DOI:
10.1039/D1RA06150J
(Paper)
RSC Adv., 2021,
11, 32837-32840
One-pot synthesis, structural analysis, and oxidation applications of a series of diaryltellurium dicarboxylates†
Received
14th August 2021
, Accepted 29th September 2021
First published on 5th October 2021
Abstract
This paper presents a concise and efficient one-pot synthesis of a variety of functionalized diaryltellurium dicarboxylates. The method is based on a mild photosensitized oxygenation of cheap and readily available carboxylic acids. The molecular structures of the diaryltellurium dicarboxylates were determined unambiguously using single-crystal X-ray diffraction analysis. The thus obtained diaryltellurium dicarboxylates were used to study the oxidation of benzoin derivatives.
Recently we have taken interest in the synthesis of organotellurium compounds and the study of their behavior in organic reactions. Specifically, we have reported on the synthesis of organotellurium compounds as well as the oxidation of organic compounds using organotellurium compounds such as telluroxides.1–4
We have been interested in extending our methodology to telluroxide derivatives such as diaryltellurium dicarboxylates. Although a method for the synthesis of related diallyl tellurium dicarboxylates has already been reported, it is a complicated reaction that requires very precise reaction conditions. Moreover, there are few reports on the detailed crystallographic characterization of diallyl tellurium dicarboxylates.
So far, the main method for the synthesis of diallyl tellurium dicarboxylates has been to react a diallyl tellurium or a diallyl tellurium derivative with a hypervalent iodine reagent.5,6 This method requires hypervalent reagents that are expensive and potentially explosive. Chandrasekhar et al. have reported the synthesis of diallyl tellurium dicarboxylates using diallyl telluroxides and carboxylic acids. However, this method requires the conversion of a diallyl tellurium to a diallyl telluroxide.7 It is generally known that the oxidation of a diallyl tellurium to a diallyl telluroxide using peroxides such as NaIO4 carries the risk of explosion. Chandrasekhar et al. also succeeded in synthesizing tellurium ferrocene carboxylates using this same synthetic method.8 Furthermore, there is a report on a similar synthetic method for the generation of macrocyclic multi-telluranes using telluronium salts prepared from telluroxides and triflic anhydride.9
In this study, we demonstrate a simple and safe method for the synthesis of diaryltellurium dicarboxylates using inexpensive carboxylic acids and air as the source of oxygen. In addition, the molecular structures of the diaryltellurium dicarboxylates in the solid state were determined unambiguously via a single-crystal X-ray diffraction analysis.
Synthesis
Initially, we investigated the transformation of a diaryltelluride into a diaryltellurium dicarboxylate under a variety of reaction conditions. For that purpose, a solution of dimesityltelluride, 2.2 equivalents of trifluoroacetic acid (TFA), and a photosensitizer [fullerene-C60, tetraphenylporphyrin (TPP), methylene blue (MB), rose bengal (RB), or eosin Y] was stirred at room temperature, whilst being exposed to irradiation from a white LED. The product yields are summarized in Table 1. We found that fullerene-C60 and TPP were effective photosensitizers for this reaction, while RB, MB, and eosin Y (Table 1, entries 8–10) were ineffective. Furthermore, the reaction did not proceed in the dark or under a nitrogen atmosphere (Table 1, entries 6, 7). In other words, this reaction requires oxygen from the surrounding air and light for optimal results. The best results for this model reaction were obtained using TPP as the photosensitizer with LED irradiation in an open flask (Table 1, entry 5).
