DOI:
10.1039/D1RA04297A
(Paper)
RSC Adv., 2021,
11, 22120-22124
A practical method for the aziridination of α,β-unsaturated carbonyl compounds with a simple carbamate utilizing sodium hypochlorite pentahydrate†
Received
3rd June 2021
, Accepted 17th June 2021
First published on 22nd June 2021
Abstract
The efficient formation of tert-butyl N-chloro-N-sodio-carbamate by the reaction of simple tert-butyl carbamate with sodium hypochlorite pentahydrate (NaOCl·5H2O) would be a practical and green method for the aziridination of α,β-unsaturated carbonyl compounds. The process described herein is transition-metal free, all of the materials are commercially available, the byproducts (NaCl and H2O) are environmentally benign and the reaction is stereoselective. The resulting aziridines are potential precursors of amino acids.
Introduction
Aziridines containing a carbonyl functionality at the 2-position represent potential precursors of natural and unnatural α- or β-amino acids by ring-opening reactions with suitable nucleophiles.1,2 Among the possible synthetic methods for preparing such aziridines, the one-step installation of a nitrogen unit into an α,β-unsaturated carbonyl compound would be a practical approach, and catalytic and stereoselective variants have been developed.3,4 The preparation of activated aziridines that contain electron-withdrawing groups on the nitrogen, which would be readily ring-opened, from α,β-unsaturated carbonyl compounds would be useful route to the synthesis of amino acid derivatives.4 The nitrogen sources used for synthesizing these aziridines are highly oxidized, and include hypervalent iodine reagents (ArSO2N
IPh)4a,b,d and nitrogen-oxygenated agents (ArSO3–NHCO2R).4c,f,g These reagents require transition-metal catalysts or bases for the desired aziridination to be successful, and they have drawbacks such as difficulties associated with their preparation and instability. When these nitrogen sources are used in aziridination reactions, the by-products are iodobenzene and aryl sulfonates, thus making such reactions not atom-economical. N-Chloro-N-sodio-carbamates (chloramine salts) as an alternative nitrogen source were developed by our group for the aziridination of α,β-unsaturated carbonyl compounds.5
This aziridination involved the 1,4-addition of a chloramine salt to an α,β-unsaturated carbonyl compound, followed by cyclization of the resulting enolates accompanied by liberating only sodium chloride as the by-product. By taking advantage of the poor solubility of chloramine salts, the use of solid–liquid phase transfer catalyst, optically active quaternary ammonium salts, realized the stereoselective aziridination.5,6 Since chiral aziridines derived from enones are valuable building blocks,7 tremendous efforts have been devoted to the development of the stereoselective aziridination of enones.8,9 Among these, our protocols5,6 are promising for producing enantioenriched aziridines. Our nitrogen sources, chloramine salts, however, are prepared by the reaction of a carbamate (benzyl carbamate or tert-butyl carbamate) with tert-butyl hypochlorite followed by treatment with sodium hydroxide, in which tert-butanol is by-product, and the efficiency is low. In addition, the chloramine salts are not sufficiently stable to allow them to be stored in a freezer (Scheme 1).
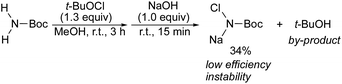 |
| Scheme 1 Inefficient formation and instability of chloramine salt of carbamate. | |
To overcome these problems and make it a more sustainable reaction, we envisaged the in situ generation of the reactive nitrogen source, a chloramine salt, using a suitable oxidant for the desired aziridination. From these points of view, we report herein on the practical aziridination of α,β-unsaturated carbonyl compounds with a simple carbamate and an ideal oxidant in the presence of quaternary ammonium salt as a phase transfer catalyst. The optimal conditions using the oxidant were applied to the stereoselective aziridination developed by our group.5,6
Results and discussion
In order to find an ideal oxidant, considering minimum combination of atoms required for the transformation of simple carbamate into N-chloro-N-sodio-carbamate, we concluded that sodium hypochlorite would be the ideal oxidant, because the by-product molecule is only water (Scheme 2). We recently found that sodium hypochlorite pentahydrate in the solid form10 and not aqueous solution of sodium hypochlorite was the ideal oxidant for the transformation of sulfonamides into the corresponding N-chloro-N-sodiosulfonamides.11 As a simple carbamate, we focused on tert-butyl carbamate because it contains a Boc group that can be readily removed. In fact, when tert-butyl carbamate was treated with NaOCl·5H2O in MeCN at room temperature for 3 h, tert-butyl N-chloro-N-sodio-carbamate (chloramine-Boc)12 was obtained in high yield (Scheme 2).
