DOI:
10.1039/D1RA05708A
(Paper)
RSC Adv., 2021,
11, 30373-30376
Industrial kinetic resolution of D,L-pantolactone by an immobilized whole-cell biocatalyst†
Received
26th July 2021
, Accepted 2nd September 2021
First published on 13th September 2021
Abstract
Immobilized whole-cells of Pichia pastoris harboring recombinant D-lactonase were entrapped in calcium alginate gels and used as an efficient biocatalyst for catalytic kinetic resolution of D,L-pantolactone. The immobilized whole-cell biocatalyst exhibited good catalytic stability, which was applied for stereospecific hydrolysis of D-pantolactone for up to 56 repeated batch reactions without obvious loss in the catalytic activity and enantioselectivity.
Introduction
Optically active D-pantolactone (D-PL) is an important chiral intermediate for the preparation of calcium D-pantothenate and its derivatives, e.g. D-pantetheine and D-panthenol. These useful compounds are key ingredients in pharmaceuticals and useful additives for animal feed.1,2 Annual global production of calcium D-pantothenate is approximately 30
000 t. One of the practical strategies for commercial production of chiral D-PL is synthesis of racemic D,L-PL via an aldol condensation and a hydrogen cyanide (HCN) addition,3 followed by an enzymatic kinetic resolution of D,L-PL using an enantioselective D-lactonase (Scheme 1). In the enzymatic resolution process, chiral D-pantotic acid (D-PA) hydrolyzed from D-PL is separated and chemically lactonized to generate chiral D-PL. The unresolved L-PL is racemized to D,L-PL and reused as a starting material for the next round of kinetic resolution.
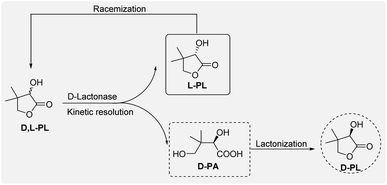 |
| Scheme 1 Chemoenzymatic process for commercial production of D-PL. | |
More than twenty years ago, Shimizu and his coworkers discovered a fungi strain Fusarium oxysporum which could produce high enantioselective and active D-lactonase for stereospecific hydrolysis of D-PL.4–6 This strain has been commercialized for enzymatic kinetic resolution of D,L-PL by Fuji/Daiichi Chemicals (Japan). Later, another D-lactonase-generated strain F. moniliforme SW-902 was identified by Tang and his coworkers, which has also been used for commercial preparation of D-PL by Zhejiang Xinfu Biochemical Co., Ltd (China).7 Many efforts have been devoted to achieve heterologous expression of these D-lactonases in strains Escherichia coli, Saccharomyces cerevisiae and Aspergillus oryzae.8–11 We recently have identified, cloned and expressed a novel D-lactonase TSDL from strain Thielavia sp. zmu20201 in E. coli BL21 (DE3).12 The recombinant E. coli (TSDL) exhibits high catalytic activity and enantioselectivity to D-PL.
The higher retention of activity for long-term repeated biotransformation is a key evaluation index of commercial biocatalyst. However, dramatic decrease in catalytic activity has been observed when reusing free cells,4,7 which is a major disadvantage for a commercial enzymatic process. The stability issue was also found in repeated reactions with free cells of the recombinant E. coli (TSDL). Immobilization of whole cells and enzymes is one of the most frequently used method to improve the stability of biocatalyst.13 In addition, the immobilized biocatalysts can be easily recovered from the reaction mixture. For long-term repeated stereospecific hydrolysis of D-PL, the mycelium of F. oxysporum and F. moniliforme have been immobilized via entrapping in calcium alginate gels and k-carrageenan, respectively.6,7 The estimated stability of immobilized mycelium are higher than that of the free mycelium. We recently constructed a recombinant strain Pichia pastoris ZMU-125 for intracellular expression of D-lactonase, while the free cells also showed short-term repeated reactions when they were used for kinetic resolution of D,L-PL. Herein, we report the preparation of immobilized P. pastoris cells for industrial enzymatic resolution of D,L-PL.
Results and discussion
The recombinant P. pastoris ZMU-125 whole-cells were prepared in a 30 L fermentor, harvested and stored at −20 °C for further study of cell immobilization. Calcium alginate was selected as the entrapment carrier for the whole cell immobilization. Our study began with the investigation of cell immobilization conditions. The immobilized cells prepared at each condition were used for ten batch of repeated reactions against 220 g L−1 D,L-PL. The reaction pH was maintained at 7.0 ± 0.2 with 15 M NH3·H2O. The concentration of sodium alginate was first investigated and the result was showed in Fig. 1a. It could be found that the immobilized cells exhibited relatively good stability with 20 g L−1 sodium alginate. The lower concentration of sodium alginate might result in the low mechanical strength of the immobilized globules, which makes immobilized cell easy to disintegrate. On the other hand, the higher concentration of sodium alginate led to the formation of irregular immobilized globules, which would reduce the efficiency of substrate in and out of gel matrix. The concentration of glutaraldehyde was then examined. As shown in Fig. 1b, the good stability of immobilized cell was obtained by using 20 g L−1 glutaraldehyde. The high concentration glutaraldehyde might cause enzyme activity loss. The cell concentration was examined and the result was displayed in Fig. 1c. The optimal cell concentration for immobilization was 80 g L−1. The high cell concentration caused the formation of irregular immobilized ball and reduce the catalytic efficiency.
