DOI:
10.1039/D1RA05388D
(Paper)
RSC Adv., 2021,
11, 32312-32328
A new simple, highly sensitive and selective spectrofluorimetric method for the speciation of thallium at pico-trace levels in various complex matrices using N-(pyridin-2-yl)-quinoline-2-carbothioamide†
Received
13th July 2021
, Accepted 7th September 2021
First published on 30th September 2021
Abstract
A very simple and non-extractive new spectrofluorimetric method for the determination of TlI and TlIII individually and for mixtures of both analytes at pico-trace levels using N-(pyridin-2-yl)-quinoline-2-carbothioamide (PQCTA) has been developed. PQCTA reacts in a slightly acidic (0.0025–0.05 M H2SO4) solution with TlIII in 20% ethanol and is oxidized itself to produce highly fluorescent oxidized product in aqueous solution, which has the excitation and emission wavelengths of λex = 324 nm and λem = 379 nm, respectively. The determination of TlI is based on the rapid oxidation of this ion by bromine water heating for 15 min with the concomitant formation of fluorescent TlIII-PQCTA. Fluorescence due to the sum of TlI and TlIII is measured and TlI is determined from the difference in fluorescence values. Constant and maximum fluorescence intensities were observed for the period between 2 min and 24 h. Linear calibration graphs were obtained for 0.001–600 μg L−1 of Tl, providing a detection limit of 0.16 ng L−1. The quantification limit of the reaction system was 1.6 ng L−1 and the RSD was 0–2%. A large excess of over 80 cations, anions, and complexing agents (such as chloride, phosphate, azide, tartrate, oxalate, and SCN−) do not interfere in the determination. The developed method was successfully used in the determination of thallium in several Standard Reference Materials (SRM) as well as in some environmental waters, biological fluids, soil and food samples, solutions containing both TlI and TlIII, and complex synthetic mixtures. The results of the proposed method for biological samples, vegetables, food, soil, and water analyses were found to be in excellent agreement with those obtained by ICP-OES, AAS, and ICP-MS.
Introduction
Thallium (Tl) is an extremely toxic heavy metal that can cause severe damage to all organisms in both the Tl(I) and Tl(III) oxidation states.1 Despite its high toxicity, Tl is much less studied than the other widely studied heavy metals such as mercury, cadmium, and lead.2 The more stable Tl(I) species tends to create stable toxic complexes with sulfur-containing compounds, while Tl(III) is prone to form organic complexes of greater stability with DTPA3 or EDTA.4 Therefore, each redox state of Tl exhibits different bioavailability and toxicity properties.5 Thallium is a cumulative poison with high toxicity and is without taste, smell, and other altering attributes. The element is also toxic in the dust form as it oxidizes on contact with air. Food and respiratory thallium poisonings are possible. One of the characteristic poisoning symptoms is hair loss preceded by hair follicle atrophy. Other signs include digestion disorders, pain, neuropsychiatric complications, as well as cardiovascular system damages.6 However, in contrast to other elements, little is known about the chemical speciation of Tl in the environment with related ecological effect and potential human health hazards.7 In the past years, increasing attention has been paid to Tl speciation in different environmental media due to its toxic effect on humans, aquatic life, and its distribution in the food chain. Recently, several river waters, food, and sediment contaminations by Tl have been reported,8 which suggests an urgent need to systematically study the Tl-related environmental chemistry.9 Tl is released into the environment from natural and anthropogenic processes. Thallium exposure may occur at smelters in the maintenance and cleaning of ducts and flues and through contamination of cocaine, heroin, and herbal products. Criminal and unintentional thallium poisonings are still reported, some leading to death.10 Thallium and its compounds can also enter the organism of persons working with them through the respiratory organs, through the gastrointestinal tract, and through the skin.11 It is also found in cigarette smoke and is sometimes used in fireworks, pigments, and even jewelry. Thus, even thallium is a highly toxic element, it is very much a part of our daily lives. Therefore, the accurate determination of trace and ultra-trace levels using simple and rapid methods has great importance.
Many methods have been proposed for the determination of thallium and its speciation in environmental, biological, and soil samples, which includes inductively coupled plasma mass spectrometry (ICP-MS),12,13 ICP-OES,14–16 flame atomic absorption spectrophotometry (AAS),17 electrothermal atomic absorption spectrophotometry (EAAS),18 HPLC,19 spectrophotometry,20 differential-pulse anodic stripping voltammetry (DPASV),21 and spectrofluorimetry.22–40 ICP-MS and ICP-OES, being highly expensive, are not used for routine analysis in developing countries such as Bangladesh. AAS is sensitive but suffers from sample matrix interferences, such as salinity.41 However, the spectrofluorimetry is essentially a trace analysis technique and is one of the most powerful tools in chemical analysis. This method still has the advantages of being simple and does not require expensive or complicated test equipment. For this reason, a wide variety of spectrofluorimetric methods for the determination of thallium has been developed.22–40 Several authors have reported on the extractive spectrofluorimetric determination for speciation of thallium using complexes formed via a variety of reagents.22–40 In most of the methods25–27,30–33 cited in the literature, as shown in Table 1, thallium forms soluble or insoluble complexes with those reagents with various organic solvents for the spectrofluorimetric determination of thallium. Most of these reagents are expensive and non-recoverable, and most of the organic solvents used are carcinogenic according to EPA.42 However, interference from coexisting ions, especially some rare earth ions, Fe3+, and PO43−, is always faced in the existing methods.
Table 1 Summary of the reviews on the existing spectrofluorimetric methods for the determination of thallium
Ligands |
Analytical parameters |
Results |
Remarks |
Ref. |
Hydrazine dihydrochloride |
Solvent |
Ethanol |
Beer's law 70–4350 |
(i) Less sensitive |
25 |
Medium |
Aqueous |
Detection limit 31 |
(ii) pH dependent |
pH/acidity |
7.0 |
RSD% 3 |
(iii) Less selective due to much interference |
λex : λem |
227 : 419 |
|
(iv) Quenching of fluorimetric response |
Interference |
SO32−, Br−, Ni2+, Co2+, Hg2+, V5+, As3+, Cd2+, Hg2+, Fe2+, Ce4+, etc. |
|
|
![[thin space (1/6-em)]](https://www.rsc.org/images/entities/char_2009.gif) |
Pyrogallol red (PR) |
Solvent |
Methanol |
Beer's law 0–400 |
(i) Time consuming |
27 |
Medium |
Amphoteric surfactant |
Detection limit 50 |
(ii) Less sensitive |
pH/acidity |
7.8 |
RSD% 2.5 |
(iii) Less selective due to much interference |
λex:λem |
525 : 545 |
|
(iv) pH-dependent |
Interference |
Fe2+, Ni2+, Co2+, Al3+, etc. |
|
|
![[thin space (1/6-em)]](https://www.rsc.org/images/entities/char_2009.gif) |
Thiourea |
Solvent |
Chloroform |
Beer's law 10–2043 |
(i) Solvent extractive |
30 |
Medium |
Solvent extraction |
Detection limit 20 |
(ii) Less sensitive |
pH/acidity |
3.5 |
RSD% 7 |
(iii) Less selective due to much interference |
λex : λem |
227 : 419 |
|
(iv) pH-dependent, hence lengthy and time consuming |
Interference |
Br−, Ce4+, Sb3+, I−, Fe2+ |
|
|
![[thin space (1/6-em)]](https://www.rsc.org/images/entities/char_2009.gif) |
3-p-Chlorophenyl-5-(2′-arsenoxylphenylazo) rhodanine |
Solvent |
CCl4 |
Beer's law 0.43–10 |
(i) Solvent extractive |
31 |
Medium |
Ether |
Detection limit 26 |
(ii) Lengthy & time consuming |
pH/acidity |
5.2 |
RSD% 7 |
(iii) Less sensitive |
λex : λem |
309 : 406 |
|
(iv) Less selective due to much interference |
Interference |
Fe3+, Cr3+, Al3+, Zr2+, Ni2+, etc. |
|
(v) pH-dependent |
![[thin space (1/6-em)]](https://www.rsc.org/images/entities/char_2009.gif) |
Methylene blue |
Solvent |
Water |
Beer's law 50–5000 |
(i) Less sensitive |
32 |
Medium |
Aqueous |
Detection limit 40 |
(ii) pH dependent |
pH/acidity |
5.0 |
RSD% 10 |
(iii) Less selective due to much interference |
λex : λem |
468 : 730 |
|
(iv) Less sensitive |
Interference |
Many |
|
|
![[thin space (1/6-em)]](https://www.rsc.org/images/entities/char_2009.gif) |
Potassium iodide |
Solvent |
Water |
Beer's law 85–360 |
(i) Lengthy & time consuming |
33 |
Medium |
Fluorescence quenching |
Detection limit 45 |
(ii) Less sensitive |
pH/acidity |
7.0 |
RSD% 5 |
(iii) Less selective due to much interference |
λex : λem |
435 : 650 |
|
(iv) pH-dependent |
Interference |
Many |
|
|
![[thin space (1/6-em)]](https://www.rsc.org/images/entities/char_2009.gif) |
N-(Pyridin-2-yl)-quinoline-2-carbothioamide (PQCTA) |
Solvent |
Ethanol |
Beer's law: 0.001–600 μg L−1 |
(i) Ultra-sensitive |
Present Method and ref. 41 |
Medium |
Aqueous |
Detection limit: 0.16 ng L−1 |
(ii) Highly selective |
pH/acidity |
0.0025–0.05 M H2SO4 |
RSD% 0–2 |
(iii) Pico-trace levels determination (pg mL−1) |
λex : λem |
324 : 379 |
|
(iv) Fluorescence is stable for 24 h |
Interference |
Nil (using suitable masking agents) |
|
(v) Very simple, rapid, and non-extractive |
Thallium (Tl) is a typically toxic element, whose biological effects and geochemical behavior are closely related to its chemical speciation in the environment. In this context, the aim of this study was to develop a simpler direct spectrofluorimetric method for the pico-trace determination of thallium. In the search for a more sensitive reagent, in this work, a new reagent was synthesized, according to the method of Porter,43 and the oxidation reaction of N-(pyridin-2-yl)-quinoline-2-carbothioamide (PQCTA) with thalliumIII formed an intensively fluorescent oxidized product. The determination of TlI is based on the rapid oxidation of this ion by bromine water heating for 15 min with the concomitant formation of fluorescent TlIII-PQCTA. Fluorescence due to the sum of TlI and TlIII is measured and TlI is determined from the difference in fluorescence. PQCTA has been reported as a spectrofluorimetric reagent but, to our knowledge, it has not been used previously for the simultaneous determination of TlI and TlIII in a fluorescence system. The method possesses distinct advantages over existing methods,25–32,44–49 as shown in Table 1, with respect to several analytical parameters (Table 1). The method is based on the oxidative reaction of non-fluorescent PQCTA in a slightly acidic (0.0025–0.05 M H2SO4) solution with TlIII in the presence of ethanol to produce a highly fluorescent oxidized product, followed by the direct measurement of the fluorescence intensity in an aqueous solution at room temperature. The reagent blank did not show any significant fluorescence intensity. All the reactions involved in the procedure were fast and no extraction or separation was required. With suitable masking, the reaction can be made highly selective.
