DOI:
10.1039/D1RA04425G
(Paper)
RSC Adv., 2021,
11, 25624-25627
Access to 6-hydroxy indolizines and related imidazo[1,5-a]pyridines through the SN2 substitution/condensation/tautomerization cascade process†
Received
8th June 2021
, Accepted 19th July 2021
First published on 23rd July 2021
Abstract
A simple and efficient cascade reaction was developed for the construction of hydroxy substituted indolizines from pyrrole-2-carbaldehydes and commercially available 4-halogenated acetoacetic esters. Their optical properties were also evaluated.
Introduction
Indolizine, a biostere for indole, is commonly found in numerous natural products and pharmaceuticals. Indolizine derivatives exhibit diverse biological activities such as anti-HIV, anti-inflammatory, anti-tubercular, and anticancer activities.1–5 They are also used in dyes and optical materials owing to their bright colors.6–11 As a consequence, much effort has been devoted to their synthesis and functionalization, and thus many methods have been developed.12–14 In addition to classical Scholtz or Tschichibabin reactions, a variety of straightforward and efficient methods have been reported in recent years15–20 including 1,3-dipolar cycloaddition of pyridinium salts and intramolecular cyclization catalyzed by transition metals and intermolecular cyclization. Despite the efficiency of these methods, they suffer from the requirement of specific preorganized substrates, necessity of expensive metal catalysts, multistep synthesis, and a lack of product diversity. Moreover, no method has been reported for the preparation of indolizines bearing a hydroxyl group.
Recently, we synthesized a series of indolizine and related N-bridgehead heterocycles via a cascade reaction (Scheme 1a).21 To achieve the related pyrazolo[1,5-a]pyridines through a shorter and convenient route, a simple and efficient synthetic method was also reported subsequently using commercially accessible starting materials (Scheme 1b).22 Based on the results obtained in our laboratory, we expected that a cascade reaction of pyrrole-2-carbaldehyde 1 with 4-halogenated acetoacetic ester 2 might be successful in the presence of a weak base (Scheme 1). In continuation of our effort to search for new fluorophores for imaging,23–26 herein, we report a simple and efficient method for the synthesis of hydroxy substituted indolizines via an SN2 substitution/condensation/tautomerization cascade process in a metal-free fashion. Their optical properties were also evaluated.
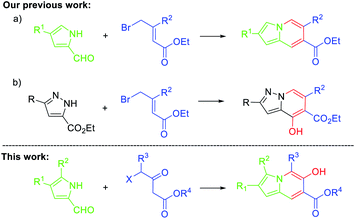 |
| Scheme 1 Access to indolizines via a cascade reaction. | |
Results and discussion
Initially, commercially available pyrrole-2-formaldehyde and ethyl 4-chloro-3-oxobutanoate 2a were selected for the experimental design. However, only the dimerization product of 2a was obtained. Subsequently, we optimized the reaction conditions using 4-propionyl-pyrrole-2-formaldehyde 1a and ethyl 4-chloro-3-oxobutanoate 2a as the model substrates. To our delight, the desired cyclized product 3a was obtained in an acceptable 56% yield in the presence of K2CO3 (Table 1, entry 1). The reaction even progressed very well at room temperature (entry 2). Other bases such as DBU, Cs2CO3, NaOH, t-BuOK, NaOAc, and CsOAc were then evaluated. However, the yields decreased, especially for a weak base (NaOAc) (entries 3–8). Regarding the effect of solvents, no improvement in the yield was obtained when the reaction was carried out in DMF, EtOH, and acetone (entries 9–11).
