliminary mechanistic studies. As anticipated, ON/OFF experiments revealed that our reaction required continuous visible light irradiation to proceed (see ESI†). The inhibition of catalysis upon addition of TEMPO further indicates that the reaction proceeds via radical intermediates. Based on these experimental observations and the known literature reports we propose that the aryl migration proceeds via Ligand to Metal Charge Transfer (LMCT), which generates the key aromatic carboxy-radical. Given that decarboxylation of aromatic carboxyl radicals is slower than that of their aliphatic homologues,17 the generated aromatic carboxy-radical could be further trapped by the aryl ether substituent in an intramolecular fashion, and further reduction would generate the aryl-2-hydroxybenzoates (Scheme 2). The simplified mechanistic proposal is shown in Scheme 2. The putative Ce(III) species could be oxidized to CeIV (E1/2(CeIII/CeIV) = 0.41 V vs. SCE in MeCN)14a either by the phenoxy radical E or by the (NH4)2S2O8 (E = 1.75 V vs. SCE).18 The coordination of the substrate forms complex B, which undergoes the photoinduced Ce–O(CO) homolytic cleavage regenerates the catalytically competent Ce(III) species (detected by UV spectroscopy, see ESI†) and the carboxy-radical C. A subsequent intramolecular ipso attack on the aryl ether moiety generates the cyclized intermediate D, followed by a aryl migration led to phenoxy radical intermediate E. Given the oxidation potential obtained for phenolate of 2a,12a the corresponding phenoxy radical can easily oxidize Ce(III) closing the catalytic cycle without requiring any external stoichiometric oxidant. The final proton transfer from 1a to phenolate leads to product 2a.
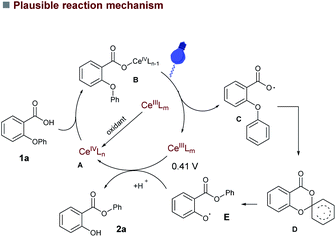 |
| Scheme 2 Plausible mechanism for 1,5-aryl migration of 2-aryloxybenzoic acids. | |
Conclusions
In summary, we have developed a cerium photocatalyzed selective 1,5-aryl migration of 2-aryloxybenzoic acids through radical Smiles rearrangement. This operationally simple protocol utilizes inexpensive CeCl3 as a photocatalyst and converted a variety of 2-aryloxybenzoic acids into aryl-2-hydroxybenzoates in absence of stoichiometric oxidant and base. Furthermore, we have applied our methodology for the gram scale synthesis of Guacetisal an important drug molecule in pharmaceutical industry.
Conflicts of interest
There are no conflicts to declare.
Acknowledgements
V. R. Y. acknowledges IISER-TVM for the financial support. ART and GSY acknowledges the IISER TVM for the doctoral fellowship. The authors thanks to Shradha Ajith for the synthesis of starting materials.
Notes and references
-
(a) S. Sugita, M. Ueda, N. Doi, N. Takeda and O. Miyata, Tetrahedron Lett., 2016, 57, 1786–1789 CrossRef CAS;
(b) J.-J. Feng and J. Zhang, J. Am. Chem. Soc., 2011, 133, 7304–7307 CrossRef CAS PubMed;
(c) S. N. MacMillan and J. M. T. R. Waterman, Chem. Commun., 2007, 4172–4174 RSC ; for a review, see:;
(d) B. M. Trost, Acc. Chem. Res., 2002, 35, 695–705 CrossRef CAS PubMed.
- For selected reviews, see:
(a) T. J. Snape, Chem. Soc. Rev., 2008, 37, 2452–2458 RSC;
(b) A. R. P. Henderson, J. R. Kosowan and T. E. Wood, Can. J. Chem., 2017, 95, 483–504 CrossRef CAS ; for a recent review, see:;
(c) L. El Kaïm and L. Grimaud, Eur. J. Org. Chem., 2014, 7749–7762 CrossRef.
- For reviews, see:
(a) J. F. Bunnett and R. E. Zahler, Chem. Rev., 1951, 49, 273–412 CrossRef CAS;
(b) A. A. Levy, H. C. Rains and S. Smiles, J. Chem. Soc., 1931, 3264–3269 RSC;
(c) S. Xia, L.-Y. Wang, H. Zuo and Z.-B. Li, Curr. Org. Synth., 2014, 10, 935–946 CrossRef.
-
(a) R. Loven and W. N. Speckamp, Tetrahedron Lett., 1972, 13, 1567–1570 CrossRef;
(b) J. J. Koehler and W. N. Speckamp, Tetrahedron Lett., 1977, 18, 631–634 CrossRef.
-
(a) J. J. Douglas, M. J. Sevrin, K. P. Cole and C. R. J. Stephenson, Org. Process Res. Dev., 2016, 20, 1148–1155 CrossRef CAS;
(b) M. Chen, C. Yang, Y. Wang, D. Li and W. Xia, Org. Lett., 2016, 18, 2280–2283 CrossRef CAS PubMed;
(c) P. Lan, C. J. Jackson, M. G. Banwell and A. C. Willis, J. Org. Chem., 2014, 79, 6759–6764 CrossRef CAS PubMed;
(d) M. Tada, H. Shijima and M. Nakamura, Org. Biomol. Chem., 2003, 1, 2499–2505 RSC;
(e) E. Brachet, L. Marzo, M. Selkti, B. König and P. Belmont, Chem. Sci., 2016, 7, 5002–5006 RSC.