Experimental section
Apparatus
A Shimadzu (Kyoto, Japan) (Model-RF-5301PC) spectrofluorophotometer with 1 cm quartz cells were used and a Jenway (England, UK) (Model-3010) pH meter with a combination of electrodes were used for measuring the fluorescence intensity and pH, respectively. The calibration and linearity of the instrument were frequently checked with standard quinine sulphate (10 mg L−1). A Shimadzu (Kyoto, Japan) (Model-9800) Inductively Coupled Plasma-Optical Emission Spectrometer (ICP-OES) [λ = 418 nm, plasma gas flow rate (L min−1) = 15, LOD: 1 μg L−1 of Tl, RF power (W) = 1400, nebulizer gas flow rate (L min−1) = 1–10], a Shimadzu (Kyoto, Japan) (Model AA7000) atomic absorption spectrophotometer equipped with a microcomputer-controlled air-acetylene flame and an Inductively Coupled Plasma-Mass Spectrometer (ICP-MS) (Agilent, 7700x, California, USA) at the Analytical Division of BCSIR Laboratories, Dhaka, were used to compare the results. The elemental analyzer (Exeter Analytical Inc. Model CE 440) equipped with a supersensitive thermal conductivity detector for the simultaneous determination of CHN and a Refurbished Shimadzu QP-2010S Mass Spectrometer were used for the characterization of the ligand. The infrared spectra were recorded with an FTIR Spectrophotometer, Shimadzu (Kyoto, Japan) (Model-IR Prestige 21, Detector DTGS KBr) in the range of 7500–350 cm−1 and model: JEOL 500SS four channel NMR spectrometer with signal-to-noise ratio of 5000
:
1 for proton, magnetic field strength = 500 MHz, DMSO D6 solvent, and TMS standard, were used for the characterization of the ligand.
Reagents and solutions
Analytical grade reagents were used throughout. High-purity absolute ethanol and high-purity de-ionized water were used throughout. N-(Pyridin-2-yl)-quinoline-2-carbothioamide (PQCTA, C15H11N3S) (molecular wt = 265.28) was synthesized according to the method of Ahmed et al.46 The reagent (PQCTA) was characterized according to the method of Ahmed et al.48 by the melting point analysis, elemental analysis, FTIR and 1H NMR spectroscopy, and thermo gravimetric analysis. The reagent solution was prepared by dissolving the requisite amount (0.0026 g) of PQCTA in a known volume (10 mL) of absolute ethanol. A freshly prepared reagent solution (3.77 × 10−3 M) was used whenever required. 100 mL stock solution (1 mg mL−1) of trivalent thallium was prepared by dissolving 217.5 mg thallium(III) nitrate trihydrate, Tl(NO3)3·3H2O (Sigma-Aldrich, www.sigmaaldrich.com; purity 99.5%) in doubly distilled de-ionized water, and subsequently standardized by titrimetric analysis with EDTA.50 More dilute standard solutions were prepared by the appropriate dilution of aliquots from the stock solution with de-ionized water as and when required. A freshly standardized solution was always used. 100 mL stock solution (1 mg mL−1) of monovalent thallium was prepared by dissolving 130.3 mg anhydrous thallium(I) nitrate, TlNO3 (Sigma-Aldrich pro-analysis grade; www.sigmaaldrich.com; purity 99.99%) in doubly distilled de-ionized water. 100 mL amount of saturated bromine water solution (3% w/v) was freshly prepared by dissolving 4.79 g NaBr (ACS grade, 99% pure) in 100 mL deionized water. Solutions of a large number of inorganic ions and complexing agents were prepared from their AnalaR grade or equivalent grade water-soluble salts (or the oxides and carbonates in hydrochloric acid); those of niobium, tantalum, titanium, zirconium, and hafnium were specially prepared from their corresponding oxides (Specpure, Johnson Matthey) according to the recommended procedures of Mukharjee.51 In the case of insoluble substances, special dissolution methods were adopted.52
The elemental analysis and mass spectroscopy were performed at the National Center of Excellence in Analytical Chemistry, University of Sindh, Pakistan and the FTIR spectra were recorded with an FTIR spectrophotometer, Shimadzu (Kyoto, Japan) (Model-IR Prestige 21, Detector DTGS KBr) in the range of 7500–350 cm−1 from our laboratory and the 1H NMR spectrum was recorded using a Model JEOL 500SS spectrometer, Kanazawa, Japan. Both the FTIR and 1H NMR spectra are shown in Fig. 1 and 2 (ESI = Electronic Supplementary Information†).
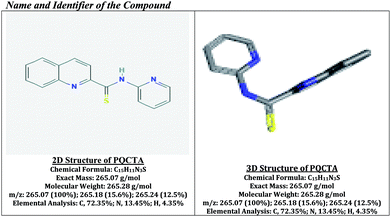 |
| Fig. 1 Name and identifier and the detailed characterizations of N-(pyridin-2-yl)-quinoline-2-carbothioamide (PQCTA). | |
Procedure
To 0.1–1.0 mL of a neutral aqueous solution containing 0.01–6000 ng of thallium(III) in a 10 mL calibrated flask was mixed with a 1
:
93–1
:
155-fold molar excess (preferably 1.5 mL) of 3.77 × 10−3 M of the N-(pyridin-2-yl)-quinoline-2-carbothioamide (PQCTA) reagent solution, followed by the addition of 0.5–2 mL (preferably 1 mL) of 0.05 M of sulfuric acid. The solution was mixed well and allowed to stand for 5 min, after which 2 mL of absolute ethanol was added and the mixture was diluted to the mark with de-ionized water. The fluorescence intensity of the system was measured at 379 nm against a corresponding reagent blank, prepared concurrently, keeping the excitation wavelength maximum at 324 nm and the instrument setting the same. The determination of thallium(I) is based on the rapid oxidation of this ion by bromine water heating for 15 min with the concomitant formation of fluorescent Tl(III)-PQCTA. The fluorescence due to the sum of TlI and TlIII is measured and TlI is determined from the difference in the fluorescence values. The thallium content in an unknown sample was determined using a concurrently prepared calibration graph.
Sample collection and preservation
Environmental samples. Water and soil samples were collected in polythene bottles from different places of Bangladesh. After collection, HNO3 (1 mL L−1) was added as the preservative.
Blood, urine, and milk. Blood and urine samples were collected in polythene bottles from affected persons of Chittagong Medical College Hospital, Bangladesh. The milk sample was collected from a Bangladeshi lactating mother. Immediately after collection, the samples were stored in a salt–ice mixture and later at the laboratory at −20 °C.
Soil samples. Soil samples were collected from different locations of Bangladesh. The samples were dried in air and homogenized with a mortar.
Food samples. Food samples (rice, wheat, fruits, and vegetables) were collected from the local market of Chittagong. After collection, the samples (fruits and vegetables) were stored in a refrigerator for preservation. The samples (rice, wheat) were used in the dry condition and homogenized with a mortar.
Results and discussion
Factors affecting the fluorescence intensity
Excitation and emission spectra. Tl(III) fluoresces strongly in PQCTA solution when irradiated with ultraviolet light. The excitation (λex) and emission (λem) spectra of the fluorescent Tl(III)-PQCTA in 0.05 M sulfuric acid medium was recorded using a spectrofluorometer. The excitation and emission maxima were 324 nm and 379 nm, respectively. The reagent blank exhibited negligible fluorescence despite having wavelength maximum in the same region. In all the instances, measurements were made against the reagent blank. The spectra are shown in Fig. 2. It was the relative fluorescence intensity that was determined at different excitation wavelengths at a fixed emission wavelength,53 which was the excitation spectra (λex = 324 nm). Then, we fixed the excitation wavelength and scanned the emission wavelength, which was the emission spectra (λem = 379 nm). Thus, for every case, the condition of measurement should be identical for both the sample and the reagent blanks.
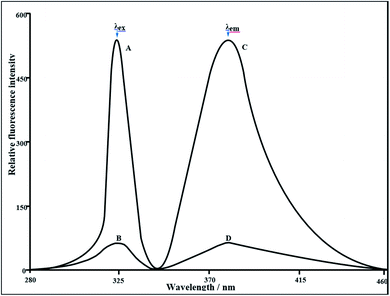 |
| Fig. 2 Spectra (A) and (B) are the excitation spectra of the TlIII-PQCTA system and the reagent blank (λex = 324 nm), respectively; (C) and (D) are the corresponding emission spectra (λem = 379 nm) in aqueous solutions. Band width: ex, Slit-1.5, em. Slit-1.5, sensitivity: high. | |
Optimization of some parameters on the fluorescence intensity
Effect of the solvent. Because PTQA is insoluble in water, an organic solvent was used for the system. Various solvents [chloroform, benzene, carbon tetrachloride, n-butanol, isobutanol, ethanol, 1,4-dioxane, and N-dimethylformamide (DMF)] were tested; ethanol was found to be the best solvent for the system. The effect of ethanol on the fluorescence intensity was studied and no adverse effect was observed over a wide range (20–80%) of ethanol concentrations (final volume). It was observed that the TlIII-PQCTA system with 10 μg L−1 TlIII in absolute ethanol solution produced constant fluorescence intensity at a constant ethanol volume range, as shown in Fig. 3. A concentration of 20% v/v ethanol in the final volume was sufficient to prevent any precipitation or turbidity and to allow accurate measurements. Therefore, 20% v/v ethanolic solution was used in the recommended procedure.
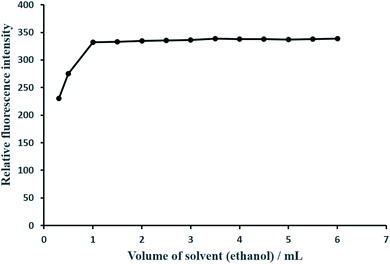 |
| Fig. 3 Effect of volume of ethanol on the fluorescence of the TlIII-PQCTA system. | |
Effect of acidity. The oxidation reaction was conducted in acid medium to avoid the formation of a precipitate of thallium. In order to determine the most suitable acid for the reaction, different acids (nitric, sulfuric, hydrochloric, and phosphoric) were studied. However, sulfuric acid was found to be the best acid compared to any other mineral acids for the system. The fluorescence intensity was the maximum and constant when 10 mL of the solution (10 μg L−1 of TlIII) contained 0.5–2 mL of 0.05 M sulfuric acid at room temperature (25 ± 5 °C). Outside this range of acidity, the fluorescence intensity decreased (Fig. 4). The optimum acidity range in the final solution is therefore 0.0025–0.05 M (preferably 0.05 M) H2SO4. Therefore, 1 mL 0.05 M sulfuric acid solution was used for all subsequent measurements.