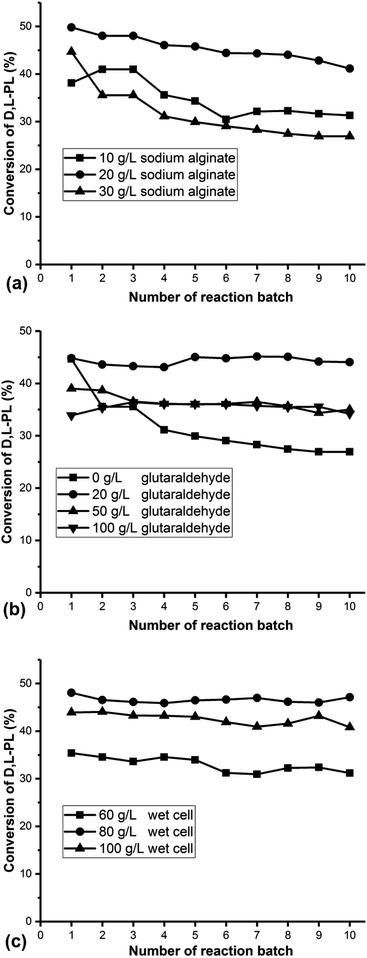 |
| Fig. 1 Optimization of cell immobilization conditions: (a) concentration of sodium alginate; (b) concentration of glutaraldehyde; (c) concentration of wet cell of P. pastoris ZMU-125. | |
Subsequently, the immobilized whole-cells prepared under the optimized conditions (20 g L−1 sodium alginate, 20 g L−1 glutaraldehyde and 80 g L−1 wet cell of P. pastoris ZMU-125) were used for catalytic kinetic resolution of D,L-PL at different substrate concentrations ranging from 220 to 350 g L−1. As shown in Fig. 2, 47% conversion was achieved for hydrolysis of 220 g L−1 D,L-PL after reaction for 10 h. In the same reaction time, slight decreases in conversions (42–45%) were observed by increasing substrate concentrations. These results indicated that high substrate concentrations are tolerated by the immobilized cells.
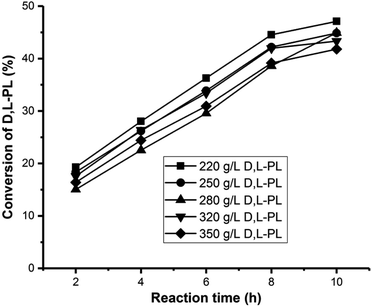 |
| Fig. 2 Time courses of kinetic resolution of D,L-PL using immobilized P. pastoris ZMU-125 against different substrate concentrations. | |
With stable immobilized cells in hand, we next investigated the continuous hydrolysis of D,L-PL for the production of D-PA. The continuous hydrolysis craft was investigated in a 2 L round-bottom flask by continuously feeding substrate solution with the concentration of 350 g L−1 (see ESI† for details). The flow rates of substrate entrance and product output were controlled by maintaining the resolved reaction mixture with >40% hydrolysis conversion. As shown in Fig. 3, catalytic hydrolysis of about 1490 g D,L-PL were completed after 312 h. However, the optical purity of the D-PA decreased from 95.8% (20 h) to 89.2% (300 h). The productivity of continuous hydrolysis reaction was three times lower than that of single batch reaction.
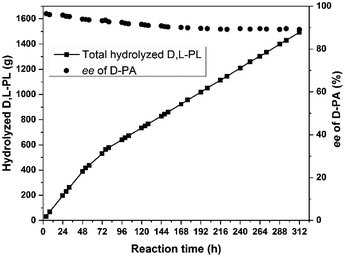 |
| Fig. 3 Time course of continuous catalytic hydrolysis of D,L-PL. | |
The single batch reaction has the simpler operation. Additionally, both reaction temperature and the pH of the reaction mixture could be easily controlled in a single batch reaction. Therefore, we decided that the optimum reaction conditions for industrial kinetic resolution of D,L-PL on a 50 t stirred tank reactor were: substrate, 280 g L−1 D,L-PL, 26 t; biocatalyst, immobilized cell (about 2.4 t wet whole cells of P. pastoris ZMU-125, prepared in a 1.5 t fermentor), 16 t; stabilizer, CaCl2, 130 kg; temperature, 28 °C; pH, 7.0 (adjusted by addition of 15 M NH3·H2O); reaction time, 11–12 h. As shown in Fig. 4, we monitored the repeated reactions with 56 batch for a total reaction time of about 620 h. No obvious decrease of the residual enzyme activity and no significant disintegration of the immobilized gel were observed after 56 repeated reactions. The optical purity of the formed D-PA was >90% ee and hydrolysis conversion for D,L-PL was >40%. To obtain the chiral D-PL in each batch reaction, 2.2 t concentrated sulfuric acid was added to the reaction mixture for chemical lactonization of D-PA, after filtration separation of immobilized biocatalyst. The mixture was stirred at 95 °C for 1 h, followed by extraction with ethyl acetate and vacuum distillation to give 3.08 t D-PL (42% yield, ee >90%).