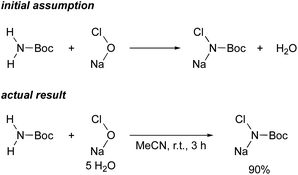 |
| Scheme 2 Initial assumption and actual result for efficient formation of chloramine-Boc. | |
The efficient formation of chloramine-Boc with the ideal oxidant prompted us to investigate the aziridination of α,β-unsaturated carbonyl compounds using the simple tert-butyl carbamate. Although chloramine-Boc is formed in 90% yield, the use of 1.4 equivalents of H2NBoc and NaOCl·5H2O relative to the olefin substrates was found to be suitable for the desired aziridination. When benzyl acrylate (1a) was treated with tert-butyl carbamate in the presence of NaOCl·5H2O as an oxidant and benzyltriethylammonium chloride under conditions of as a phase transfer catalysis, the desired aziridine 2a was obtained in 15% yield (path a) along with the β-aminated ester 3a as the main product (Table 1, Entry 1). Since the enolate derived from the 1,4-addition of in situ generated chloramine-Boc to 1a would be protonated with water leading to 3a (path b), we screened a series of dehydrating agents. Although the addition of MS3A drastically improved the yield of 2a (Entry 2), the formation of 3a could also be effectively reduced by using K2CO3 (Entry 3). The rate of aziridination was very slow without the addition of the PTC (Entry 4) but when the reaction time was extended, 5 mol% of PTC was sufficient to allow the aziridination to proceed (Entry 5). Decreasing the amount of K2CO3 resulted in a decrease in the yield of aziridine 2a and an increase in the protonated product 3a (Entry 6). Other additives were not effective for the aziridination (Entries 7–12).
Table 1 Optimization of reaction conditions (1)a
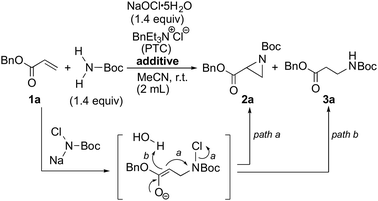
|
Entry |
Additive |
PTC (mol%) |
Time (h) |
Yieldb (%) |
2a |
3a |
Reactions were performed on a 0.5 mmol scale. Determined by 1H NMR analysis of the crude product. Isolated yield. |
1 |
None |
10 |
12 |
15 |
40 |
2 |
MS3A (800 mg) |
10 |
12 |
74 |
25 |
3 |
K2CO3 (9 equiv.) |
10 |
12 |
80 |
9 |
4 |
K2CO3 (9 equiv.) |
None |
24 |
58 |
7 |
5 |
K2CO3 (9 equiv.) |
5 |
18 |
82c |
1 |
6 |
K2CO3 (6 equiv.) |
5 |
18 |
60 |
12 |
7 |
Cs2CO3 (9 equiv.) |
5 |
24 |
73 |
3 |
8 |
K3PO4 (9 equiv.) |
5 |
21 |
69 |
1 |
9 |
Et3N (9 equiv.) |
5 |
24 |
33 |
15 |
10 |
Na2CO3 (9 equiv.) |
5 |
18 |
11 |
35 |
11 |
KHCO3 (9 equiv.) |
5 |
24 |
9 |
61 |
12 |
NaHCO3 (9 equiv.) |
5 |
24 |
9 |
47 |
In order to examine the superiority of NaOCl·5H2O, related oxidants, including tert-BuOCl, Ca(ClO)2, N-chlorosuccinimide (NCS), and aqueous NaOCl were employed in the reaction, but the efficiencies were low compared to the solid state NaOCl (Table 2, Entries 1–4). After screening other solvents, MeCN was found to be the optimal solvent for the aziridination (Entries 5–9).