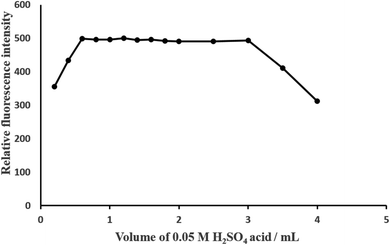 |
| Fig. 4 Effect of volume of H2SO4 on the fluorescence of the TlIII-PQCTA system. | |
Effect of temperature. The influence of temperature was studied between 10–80 °C. It could be observed from Fig. 5 that the temperature effect is not pronounced between 10–80 °C; thus, room temperature (25 ± 5) °C is recommended for all subsequent measurements.
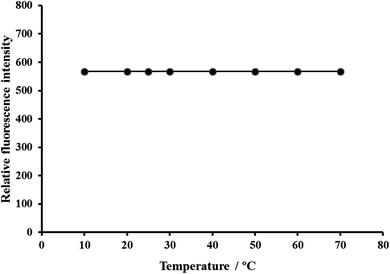 |
| Fig. 5 Effect of temperature on the fluorescence of the TlIII-PQCTA system. | |
Effect of time. The reaction is instantaneous. The TlIII-PQCTA system attained maximum and constant fluorescence intensity immediately (within 5 min) after dilution of the solution to the final volume, which then remained strictly unaltered for 24 h at room temperature (25 ± 5) °C, as shown in the ESI Fig. S5.†
Effect of reagent concentration. The intensities of fluorescence of a series of solutions containing a constant amount of Tl(III) with varying amounts of PQCTA were measured in order to establish the optimum concentration of PQCTA. The change in the fluorescence intensity with PQCTA concentration is shown in Fig. 6, while the concentration of Tl(III) was kept constant. It was found that the fluorescence intensity increased at first as the PQCTA concentration rose and reached a maximum but the further addition of PQCTA hardly affected the intensity even though PQCTA is more concentrated than Tl(III). It was observed that 10 μg L−1 TlIII metal and reagent molar ratios of 1
:
93–1
:
155 produced a constant fluorescence intensity of the oxidized product. Outside this range of reagents, the fluorescence intensity decreased (Fig. 6). At different TlIII concentrations (0.5 and 1 μg L−1), the effect of varying the reagent concentration was similar. For all subsequent measurements, 1.5 mL of 3.77 × 10−3 M PTQA reagent was added.
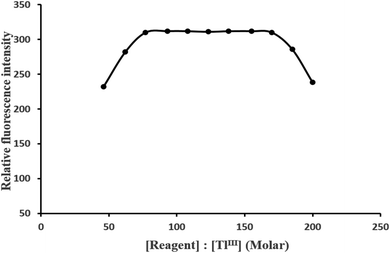 |
| Fig. 6 Effect of the reagent (PQCTA : TlIII molar concentration, i.e., molar ratio of PQCTA/TlIII) on the fluorescence of the TlIII-PQCTA system. | |
Effect of metal concentration. The effect of the Tl(III) solution was investigated by carrying out the reaction using 1 mL of Tl(III) solution of different concentrations ranging from 0.001–1000 μg L−1. It was found that the relative fluorescence intensity increased by increasing the concentration of Tl(III) up to 600 μg L−1 but a higher concentration has a negative effect on the values of the relative fluorescence intensity.
Calibration curves (Beer's law)
The calibration graphs for the determination of Tl(III) were constructed under optimum conditions. The well-known equation for spectrofluorimetric analysis in very dilute solutions was derived from Beer's law. The effect of metal concentration was studied over 0.001–1000 μg L−1 distributed in six different sets (0.001–0.01, 0.01–0.1, 0.1–1, 1–10, 10–100, and 100–1000 μg L−1) for convenience of measurement. The fluorescence intensity was linear over a wide range (0.001 μg L−1 to 600 μg L−1) for 0.001–600 μg L−1 of thallium(III) at an excitation wavelength of 324 nm and an emission wavelength of 379 nm. Of the six calibration graphs, one shows the limit of the linearity range, shown in Fig. 7; the remaining five are straight-line graphs passing through the origin (R2 = 0.9996), which are shown in the ESI (Fig. S6, S7, S9, S10 and S11 ESI†). The limit of detection (LOD) and limit of quantization (LOQ) were found to be 0.00016 μg L−1 and 0.001.6 μg L−1, respectively. LOD59 = 3s/S and LOQ = LOD × 10, where s is the standard deviation of the blank and S is the slope of the calibration graph. The selected analytical parameters obtained with the optimization experiments are summarized in Table 2.
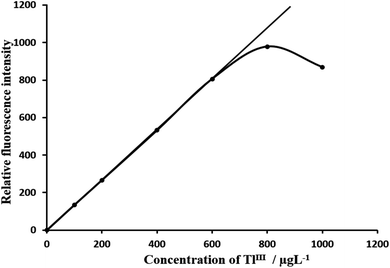 |
| Fig. 7 Calibration graph F: 100–600 μg L−1 of thalliumIII. Bandwidth: ex. slit-3, em. slit-3. Sensitivity: high. | |
Table 2 Selected analytical parameters for the optimization experiments
Parameters |
Studied range |
Selected value |
Excitation wavelength maximum/λex/nm |
200–700 |
324 |
Emission wavelength maximum/λem/nm |
200–700 |
379 |
Solvent/amount of absolute ethanol/mL |
0–8 |
2–8 (preferably 2) |
Acidity/M H2SO4 |
0.0001–0.5 |
0.0025–0.05 (preferably 0.05) |
pH |
1.0–5.0 |
1.3–3.8 (preferably 1.5) |
Time/h |
0–72 |
1 min–24 h (preferably 2 min) |
Temperature/°C |
10–90 |
15–40 (preferably 25 ± 5) |
Reagent (fold molar excess, R : M) |
1 : 1–1 : 500 |
1 : 93–1 : 155 (preferably 1 : 115) |
Linear range/μg L−1 |
0.0001–1000 |
0.001–600 |
Detection limit (LOD)/ng L−1 |
0.001–10.0 |
0.16 |
Limit of quantification/ng L−1 |
0.01–100 |
1.6 |
Reproducibility/RSD% |
0–10 |
0–2 |
Regression coefficient (R2) |
0.9992–0.9999 |
0.9996 |
Effect of foreign ions
Several ions and complexing agents were considered independently to examine their effect on the quantification of 1 μg L−1 of thallium. A fixed criterion54 was set for the interference study, which was ±5% deviation from the anticipated result of only Tl. The results are summarized in Table S2 (ESI†). The formula for calculating the tolerance ratio is
here, X is the fluorescence signal for 1 μg L−1 Tl with varying amounts of species (x). Y is the fluorescence signal of the 1 μg L−1 standard Tl. Briefly, 1 μg L−1 standard Tl was mixed with varying amounts of potentially interfering species, and the tolerance limit was calculated from the above formula. If the value was found outside the range (95–105) %, which is a ±5% deviation from the standard Tl signal, then that amount of the species was taken as the interfering with the signal. Thus, the ratio of that weight of the interfering species and 1 μg of Tl is termed as its tolerance ratio. More than 80 anions, cations, and complexing agents were studied individually to investigate their effect on the determination of 1 μg L−1 of thallium. Most of the ions were tolerated over 1000 times. Very little interference was observed for a large number of ions. The most serious interference was from Se(IV), Cr(VI), and Mn(VII) ions. Masking agents allowed us to get a higher tolerance limit for the interfering ions. In order to eliminate the interference of Se(IV), Cr(VI), and Mn(VII) ions, EDTA and tartrate can be used as masking agents, respectively.55 A 100-fold excess of Se(IV), Cr(VI), and Mn(VII) ions could be masked with EDTA and tartrate, respectively. These three ions were masked because Se(IV), Cr(VI), and Mn(VII) would probably form complexes with EDTA and tartrate, respectively. Centrifugation and filtration were employed in the case of any precipitation occurring during the masking process. Strong reducing agents such as tin(II), chloride, iron(II), sulfate, hydroxylamine, hydrochloride, and sodium azide, which would otherwise reduce thallium(III), undergo oxidation during the treatment of the thallium(III) solution with bromine water and hence are not a problem.56 The amount mentioned is the actual amount studied instead of indicating the tolerance limit.
Nature of the fluorescing species
The reagent PQCTA produces the same spectral characteristics with strictly the same wavelength maxima of excitation (324 nm) and emission (379 nm) when separately treated with strong oxidants, namely, thallium(III), manganese(VII), chromium(VI), cerium(IV), and with persulphate and hydrogen peroxide in slightly acidic media. However, the only difference is in the magnitude of the fluorescence intensity so that the fluorescing species is thought as being the oxidation product. Similar oxidized fluorescent products were reported earlier with other reagents for several metal ions of variable valence states.57,58
In the present case, the substrates have many potential reaction sites so that the structure of the oxidized fluorescent species is difficult to predict in the absence of further data. However, it is assumed that oxidative ring closure takes place, leading to the formation of structure A in resonance with structure B. As both structures have increased rigidity, energy dissipation by radiation-less transitions is minimized so that more energy is available for fluorescence59 (Fig. 8).
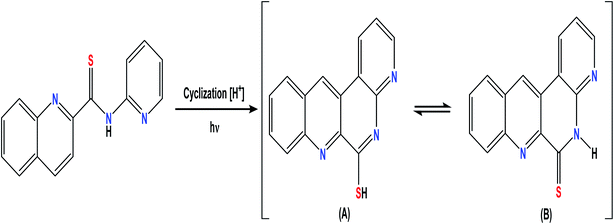 |
| Fig. 8 Mechanism of oxidative reaction of N-(pyridin-2-yl)-quinoline-2-carbothioamide (PQCTA). | |
Precision and accuracy
The precisions of the present method were proved by measuring 10 solutions of the same sample (each analyzed at least five times). The relative standard deviation (n = 5) was 0–2% for 0.01–6000 ng of thallium(III) in 10 mL, indicating that this method is highly precise and reproducible (Table 2). The detection limit (3s/S of the blank) and the limit of quantization (10 times of the detection limit) for thallium(III) were found to be 0.16 ng L−1 and 1.6 ng L−1, respectively. The method was also tested by analyzing several synthetic mixtures containing thallium(III) and diverse ions (Table 3). The results for total thallium were in excellent agreement with the certified values (Table 4). The reliability of the procedure was tested by recovery studies. The average percentage recovery obtained for the addition of thallium(III) spike to some environmental water samples was quantitative, as shown in Table 5. The results of biological analyses by the spectrofluorimetric method were in excellent agreement with those obtained by ICP-OES (Table 6). The results of soil analyses by the spectrofluorimetric method were in excellent agreement with those obtained by AAS (Table 7). The results of food and vegetable analyses by the spectrofluorimetric method were also found to be in excellent agreement with those obtained by ICP-OES (Table 8). The results of the speciation of thallium(I) and thallium(III) in the mixtures were highly reproducible (Table 9). The results of speciation analyses by the spectrofluorimetric method were also found to be in excellent agreement with those obtained by ICP-MS (Table 10). Hence, the precision and accuracy of the method were found to be excellent.