Table 1 Optimization of reaction conditionsa
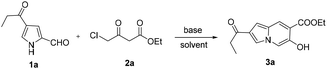
|
Entry |
Base |
T (°C) |
Solvent |
Time |
Yield (%) |
1 mmol 1a, 2 mmol 2a, 3 mmol base, and 10 mL solvent were used. |
1 |
K2CO3 |
50 |
MeCN |
6 h |
56 |
2 |
K2CO3 |
25 |
MeCN |
6 h |
70 |
3 |
DBU |
25 |
MeCN |
6 h |
54 |
4 |
Cs2CO3 |
25 |
MeCN |
6 h |
63 |
5 |
NaOH |
25 |
MeCN |
6 h |
42 |
6 |
t-BuOK |
25 |
MeCN |
6 h |
59 |
7 |
NaOAc |
25 |
MeCN |
6 h |
12 |
8 |
CsOAc |
25 |
MeCN |
6 h |
26 |
9 |
K2CO3 |
25 |
DMF |
6 h |
67 |
10 |
K2CO3 |
25 |
EtOH |
6 h |
60 |
11 |
K2CO3 |
25 |
Acetone |
6 h |
62 |
Then, the reactions of various substituted pyrrole-2-formaldehyde were tested (Scheme 2). Generally, the desired products were obtained in moderate-to-good yields when the pyrroles contained electron-withdrawing groups at the 4- or 5-position. However, no product was obtained for pyrrole-2-formaldehyde, presumably due to the reduction of nucleophilicity of the pyrrole ring. The structure of compound 3k was confirmed by X-ray crystal structure analysis (Fig. 1, CCDC 2081693†).
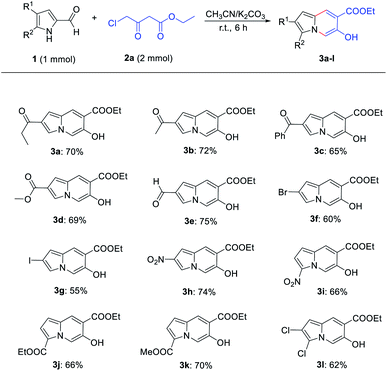 |
| Scheme 2 Substrate scope of pyrrole-2-formaldehyde. Reaction conditions: 1 (1 mmol), 2 (2 mmol), K2CO3 (3 mmol), CH3CN (10 mL), 20 °C, 6 h. | |
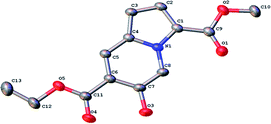 |
| Fig. 1 X-ray crystal structure of compound 3k. | |
Next, the applicability of this cascade reaction was expanded to the synthesis of imidazo[1,5-a]pyridine, furnishing the desired product 5a in 61% yield. The scope of the 4-halogenated acetoacetic ester was also evaluated (Scheme 3). The results indicate that the halogen and alkyl groups on position 4 and the ether group hardly influenced the yields.
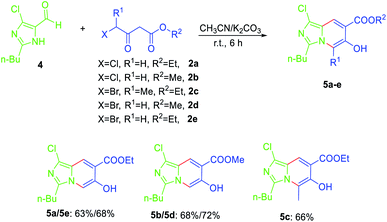 |
| Scheme 3 Substrate scope studies of 4-halogenated acetoacetic ester. Reaction conditions: 4 (1 mmol), 2 (2 mmol), K2CO3 (3 mmol), CH3CN (10 mL), 20 °C, 6 h. | |
Based on the above mentioned results and our previous work, we propose a mechanism as shown in Scheme 4. First, SN2 substitution of 4-halogenated acetoacetic ester 2 and pyrrole-2-formaldehyde 1 yields intermediate 6. Subsequently, cyclized intermediate 7 is formed through intramolecular nucleophilic substitution. Finally, the desired products 3 are obtained through dehydration and tautomerism.