Table 2 Optimization of reaction conditions (2)a
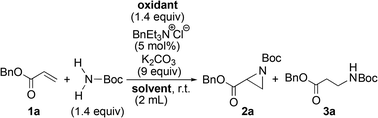
|
Entry |
Oxidant |
Solvent |
Time (h) |
Yieldb (%) |
2a |
3a |
Reactions were performed on a 0.5 mmol scale. Determined by 1H NMR analysis of the crude product. K2CO3: 10.4 equiv. Ca(ClO)2: 0.7 equiv. 13 wt% (360 μl). |
1 |
tert-BuOClc |
MeCN |
18 |
51 |
23 |
2 |
Ca(ClO)2d |
MeCN |
24 |
44 |
0 |
3 |
NCS |
MeCN |
48 |
0 |
0 |
4 |
NaOCl aq.e |
MeCN |
18 |
42 |
21 |
5 |
NaOCl·5H2O |
CH2Cl2 |
18 |
66 |
5 |
6 |
NaOCl·5H2O |
Toluene |
18 |
66 |
0 |
7 |
NaOCl·5H2O |
THF |
18 |
44 |
0 |
8 |
NaOCl·5H2O |
DMF |
24 |
8 |
0 |
9 |
NaOCl·5H2O |
EtOH |
6 |
0 |
0 |
Because the Boc group on the nitrogen of the resulting aziridine can be readily detached under mild conditions, we concluded that BocNH2 should be a useful nitrogen source. To examine the scope of the aziridination, other simple carbamates and amides as a nitrogen source were subjected to the conditions. Unfortunately, only benzyl carbamate could be used for the aziridination (2a′), albeit in low yield (Scheme 3). Trifluoroacetamide, benzamide, methanesulfonamide, benzensulfonamide, and tosylamide had no effect on the process. Carboxamides such as trifluoroacetamide and benzamide failed to react with NaOCl·5H2O under the conditions because of the low acidity of N–H protons. Sulfonamides were converted to chloramine salts by the reaction with NaOCl·5H2O, but their low nucleophilicity did not induce the 1,4-addition to 1a.
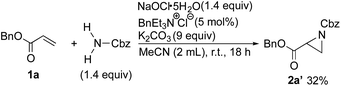 |
| Scheme 3 Formation of N-Cbz aziridine. | |
With the optimized conditions (Table 1, Entry 5) in hand, the scope of the α,β-unsaturated esters were investigated (Table 3). Methyl and ethyl acrylates were transformed into the corresponding aziridines (Entries 1 and 2). Although, under the same conditions, the yield for the reaction of tert-butyl acrylate was low, increasing the amount of the catalyst used and the reaction temperature improved the efficiency (Entries 3–5). Methyl cinnamate was completely unreactive, and it was fully recovered (Entry 6). Arylmethyl acrylates were converted into the corresponding aziridines in good yields (Entries 7 and 8).
Table 3 Scope of α,β-unsaturated estersa
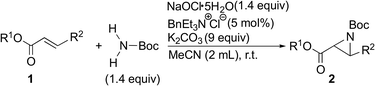
|
Entry |
R1 |
R2 |
|
Temp. (°C) |
Time (h) |
Yieldb (%) |
Reactions were performed on a 0.5 mmol scale. Isolated yield. PTC: 10 mol%. Recovery of olefin: 100%. |
1 |
Me |
H |
1b |
r.t. |
18 |
60 (2b) |
2 |
Et |
H |
1c |
r.t. |
18 |
55 (2c) |
3 |
tBu |
H |
1d |
r.t. |
24 |
12 (2d) |
4 |
tBu |
H |
1d |
40 |
20 |
27 (2d) |
5c |
tBu |
H |
1d |
80 |
18 |
35 (2d) |
6d |
Me |
Ph |
|
r.t. |
20 |
0 |
7 |
2-MeOC6H4CH2 |
H |
1e |
r.t. |
18 |
75 (2e) |
8 |
9-Anthracenylmethyl |
H |
1f |
r.t. |
18 |
57 (2f) |
In order to further expand the scope of substrates, an α,β-unsaturated ketone, phenyl vinyl ketone (1g), which was not examined in our previous studies,5,6 was employed in the present process, unexpectedly affording epoxide 4g as a main product as well as the desired aziridine 2g (Scheme 4).