Table 3 Determination of thallium in some synthetic mixtures
Sample |
Composition of mixtures (μg L−1) |
Thallium/μg L−1 |
Added |
Founda (n = 5) |
Recovery ± SDb (%) |
Average of five analyses of each sample. The measure of precision is the standard deviation (SD). |
A |
Tl(III) |
1.0 |
0.99 |
99.0 ± 0.6 |
50.0 |
49.5 |
99.0 ± 1.0 |
B |
As in A + Cr+3(50) + As3+(50) + Co2+(50) + Fe3+(50) + Ag(50) |
1.0 |
99.5 |
99.6 ± 1.0 |
50.0 |
50.1 |
100 ± 0.5 |
C |
As in B + Pb2+(50) + Bi3+(50) + SeIV(50) + Mg(50) + Ba(50) +Tl(50) |
1.0 |
0.98 |
98.0 ± 1.0 |
50.0 |
49.8 |
99.0 ± 1.5 |
D |
As in C + Sn2+(50) + CrVI(50) + TiIV(50) + Mg(50) + Fe+2(50) |
1.0 |
1.01 |
101.0 ± 0.8 |
50.0 |
49.9 |
99.9 ± 1.3 |
E |
As in D + Ca(50) + Al(50) + WVI(50) + AsV(50) + Ni+2(50) + Cd (50) |
1.0 |
0.99 |
99.0 ± 1.5 |
50.0 |
49.69 |
99.5 ± 1.8 |
F |
As in E + Na(50) + Li(50) + K(50) + Zn(50) + CeIV(50) |
1.0 |
0.98 |
98.0 ± 1.6 |
50.0 |
49.95 |
99.9 ± 1.8 |
Application of the proposed method
The proposed method was applied to the determination of total thallium in several certified reference materials with various composition, as shown in Table 4. To verify the validity, the proposed method was successfully applied to the determination of total thallium in a number of environmental, biological, soil, vegetable, and food samples. The method was also successfully used for the simultaneous determination of TlI and TlIII in mixtures with known TlI and TlIII contents. Furthermore, the method was extended to the simultaneous determination of TlI and TlIII in environmental waters in spiked samples. The results of the speciation analyses by the spectrofluorimetric method were also found to be in excellent agreement with those obtained by ICP-MS (Table 10).
Table 4 Determination of thallium in some certified reference materials
Sample no. |
Certified reference materials composition (mg kg−1) |
Thallium/mg kg−1 |
In CRM sample |
Found (n = 5)a |
RSDb(%) |
Average of five analysis of each samples. The measure of precision is the relative standard deviation (RSD). Values in μg L−1. These CRMs were obtained from European Reference Materials, Institute of Reference Materials & Measurements, Joint Research Centre, European Commission, Brussels, Belgium. This CRM was obtained from High Purity Standards (HPS), Amazon, North Charleston, USA. These CRMs were obtained from the National Research Council, Govt of Canada. |
1 |
ERM®-EB 325: in four grades of unalloyed zincd |
5.28 |
5.21 ± 0.35 |
1.25 |
BCR-322R: unalloyed zinc; Cd(15.08), Cu(5.89), Fe(19.1), Pb(15.0), Sn(5.6), Tl(5.28) |
2 |
BCR-323R: unalloyed zinc; Cd(6.51), Cu(18.9), Fe(11.3), Pb(48.6), Sn(18.7), Tl(10.8) |
10.8 |
10.7 ± 0.5 |
1.5 |
3 |
BCR-324R: unalloyed zinc; Cd(48.6), Cu(9.87), Fe(58.5), Pb(26.1), Sn(9.8), Tl(19.9) |
19.9 |
19.8 ± 0.8 |
1.8 |
4 |
BCR-325R: unalloyed zinc; Cd(94.7), Cu(47.5), Fe(56.1), Pb(142.0), Sn(46.1), Tl (36.8) |
36.8 |
36.7 ± 1.2 |
2.0 |
5 |
CRM-TMDW-A-100: water standards: (Sb = 55, As = 55, Be = 15, Cd = 10, Se = 11, Ag = 2, Tl = 10, Mn = 40, Ni = 60, Zn = 75)e |
10.00c |
9.95 ± 0.55 |
1.65 |
6 |
NIST®-SRM®-1643b: river waterf |
8.0c |
7.8 ± 1.0 |
1.8 |
7 |
NIST®SRM®-1577b: bovine liverf |
3.0c |
2.9b ± 0.1 |
1.5 |
8 |
NIST®-SRM®-2711: soilf |
0.61 ± 0.02 |
0.59 ± 0.07 |
1.67 |
9 |
NIST®-SRM®-2711a: estuarine sedimentsf |
2.19 ± 0.9 |
2.17 ± 0.5 |
1.85 |
10 |
NIST®-SRM®-2670: human urine (normal)f |
10.0c |
9.9 ± 0.8 |
2.0 |
Determination of thallium synthetic mixtures. The procedure was applied to determine trace amounts of thallium(III) in some synthetic mixtures and good recovery was achieved. The result indicates that the proposed method is suitable and can be successfully applied for the determination of thallium(III). Several synthetic mixtures of varying compositions containing thallium(III) and diverse ions of known concentrations were determined by the present method using EDTA as the masking agent. The results were found to be highly reproducible, as shown in Table 3. Accurate recoveries were achieved in all the solutions in the range from 99.6 ± 1.5 to 99.9 ± 0.6. The reliability of our thallium-PQCTA oxidation procedure was approved by the quantitative recovery of thallium(III) spiked in several synthetic mixtures containing thallium(III) and diverse ions. This method has high precision and accuracy (s = ±0.01 for 0.5 μg L−1).
Determination of thallium in some certified reference materials. A 0.1 g amount of an alloy or steel sample containing 0.15–4.95% of thallium was weighed accurately and placed in a 50 mL Erlenmeyer flask in the presence of excess bromine water and HNO3 to oxidize thallium(I) to thallium(III), following the method recommended by Mitra.60 To it, 10 mL of 20% (w/v) sulfuric acid was added while carefully covering with a watch glass until the brisk reaction subsided. The solution was heated and simmered gently after the addition of 10 mL of concentrated HNO3 until all the residual carbides were decomposed. Then, a further 2 mL of 1 + 1H2SO4 and 5 mL 3% (w/v) freshly prepared bromine water was added and the solution was evaporated carefully at that point by warming to a vapor, then cooled to the room temperature (25 ± 5) °C. After suitable dilution with de-ionized water, the contents of the Erlenmeyer flask were warmed so as to dissolve the soluble salts. The solution was then cooled and neutralized with dilute NH4OH solution in the presence of 1–2 mL of 0.01% (w/v) EDTA solution. The resulting solution was filtered, if necessary, through a Whatman no. 40 filter paper into a 100 mL calibrated flask. The residue (silica and tungstic acid) was washed with a small volume of hot 1 + 99H2SO4, followed by water; the volume was made up to the mark with de-ionized water.A suitable aliquot (1–2 mL) of the above-mentioned solution was taken into a 10 mL calibrated flask and the thallium(III) content was determined as described under the procedure using tartrate or EDTA as the masking agent. The proposed procedure for the spectrofluorimetric determination of thallium was applied to the analysis of Soil (NIST®-SRM®-2711), Estuarine Sediments (NIST®SRM®-711a), River water (NIST® SRM®-1643b), Human urine (NIST®SRM®-2670), and Bovine liver (NIST® SRM®-1577c). These CRMs were obtained from the National Research Council, Govt. of Canada, using tartrate or EDTA as the masking agents, following a method recommended by Sun et al.61 Based on five replicate analyses, the average thallium concentration determined by the spectrofluorimetric method was in an excellent agreement with the certified values. The results are given in Table 5.
Table 5 Determination of thallium in some environmental water samples
Sample |
Thallium/μg L−1 |
Recovery ± s (%) |
srb (%) |
Added |
Founda (n = 5) |
Average of five replicate determinations of each sample. The measure precision is the relative standard deviation (sr). PHP Glass Factory, Kumira, Chittagong. Bangladesh Steel Re-rolling Mills Ltd (BSRM), Baizid Bosthami, Chittagong. Eastern Refinary, Patenga, Chittagong. Cement Industry, Patenga, Chittagong. Berger Paints Bangladesh Limited, Kalurghat, Chittagong. |
Tap water |
0.0 |
2.0 |
98 ± 0.6 |
0.3 |
10.0 |
11.8 |
100.2 ± 0.5 |
0.2 |
50.0 |
52.1 |
|
|
Well water |
0.0 |
5.0 |
103 ± 0.7 |
0.3 |
10.0 |
15.5 |
99 ± 0.5 |
0.3 |
50.0 |
49.5 |
|
|
Rain water |
0.0 |
1.0 |
100.9 ± 0.8 |
0.4 |
10.0 |
11.1 |
99.6 ± 0.8 |
0.4 |
50.0 |
50.8 |
|
|
River water |
Karnaphully (upper) |
0.0 |
12.0 |
99.5 ± 0.5 |
0.3 |
10.0 |
21.9 |
100.5 ± 0.8 |
0.4 |
50.0 |
62.3 |
|
|
Karnaphully (lower) |
0.0 |
15.0 |
100.4 ± 0.9 |
0.3 |
10.0 |
25.1 |
102.3 ± 1.0 |
0.4 |
50.0 |
66.5 |
|
|
Halda (upper) |
0.0 |
11.0 |
100.9 ± 1.2 |
0.5 |
10.0 |
22.2 |
102.3 ± 1.0 |
0.4 |
50.0 |
61.0 |
|
|
Halda (lower) |
0.0 |
13.0 |
100.4 ± 0.8 |
0.3 |
10.0 |
23.1 |
102.3 ± 1.0 |
0.3 |
50.0 |
64.0 |
|
|
Sea water |
Bay of Bengal (upper) |
0.0 |
8.0 |
100.5 ± 1.3 |
0.4 |
10.0 |
18.1 |
101.1 ± 1.0 |
0.5 |
50.0 |
59.0 |
|
|
Bay of Bengal (lower) |
0.0 |
10.0 |
100.9 ± 1.0 |
0.4 |
10.0 |
21.2 |
100.8 ± 0.8 |
0.3 |
50.0 |
60.5 |
|
|
Drain water |
PHP glassc |
0.0 |
30.0 |
102.5 ± 1.0 |
0.5 |
10.0 |
41.0 |
100.6 ± 0.5 |
0.2 |
50.0 |
80.5 |
|
|
BSRMd |
0.0 |
55.0 |
100.7 ± 1.0 |
0.5 |
10.0 |
65.5 |
100.3 ± 0.5 |
0.4 |
50.0 |
105.5 |
|
|
Eastern cablee |
0.0 |
65.0 |
100.6.±1.5 |
0.8 |
10.0 |
75.5 |
102.1 ± 1.2 |
0.6 |
50.0 |
117.5 |
|
|
Cement Industryf |
0.0 |
95.0 |
101.3 ± 1.0 |
0.4 |
10.0 |
107.0 |
100.8 ± 0.8 |
0.3 |
50.0 |
145.8 |
|
|
Berger Paintsg |
0.0 |
75.0 |
100.6 ± 0.0 |
0.3 |
10.0 |
85.5 |
102.4 ± 1.0 |
0.5 |
50.0 |
128.0 |
|
|
Determination of thallium in environmental water samples. In each filtered (with Whatman no. 40) environmental sample (25 mL) contained in a 50 mL Pyrex beaker, 1 mL of concentrated H2SO4 and 5 mL of concentrated HNO3 were added in the presence of freshly prepared excess bromine water solution in a fume cupboard to oxidize thallium(I) to thallium(III), and the mixture was heated on a hot plate until white fumes were obtained, following the method recommended by Greenberg et al.62 The solution was cooled and neutralized with dilute NH4OH solution in the presence of 1–2 mL of 0.01% (w/v) EDTA solution. The resulting solution was then filtered through a Whatman no. 40 filter paper and quantitatively transferred to a 25 mL calibrated flask and made up to the mark with de-ionized water.An aliquot (1–2 mL) of this water sample was pipetted into a 10 mL calibrated flask and the thallium content was determined as described under the general procedure using tartrate or EDTA as the masking agent. To test the validity of our method, we analyzed different types of Potable and polluted waters in spiked and unspiked conditions. The reliability of our spectrofluorimetric method was tested by recovery studies. The average percentage recovery obtained for the addition of a thallium(III) spike to some environmental water samples was quantitative. The results of the analyses of environmental water samples from various sources for thallium are shown in Table 5.