Table 1 Optimisation of the reaction conditionsa
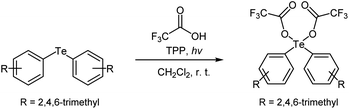
|
Entry |
Sensitizerb |
Time (h) |
Conditions |
Yieldc (%) |
Conditions: dimesityltelluride (0.1 mmol), TFA (0.22 mmol), sensitizer, and CH2Cl2 (10 mL) under aerobic conditions at room temperature. TPP, RB and MB denote tetraphenylporphyrin, rose bengal and methylene blue, respectively. Determined using 1H NMR spectroscopy. |
1 |
Fullerene-C60 (1 mol%) |
1.5 |
Air |
97 |
2 |
Fullerene-C60 (0.1 mol%) |
1.5 |
Air |
— |
3 |
TPP (1 mol%) |
1.5 |
Air |
97 |
4 |
TPP (0.1 mol%) |
1.5 |
Air |
Quant. |
5 |
TPP (0.01 mol%) |
3 |
Air |
Quant. |
6 |
TPP (0.01 mol%) |
3 |
N2 |
33 |
7 |
TPP (0.01 mol%) |
3 |
Dark, air |
6 |
8 |
RB (1 mol%) |
1.5 |
Air |
n.r. |
9 |
MB (1 mol%) |
1.5 |
Air |
19 |
10 |
EosinY (1 mol%) |
1.5 |
Air |
3 |
11 |
None |
1.5 |
Air |
n.r. |
Next, we used the optimal reaction conditions to investigate the substrate scope of the reaction (Table 2). When diphenyltelluride, dimesityl telluride, or bis(4-methoxyphenyl) telluride ware reacted with acetic acid, the corresponding diaryltellurium dicarboxylates (1a, 1b, and 1d) were obtained in good yields. However, bis(4-fluorophenyl) telluride was not reactive under these conditions and the reaction at room temperature yielded many by-products. However, when this reaction was performed at 0 °C, the target product (1c) was obtained. Next, the reactions of various carboxylic acids with diphenyltelluride or dimesityl telluride were investigated. In all cases, the reaction proceeded to give the expected diaryltellurium dicarboxylates in good to excellent yields (2a–6b).
Table 2 Substrate scope of the reactionc
Run at 0 °C. 0.25 mmol of carboxylic acid was used. Conditions: diaryltelluride (0.1 mmol), carboxylic acid (0.22 mmol), TPP (0.01 mol%), and CH2Cl2 (10 mL) under aerobic conditions at room temperature. |
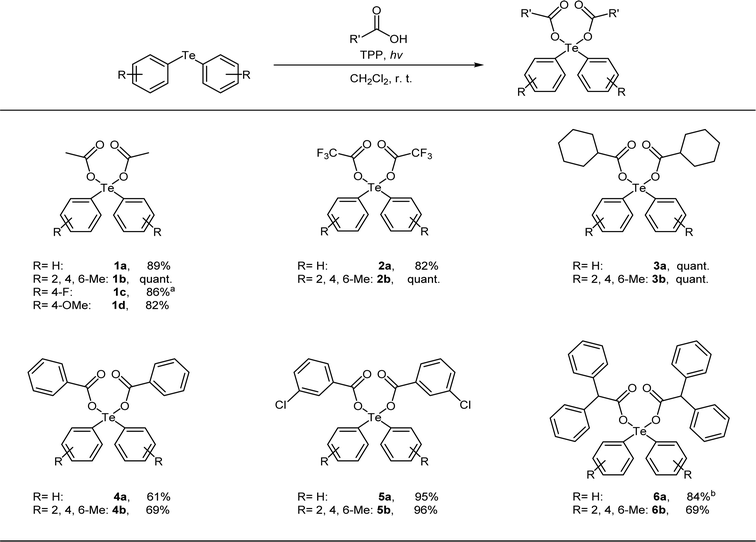 |
The reaction is thought to produce the diaryltellurium dicarboxylates after the generation of the telluroxides via photooxidation (Scheme 1). First, triplet oxygen is converted to singlet oxygen using the photosensitizer in the presence of light. Singlet-oxygen oxidation of the telluride then gives the corresponding telluroxide, a reaction that has already been reported.10 The telluroxide then reacts with the carboxylic acid to produce the corresponding tellurium dicarboxylate and water. To investigate this proposed mechanism further, we reacted diphenyltelluroxide with 2 equivalents of acetic acid under mild conditions, which furnished the tellurium dicarboxylate diphenyl-tellanediyl diacetate in quantitative yield.