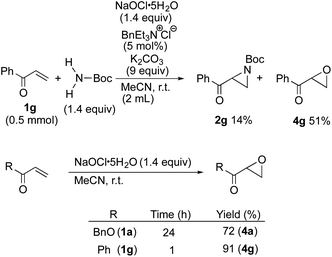 |
| Scheme 4 Comparison of the rate of the epoxidation of an α,β-unsaturated ester and ketone with NaOCl·5H2O. | |
To clarify the difference between the reaction of α,β-unsaturated esters and that of the ketone, considering that the oxygen source of the epoxide is NaOCl·5H2O, the olefins 1a and 1g were treated with NaOCl·5H2O. The rapid epoxidation of 1g was observed compared with that of 1a (Scheme 4). These results indicate that NaOCl·5H2O reacts with α,β-unsaturated ketones faster than with H2NBoc.
To avoid epoxide formation, when H2NBoc was first treated with NaOCl·5H2O in the presence of the PTC and K2CO3 at room temperature for 3 h, followed by the addition of phenyl vinyl ketone (1g), the desired aziridine 2g was produced in 87% yield (Table 4, Entry 1). Changing the solvent from MeCN to CH2Cl2 improved the reaction efficiency in this case. Since the electron-deficient olefins shown in Table 4 reacted first with NaOCl·5H2O, these conditions were employed in reactions of these substrates. Enones containing pyrazole, oxazolidinone, and carbazole moieties were smoothly transformed into the corresponding aziridines in good to excellent yields (Entries 2–4). The present method was applicable to a vinyl sulfone 1k, affording the aziridine 2k in good yield (Entry 5).
Table 4 Scope of electron-deficient olefins
The robust aziridination that occurs when utilizing NaOCl·5H2O could be applied to enantio- and diastereoselective reactions using optically active phase transfer catalysts, the conditions for which were optimized in our previous studies (Scheme 5).5,6 Hybridization of our present and previous conditions using the cinchonine-derived anthracenylmethylated ammonium salt CN1 as a chiral PTC induced asymmetric aziridination in good yield and enantioselectivity. The resulting aziridine containing dimethylpyrazole was readily transformed into the methyl ester-substituted aziridine 2b with no change in optical purity.
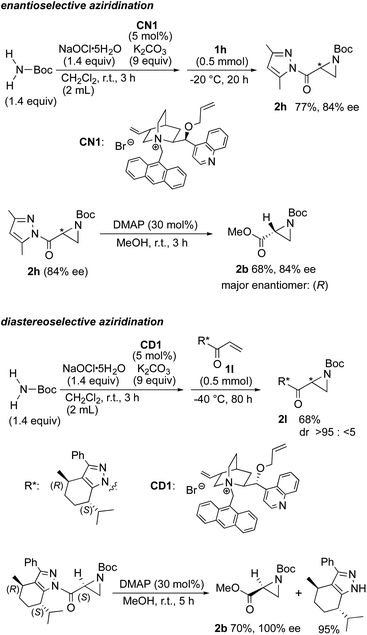 |
| Scheme 5 Enantio- and diastereoselective aziridination and deprotection. | |
Regarding the diastereoselectivity of the reaction, the combination of an L-menthopyrazole chiral auxiliary and a cinchonidine-derived chiral ammonium catalyst is essential for achieving a high diastereoselectivity. When tert-butyl carbamate was treated with NaOCl·5H2O in the presence of CD1 and K2CO3 in CH2Cl2 at room temperature for 3 h, followed by the addition of the enone 1l containing a chiral auxiliary at −40 °C for 80 h, the corresponding aziridine was produced in good yield with complete diastereoselectivity. The auxiliary was easily deprotected without epimerization giving the methyl ester derivative, with the efficient recovery of L-menthopyrazole auxiliary which can be recycled.