Most spectrofluorimetric methods for the determination for speciation of thallium in natural water and sea-water require preconcentration or the standard addition of thallium.63 The content of thallium in river waters in Poland ranged from 5 to 17 ng L−1. Sea waters usually contain 10–15 ng L−1 of thallium.64,65
Determination of thallium in biological samples. Human blood, milk (2–3 mL), urine (10–20 mL), or hair (3–5 g) sample was taken into a 100 mL micro-Kjeldahl flask. A glass bead and 10 mL of concentrated nitric acid were added, and the flask was placed on a digester under gentle heating. The sample was digested in the presence of an excess of freshly prepared bromine water solution (5 mL of 3% w/v) to oxidize thallium(I) to thallium(III), according to the method recommended by Stahr.66 As the heating process continued, 1 mL of H2SO4 was added and heated for about 0.5 hour to dense white fumes. When the initial brisk reaction was completed, the solution was removed and cooled at room temperature and neutralized with dilute NH4OH solution in the presence of 1–2 mL of 0.01% (w/v) EDTA solution. The resulting solution was then filtered through a Whatman no. 40 filter paper, quantitatively transferred into a 25 mL calibrated flask, and made up to the mark with de-ionized water.A suitable aliquot (1–2 mL) of the final solution was pipetted out into a 10 mL calibrated flask and the thallium content was determined as described under the general procedure using EDTA or tartrate as the masking agent. The results of the biological analyses by the spectrofluorimetric method were found to be in excellent agreement with those obtained by ICP-OES. The results are shown in Table 6.
Table 6 Determination of thallium in some human fluids and hair samples
Serial no. |
Sample sourcea |
Sample |
Thallium/μg L−1 |
ICP-OES (n = 5) |
Proposed method (n = 5) |
Found |
RSDb (%) |
Found |
RSDb (%) |
Samples were collected from Chittagong Medical College Hospital, Bangladesh. The measure of precision is the relative standard deviation (RSD). Values in μg kg−1. |
1 |
Hypertension patient (female) |
Blood |
10.0 |
1.5 |
10.5 |
1.6 |
Urine |
2.6 |
1.2 |
2.5 |
1.0 |
2 |
Liver cirrhosis patient (male) |
Blood |
25.0 |
1.8 |
25.8 |
2.0 |
Urine |
7.5 |
1.5 |
8.0 |
1.5 |
3 |
Lung cancer patient (male) |
Blood |
35.5 |
2.0 |
36.2 |
2.0 |
Urine |
8.5 |
1.5 |
9.3 |
1.7 |
4 |
Kidney damage patient (female) |
Blood |
45.0 |
2.5 |
45.5 |
2.5 |
Urine |
11.0 |
1.6 |
12.0 |
1.8 |
5 |
Skin disease patient (female) |
Blood |
27.0 |
2.0 |
28.0 |
2.0 |
Urine |
7.0 |
1.3 |
7.5 |
1.5 |
6 |
Diarrhea (male) |
Blood |
24.5 |
2.0 |
25.0 |
2.1 |
Urine |
6.8 |
1.5 |
7.0 |
1.5 |
7 |
Hair loss patient (female) |
Blood |
32.5 |
2.5 |
32.8 |
2.3 |
Urine |
8.8 |
1.8 |
9.0 |
1.8 |
8 |
Neurological disorder(Male) |
Blood |
21.8 |
2.0 |
22.0 |
2.0 |
Urine |
5.5 |
1.6 |
5.8 |
1.8 |
9 |
Normal adult non-smoker (female) |
Blood |
2.0 |
1.0 |
2.1 |
1.0 |
Urine |
0.55 |
0.8 |
0.60 |
1.0 |
10 |
Lactating mother |
Human milk |
6.0 |
1.5 |
6.5 |
1.8 |
11 |
Normal nonsmoker (female) |
Human hair |
8.0c |
1.6 |
8.5 |
1.8 |
The occurrence of such high thallium contents is reported in lung cancer,67 kidney damage, and hair loss (alopecia)68,69 from some developed countries.70
Determination of thallium in some surface soil samples. An air-dried homogenized soil sample (10 g) was accurately weighed and placed in a 100 mL micro-Kjeldahl flask. The sample was digested in the presence of an excess of oxidizing agent (5 mL of 3% (w/v) freshly prepared bromine water solution) to oxidize thallium(I) to thallium(III), following the method recommended by Jackson.71 As the heating process continued, 1 mL of H2SO4 was added and heated for about 5 min to dense white fumes. The solution was then cooled to room temperature and neutralized with dilute NH4OH solution in the presence of 1–2 mL of 0.01% (w/v) EDTA solution. The content of the flask was then filtered through a Whatman no. 40 filter paper and quantitatively transferred into a 25 mL calibrated flask and made up to the mark with de-ionized water.A suitable aliquot (1–2 mL) of the final solution was pipetted out into a 10 mL calibrated flask and the thallium content was determined as described under the general procedure using tartrate or EDTA as the masking agent. The thallium content was then determined by the above procedure and quantified from a calibration graph prepared concurrently. The results of soil analyses by the spectrofluorimetric method were also found to be in excellent agreement with those obtained by AAS. The results are shown in Table 7.
Table 7 Determination of thallium in some soil samples
Serial no. |
Sample sourcec |
Thallium/mg kg−1 (n = 5) |
AAS (n = 5) |
Proposed method (n = 5) |
Founda |
RSDb (%) |
Found |
RSDb (%) |
Average of five replicate analyses of each sample. The measure of precision is the relative standard deviation (RSD). Composition of the soil samples: C, N, P, K, Na, Ca, Mg, Fe, Pb, Cu, Zn, Mn, Tl, Co, NO3, NO2, SO4, etc. |
1 |
Chemistry dept. Soil (Chittagong University) |
1.2 |
1.0 |
1.5 |
0.8 |
2 |
Cement industrial soil, Potenga, Chittagong |
85.5 |
2.0 |
86.8 |
2.0 |
3 |
Steel industrial soil, (BSRM, Chittagong, Bangladesh) |
55.0 |
2.0 |
56.0 |
1.8 |
4 |
Road side (dhaka-Chittagong) |
26.0 |
1.8 |
27.5 |
2.0 |
5 |
Paint industrial soil, (Berger Paints) |
35.5 |
2.5 |
36.0 |
2.5 |
6 |
Industrial soil, (Eastern Cables Ltd) |
31.0 |
2.0 |
32.5 |
2.2 |
7 |
Fertilizer industrial soil, (T.S.P. Complex Ltd, Chittagong) |
15.0 |
1.8 |
15.8 |
1.9 |
8 |
Madina tannery soil, Jalabad, Chittagong |
22.0 |
2.0 |
21.8 |
2.0 |
9 |
Estuarine soil (Karnafuli river, Chittagong) |
45.0 |
2.5 |
45.8 |
2.5 |
10 |
Glass industrial soil, (Usmania glass) |
18.8 |
2.0 |
19.5 |
2.0 |
Determination of thallium in some vegetable, food, and fruit samples. The vegetable and fruit samples collected prior to the determination were pretreated in the following way: the edible portions of samples were first washed with tap water followed and rewashed with de-ionized water. After removing de-ionized water from the surface of the vegetables and fruits, the samples were cut into small pieces and dried at 65 °C in an oven. The air-dried vegetables and fruits samples (10 g) were ground in a mortar and taken in a 100 mL micro-Kjeldahl flask in the presence of excess oxidizing agent and digested following the method recommended by Stahr,65 10 mL of concentrated nitric acid was added and the flask was placed on the digester under gentle heating. When the initial brisk reaction was over, the solution was removed and cooled at room temperature. 1 mL of concentrated sulfuric acid was added carefully, followed by the addition of 2 mL of concentrated HF, and heating was continued for at least 1/2 h, followed by cooling. In the resulting solution, 5 mL of 3% (w/v) freshly prepared bromine water was added. The mixture of each foodstuff was heated for 15 min at that point by warming to a vapor to oxidize thallium(I) to thallium(III). The solutions were then cooled and neutralized with dilute NH4OH in the presence of 1–2 mL of 0.01% (w/v) EDTA solution. The resulting solution was filtered through a Whatman no. 40 filter paper and quantitatively transferred into a 25 mL calibrated flask, mixed well, and made up to the mark with de-ionized water.The food samples used were rice and wheat, and these were used under dry conditions. Each sample was first ground in a mortar. Corn and fruit samples (2 g) or rice and wheat samples (1 g) were weighed accurately and placed in a porcelain crucible and charred in an electric furnace; the sample was ashed at 555 °C in a muffle furnace in the presence of excess oxidizing agent, following a method recommended by Mitra.59 To it, 2.0 mL of HCl and 10 mL of water were added. The mixture of each foodstuff was heated with 5 mL of 3% (w/v) freshly prepared bromine water solution below the boiling point for 15 min to complete the oxidation from thallium(I) to thallium(III). The solutions were cooled and neutralized with dilute NH4OH in the presence of 1–2 mL of 0.01% (w/v) EDTA solution and filtered. The resulting solution was quantitatively transferred into a 25 mL calibrated flask, mixed well, and made up to the mark with de-ionized water.
