DOI:
10.1039/D1RA05134B
(Paper)
RSC Adv., 2021,
11, 30937-30942
Vitamin B1-catalyzed aerobic oxidative esterification of aromatic aldehydes with alcohols†
Received
3rd July 2021
, Accepted 24th August 2021
First published on 17th September 2021
Abstract
A straightforward aerobic oxidative esterification of aryl aldehydes with alcohols has been developed for the synthesis of substituted esters by employing vitamin B1 as a cost-effective, metal-free, and eco-friendly NHC catalyst. Air is used as a green terminal oxidant. The reaction is a useful addition to the existing NHC-catalytic oxidative esterification.
Introduction
Vitamin B1 (VB1), also known as thiamine, is one of the eight water-soluble B vitamins. In the human body, VB1 plays an essential role in the synthesis of nucleic acids (e.g., DNA), the conduction of nerve impulses, and the production of energy from food.1 The structure of VB1 contains a thiazole ring and a pyrimidine ring linked by a methylene bridge (Fig. 1). As an important subarea of biocatalysis, VB1 and its analogs have been widely used as non-toxic, low cost, and stable catalysts in diverse organic transformations2 including oxidation,3 cyclization,4 Michael addition, C–X bond coupling,5 benzoin condensation,6 Knoevenagel condensation,7 etc.8 Despite this impressive progress which has been achieved, reports related to the C–O bond formation by employing this renewably biobased catalyst are still highly desirable, offering benefits from an environment point of view.
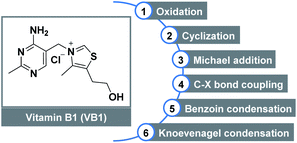 |
| Fig. 1 Thiamine (VB1) as a versatile catalyst in organic transformations. | |
The esters have been found a myriad of applications in fields ranging from agrochemistry, pharmacochemistry, and materials science to organic synthesis, mainly owing to their unique biological and chemical properties.9 The development of efficient strategies towards ester functionality has inspired chemists for more than 100 years, therefore, a variety of well-established methods are developed. Classical condensation of carboxylic acids with alcohols is carried out under strongly acidic conditions in the presence of a large excess of either substrate.10 In addition, esterification can also be achieved through the stoichiometric activation of the corresponding acids (e.g., acid halides, anhydrides, or activated esters) following nucleophilic substitution with appropriate alcohols. On the other hand, transition metal-catalyzed direct oxidative functionalization of aldehydes provides a powerful platform for ester synthesis over the past decades (Scheme 1A). Despite advances, these transformations commonly suffered from harsh reaction conditions, the use of costly metal catalysts, stoichiometric oxidants, and extra additives, which constitute drawbacks for large-scale applications and late-stage modifications.11 Alternatively, N-heterocyclic carbenes (NHCs) derived from thiazolium ions have the capability to accomplish the oxidative esterification with the assistance of various oxidants, such as MnO2,12 nitroarene,13 TEMPO,14 NFSI,15 diphenoquinone,16 azobenzene,17 phenazine,18 CCl3CN,19 and O2/air (Scheme 1B).20 From a viewpoint of green chemistry, a metal-free NHC-catalyzed one-pot esterification of aldehydes under air has become more sought after. In this regard, most of the existing methods relied on the preformed NHC catalysts which required multi-step synthesis. Continuing with our interests in green chemistry,21 herein we introduce the commercially available VB1 as an efficient catalyst for oxidative esterification (Scheme 1C). As a result, a cost-effective, metal-free, eco-friendly, and catalytic route that minimizes hazardous waste is developed for the synthesis of esters by employing a variety of aryl aldehydes and alcohols as coupling partners or reaction solvents. This metal-free approach offers a valuable alternative when compared to the above-mentioned methods: (i) biocompatible VB1 is used as an NHC catalyst; (ii) transition metal and expensive oxidant are not necessary; (iii) DABCO serves as a basic promoter. In particular, aerobic oxidation using dioxygen gas as a green oxidant is attractive.