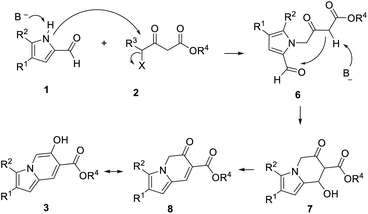 |
| Scheme 4 The proposed mechanism. | |
To advance our efforts to search for new fluorophores for cell imaging and their strong luminescence, we investigated the UV-vis and fluorescence spectra of these new compounds (Fig. 2). Compounds 3a–3l show similar absorptions at ca. 250 nm (Table S1†), which should be assigned to the π–π* electronic transition originating from the indolizine ring. Notably, the substituent and their position on the indolizine ring slightly affect these absorption peaks. However, the weak absorption bands between 290 nm and 445 nm due to n–π* electronic transition are especially different for compounds 3h and 3i containing a strong electron-withdrawing group (NO2). The maximum emission bands of 3c, 3e, and 3f are similar (425–455 nm, Table S1†), while those of 3a, 3b, and 3d are 540 nm, 535 nm, and 505 nm, respectively, with a much higher red shift.
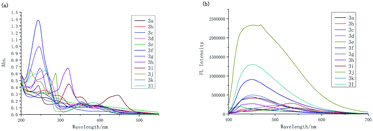 |
| Fig. 2 UV-vis and FL spectra of compounds 3a–3l. | |
Conclusions
In summary, we developed an efficient cascade reaction to construct indolizines and related imidazo[1,5-a]pyridines with a hydroxyl group, which is difficult to introduce through other methods. The structure was confirmed by single-crystal X-ray diffraction analysis. The compounds showed strong fluorescence in a dilute solution. Further studies on the optical properties of these indolizines are in progress.
Conflicts of interest
There are no conflicts to declare.
Acknowledgements
This work was supported by the Innovative Research Programs of Higher Education of Shandong Province (2019KJC009), the Science Fund of Shandong Province for Excellent Young Scholars (ZR2017JL015) and the Natural Science Foundation of China (21801180).
Notes and references
- K. M. Dawood and A. A. Abbas, Inhibitory activities of indolizine derivatives: a patent review, Expert Opin. Ther. Pat., 2020, 30, 695–714 CrossRef CAS PubMed.
- W. Huang, T. Zuo, H. Jin, Z. Liu, Z. Yang, X. Yu, L. Zhang and L. Zhang, Design, synthesis and biological evaluation of indolizine derivatives as HIV-1 VIF–ElonginC interaction inhibitors, Mol. Diversity, 2013, 17, 221–243 CrossRef CAS PubMed.
- R. S. Kumar, P. Antonisamy, A. I. Almansour, N. Arumugam, G. Periyasami, M. Altaf, H. R. Kim and K. B. Kwon, Functionalized spirooxindole-indolizine hybrids: stereoselective green synthesis and evaluation of anti-inflammatory effect involving TNF-α and nitrite inhibition, Eur. J. Med. Chem., 2018, 152, 417–423 CrossRef CAS PubMed.
- S. Park, E. H. Kim, J. Kim, S. H. Kim and I. Kim, Biological evaluation of indolizine–chalcone hybrids as new anticancer agent, Eur. J. Med. Chem., 2018, 144, 435–443 CrossRef CAS PubMed.
- Y. Xue, J. Tang, X. Ma, Q. Li, B. Xie, Y. Hao, H. Jin, K. Wang, G. Zhang, L. Zhang and L. Zhang, Synthesis and biological activities of indolizine derivatives as alpha-7 nAChR agonists, Eur. J. Med. Chem., 2016, 115, 94–108 CrossRef CAS PubMed.
- E. Kim, Y. Lee, S. Lee and S. B. Park, Discovery, Understanding, and bioapplication of organic fluorophore: a case study with an indolizine-based novel fluorophore, Seoul-Fluor, Acc. Chem. Res., 2015, 48, 538–547 CrossRef CAS PubMed.
- A. J. Huckaba, A. Yella, L. E. McNamara, A. E. Steen, J. S. Murphy, C. A. Carpenter, G. D. Puneky, N. I. Hammer, M. K. Nazeeruddin, M. Gratzel and J. H. Delcamp, Molecular design principles for near-infrared absorbing and emitting indolizine dyes, Chem.–Eur. J., 2016, 22, 15536–15542 CrossRef CAS PubMed.