A suitable aliquot (1–2 mL) of the final digested solution was pipetted into a 10 mL calibrated flask and the thallium content was determined as described under the general procedure using tartrate as the masking agent. The high value of thallium for tobacco was found.72 The results of food and vegetables analyses by the spectrofluorimetric method were also found to be in excellent agreement with those obtained by ICP-OES. The results are shown in Table 8.
Table 8 Determination of thallium in some food, fruit, and vegetable samples
Serial no. |
Sample |
Thallium/μg kg−1, founda |
Sample source |
ICP-OES (n = 5) |
Proposed method (n = 5) |
Found |
RSDb (%) |
Found |
RSDb (%) |
Average of five replicate analyses of each sample. The measure of precision is the relative standard deviation (RSD). |
1 |
Carrot (Daucuscarota) |
22.1 |
2.5 |
22.8 |
2.5 |
Local market, Chittagong |
2 |
Rice (Oryza sativa) |
2.4 |
1.0 |
2.5 |
1.0 |
Local market, Chittagong |
3 |
Wheat (Trictiumaestivum) |
3.8 |
1.2 |
4.0 |
1.2 |
Local market, Chittagong |
4 |
Potato (Solanumtuberosum) |
7.8 |
1.5 |
7.5 |
1.6 |
Local market, Chittagong |
5 |
Spinach (Spinaciaoleracea) |
15.9 |
2.1 |
15.8 |
2.2 |
Local market, Chittagong |
6 |
Radish (Raphanussativus) |
10.4 |
2.0 |
10.8 |
2.0 |
Local market, Chittagong |
7 |
Cabbage (Brassica oleracea) |
7.86 |
1.5 |
8.0 |
1.5 |
Local market, Chittagong |
8 |
Garlic (Allium sativum) |
6.8 |
1.3 |
6.5 |
1.3 |
Local market, Chittagong |
9 |
Cauliflower (Brassica oleracea) |
8.8 |
1.8 |
9.2 |
1.8 |
Local market, Chittagong |
10 |
Apple (Malusdomestica) |
7.5 |
1.5 |
7.8 |
1.5 |
Local market, Chittagong |
11 |
Tobacco (Nicotana tabacum) |
33.8 |
2.5 |
35.0 |
2.5 |
Local market, Chittagong |
12 |
Onion (Allium cepa) |
1.5 |
0.8 |
1.8 |
0.8 |
Local market, Chittagong |
13 |
Ginger (Zingiberofficinale) |
5.6 |
1.5 |
5.8 |
1.5 |
Local market, Chittagong |
14 |
Eggs (Gallus domesticus) |
3.8 |
1.0 |
4.5 |
1.0 |
Local market, Chittagong |
15 |
Lettuce (Lactuca sativa) |
22.2 |
2.0 |
22.5 |
2.0 |
Local market, Chittagong |
Determination of thallium(I) to thallium(III) speciation in mixtures. Suitable aliquots (1–2 mL) of thallium (I + III) mixtures (preferably 1
:
1, 1
:
5, 1
:
10) were taken in a 250 mL Pyrex conical flask and digested according to the procedure recommended by Rasool et al.73 10 mL of 8 M HNO3 and 5–10 mL of 3% (w/v) freshly prepared bromine water solution were added to oxidize thallium(I) to thallium(III) and the mixture was heated gently with the further addition of 10 mL water, if necessary, for 15 min, warming to a vapor, and then the mixture was cooled to room temperature (25 ± 5) °C. The reaction mixture was then cooled and neutralized with dilute NH4OH in the presence of 3–5 mL of 0.01% (w/v) EDTA solution. The solution was transferred quantitatively into a 25 mL volumetric flask and 1.5 mL of 3.77 × 10−3 M PQCTA reagent solution was added, followed by the addition of 1.0 mL of 0.05 M H2SO4. It was made up to the mark with de-ionized water. The fluorescence intensity was then measured by cooling to room temperature (25 ± 5) °C at 379 nm when excited at 324 nm, against a reagent blank. The total thallium content was calculated with the help of a calibration graph prepared concurrently.An equal aliquot (1–2 mL) of the above thallium (I + III) mixture was taken into a 250 mL Pyrex conical flask. The solution was neutralized with dilute NH4OH in the presence of 3–5 mL of 0.01% (w/v) EDTA solution. Afterward, the content of the beaker was transferred quantitatively into a 25 mL volumetric flask, 1.5 mL of 3.77 × 103 M PQCTA reagent solution was added, followed by the addition of 1.0 mL of 0.05 M H2SO4. It was made up to the mark with de-ionized water. After 5 min, the fluorescence intensity was measured following the general procedure at 379 nm when excited at 324 nm against a reagent blank, as before. The thallium concentration was calculated in μg L−1 or ng L−1 with the aid of a calibration graph. This gives a measure of thallium(III) originally present in the mixture. This value was subtracted from that of the total thallium to determine the thallium(I) amount present in the mixture. The results of the assessment of speciation of thallium(I) to thallium(III) were found to be highly reproducible. The occurrence of such reproducible results is also reported for different oxidation states of thallium.73,74 The results of a set of determination are given in Table 9.
Table 9 Determination of thallium(I) and thallium(III) speciation in mixtures
Tl(III) : Tl(I) |
Tl taken (μg L−1) |
Tl, found (μg L−1) |
Error (μg L−1) |
Tl(III) |
Tl(I) |
Tl(III) |
Tl(I) |
Tl(III) |
Tl(I) |
1 : 1 |
10.00 |
10.00 |
9.98 |
9.99 |
0.02 |
0.01 |
1 : 1 |
10.00 |
10.00 |
10.01 |
10.02 |
0.01 |
0.02 |
1 : 1 |
10.00 |
10.00 |
9.98 |
9.97 |
0.02 |
0.03 |
Mean error |
Tl(III) = ±0.017, Tl(I) = ±0.017 |
Standard deviation |
Tl(III) = ±0.012, Tl(I) = ±0.011 |
1 : 5 |
10.00 |
50.00 |
9.99 |
49.7 |
0.01 |
0.03 |
1 : 5 |
10.00 |
50.00 |
9.98 |
49.8 |
0.02 |
0.02 |
1 : 5 |
10.00 |
50.00 |
9.98 |
49.8 |
0.02 |
0.02 |
Mean error |
Tl(III) = ±0.017, Tl(I) = ±0.23 |
Standard deviation |
Tl(III) = ±0.0015, Tl(I) = ±0.0018 |
1 : 10 |
10.00 |
100.00 |
9.97 |
99.9 |
0.03 |
0.01 |
1 : 10 |
10.00 |
100.00 |
9.98 |
99.8 |
0.02 |
0.02 |
1 : 10 |
10.00 |
100.00 |
9.99 |
99.8 |
0.01 |
0.02 |
Mean error |
Tl(III) = ±0.02, Tl(I) = ±0.17 |
Standard deviation |
Tl(III) = ±0.0019, Tl(I) = ±0.0018 |
Speciation of thallium standard solution recovery and validation
To evaluate the applicability of the proposed method for real sample analysis, river water samples were selected for the determination of Tl(III) and/or Tl(I). River water samples were passed through a filter paper (Whatman no. 1) and then used directly for analysis. The standard addition method was used by the spiking of the samples with Tl(III) and/or Tl(I). In the event of adding PQCTA, Tl(III) would give fluorescence with PQCTA by oxidation. But Tl(I) could not oxidize the ligand PQCTA. Thus, Tl(I) and Tl(III) could be determined spectrofluorometrically in a slightly acetic aqueous solution. The concentration of the spiked samples of Tl(I) and Tl(III) are reported in Table 10. The highest percent spike recovery of Tl(III) in the FWS1 water sample was found with 74.84%, while the lowest spike recovery of 42.34% was found in sample FWS2, respectively (Table 10). Similarly, in the sample, the FWS3 percent spike recovery for Tl(I) and Tl(III) was observed to be 52.77% and 47.22%, respectively (Table 10). The average spiking recoveries of Tl(I) and Tl(III) in the water samples were 100.15 ± 1.05% and 103.34 ± 1.2%, respectively. However, the proposed method was successful for the determination of Tl(I) and Tl(III) in the freshwater using PQCTA. To verify the proposed method, the samples were analyzed by ICP-MS, and the average spiking recoveries of Tl(I) and Tl(III) in the water samples were 99.85 ± 1.8% and 99.93 ± 1.5%, respectively. The results of the speciation analyses by the spectrofluorimetric method were also found to be in excellent agreement with those obtained by ICP-MS. The results are shown in Table 10.
Table 10 Recovery of the spiked samples (added standard solution with original sample) during total thallium analysis
Spike sample number |
ICP-MS (n = 5)a |
Proposed method (n = 5)a |
Proposed method (n = 5)a |
Proposed method (n = 5)a |
Total Tl (μg L−1) |
Recovery (%) |
Total Tl (μg L−1) |
Recovery (%) |
Tl(I) (μg L−1) |
Recovery (%) |
Tl(III) (μg L−1) |
Recovery (%) |
Average of five replicate analyses of each sample. |
FWS1 |
135.98 |
99.85 |
136.38 |
100.15 |
35.39 |
25.45 |
100.85 |
74.84 |
FWS2 |
72.55 |
98.85 |
75.52 |
102.88 |
42.71 |
57.53 |
30.69 |
42.34 |
FWS3 |
28.99 |
99.93 |
29.98 |
103.34 |
15.32 |
52.77 |
13.69 |
47.22 |
Conclusions
The present study provided encouraging insights into the speciation of thallium in stream water and fresh water from a Tl-rich sulfide mineralized contaminated area. The main advantage of the proposed method is that it is simple and easy to implement with high reproducibility and selectivity for the determination of thallium speciation, and this methodology can be used for in situ thallium speciation analysis. This method can not only be applied to a wide range of pH but can tolerate a high ratio of Tl(I)/Tl(III). This method was successfully applied in the case of the analyzed real environmental, biological, food, and soil samples.
A new simple rapid, ultra-sensitive, highly selective, and inexpensive spectrofluorimetric method with the thallium-PQCTA system was developed for the determination of speciation of thallium (I and III) in some real, environmental, biological, food, vegetables, and soil samples for continuous monitoring to establish the pico-trace levels of thallium in different sample matrices.
Although many sophisticated techniques such as pulse polarography, HPLC, AAS, ICP-OES, and ICP-MS are available for the determination of thallium at the trace level in numerous complex materials, factors such as the low cost of the instrument, easy handling, less use of consumables, and almost no maintenance have made spectrofluorimetry a popular technique, particularly in laboratories of developing countries with limited budgets.