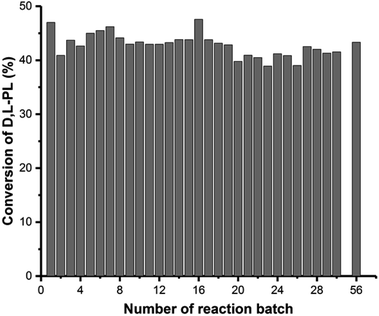 |
| Fig. 4 Repeated batch reactions for industrial kinetic resolution of D,L-PL with immobilized cell. | |
Experimental
General procedure for preparation of immobilized cell
To a 5 L round-bottom flask, 1000 mL water containing 80 g wcw per L cells of recombinant P. pastoris ZMU-125 was added. To it 40 g glutaraldehyde (50%) was then added under stirring condition. After stirring at 4 °C for 10 h, the mixture was centrifuged and separated to obtain precipitate. After washing with water (2 × 1000 mL), the precipitate was resuspended with 1000 mL water to obtain cell suspension. To another 5 L round-bottom flask, 10 g sodium alginate and 1000 mL water were added. The mixture was stirred at 85 °C until complete dissolution of sodium alginate, maintained at 85 °C for further 2 h, and then cooled to 30 °C. To the sodium alginate solution 1000 mL cell suspension was added, and the mixture was stirred at 30 °C for 1 h. Then the cell-gel mixed solution was dripped slowly to 2% CaCl2 solution and stirred for 2 h to obtain the immobilized cell. The obtained immobilized cell was then washed with water and stored at 4 °C for further use.
General procedure for kinetic resolution of D,L-PL with immobilized cell
100 mL substrate solution (280 g L−1 D,L-PL), 70 g immobilized cell and 0.5 g CaCl2 were added to a 500 mL round-bottom flask. The reaction pH was controlled at 7.0 ± 0.2 using 15 M NH3·H2O. After incubation at 28 °C for 8 h with stirring, the mixture was filtered and separated. The isolated immobilized cell was used for the next biotransformation reaction. The filtrates were analyzed by HPLC for calculating the conversions of D,L-PL and ee values of D-PA.
General procedure for continuous hydrolysis of D,L-PL with immobilized cell
To a 2 L round-bottom flask, 1000 mL substrate solution (350 g L−1 D,L-PL), 700 immobilized cell and 5 g CaCl2 were added. The mixture was incubated at 28 °C with stirring, and the reaction pH was controlled at 7.0 ± 0.2 using 15 M NH3·H2O. Substrate solution (350 g L−1) was added via peristaltic pump and the reaction mixture overflowed from the reactor with feeding substrate solution. Reaction was monitored by taking samples for HPLC analysis of the conversions of D,L-PL and ee values of D-PA. The schematic diagram for continuous hydrolysis of D,L-PL was displayed in ESI.†
General procedure for industrial kinetic resolution with immobilized cell
26 t substrate solution (280 g L−1 D,L-PL), 16 t immobilized cell and 130 kg CaCl2 were added to a 50 t stirred tank reactor. The mixture was stirred (100 rpm) and 28 °C, and the reaction pH was controlled at 7.0 ± 0.2 using 15 M NH3·H2O. The reaction was finished after 11–12 h with the hydrolysis conversion >40% by taking sample for analysis. The mixture was filtered and the isolated immobilized cell was used for the next repeated reaction under the same conditions. After chemical lactonization and purification, about 3.08 t D-PL was obtained in 42% yield. Optical rotation: [α]20D = −49.5 (c = 5, H2O). 1H NMR (400 MHz, CDCl3) δ 4.16 (s, 1H), 3.95 (dd, J = 26.9, 8.9 Hz, 2H), 3.88 (s, 1H), 1.18 (s, 3H), 1.04 (s, 3H); 13C NMR (100 MHz, CDCl3) δ 178.3, 76.6, 75.7, 40.8, 22.8, 18.9.