- W. E. Meador, S. A. Autry, R. N. Bessetti, J. N. Gayton, A. S. Flynt, N. I. Hammer and J. H. Delcamp, Water-soluble NIR absorbing and emitting indolizine cyanine and indolizine squaraine dyes for biological imaging, J. Org. Chem., 2020, 85, 4089–4095 CrossRef CAS PubMed.
- R. Ji, A. Liu, S. Shen, X. Cao, F. Li and Y. Ge, An indolizine-rhodamine based FRET fluorescence sensor for highly sensitive and selective detection of Hg2+ in living cells, RSC Adv., 2017, 7, 40829–40833 RSC.
- Y. Ge, A. Liu, J. Dong, G. Duan, X. Cao and F. Li, A simple pH fluorescent probe based on new fluorophore indolizine for imaging of living cells, Sens. Actuators, B, 2017, 247, 46–52 CrossRef CAS.
- X. Zheng, R. Ji, X. Cao and Y. Ge, FRET-based ratiometric fluorescent probe for Cu2+ with a new indolizine fluorophore, Anal. Chim. Acta, 2017, 978, 48–54 CrossRef CAS PubMed.
- B. Sadowski, J. Klajn and D. T. Gryko, Recent advances in the synthesis of indolizines and their π-expanded analogues, Org. Biomol. Chem., 2016, 14, 7804–7828 RSC.
- D. Yadagiri, M. Rivas and V. Gevorgyan, Denitrogenative transformations of pyridotriazoles and related compounds: synthesis of N-containing heterocyclic compounds and beyond, J. Org. Chem., 2020, 85, 11030–11046 CrossRef CAS PubMed.
- S. Dong, X. Fu and X. Xu, [3 + 2]-Cycloaddition of catalytically generated pyridinium ylide: a general access to indolizine derivatives, Asian J. Org. Chem., 2020, 9, 1133–1143 CrossRef CAS.
-
(a) R. R. Liu, J. J. Hong, C. J. Lu, M. Xu, J. R. Gao and Y. X. Jia, Indolizine synthesis via oxidative cross-coupling/cyclization of alkenes and 2-(pyridin-2-yl)acetate derivatives, Org. Lett., 2015, 17, 3050–3053 CrossRef CAS PubMed;
(b) C. Dohmen, H. Ihmels, R. Kreienmeier and B. O. Patrick, Synthesis of a crystallochromic indolizine dye by a base- and catalyst-free photochemical route, Chem. Commun., 2019, 55, 11071–11074 RSC.
-
(a) Y. Liu, H. Hu, J. Zhou, W. Wang, Y. He and C. Wang, Synthesis of indolizine derivatives containing eight-membered rings via a gold-catalyzed twofold hydroarylation of diynes, Org. Biomol. Chem., 2017, 15, 5016–5024 RSC;
(b) B. Cheng, H. Li, S. Duan, X. Zhang, Y. He, Y. Li, Y. Li, T. Wang and H. Zhai, Application of primary halogenated hydrocarbons for the synthesis of 3-aryl and 3-alkyl indolizines, Org. Biomol. Chem., 2020, 18, 6253–6257 RSC;
(c) X. Wu, P. Zhao, X. Geng, J. Zhang, X. Gong, Y. D. Wu and A. X. Wu, Synthesis of indolizines from pyridinium 1,4-zwitterionic thiolates and propiolic acid derivatives via a formal [4 + 1] pathway, Org. Lett., 2017, 19, 3319–3322 CrossRef CAS PubMed.