The proposed method has several remarkable analytical characteristics. It is sensitive enough that the ng L−1 amount of thallium can be determined without preconcentration in biological fluids. The low detection limit of 0.16 ng L−1 can be measured without preconcentration or the standard addition method. The reaction of thallium with PQCTA was to be found instantaneous in aqueous medium at room temperature (25 ± 5) °C. With suitable masking agents, the reaction can be made highly selective with better reproducibility (sr = 0.1). It is a new method that needs neither heating nor extraction to the organic phase, works satisfactorily, and could be an alternative method for the rapid routine total determination and speciation of thallium(I) and thallium(III) in a wide variety of sample matrices. It has also been found superior to existing spectrofluorimetric methods reported in the literature.22–40
Live subject statement
We were not aiming to carry out detailed human studies but some samples from individuals were used in our study and as such we abided by all the necessary procedures and regulations and our University gave consent. University of Chittagong, Bangladesh is committed to the protection and safety of human subjects involved in research.
Conflicts of interest
There are no conflicts of interest to declare.
Acknowledgements
We are thankful to the Authorities of Chittagong Medical College Hospital and Chittagong Treatment Hospital for supplying biological samples. We are also thankful to the Authorities of “Training Institute for Chemical Industries”(TICI), Polash, Norsindi, for analyzing water, biological, and food samples by Inductively Coupled Plasma-Optical Emission Spectrophotometer (ICP-OES) and ICP-MS. We are further thankful to the Center of Excellence in Analytical Chemistry, University of Sindh, Pakistan and Department of Chemistry, University of Kanazawa, Japan for elemental analysis, mass spectrometry, and 1H NMR spectroscopy for PQCTA, respectively.
References
- D. E. Guberman, Mineral Commodity Summaries 2010: Thallium, United States Geological Survey, 2010, pp. 64–75 Search PubMed.
- V. Zitko, W. V. Carson and W. G. Carson, Thallium: Occurrence in the environment and toxicity to fish, Bull. Environ. Contam. Toxicol., 1975, 13, 23–30, DOI:10.1021/ar50034a003.
- A. Peter and T. Viraraghavan, Thallium: a review of public health and environmental concerns, Environ. Int., 2005, 31, 493–501, DOI:10.1021/ar50034a003.
- E. Sabbioni, L. Ceotz and G. Bignoli, Health and environmental implications of trace metals released from coal-fired power plants: an assessment study of the situation in the European Community, Sci. Total Environ., 1984, 40, 141–154, DOI:10.1016/0048-9697(84)90348-6.
- A. Saddique and C. D. Peterson, Thallium poisoning: a review, Vet. Hum. Toxicol., 1983, 25, 16–22 CAS , PMID: 6338655..
- J. O. Nriagu, History, production and uses of thallium, in Thallium in the environment, ed. J. O. Nriagu, Wiley, New York, 1998, vol. 29, pp. 1–14 Search PubMed.
- Z. Groesslova, A. Vanek, M. Mihaljevic, V. Ettler, M. Hojdovác and T. Zádorováa, Bioaccumulation of thallium in a neutral soil as affected by solid-phase association, J. Geochem. Explor., 2015, 159, 208–212, DOI:10.1016/j.gexplo.2015.09.009.
- T. Xiao, J. Guha, D. Boyle, C. Q. Liu, B. Zheng and G. C. Wilson, Naturally occurring thallium: a hidden geo-environmental health hazard?, Environ. Int., 2004, 30, 501–507, DOI:10.1016/j.envint.2003.10.004.
- D. Shaw, The geochemistry of thallium, Geochim. Cosmochim. Acta, 1952, 2, 118–154, DOI:10.1016/0016-7037(52)90003-3.
- C. Wang, Y. Chen, J. Liu, J. Wang, X. Li and Y. Zhang, Health risks of thallium in contaminated arable soils and food crops irrigated with wastewater from a sulfuric acid plant in western Guangdong province, China, Ecotoxicol. Environ. Saf., 2013, 90, 76–81, DOI:10.1016/j.ecoenv.2012.12.014.
- J. P Mulkey and F. W. Oehme, A review of thallium toxicity, Vet. Hum. Toxicol., 1993, 35, 445–453 Search PubMed , PMID: 8249271..
- P. Medek, J. Pavlíčková, J. Zbíral, E. Čižmárová and V. Kubáň, Inductively Coupled Plasma Mass Spectrometric (ICP/MS) Determination of Thallium in Soils and Winter Rapeseeds, Int. J. Environ. Anal. Chem., 2001, 81, 207–219, DOI:10.1021/ar50034a003.
- K. Ostręga, M. Sadowska, K. Piotrowska and M. Wojda, Thallium (III) determination in the Baltic seawater samples by ICP-MS after preconcentration on SGX C18 modified with DDTC, Talanta, 2013, 112, 73–79, DOI:10.1021/ar50034a003.
- K. Urbánková and L. Sommer, The microdetermination of thallium by ICP-AES after previous preconcentration on modified silica. Comparison with integrated-platform graphite atomic absorption spectrometry, Microchim. Acta, 2008, 162, 127–132, DOI:10.1021/ar50034a003.
- N. R. Biata, K. M. Dimpe, J. Ramontja, N. Mketo and P. N. Nomngongo, Determination of thallium in water samples using inductively coupled plasma optical emission spectrometry (ICP-OES) after ultrasonic assisted-dispersive solid phase microextraction, Microchem. J., 2018, 137, 214–222, DOI:10.1021/ar50034a003.
- N. R. Biata, G. P. Mashile, J. Ramontja, N. Mketo and P. N. Nomngongo, Application of ultrasound-assisted cloud point extraction for preconcentration of antimony, tin and thallium in food and water samples prior to ICP-OES determination, J. Food Compos. Anal., 2019, 76, 14–21, DOI:10.1016/j.jfca.2018.11.004.
- R. A. Gil, P. H. Pacheco and P. Smichowski, Speciation analysis of thallium using electrothermal AAS following on-line pre-concentration in a microcolumn filled with multiwalled carbon nanotubes, Microchim. Acta, 2009, 167, 187–196, DOI:10.1007/s00604-009-0241-4.
- L. Husáková, T. Černohorský, J. Šrámková, K. Hubáčková and I. Doležalová, “Interference-free determination
of thallium in aqua regia leaches from rocks, soils and sediments by D2-ETAAS method using mixed palladium–citric acid–lithium chemical modifier”, Anal. Chim. Acta, 2008, 614, 38–45, DOI:10.1021/ar50034a003.
- M. Akhlaghi, M. Kamalidehghan, A. R. Jalilian and N. Shadanpoor, Determination of [201Tl] Tl(III) in [201Tl] TlCl solutions using HPLC, Appl. Radiat. Isot., 2008, 66, 479–481, DOI:10.1021/ar50034a003.
- A. S. Parakudyil, A. K. Pillai and S. B. Mathew, Sensitive spectrophotometric determination of thallium(I) using rhodamine B hydrazide in micellar medium, Anal. Methods, 2011, 3, 1546–1559, DOI:10.1021/ar50034a003.
- Z. Lukaszewski, W. Zembrzuski and A. Piela, Direct determination of ultratraces of thallium in water by flow-injection differential-pulse anodic stripping voltammetry, Anal. Chim. Acta, 1996, 318, 159–165, DOI:10.1021/ar50034a003.
- G. F. Kirkbright, T. S. West and C. Woodward, Spectrofluorimetric determination of microgram amounts of thallium, Talanta, 1965, 12, 517–524, DOI:10.1021/ar50034a003.
- M. M. Shnepfe, Spectrofluorimetric determination of thallium in silicate rocks with rhodamine b in the presence of aluminum chloride, Anal. Chim. Acta, 1975, 79, 101–108, DOI:10.1021/ar50034a003.
- A. García and D. B. Gomis, “Extraction–spectrofluorimetric determination of Thallium using cryptand ethers”, Anal. Lett., 2002, 35, 2337–2346, DOI:10.1081/AL-120016107.
- A. A. Ensafi and B. Rezaei, Speciation of Thallium by Flow Injection Analysis with Spectrofluorimetric Detection, Microchem. J., 1998, 60, 75–83, DOI:10.1021/ar50034a003.
- T. P. Ruiz, J. A. Ortuno and P. Molina, Extraction-fluorimetric determination of microgram amounts of thallium with 2-phenylbenzo[8,9]quinolizino[4,5,6,7-fed]phenanthridinylium perchlorate, Microchem. J., 1985, 31, 196–201, DOI:10.1021/ar50034a003.
- I. Mori, T. Matsuo, Y. Fujita, M. Toyoda, K. Ichitani and N. Inoue, Spectrofluorometric determination of thallium (III) with pyrogallol red and 3,4,5,6-tetrachlorofluorescein. Fresenius, J. Anal. Chem., 1994, 348, 346–349, DOI:10.1021/ar50034a003.
- H. Parham and M. Shamsipur, Spectrofluorometric study of thallium (I) complexes with several macrocyclic ligands in methanol solution, Talanta, 1993, 40, 1353–1356, DOI:10.1021/ar50034a003.
- T. P. Ruiz, C. S. Pedreo, M. H. córdoba, C. M. Lozano and P. M. Buendia, Extraction-spectrophotometric and fluorimetric determination of thallium with 2-(4-methoxyphenyl)-5,7-diphenyl-4h-l,3,4-thiadiazolo[3,2-α] pyridinium chloride, Anal. Chim. Acta, 1982, 143, 185–190, DOI:10.1021/ar50034a003.
- T. P. Ruiz, C. M. Lozano, V. Tomás and R. Casajús, Simple flow injection spectrofluorimetric method for speciation of thallium, Analyst, 1996, 121, 813–816, DOI:10.1021/ar50034a003.
- G. Shenguang, D. Ping, Y. Jinghua, Z. Yuanna, H. Jiadong, Z. Congcong, G. Lei and W. Fuwei, Determination of thallium(III) with novel arsenoxylphenylazo rhodanine after pre-concentration and separation, Int. J. Environ. Anal. Chem., 2010, 90, 1139–1147, DOI:10.1021/ar50034a003.
- M. Sagar, Chemical speciation and environmental mobility of thallium in sediments and soils, Techniques & Instrumental in Analytical Chemistry, 1992, 12, 133–175, DOI:10.1016/S0167-9244(08)70106-9.
- D. Xiangzhou, W. Liu, Q. Zhang, X. Li, Y. Ou and Z. Zhong, Determination of Thallium (III) with Fluorescence Quenching Method, Guangdong Chem. Ind., 2005, 32, 63–95 Search PubMed . http://kpmg.com/cn.
- R. Böhmer, Determination of thallium in rock and soil samples, Talanta, 1977, 24, 521–523, DOI:10.1021/ar50034a003.
- H. Koshima and H. Onishi, Fluorimetric determination of thallium in silicate rocks with Rhodamine B after separation by adsorption on a crown ether polymer, Analyst, 1989, 114, 615–621, DOI:10.1021/ar50034a003.
- H. Onishi, Fluorometric Determination of Thallium with Rhodamine B, Bull. Chem. Soc. Jpn., 1957, 30, 827–828, DOI:10.1021/ar50034a003.