Conclusions
In conclusion, a practical and efficient process for the synthesis of valuable aziridines is reported. Although this basic transformation was previously established by our group, the use of a potential oxidant, NaOCl·5H2O allowed it to be an efficient, sustainable and robust reaction with the stereoselectivity of the aziridination being retained. The reaction is metal free and organocatalysts can be used with a high enantio- and diastereoselectivity, and the resulting aziridines represent potential precursors of α- or β-amino acids.
Conflicts of interest
There are no conflicts to declare.
Acknowledgements
In memory of Prof. Dr Kilian Muñiz (1970–2020). This project was partially supported by the Nippon Light Metal Company, Ltd., and a Grant-in-Aid for Science Research from the Japan Society for the Promotion of Science (Grant No. JP19H02716). We acknowledge the donation of NaOCl·5H2O from the Nippon Light Metal Company, Ltd.
Notes and references
- W. McCoull and F. A. Davis, Synthesis, 2000, 1347–1365 CrossRef CAS.
-
(a) G. Cardillo, L. Gentilucci and A. Tolomelli, Aldrichimica Acta, 2003, 36, 39–50 CAS;
(b) X. E. Hu, Tetrahedron, 2004, 60, 2701–2743 CrossRef CAS;
(c) P. Lu, Tetrahedron, 2010, 66, 2549–2560 CrossRef CAS;
(d) S. Stanković, M. D'hooghe, S. Catak, H. Eum, M. Waroquier, V. V. Speybroeck, N. De Kimpe and H.-J. Ha, Chem. Soc. Rev., 2012, 41, 643–665 RSC;
(e) J. Kidd, K. Maiden and J. B. Morgan, Tetrahedron, 2016, 72, 3802–3807 CrossRef CAS PubMed.
- For the synthesis of N–H aziridines from olefins including enones:
(a) N. Furukawa, T. Yoshimura, M. Ohtsu, T. Akasaka and S. Oae, Tetrahedron, 1980, 36, 73–80 CrossRef CAS;
(b) P. Métra and J. Hamelin, J. Chem. Soc., Chem. Commun., 1980, 1038–1039 RSC;
(c) I. Ikeda, Y. Machii and M. Okahara, Synthesis, 1980, 650–651 CrossRef CAS;
(d) K. Hori, H. Sugihara, Y. N. Ito and T. Katsuki, Tetrahedron Lett., 1999, 40, 5207–5210 CrossRef CAS;
(e) Y.-M. Shen, M.-X. Zhao, J. Xu and Y. Shi, Angew. Chem., Int. Ed., 2006, 45, 8005–8008 CrossRef CAS PubMed.
- For the synthesis of activated aziridines from olefins including enones:
(a) D. A. Evans, M. M. Faul and M. T. Bilodeau, J. Org. Chem., 1991, 56, 6744–6746 CrossRef CAS;
(b) D. A. Evans, M. M. Faul and M. T. Bilodeau, J. Am. Chem. Soc., 1994, 116, 2742–2753 CrossRef CAS;
(c) S. Fioravanti, L. Pellacani, S. Tabanella and P. A. Tardella, Tetrahedron, 1998, 54, 14105–14112 CrossRef CAS;
(d) P. Dauban, L. Saniére, A. Tarrade and R. H. Dodd, J. Am. Chem. Soc., 2001, 123, 7707–7708 CrossRef CAS PubMed;
(e) T. Siu and A. K. Yudin, J. Am. Chem. Soc., 2002, 124, 530–531 CrossRef CAS PubMed;
(f) D. Colantoni, S. Fioravanti, L. Pellacani and P. A. Tardella, Org. Lett., 2004, 6, 197–200 CrossRef CAS PubMed;
(g) S. Fioravanti, D. Colantoni, L. Pellacani and P. A. Tardella, J. Org. Chem., 2005, 70, 3296–3298 CrossRef CAS PubMed.