-
(a) T. Jin, Z. Tang, J. Hu, H. Yuan, Y. Chen, C. Li, X. Jia and J. Li, Direct oxidative cleavage of multiple Csp3–H bonds and a C–C bond in 2-(pyridin-2-yl)acetate derivatives: formal [3 + 1 + 1] synthesis of 3-(pyridin-2-yl)indolizine skeletons, Org. Lett., 2018, 20, 413–416 CrossRef CAS PubMed;
(b) F. Sirindil, S. Golling, R. Lamare, J. M. Weibel, P. Pale and A. Blanc, Synthesis of indolizine and pyrrolo[1,2-a]azepine derivatives via a gold(I)-catalyzed three-step cascade, Org. Lett., 2019, 21, 8997–9000 CrossRef CAS PubMed;
(c) Y.-H. Zhang, Y.-H. Yuan, S.-Y. Zhang, Y.-Q. Tu and J.-M. Tian, Asymmetric intramolecular Friedel–Crafts reaction catalyzed by a spiropyrrolidine organocatalyst: Enantioselective construction of indolizine and azepine frameworks, Tetrahedron Lett., 2018, 59, 4015–4018 CrossRef CAS.
-
(a) T. Douglas, A. Pordea and J. Dowden, Iron-catalyzed indolizine synthesis from pyridines, diazo compounds, and alkynes, Org. Lett., 2017, 19, 6396–6399 CrossRef CAS PubMed;
(b) A. S. Kulandai Raj, K. C. Tan, L. Y. Chen, M. J. Cheng and R. S. Liu, Gold-catalyzed bicyclic annulations of 4-methoxy-1,2-dienyl-5-ynes with isoxazoles to form indolizine derivatives via an Au–p-allene intermediate, Chem. Sci., 2019, 10, 6437–6442 RSC.
-
(a) C. Jadala, V. Ganga Reddy, N. Hari Krishna, N. Shankaraiah and A. Kamal, Base-mediated 1,3-dipolar cycloaddition of pyridinium bromides with bromoallyl sulfones: a facile access to indolizine scaffolds, Org. Biomol. Chem., 2020, 18, 8694–8701 RSC;
(b) J. Li, S. Zhang and H. Zou, One-pot chemoselective domino condensation to form fused pyrrolo-pyrazino-indolizines framework: discovery of novel AIE molecules, Org. Chem. Front., 2020, 7, 1218–1223 RSC.
-
(a) C.-J. Lu, X. Yu, Y.-T. Chen, Q.-B. Song and H. Wang, Indolizine synthesis via copper-catalyzed cyclization of gem difluoroalkenes and 2-(pyridin-2-yl)acetate derivatives, Org. Chem. Front., 2020, 7, 2313–2318 RSC;
(b) S. Jin, L. Wang, H. Han, X. Liu, Z. Bu and Q. Wang, Assembly of functionalized p-extended indolizine polycycles through dearomative [3 + 2] cycloaddition/oxidative decarbonylation, Chem. Commun., 2021, 57, 359–362 RSC.
-
(a) Y. Q. Ge, J. Jia, H. Yang, X. T. Tao and J. W. Wang, The synthesis, characterization and optical properties of novel pyrido[1,2-a]benzimidazole derivatives, Dyes Pigm., 2011, 88, 344–349 CrossRef CAS;
(b) Y. Ge, J. Jia, H. Yang, G. Zhao, F. Zhan and J. Wang, A facile approach to indolizines via tandem reaction, Heterocycles, 2009, 78, 725–736 CrossRef CAS.
- P. Yan, G. Duan, R. Ji and Y. Ge, A simple and efficient synthesis of new fluorophore 4-hydroxy pyrazolo[1,5-a]pyridines through a tandem reaction, Tetrahedron Lett., 2018, 59, 2426–2429 CrossRef CAS.