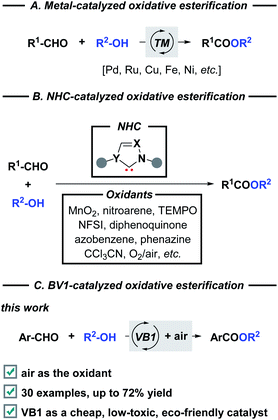 |
| Scheme 1 General routes for the oxidative esterification. | |
Results and discussion
We commenced the study by investigating the model reaction of 4-nitrobenzaldehyde (1a) with ethanol (2a) (Table 1). Gratifyingly, the reaction proceeded smoothly in the presence of VB1 (20 mol%) as a catalyst and triethylenediamine (DABCO, 2 equiv.) as a base at 40 °C for 6 h under air, leading to the desired coupled product 3aa in 58% yield (entry 1). In contrast, eroded efficiency was observed when the amount of VB1 was increased from 20 mol% to 30 mol% (entry 2), or when the amount of DABCO was increased from 2 equiv. to 3 equiv. (entry 3). Based on the control experiments, the necessity of adding catalyst and base were adequately demonstrated for the present reaction (entries 4 and 5). Subsequently, variations of various reaction parameters, including base, reaction temperature, reaction time, and solvent, were also carried out. It was found that DABCO was the base of choice, as the same reactions performed with other bases (including Na2CO3, Et3N, and NaOH) produced the corresponding product 3aa in low yields (entries 6–8). Furthermore, evaluation of reaction temperature and reaction time showed that the isolated yield of product 3aa could be increased to 65% when the reaction was performed at 80 °C for 12 h (entry 9). Interestingly, a low equivalent of ethanol (1 mmol) in CHCl3 did not considerably reduce the yield efficiency (49% yield, entry 10). Further screening of other solvents (e.g., DMSO, toluene, THF, and acetone) revealed that the reaction performance could be improved in acetone as the solvent (57% yield, entry 14). Finally, it was found that 63% yield could be obtained by carrying out the reaction at 60 °C in acetone for 12 h (entry 15). However, further improvement of the yield of product 3aa and diminishment of the undesired process were failed by replacing air with pure O2 (entry 16).
Table 1 Optimization of reaction conditionsa

|
Entry |
VB1 (x mol%) |
Base (y equiv.) |
Solvent |
T (°C) |
Yieldb (%) |
Reaction conditions: 4-nitrobenzaldehyde (1a, 1 mmol), VB1 (0–0.6 mmol), and base (0–3 mmol) in EtOH (2a, 2 mL) at 40–80 °C (oil bath) under air for 6 h. Isolated yield. 12 h. Reaction conditions: 4-nitrobenzaldehyde (1a, 3 mmol), EtOH (2a, 1 mmol), VB1 (0.2 mmol), and DABCO (2 mmol) in solvent (2 mL) at 40–60 °C (oil bath) under air for 12 h. O2 is used as an oxidant. |
1 |
20 |
DABCO (2) |
EtOH |
40 |
58 |
2 |
30 |
DABCO (2) |
EtOH |
40 |
49 |
3 |
20 |
DABCO (3) |
EtOH |
40 |
47 |
4 |
— |
DABCO (2) |
EtOH |
40 |
0 |
5 |
20 |
— |
EtOH |
40 |
0 |
6 |
20 |
Na2CO3 (2) |
EtOH |
40 |
<10 |
7 |
20 |
Et3N (2) |
EtOH |
40 |
10 |
8 |
20 |
NaOH (2) |
EtOH |
40 |
<10 |
9 |
20 |
DABCO (2) |
EtOH |
80 |
65c |
10 |
20 |
DABCO (2) |
CHCl3 |
40 |
49d |
11 |
20 |
DABCO (2) |
DMSO |
40 |
22d |
12 |
20 |
DABCO (2) |
Toluene |
40 |
Traced |
13 |
20 |
DABCO (2) |
THF |
40 |
Traced |
14 |
20 |
DABCO (2) |
Acetone |
40 |
57d |
15 |
20 |
DABCO (2) |
Acetone |
60 |
63d |
16 |
20 |
DABCO (2) |
Acetone |
60 |
61d,e |