- D. Matthews and J. P. Kiley, The determination of thallium in silicate rocks, marine sediments and sea water, Anal. Chim. Acta, 1969, 48, 25–34, DOI:10.1016/S0003-2670(01)85238-7.
- P. Salinas, C. Genestar and P. Grases, Kinetic fluorimetric determination of iron and thallium based on oxidation transformation of 1, 4-diamino-2, 3-dihydroanthraquinone, Anal. Chim. Acta, 1981, 130, 337–344, DOI:10.1021/ar50034a003.
- F. Salinas, F. G. sanchez and C. Genestar, Fluohinetric Determination of Iron and Tpalliur with 1, 4-Diami No-2, 3-Dihyoroanthraquinone, Anal. Lett., 1982, 15, 747–756, DOI:10.1021/ar50034a003.
- E. D. Roper, V. S. Talanov, M. G. Gorbunova, R. A. Bartsch and G. G. Talanova, Optical Determination of Thallium(I) and Cesium(I) with a Fluorogenic Calix[4]arenebis(crown-6 ether) Containing One Pendent Dansyl Group, Anal. Chem., 2007, 79, 1983–1989, DOI:10.1021/ar50034a003.
- M. Sanchez-Vinas, G. M. Bagur, D. Gasquez, M. Camino and R. J. Romero, Determination of Tin, Vanadium, Iron, and Molybdenum in Various Matrices by Atomic Absorption Spectrometry Using a Simultaneous Liquid-Liquid Extraction Procedure, J. Anal. Toxicol., 1999, 23, 108–116, DOI:10.4236/ajac.2019.1011038.
- U.S. Environmental Protection Agency, Pyridine, 90-Day Sub-Chronic Oral Toxicity in Rats, Office of Solid Waste, Washington DC, 1986 Search PubMed.
- H. D. Porter, The Willgerodt Reaction Applied to α- and γ-Alkylpyridines, J. Am. Chem. Soc., 1954, 76, 127–128, DOI:10.1021/ja01630a035.
- B. K. Pal, A. K. Chakraborti and M. Jamaluddin Ahmed, 2-(α-pyridyl)- thioquinaldinamide: a novel fluorimetric reagent in inorganic trace analysis. Part 2. A simple selective determination of selenium (IV) at ultratrace levels, Anal. Chim. Acta, 1988, 206, 351–355, DOI:10.1016/S0003-2670(00)80856-9.
- B. K. Pal, M. Jamaluddin Ahmed and A. K. Chakrabarti, 2-(α-Pyridyl)-thioquinaldinamide (PTQA)- A Novel Fluorimetric Reagent in Inorganic Trace Analysis. Part I: The Non-extractive, Non-quenching Fluorescent Method for the Determination of Chromium (VI), Microchim. Acta, 1989, I, 393–401, DOI:10.1007/BF01242262.
- M. Jamaluddin Ahmed, M. T. Islam and F. Hossain, A highly sensitive and selective spectrofluorimetric method for the determination of manganese at nanotrace levels in some real, environmental, biological, soil, food and pharmaceutical samples using 2-(α-pyridyl)-thioquinaldinamide, RSC Adv., 2018, 8, 5509–5522, 10.1039/C7RA12762F.
- M. Jamaluddin Ahmed, A. Afrin and M. Rashid, A highly sensitive and selective spectrofluorimetric method for the determination of arsenic at Pico-trace levels in some groundwater, real, environmental, biological, food and soil samples using 2-(α-Pyridyl)-thioquinaldinamide, Am. J. Anal. Chem., 2019, 10, 316–347, DOI:10.4236/ajac.2019.108023.
- M. Jamaluddin Ahmed, M. T. Islam and F. Farhana, A highly sensitive and selective spectrofluorimetric method for the determination of cerium at pico-trace levels in some real, environmental, biological, soil, food and bone samples using 2-(α-pyridyl)-thioquinaldinamide, RSC Adv., 2019, 9, 25609–25626, 10.1039/C9RA02850A.102.
- M. Jamaluddin Ahmed, A. Afrin and M. Emdadul Hoque, A Highly Sensitive and Selective Spectrofluorimetric Method for the Determination of Molybdenum at Pico-trace Levels in some Real, Environmental, Biological, Food and Soil Samples Using N-(pyridin-2-yl)-quinoline-2-carbothioamide‖, Eur. J. Chem., 2021, 12, 1–12 CrossRef https://10.5155/eurjchem.12.1.1-12.2031.
- Vogel's Textbook of Quantitative Chemical Analysis, ed. G. H. Jeffery, J. Bassett, J. Mendham and R. C. Denney, Bath Press Ltd., London, ELBS of 5th edn, 1994, pp. 275–280 Search PubMed.
- A. K. Mukharjee, Analytical Chemistry of Zirconium and Hafnium, Pergamon Press, New York, 1st edn, 1970, pp. 12–35 Search PubMed.
- B. K. Pal and B. Chowdhury, Triazene-N-oxides as new type of fluorimetric reagents. Part I. trace determination of zirconium at the nanogram level, Mikrochim. Acta, 1984, 83, 121–131, DOI:10.1007/BF01237266.
- M. A. Omar, D. M. Nagy and M. E. Halim, Luminescence, 2019, 34, 84–90 CAS.
- C. B. Ojeda, A. G. de Torres, F. S. Rojas and J. M. C. Pavon, Fluorimetric determination of trace amounts of gallium in biological tissues, Analyst, 1987, 112, 1499–1501, 10.1039/AN9871201499.
- M. Jamaluddin Ahmed, C. D. Stalikas, P. G. Veltsistas, S. M. Tzouwara-Karayanni and M. I. Karayannis, Simultaneous spectrofluorimetric determination of selenium (IV) and (VI) by flow injection analysis, Analyst, 1997, 122, 221–226, 10.1039/A606357H.
- B. K. Pal, M. Jamaluddin Ahmed and A. K. Chakraborty, 2-(α-Pyridyl) thioquinaldinamide: a spectrofluorimetric reagent in inorganic trace analysis. Part III: Determination of manganese in industrial and environmental samples, Analyst, 1990, 115, 439–443, 10.1039/AN9901500439.
- B. K. Pal, F. Toneguzzo, A. Corsini and D. E. Ryan, Anal. Chim. Acta, 1977, 88, 353–361, DOI:10.1016/S0003-2670(01)95910-0.
- J. Holzbecher and D. E. Ryan, Anal. Chim. Acta, 1973, 64, 333–341 CrossRef CAS.
- M. Jamaluddin Ahmed, A. Afrin and Y. Akhtar, A Highly Sensitive and Selective Spectrofluorimetric Method for the Determination of Vanadium at Pico-Trace Levels in Some Real, Environmental, Biological, Soil and Food Samples Using 2-(α-Pyridyl)-Thioquinaldinamide, Am. J. Anal. Chem., 2019, 10, 528–561, DOI:10.4236/ajac.2019.1011038.
- Sample Preparation Techniques in Analytical Chemistry, ed. S. Mitra, Wiley-Intersciences, New Jersey, 2003, pp. 125–162 Search PubMed.
- J. Sun, X. Zou, Z. Ning, M. Sun, J. Peng and T. Xiao, Culturable microbial groups and thallium-tolerant fungi in soils with high thallium contamination, Sci. Total Environ., 2012, 441, 258–264, DOI:10.1016/j.scitotenv.2012.09.053.
- Standard Methods for the Examination of Water and Wastewater, ed. E. A. Greenberg, S. L. Clesceri and D. A. Eaton, American Public Health Association, Washington DC, 18th edn, 1992, pp. 253–268 Search PubMed.
- G. Shenguang, D. Ping, Y. Jinghua, Z. Yuanna, H. Jiadong, Z. Congcong, G. Lei and W. Fuwei, Determination of thallium(III) with novel arsenoxylphenylazo rhodanine after pre-concentration and separation, Int. J. Environ. Anal. Chem., 2010, 90, 1139–1145, DOI:10.1021/ar50034a003.
- J. Lis, A. Pasieczna, B. Karbowska, W. Zembrzuski and Z. Lukaszewski, Thallium in soils and stream sediments of a Zn–Pb mining and smelting area, Environ. Sci. Technol., 2003, 37, 4569–4572, DOI:10.1021/ar50034a003.
- Z. Lukaszewski, B. Karbowska, W. Zembrzuski and M. Siepak, Thallium in fractions of sediments formed during the 2004 tsunami in Thailand, Ecotoxicol. Environ. Saf., 2012, 80, 184–189, DOI:10.1016/j.ecoenv.2012.02.026.
- H. M. Stahr, Analytical Methods in Toxicology, John Wiley and Sons, New York, 3rd edn, 1991, pp. 85–94 Search PubMed.
- B. Mathew, B. Narayana and B. M. Rao, Complexometric determination of thallium (III) in pure solution, alloys, and complexes using semicarbazide hydrochloride as a releasing agent, Mikrochim. Acta, 1996, 122, 295–299, DOI:10.1007/BF01245789.
- J. Dai, X. Wu, Y. Bai, W. Feng, S. Wang, Z. Chen and H. Guo, Effect of thallium exposure and its interaction with smoking on lung function decline: A prospective cohort study, Environ. Int., 2019, 127, 181–189, DOI:10.1021/ar50034a003.
- P. W. Pau, Management of thallium poisoning, Hong Kong Med. J., 2000, 3, 316–328 Search PubMed , PMID:11025853..
- S. Jha, R. Kumar and R. Kumar, Thallium poisoning presenting as paresthesias, paresis, psychosis and pain in abdomen, J. Assoc. Physicians India, 2006, 54, 53–65 CAS . http://hdl.handle.net/123456789/4034.
- M. L. Jackson, Soil Chemical Analysis, Prentice Hall, Englewood Cliffs, 1965, pp. 326–332 Search PubMed.
- A. Ghaderi, P. N. Ghafoori and M. R. Azad, et al. Examining of Thallium in Cigarette Smokers, Biol. Trace Elem. Res., 2018, 182, 224–230, DOI:10.1007/s12011-017-1107-y.
- A. Rasool, T. Xiao and S. Ali, Waqar Ali and Wajid Nasim “Quantification of Tl (I) and Tl (III) based on micro-column separation through ICP-MS in river sediment pore water”, Environ. Sci. Pollut. Res., 2020, 27, 9686–9696, DOI:10.1007/s11356-019-07553-1.
- N. N. Meeravali and S. J. Jiang, Ultra-trace speciation analysis of thallium in environmental water samples by inductively coupled plasma mass spectrometry after a novel sequential mixed-micelle cloud point extraction, J. Anal. At. Spectrom., 2008, 23, 555–560, 10.1039/B718149C.
Footnote |
† Electronic supplementary information (ESI) available. See DOI: 10.1039/d1ra05388d |
|
This journal is © The Royal Society of Chemistry 2021 |
Click here to see how this site uses Cookies. View our privacy policy here.