- S. Minakata, Y. Murakami, R. Tsuruoka, S. Kitanaka and M. Komatsu, Chem. Commun., 2008, 6363–6365 RSC.
- Y. Murakami, Y. Takeda and S. Minakata, J. Org. Chem., 2011, 76, 6277–6285 CrossRef CAS PubMed.
- D. Tanner, Angew. Chem., Int. Ed., 1994, 33, 599–619 CrossRef.
- For enantioselective aziridination of enones:
(a) D. A. Evans, M. M. Faul, M. T. Bilodeau, B. A. Anderson and D. M. Barnes, J. Am. Chem. Soc., 1993, 115, 5328–5329 CrossRef CAS;
(b) J. Aires-de-Sousa, A. M. Lobo and S. Prabhakar, Tetrahedron Lett., 1996, 37, 3183–3186 CrossRef CAS;
(c) J. Aires-de-Sousa, S. Prabhakar, A. M. Lobo, A. M. Rosa, M. J. S. Gomes, M. C. Corvo, D. J. Williams and A. J. P. White, Tetrahedron: Asymmetry, 2002, 12, 3349–3365 CrossRef CAS;
(d) E. Murugan and A. Siva, Synthesis, 2005, 2022–2028 CrossRef CAS;
(e) A. Armstrong, C. A. Baxter, S. G. Lamont, A. R. Pape and R. Wincewicz, Org. Lett., 2007, 9, 351–353 CrossRef CAS PubMed;
(f) J. Vesely, I. Ibrahem, G.-L. Zhao, R. Rios and A. Córdova, Angew. Chem., Int. Ed., 2007, 46, 778–781 CrossRef CAS PubMed;
(g) F. Pesciaioli, F. D. Vincentiis, P. Galzerano, G. Bencivenni, G. Bartoli, A. Mazzanti and P. Melchiorre, Angew. Chem., Int. Ed., 2008, 47, 8703–8706 CrossRef CAS PubMed;
(h) L. Deiana, P. Dziedzic, G.-L. Zhao, J. Vesely, I. Ibrahem, R. Rios, J. Sun and A. Córdova, Chem.–Eur. J., 2011, 17, 7904–7917 CrossRef CAS PubMed;
(i) Y. Menjo, A. Hamashima, N. Sasaki and Y. Hamada, Org. Lett., 2011, 13, 5744–5747 CrossRef CAS PubMed;
(j) C. D. Fusco, T. Fusco, G. Croce and A. Lattanzi, Org. Lett., 2012, 14, 4078–4081 CrossRef PubMed;
(k) D. C. Cruz, P. A. Sánchez-Murcia and K. A. Jørgensen, Chem. Commun., 2012, 48, 6112–6114 RSC;
(l) M. Yamaguchi, M. Hayashi, Y. Hamada and T. Nemoto, Org. Lett., 2016, 18, 2347–2350 CrossRef CAS PubMed.