With this optimized result in hand, we continued to study the substrate scope of the present esterification by employing a wide variety of structurally varied alcohols and aryl aldehydes as starting materials, and the results were summarized in Table 2. The aerobic oxidative esterification of 4-nitrobenzaldehyde (1a) worked equally well when the reactions were performed in different alcohols 2 as the solvents at 80 °C for 12–24 h, furnishing the corresponding esters 3aa–3aj in 18–65% yields. Interestingly, the chain length of the alkyl alcohols only had a slight impact on the reaction outcome (3aa–3ae, 52–65% yields). Notably, a sterically hindered secondary alcohol could be employed as substrate with equal ease (3ad, 53% yield). However, 2,2,2-trifluoroethan-1-ol with a strong electron-withdrawing CF3 group obviously reduced the reactivity presumably because of its low nucleophilicity (3af, 18% yield). To our delight, alcohols containing functional groups such as Cl atom (2g), alkene (2h), and alkyne (2i) were compatible with the mild reaction conditions, providing the anticipated products 3ag–3ai in 44–59% yields. Moreover, aryl aldehydes 1 bearing various substituents successfully underwent oxidative coupling with ethanol. In most cases, the cross-coupled products were produced in moderate yields (3ba–3fa). In addition to 4-nitrobenzaldehyde, aryl aldehydes possessing F, CN, I, and Br in the benzene ring were also proven to be appropriate substrates for the reaction (3ca–3fa). Unfortunately, further examination revealed that 4-methoxybenzaldehyde (1g) and heterocyclic aldehydes 1h–1j were reluctant to participate in the titled transformation. The failures could be attributed to the electronic effect. However, the reaction of 2-nitrobenzaldehyde (1k) with alcohol also failed to give the desired product, mainly owing to the negative effect of steric hindrance.
Table 2 Substrate scope of various alcohols and aryl aldehydesa
Reaction conditions: aryl aldehyde 1 (1.0 mmol), VB1 (0.2 mmol), and DABCO (2.0 mmol) in alcohol 2 (2.0 mL) at 80 °C (oil bath) for 12–24 h under air; isolated yields. |
 |
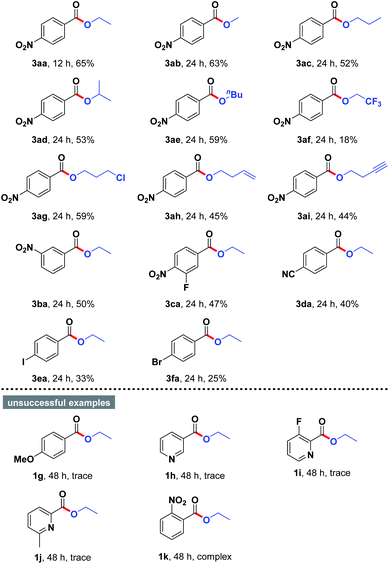 |
Encouraged by the above results, the generality of the substrate scope was further evaluated by applying different alcohols in acetone. As summarized in Table 3, benzyl alcohols 2 possessing either electron-donating group (e.g., Me, Me) or electron-withdrawing group (e.g., F, Cl, Br, and I) smoothly underwent the present organic transformations to give the desired esters 3ak–3ar in moderate to good yields. Notably, halogen functionality was satisfactorily compatible with the mild reaction conditions, which could be allowed for further divergent late-stage modifications (3ao–3ar). Similarly, thiophen-2-ylmethanol (2s), furan-2-ylmethanol (2t), and pyridin-2-ylmethanol (2v) were proven to be good candidates, affording the anticipated products 3as, 3at, and 3av in 63–69% yields. However, other heterocyclic substrate such as (1H-indol-3-yl)methanol (2u) could not participate into the reactions with 4-nitrobenzaldehyde (1a). In addition, we were able to accomplish the conversion of alkyl alcohols such as 2-(4-methoxyphenyl)ethan-1-ol (3aw), 2-(naphthalen-1-yl)ethan-1-ol (3ax), and cyclohexylmethanol (3ay) to the coupled products 3aw–3ay in 46–66% yields. Remarkably, (E)-3-phenylprop-2-en-1-ol can be easily converted into 3az in 62% yield. During the course of our studies, other types of nucleophiles including phenol 2a′ and amine 2b′ failed to react under the optimized reaction conditions.