Analytic methods
The conversion of D,L-PL was analyzed by HPLC on an ODS-3 column (4.6 × 250 mm, 5 μm, Shimadzu, Tokyo, Japan) at 210 nm with a mobile phase of 10% acetonitrile containing 0.02 mM KH2PO4 with a flow rate of 1.0 mL min−1. The retention times of D,L-PA and D,L-PL were 4.93 and 8.98 min, respectively. The optical purity of D-PA was determined by HPLC on an MCI GEL CRS10W packed column (4.6 × 50 mm, 3 μm, Mitsubishi Kasei, Tokyo, Japan) at 254 nm with a mobile phase of 10% acetonitrile containing 1.8 mM CuSO4 with a flow rate of 0.8 mL min−1. The retention times of D-PA and L-PA were 5.4 and 7.5 min, respectively. Chiral GC analysis of D-PL and L-PL was performed on Agilent 7890B gas chromatograph equipped with a flame ionization detector (FID) using CYCLODEX-B column with nitrogen as the carrier gas (80 °C for 10 min, 10 °C min to 130 °C, and 130 °C for 10 min, total 25 min). The retention times of L-PL and D-PL were 17.8 and 18.3 min, respectively.
Conclusions
In this study, we developed an efficient and practical enzymatic process for catalytic kinetic resolution of D,L-PL using immobilized cell. To the best of our knowledge, it was the first report industrial production of D-PA using a recombinant P. pastoris strain harboring D-lactonase. Compared to the enzymatic process using immobilized mycelium of F. oxysporum, and F. moniliforme, our technology highly shortened the reaction time. The kinetic resolution process has been exploited commercially in our company since 2019 for annual production of 3000 t D-PL.
Author contributions
Q.-H. Zhang, L. Yang, Y.-B. Tang, L.-N. Huang and W.-F. Luo performed the experiments, analyzed the results and participated in writing the paper. Q.-H. Zhang supervised the project.
Conflicts of interest
There are no conflicts to declare.
Acknowledgements
Authors thank the Dr Nan-Wei Wan, School of Pharmacy, Zunyi Medical University, for offering the recombinant strain P. pastoris ZMU-125. This work was supported by the Jiangxi Brother Pharmaceutical Co., LTD
Notes and references
- T. Rowicki, L. Synoradzki and M. Włostowski, Ind. Eng. Chem. Res., 2006, 45, 1259–1265 CrossRef CAS.
- L. Synoradzki, H. Hajmowicz, J. Wisialski, A. Mizerski and T. Rowicki, Org. Process Res. Dev., 2008, 12, 1238–1244 CrossRef CAS.
- W. Bonrath and T. Netscher, Appl. Catal., A, 2005, 280, 55–73 CrossRef CAS.
- M. Kataoka, K. Shimizu, K. Sakamoto, H. Yamada and S. Shimizu, Appl. Microbiol. Biotechnol., 1995, 44, 333–338 CrossRef CAS.
- M. Kataoka, K. Shimizu, K. Sakamoto, H. Yamada and S. Shimizu, Appl. Microbiol. Biotechnol., 1995, 43, 974–977 CrossRef CAS.
- K. Sakamoto, K. Honda, K. Wada, S. Kita, K. Tsuzaki, H. Nose, M. Kataoka and S. Shimizu, J. Biotechnol., 2005, 118, 99–106 CrossRef CAS PubMed.
- Y.-X. Tang, Z.-H. Sun, L. Hua, C.-F. Lv, X.-F. Guo and J. Wang, Process Biochem., 2002, 38, 545–549 CrossRef CAS.
- M. Kobayashi, M. Shinohara, C. Sakoh, M. Kataoka and S. Shimizu, Proc. Natl. Acad. Sci. U. S. A., 1998, 95, 12787–12792 CrossRef CAS PubMed.
- K. Honda, H. Tsuboi, T. Minetoki, H. Nose, K. Sakamoto, M. Kataoka and S. Shimizu, Appl. Microbiol. Biotechnol., 2005, 66, 520–526 CrossRef CAS PubMed.
- Z. Liu, Z. Sun and Y. Leng, J. Agric. Food Chem., 2006, 54, 5823–5830 CrossRef CAS PubMed.
- Z. Liu and Z. Sun, Biotechnol. Lett., 2004, 26, 1861–1865 CrossRef CAS PubMed.
- Q.-H. Zhang, Y. Fang, W.-F. Luo and L.-N. Huang, RSC Adv., 2021, 11, 721–725 RSC.
- B. Mattiasson, in Immobilized Cells and Organelles, CRC Press, vol I, 2018, pp. 3–26 Search PubMed.
Footnote |
† Electronic supplementary information (ESI) available. See DOI: 10.1039/d1ra05708a |
|
This journal is © The Royal Society of Chemistry 2021 |
Click here to see how this site uses Cookies. View our privacy policy here.