- For diastereoselective aziridination of enones:
(a) R. S. Atkinson, J. F. David, R. Russell and G. Tughan, J. Chem. Soc., Chem. Commun., 1986, 832–834 RSC;
(b) R. S. Atkinson and G. Tughan, J. Chem. Soc., Chem. Commun., 1986, 834–835 RSC;
(c) R. S. Atkinson and G. Tughan, J. Chem. Soc., Perkin Trans. 1, 1987, 2787–2796 RSC;
(d) R. S. Atkinson and G. Tughan, J. Chem. Soc., Perkin Trans. 1, 1987, 2797–2802 RSC;
(e) R. S. Atkinson and G. Tughan, J. Chem. Soc., Perkin Trans. 1, 1987, 2803–2807 RSC;
(f) Z. Chilmonczyk, M. Egli, C. Behringer and A. S. Dreiding, Helv. Chim. Acta, 1989, 72, 1095–1106 CrossRef CAS;
(g) J. T. Kapron, B. D. Santarsiero and J. C. Vederas, J. Chem. Soc., Chem. Commun., 1993, 1074–1076 RSC;
(h) P. Garnet, O. Dogan and S. Pillai, Tetrahedron Lett., 1994, 35, 1653–1656 CrossRef;
(i) G. Cardillo, L. Gentilucci, C. Tomasini and M. P. V. Castejon-Bordas, Tetrahedron: Asymmetry, 1996, 7, 755–762 CrossRef CAS;
(j) G. Cardillo, S. Casolari, L. Gentilucci and C. Tomasini, Angew. Chem., Int. Ed. Engl., 1996, 35, 1848–1849 CrossRef CAS;
(k) A. Bongini, G. Cardillo, L. Gentilucci and C. Tomasini, J. Org. Chem., 1997, 62, 9148–9153 CrossRef CAS;
(l) G. Cardillo, L. Gentilucci, I. R. Bastardas and A. Tolomelli, Tetrahedron, 1998, 54, 8217–8222 CrossRef CAS;
(m) R. S. Atkinson, E. Barker and S. Ulukanli, J. Chem. Soc., Perkin Trans. 1, 1998, 583–589 RSC;
(n) R. S. Atkinson and S. Ulukanli, J. Chem. Soc., Perkin Trans. 2, 1999, 771–776 RSC;
(o) K.-S. Yang and K. Chen, J. Org. Chem., 2001, 66, 1676–1679 CrossRef CAS PubMed;
(p) H. Ishihara, Y. N. Ito and T. Katsuki, Chem. Lett., 2001, 30, 984–985 CrossRef;
(q) R. S. Atkinson, R. D. Draycott, D. J. Hirst, M. J. Parratt and T. M. Raynham, Tetrahedron Lett., 2002, 43, 2083–2085 CrossRef CAS;
(r) H. Ishihara, K. Hori, H. Sugihara, Y. N. Ito and T. Katsuki, Helv. Chim. Acta, 2002, 85, 4272–4286 CrossRef CAS;
(s) S. Fioravanti, A. Morreale, L. Pellacani and P. A. Tardella, J. Org. Chem., 2002, 67, 4972–4974 CrossRef CAS PubMed;
(t) S. Fioravanti, A. Morreale, L. Pellacani and P. A. Tardella, Eur. J. Org. Chem., 2003, 4549–4552 CrossRef CAS;
(u) S. Fioravanti, A. Morreale, L. Pellacani and P. A. Tardella, Tetrahedron Lett., 2003, 44, 3031–3034 CrossRef CAS;
(v) S. Ulukanli, S. Karabuga, A. Celik and C. Kazaz, Tetrahedron Lett., 2005, 46, 197–199 CrossRef CAS;
(w) S. P. Bew, D. L. Hughes, V. Savic, K. M. Soapi and M. A. Wilson, Chem. Commun., 2006, 3513–3515 RSC;
(x) P.-W. Duan, C.-C. Chiu, W.-D. Lee, L. S. Pan, U. Venkatesham, Z.-H. Tzeng and K. Chen, Tetrahedron: Asymmetry, 2008, 19, 682–690 CrossRef CAS.
- M. Kirihara, T. Okada, Y. Sugiyama, M. Akiyoshi, T. Matsunaga and Y. Kimura, Org. Process Res. Dev., 2017, 21, 1925–1937 CrossRef CAS.
- S. Minakata, H. Miwa, K. Yamamoto, A. Hirayama and S. Okumura, J. Am. Chem. Soc., 2021, 143, 4112–4118 CrossRef CAS PubMed.
- Recently, S. Seo and S. Chang reported the facile preparation method of chloramine-Boc and quite interesting transformations using this nitrogen source, see:
(a) W. Lee, H. J. Jung, D. Kim, S. Seo and S. Chang, Chem, 2021, 7, 495–505 CrossRef CAS;
(b) H. J. Jeon, W. Lee, S. Seo and S. Chang, Org. Process Res. Dev., 2021, 25, 1176–1183 CrossRef CAS.
Footnote |
† Electronic supplementary information (ESI) available. See DOI: 10.1039/d1ra04297a |
|
This journal is © The Royal Society of Chemistry 2021 |
Click here to see how this site uses Cookies. View our privacy policy here.