Table 3 Substrate scope of various alcohols 2
a
Reaction conditions: 4-nitrobenzaldehyde (1a, 3.0 mmol), alcohol 2 (1.0 mmol), VB1 (0.2 mmol), and DABCO (2.0 mmol) in acetone (2.0 mL) at 60 °C (oil bath) for 24 h under air; isolated yields. |
 |
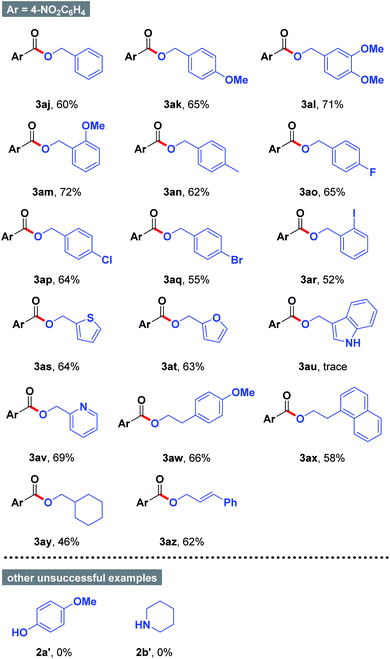 |
Subsequently, a scale-up reaction was also applied to the model reaction (Scheme 2A). It was found that 10 mmol scale reaction worked with equally high efficiency under the well-established reaction conditions to produce the desired product 3aa in 61% yield. Additionally, when the reaction of 4-nitrobenzaldehyde and ethanol was performed under N2 atmosphere, the formation of product 3aa was almost completely inhibited (Scheme 2B). This result suggests that the presence of O2 is important for the intermolecular oxidative coupling reaction.22 Furthermore, a radical trapping experiment by using 2,2,6,6-tetramethylpiperidinooxy (TEMPO, 1.0 equiv.) as a radical inhibitor provided clear evidence that the reaction might not proceed in a radical manner (Scheme 2C).
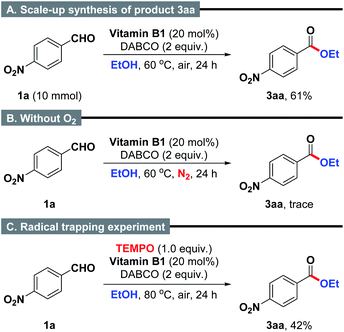 |
| Scheme 2 Scale-up synthesis of product 3aa and control experiment. | |
Based on the control experiment and a literature survey,12–20 a proposed mechanism is depicted in Scheme 3. Initially, deprotonation of VB1 under the basic condition gives rise to carbene catalyst A. Subsequently, the resulting species reacts with aryl aldehyde to produce the Breslow intermediate B, which in the presence of air can be further oxidized to acyl azolium intermediate C. Next, an intermolecular nucleophilic attack from the alcoholic OH occurred to give the desired product and regenerate the NHC catalyst.
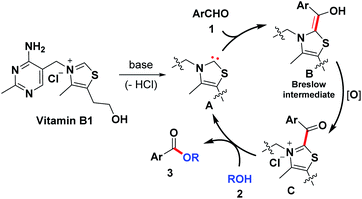 |
| Scheme 3 Proposed mechanism. | |
Conclusions
In summary, we have developed a vitamin B1-catalyzed aerobic oxidative esterification of aryl aldehydes with alcohols, providing easy access to synthetically important ester derivatives in moderate yields. The reaction proceeds in the air without an external oxidant. Moreover, commercially available VB1 was used as a cost-effective, metal-free, eco-friendly, and green catalyst, complementing the previously reported NHC-catalytic methods for the esterification.