-
(a) Y. Ge, X. Zheng, R. Ji, S. Shen and X. Cao, A new pyrido[1,2-a]benzimidazole-rhodamine FRET system as an efficient ratiometric fluorescent probe for Cu2+ in living cells, Anal. Chim. Acta, 2017, 965, 103–110 CrossRef CAS PubMed;
(b) Y. Ge, R. Ji, S. Shen, X. Cao and F. Li, A ratiometric fluorescent probe for sensing Cu2+ based on new imidazo[1,5-a]pyridine fluorescent dye, Sens. Actuators, B, 2017, 245, 875–881 CrossRef CAS;
(c) G. Duan, G. Zhang, S. Yuan, R. Ji, L. Zhang and Y. Ge, A pyrazolo[1,5-a]pyridine-based ratiometric fluorescent probe for sensing Cu2+ in cell, Spectrochim. Acta, Part A, 2019, 219, 173–178 CrossRef CAS PubMed;
(d) Y. Ge, A. Liu, R. Ji, S. Shen and X. Cao, Detection of Hg2+ by a FRET ratiometric fluorescent probe based on a novel pyrido[1,2-a]benzimidazole-rhodamine system, Sens. Actuators, B, 2017, 251, 410–415 CrossRef CAS.
-
(a) Y. Ge, X. Xing, A. Liu, R. Ji, S. Shen and X. Cao, A novel imidazo[1,5-a]pyridine-rhodamine FRET system as an efficient ratiometric fluorescent probe for Hg2+ in living cells, Dyes Pigm., 2017, 146, 136–142 CrossRef CAS;
(b) Y. Li, S. Qi, C. Xia, Y. Xu, G. Duan and Y. Ge, A FRET ratiometric fluorescent probe for detection of Hg2+ based on an imidazo[1,2-a]pyridine-rhodamine system, Anal. Chim. Acta, 2019, 1077, 243–248 CrossRef CAS PubMed;
(c) A. Liu, R. Ji, S. Shen, X. Cao and Y. Ge, A ratiometric fluorescent probe for sensing sulfite based on a pyrido[1,2-a]benzimidazole fluorophore, New J. Chem., 2017, 41, 10096–10100 RSC;
(d) Z. Xu, Z. Chen, A. Liu, R. Ji, X. Cao and Y. Ge, A ratiometric fluorescent probe for detection of exogenous mitochondrial SO2 based on a FRET mechanism, RSC Adv., 2019, 9, 8943–8948 RSC.
-
(a) F. Chen, A. Liu, R. Ji, Z. Xu, J. Dong and Y. Ge, A FRET-based probe for detection of the endogenous SO2 in cells, Dyes Pigm., 2019, 165, 212–216 CrossRef CAS;
(b) D. Zhang, A. Liu, R. Ji, J. Dong and Y. Ge, A mitochondria-targeted and FRET-based ratiometric fluorescent probe for detection of SO2 derivatives in water, Anal. Chim. Acta, 2019, 1055, 133–139 CrossRef CAS PubMed;
(c) G. Zhang, R. Ji, X. Kong, F. Ning, A. Liu, J. Cui and Y. Ge, A FRET based ratiometric fluorescent probe for detection of sulfite in food, RSC Adv., 2019, 9, 1147–1150 RSC;
(d) G. Song, A. Liu, H. Jiang, R. Ji, J. Dong and Y. Ge, A FRET-based ratiometric fluorescent probe for detection of intrinsically generated SO2 derivatives in mitochondria, Anal. Chim. Acta, 2019, 1053, 148–154 CrossRef CAS PubMed.
- Y. Ge, P. Wei, T. Wang, X. Cao, D. Zhang and F. Li, A simple fluorescent probe for monitoring pH in cells based on new fluorophore pyrido[1,2-a]benzimidazole, Sens. Actuators, B, 2018, 254, 314–320 CrossRef CAS.
Footnotes |
† Electronic supplementary information (ESI) available: 1H NMR, 13C NMR and HRMS spectra of compounds 3a–3l and 5a–5e. CCDC 2081693. For ESI and crystallographic data in CIF or other electronic format see DOI: 10.1039/d1ra04425g |
‡ Guiyun Duan and Hao Liu contributed equally. |
|
This journal is © The Royal Society of Chemistry 2021 |
Click here to see how this site uses Cookies. View our privacy policy here.