- For selected reviews:
(a) A. Studer and M. Bossart, Tetrahedron, 2001, 57, 9649–9667 CrossRef CAS;
(b) Z.-M. Chen, X.-M. Zhang and Y.-Q. Tu, Chem. Soc. Rev., 2015, 44, 5220–5245 RSC;
(c) C. M. Holden and M. F. Greaney, Chem.–Eur. J., 2017, 23, 8992–9008 CrossRef CAS PubMed;
(d) J. J. Douglas, H. Albright, M. J. Sevrin, K. P. Cole and C. R. J. Stephenson, Angew. Chem. Int. Ed., 2015, 54, 14898–14902 (Angew. Chem., 2015, 127, 15111–15115) CrossRef CAS PubMed;
(e) D. Alpers, K. P. Cole and C. R. J. Stephenson, Angew. Chem. Int. Ed, 2018, 57, 12167–12170 (Angew. Chem., 2018, 130, 12344–12348) CrossRef CAS PubMed;
(f) M. Wang, H. Zhang, J. Liu, X. Wu and C. Zhu, Angew. Chem. Int. Ed., 2019, 58, 17646–17650 (Angew. Chem., 2019, 131, 17810–17814) CrossRef CAS PubMed.
- D. F. DeTar and A. Hlynsky, J. Am. Chem. Soc., 1955, 77, 441–4412 Search PubMed.
- N.-C. C. Yang, P. Kumler and S. S. Yang, J. Org. Chem., 1972, 37, 4022–4026 CrossRef CAS.
- A. Hossian and R. Jana, Org. Biomol. Chem., 2016, 14, 9768–9779 RSC.
- For selected reviews on photoredox catalysis, see:
(a) L. Marzo, S. K. Pagire, O. Reiser and B. König, Angew. Chem. Int. Ed., 2018, 57, 10034–10072 (Angew. Chem., 2018, 130, 10188–10228) CrossRef CAS PubMed;
(b) M. Kärkäs, J. Porco Jr and C. Stephenson, Chem. Rev., 2016, 116, 9683–9747 CrossRef PubMed;
(c) M. H. Shaw, J. Twilton and D. W. C. MacMillan, J. Org. Chem., 2016, 81, 6898–6926 CrossRef CAS PubMed;
(d) A. U. Meyer, T. Slanina, A. Heckel and B. König, Chem.–Eur. J., 2017, 23, 7900–7904 CrossRef CAS PubMed.
- N. A. Romero and D. A. Nicewicz, Chem. Rev., 2016, 116, 10075–10166 CrossRef CAS PubMed.
-
(a) J. C. Gonzalez-Gomez, N. P. Ramirez, T. Lana-Villarrealb and P. Boneteb, Org. Biomol. Chem., 2017, 15, 9680–9684 RSC;
(b) S.-F. Wang, X.-P. Cao and Y. Li, Angew. Chem. Int. Ed., 2017, 56, 13809–13813 (Angew. Chem., 2017, 129, 13997–14001) CrossRef CAS PubMed.
- X. Zi-Hao, D. Lei, G. Zhong-Hua and Y. Song, Chem. Commun., 2020, 56, 1525–1528 RSC.
-
(a) A. Hu, J.-J. Guo, H. Pan, H. Tang, Z. Gao and Z. Zuo, J. Am. Chem. Soc., 2018, 140, 1612–1616 CrossRef CAS PubMed;
(b) A. Hu, J.-J. Guo, H. Pan and Z. Zuo, Science, 2018, 361, 668–672 CrossRef CAS PubMed;
(c) V. R. Yatham, P. Bellotti and B. König, Chem. Commun., 2019, 55, 3489–3492 RSC;
(d) M.-C. Fu, R. Shang, B. Zhao, B. Wang and Y. Fu, Science, 2019, 363, 1429–1434 CrossRef CAS PubMed;
(e) J. Schwarz and B. König, Chem. Commun., 2019, 55, 486–488 RSC.
- K. Wadekar, S. Aswale and V. R Yatham, Org. Biomol. Chem., 2020, 18, 983–987 RSC.
-
(a) I. Geibel, A. Dierks, T. Meller and J. Christoffers, Chem.–Eur. J., 2017, 23, 7245–7254 CrossRef CAS PubMed;
(b) J.-M. Speldrich and J. Christoffers, Eur. J. Org. Chem., 2021, 907–914 CrossRef CAS.
-
(a) D. H. R. Barton, B. Lacher and S. Z. Zard, Tetrahedron, 1987, 43, 4321–4328 CrossRef CAS;
(b) J. Chateauneuf, J. Lusztyk and K. U. Ingold, J. Am. Chem. Soc., 1988, 110, 2886 CrossRef CAS;
(c) J. K. Kochi, T. M. Bockman and S. M. Hubig, J. Org. Chem., 1997, 62, 2210–2221 CrossRef PubMed.
- C. Dai, F. Meschini, J. M. R. Narayanam and C. R. J. Stephenson, J. Org. Chem., 2012, 77, 4425–4431 CrossRef CAS PubMed.
Footnote |
† Electronic supplementary information (ESI) available. See DOI: 10.1039/d1ra04130d |
|
This journal is © The Royal Society of Chemistry 2021 |
Click here to see how this site uses Cookies. View our privacy policy here.