Experimental
General procedure of vitamin B1-catalyzed aerobic oxidative esterification for the synthesis of products 3
Method 1. A solution of aldehyde (1 mmol), vitamin B1 (60.2 mg, 0.2 mmol), and triethylenediamine (DABCO, 224.3 mg, 2 mmol) in alcohol (2 mL) was stirred at 80 °C under air for 12–24 h. The reaction was then quenched by saturated NH4Cl solution (20 mL) and extracted with EtOAc (20 mL × 3). The organic layer was washed with saturated brine twice, dried over MgSO4, filtered, and concentrated under reduced pressure. The crude product was purified by flash column chromatography (300–400 mesh) using petroleum ether/ethyl acetate (100/1–2/1) as eluent to afford the pure products 3.
Method 2. A solution of 4-nitrobenzaldehyde (453.4 mg, 3 mmol), alcohol (1 mmol), vitamin B1 (60.2 mg, 0.2 mmol), and triethylenediamine (DABCO, 224.3 mg, 2 mmol) in acetone (2 mL) was stirred at 60 °C under air for 24 h. The reaction was then quenched by saturated NH4Cl solution (20 mL) and extracted with EtOAc (20 mL × 3). The organic layer was washed with saturated brine twice, dried over MgSO4, filtered, and concentrated under reduced pressure. The crude product was purified by flash column chromatography (300–400 mesh) using petroleum ether/ethyl acetate (100/1–2/1) as eluent to afford the pure products 3.
General procedure for the scale-up synthesis of pyridine 3aa
A solution of 4-nitrobenzaldehyde (1.51 g, 10 mmol), vitamin B1 (601.6 mg, 2 mmol), and triethylenediamine (DABCO, 2.24 g, 20 mmol) in EtOH (20 mL) was stirred at 80 °C under air for 24 h. The reaction solvent was removed under reduced pressure, then the reaction mixture was quenched by saturated NH4Cl solution (20 mL) and extracted with EtOAc (20 mL × 3). The organic layer was washed with saturated brine twice, dried over MgSO4, filtered, and concentrated under reduced pressure. The crude product was purified by flash column chromatography (300–400 mesh) using petroleum ether/ethyl acetate (100/1–2/1) as eluent to afford the pure products 3aa (1.19 g, 61% yield).
Conflicts of interest
There are no conflicts to declare.
Acknowledgements
We gratefully acknowledge the financial support from Xuzhou Medical University (Start-up Grant no. RC20552038), Nanjing Tech University (start-up grant no. 39837146), National Students' platform for innovation and entrepreneurship training program (202110291019Z), Natural Science Foundation of Jiangsu Province (BK20180690), and National Natural Science Foundation of China (22001121).
Notes and references
-
(a) C. J. Abularrage, A. N. Sidawy, P. W. White, G. Aidinian, K. J. DeZee, J. M. Weiswasser and S. Arora, Effect of Folic Acid and Vitamins B6 and B12 on Microcirculatory Vasoactivity in Patients with Hyperhomocysteinemia, Vascular and Endovascular Surgery., 2007, 41, 339–345 CrossRef PubMed;
(b) W. G. Christen, R. J. Glynn, E. Y. Chew, C. M. Albert and J. E. Manson, Folic Acid, Pyridoxine, and Cyanocobalamin Combination Treatment and Age-Related Macular Degeneration in WomenThe Women's Antioxidant and Folic Acid Cardiovascular Study, Arch. Intern. Med., 2009, 169, 335–341 CrossRef CAS PubMed.
- R. Singh, S. A. Ganaie and A. Singh, Vitamin B1: A versatile organocatalyst for organic synthesis, Curr. Organocatal., 2017, 4, 84–103 CAS.
- Y. Liu, C. Wang, D. Xue, M. Xiao, J. Liu, C. Li and J. Xiao, Reactions Catalysed by a Binuclear Copper Complex: Relay Aerobic Oxidation of N-Aryl Tetrahydroisoquinolines to Dihydroisoquinolones with a Vitamin B1 Analogue, Chem.–Eur. J., 2017, 23, 3062–3066 CrossRef CAS PubMed.
- M. Lei, L. Ma and L. Hu, One-Pot Synthesis of 1H-Benzimidazole Derivatives Using Thiamine Hydrochloride as A Reusable Organocatalyst, Synth. Commun., 2012, 42, 2981–2993 CrossRef CAS.
-
(a) V. B. Purohit, S. C. Karad, K. H. Patel and D. K. Raval, Palladium N-heterocyclic carbene catalyzed regioselective thiolation of 1-aryl-3-methyl-1H-pyrazol-5(4H)-ones using aryl thiols, Tetrahedron, 2016, 72, 1114–1119 CrossRef CAS;
(b) V. B. Purohit, S. C. Karad, K. H. Patel and D. K. Raval, Palladium N-heterocyclic carbene catalyzed expected and unexpected C–C and C–N functionalization reactions of 1-aryl-3-methyl-1H pyrazol-5(4H)-ones, RSC Adv., 2016, 6, 111139–111143 RSC.
-
(a) T. Lu, X. Li, L. Gu and Y. Zhang, Vitamin B1-Catalyzed Acetoin Formation from Acetaldehyde: A Key Step for Upgrading Bioethanol to Bulk C4 Chemicals, ChemSusChem, 2014, 7, 2423–2426 CrossRef CAS PubMed;
(b) Y. Chen, W. Shan, M. Lei and L. Hu, Thiamine hydrochloride (VB1) as an efficient promoter for the one-pot synthesis of 2,3-dihydroquinazolin-4(1H)-ones, Tetrahedron Lett., 2012, 53, 5923–5925 CrossRef CAS.
-
(a) I. R. Siddiqui, P. Rai, Rahila, A. Srivastava, A. Srivastava and A. Srivastava, Synthesis of fused pyridines in the presence of thiamine hydrochloride as an efficient and reusable catalyst in aqueous conditions, New J. Chem., 2013, 37, 3798–3804 RSC;
(b) K. Pradhan, P. Bhattacharyya, S. Paul and A. R. Das, Synthesis of 3,4-dihydropyridin-2-one derivatives in convergent mode applying bio catalyst vitamin B1 and polymer supported catalyst PEG–SO3H from two different sets of building blocks, Tetrahedron Lett., 2012, 53, 5840–5844 CrossRef CAS;
(c) M. Lei, L. Ma and L. Hu, Highly Chemoselective Condensation of β-Naphthol, Aldehyde, and Urea Catalyzed by Thiamine Hydrochloride, Synth. Commun., 2011, 41, 3424–3432 CrossRef CAS;
(d) M. Lei, L. Ma and L. Hu, VB1–Al2O3-catalyzed one-pot condensation of aromatic ketone, aromatic aldehyde, and amide, Tetrahedron Lett., 2010, 51, 4746–4749 CrossRef CAS;
(e) J. Liu, M. Lei and L. Hu, Thiamine hydrochloride (VB1): an efficient promoter for the one-pot synthesis of benzo[4,5]imidazo[1,2-a]pyrimidine and [1,2,4]triazolo[1,5-a] pyrimidine derivatives in water medium, Green Chem., 2012, 14, 840–846 RSC.
-
(a) M. Lei, L. Ma and L. Hu, A convenient one-pot synthesis of formamide derivatives using thiamine hydrochloride as a novel catalyst, Tetrahedron Lett., 2010, 51, 4186–4188 CrossRef CAS;
(b) K. Azizi and A. Heydari, Vitamin B1 supported on silica-encapsulated γ-Fe2O3 nanoparticles: design, characterization and application as a greener biocatalyst for highly efficient acylation, RSC Adv., 2014, 4, 8812–8816 RSC.
- J. Otera and J. Nishikido, Esterification: Methods, Reactions, and Applications, Wiley-VCH, Weinheim, 2nd edn, 2010 Search PubMed.
- For selected examples, see:
(a) K. Ishihara, S. Ohara and H. Yamamoto, Direct Condensation of Carboxylic Acids with Alcohols Catalyzed by Hafnium(IV) Salts, Science, 2000, 290, 1140–1142 CrossRef CAS PubMed;
(b) K. Manabe, X.-M. Sun and S. Kobatashi, Dehydration Reactions in Water. Surfactant-Type Brønsted Acid-Catalyzed Direct Esterification of Carboxylic Acids with Alcohols in an Emulsion System, J. Am. Chem. Soc., 2001, 123, 10101–10102 CrossRef CAS PubMed.
-
(a) X.-F. Wu and C. Darcel, Iron-Catalyzed One-Pot Oxidative Esterification of Aldehydes, Eur. J. Org. Chem., 2009, 2009, 1144–1147 CrossRef;
(b) C. Liu, J. Wang, L.-K. Meng, Y. Deng, Y. Li and A.-W. Lei, Palladium-Catalyzed Aerobic Oxidative Direct Esterification of Alcohols, Angew. Chem., Int. Ed., 2011, 50, 5144–5148 CrossRef CAS PubMed;
(c) M.-L. Zhang, S.-H. Zhang, G.-Y. Zhang, F. Chen and J. Cheng, Palladium/NHC-catalyzed oxidative esterification of aldehydes with phenols, Tetrahedron Lett., 2011, 52, 2480–2483 CrossRef CAS;
(d) L. Fu, C.-J. Yao, N.-J. Chang, J.-R. Chen, L.-Q. Lu and W.-J. Xiao, Oxidative cross-esterification of dithiolanes with alcohols through a cross-dehydrogenative coupling (CDC)/deprotection sequence, Org. Biomol. Chem., 2012, 10, 506–508 RSC;
(e) W.-J. Yoo and C.-J. Li, Copper-catalyzed oxidative esterification of alcohols with aldehydes activated by Lewis acids, Tetrahedron Lett., 2007, 48, 1033–1035 CrossRef CAS.
- B. Maji, S. Vedachalan, X. Ge, S.-T. Cai and X.-W. Liu, N-Heterocyclic Carbene-Mediated Oxidative Esterification of Aldehydes: Ester Formation and Mechanistic Studies, J. Org. Chem., 2011, 76, 3016–3023 CrossRef CAS PubMed.
- J. Castells, F. Pujol, H. Llitjós and M. Moreno-Mañas, Oxidative benzoin reactions, Tetrahedron, 1982, 38, 337–346 CrossRef CAS.
- S. D. Sarkar, S. Grimme and A. Studer, NHC Catalyzed Oxidations of Aldehydes to Esters: Chemoselective Acylation of Alcohols in Presence of Amines, J. Am. Chem. Soc., 2010, 132, 1190–1191 CrossRef PubMed.
- X. Dong, W. Yang, W. Hu and J. Sun, N-Heterocyclic Carbene Catalyzed Enantioselective α-Fluorination of Aliphatic Aldehydes and α-Chloro Aldehydes: Synthesis of α-Fluoro Esters, Amides, and Thioesters, Angew. Chem., Int. Ed., 2015, 54, 660–663 CAS.
- S. De Sarkar, S. Grimme and A. Studer, NHC Catalyzed Oxidations of Aldehydes to Esters: Chemoselective Acylation of Alcohols in Presence of Amines, J. Am. Chem. Soc., 2010, 132, 1190–1191 CrossRef CAS PubMed.
- C. Noonan, L. Baragwanath and S. J. Connon, Nucleophilic carbene-catalysed oxidative esterification reaction, Tetrahedron Lett., 2008, 49, 4003–4006 CrossRef CAS.
- X. Zhao, K. E. Ruhl and T. Rovis, N-Heterocyclic-Carbene-Catalyzed Asymmetric Oxidative Hetero-Diels–Alder Reactions with Simple Aliphatic Aldehydes, Angew. Chem., Int. Ed., 2012, 51, 12330–12333 CrossRef CAS PubMed.
- Z. Wu, D. Jiang and J. Wang, Carbene-catalyzed oxidative acylation promoted by an unprecedented oxidant CCl3CN, Org. Chem. Front., 2019, 6, 688–693 RSC.
- W. Harnying, P. Sudkaow, A. Biswas and A. Berkessel, N-Heterocyclic Carbene/Carboxylic Acid Co-Catalysis Enables Oxidative Esterification of Demanding Aldehydes/Enals, at Low Catalyst Loading, Angew. Chem., Int. Ed., 2021, 60, 19631–19636 CrossRef CAS PubMed.
-
(a) T. Xie, G.-Q. Wang, Y.-W. Wang, W. Rao, H. Xu, S. Li, Z.-L. Shen and X.-Q. Chu, Selective Quadruple C(sp3)-F Functionalization of Polyfluoroalkyl Ketones, iScience, 2020, 23, 101259 CrossRef CAS PubMed;
(b) Q.-D. Wang, Y.-W. Wang, T. Xie, Y.-Y. Cui, M. Ma, Z.-L. Shen and X.-Q. Chu, Three-Component Bisannulation for the Synthesis of Trifluoromethylated Tetracyclic Aza-Aromatics through Six C(sp3)-F Bond Cleavage and Four C-N Bond Formation, J. Org. Chem., 2021, 86, 8236–8247 CrossRef CAS PubMed;
(c) D. Ge, X.-L. Luo, X. Tang, C.-B. Pang, X. Wang and X.-Q. Chu, Metal-Free [3 + 2 + 1] Annulation of Allylic Alcohols, Ketones, and Ammonium Acetate: Radical-Involving Synthesis of 2,3-Diarylpyridine Derivatives, Org. Biomol. Chem., 2021, 19, 2277–2283 RSC;
(d) D. Ge, X. Wang and X.-Q. Chu, SOMOphilic Alkynylation Using Acetylenic Sulfones as Functional Reagents, Org. Chem. Front., 2021, 8, 5145–5164 RSC;
(e) S.-Z. Cai, D. Ge, L.-W. Sun, W. Rao, X. Wang, Z.-L. Shen and X.-Q. Chu, Three-Component Heteroannulation for Tetrasubstituted Furan Construction Enabled by Successive Defluorination and Dual Sulfonylation Relay, Green Chem., 2021, 23, 935–941 RSC;
(f) X.-Q. Chu, D. Ge, Y.-Y. Cui, Z.-L. Shen and C.-J. Li, Desulfonylation via Radical Process: Recent Developments in Synthetic Applications, Chem. Rev., 2021 DOI:10.1021/acs.chemrev.1c00084.
- For selected examples of oxidation reactions with air as the oxidant, see:
(a) K.-J. Liu, Z. Wang, L.-H. Lu, J.-Y. Chen, F. Zeng, Y.-W. Lin, Z. Cao, X. Yu and W.-M. He, Synergistic cooperative effect of CF3SO2Na and bis(2-butoxyethyl)ether towards selective oxygenation of sulfides with molecular oxygen under visible-light irradiation, Green Chem., 2021, 23, 496–500 RSC;
(b) K.-J. Liu, J.-H. Deng, T.-Y. Zeng, X.-J. Chen, Y. Huang, Z. Cao, Y.-W. Lin and W.-M. He, 1,2-Diethoxyethane catalyzed oxidative cleavage of gem-disubstituted aromatic alkenes to ketones under minimal solvent conditions, Chin. Chem. Lett., 2020, 31, 1868–1872 CrossRef CAS.
Footnotes |
† Electronic supplementary information (ESI) available. See DOI: 10.1039/d1ra05134b |
‡ X.-L. Luo and D. Ge contributed equally to this work. |
|
This journal is © The Royal Society of Chemistry 2021 |
Click here to see how this site uses Cookies. View our privacy policy here.