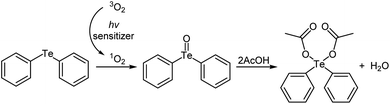 |
| Scheme 1 Multistep synthesis of diaryltellurium dicarboxilates | |
Single-crystal-X-ray diffraction analysis
Colorless crystals of 1a–1d, 2b and 6a, suitable for single-crystal X-ray diffraction analysis, were collected following the slow diffusion of n-hexane into chloroform solutions of 1a–1d, 2b and 6a at room temperature. Some of the structures of these diaryl tellurium dicarboxylates were determined using X-ray crystallographic analysis. The molecular structure of bis(2,4,6-trimethylphenyl) tellurium diacetate 1b is shown in Fig. 1. Selected bond distances and angles for 1b are summarized in the caption of Fig. 1. The acetoxy groups are located in apical positions and the aryl groups are located at equatorial positions. The presence of the lone pair at the third equatorial position is inferred by the reduction of the equatorial C–Te–C and apical O–Te–O bond angles which are 111.17(8)° and 164.43(6)°, respectively. The observed Te–O (2.1388(14), 2.1565(14) Å) and Te–C (2.133(2), 2.1368(19) Å) bond lengths are in good agreement with those of analogous diaryl tellurium dicarboxylates.11 The selected bond lengths and bond angles for 1a–1b, 2b and 6a are given in Table 3. The central Te core of all four structures is very similar as are the structures of all the diaryltellurium dicarboxylates shown in this article. The major difference between these compounds is the bond angle between the substituents located in the equatorial position. The compounds with bulky aryl groups (1b and 2b: Mes) have a slightly wider C1–Te–C2 angle (1b: 111.2°; 2b: 111.4°) than the other compounds. On the other hand, differences in the other aryl groups have little effect on the bond angle between the substituents located in the equatorial position.
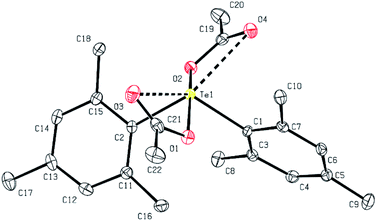 |
| Fig. 1 Structure of bis(2,4,6-trimethylphenyl) tellurium diacetate 1b thermal ellipsoids at 60% probability level. Selected bond distances (Å) and angles (°): Te1–C1, 2.133(2); Te1–C2, 2.1368(19); Te1–O1, 2.1388(14); Te1–O2, 2.1565(14); Te1⋯O3, 2.9561(17); Te1⋯O4, 2.9951(15); O1–Te1–O2, 164.43(6); C1–Te1–C2, 111.17(8); C1–Te1–O1, 84.15(7); C2–Te1–O1, 86.46(7); C1–Te1–O2, 88.19(7); C2–Te1–O2, 83.69(6); O3⋯Te1⋯O4, 136.47(5). | |
Table 3 Comparison of the relevant Te bond lengths and angle
Bond length (Å) or angle (°) |
1a |
1b |
1c |
1d |
1d |
2b |
6a |
Te–O1 length (shoter) |
2.164 |
2.139 |
2.152 |
2.143 |
2.168 |
2.154 |
2.158 |
Te–O2 length (longer) |
2.164 |
2.157 |
2.152 |
2.177 |
2.173 |
2.174 |
2.158 |
Te–C1 length (shoter) |
2.124 |
2.133 |
2.098 |
2.093 |
2.080 |
2.125 |
2.097 |
Te–C2 length (longer) |
2.124 |
2.137 |
2.098 |
2.095 |
2.106 |
2.126 |
2.097 |
O1–Te–O2 angle |
167.0 |
164.4 |
168.0 |
164.1 |
169.0 |
165.9 |
163.5 |
C1–Te–C2 angle |
93.6 |
111.2 |
95.7 |
99.4 |
99.9 |
111.4 |
100.2 |
Applications in oxidation reactions
As a exemplary applications for the obtained diaryltellurium dicarboxylates, we examined the oxidation of benzoin to benzil. The oxidative transformation of benzoin to benzil moieties has been accomplished using a wide variety of reagents or catalysts and different reaction procedures. Several reagents have been used for this transformation, including nitric acid, bismuth(III) nitrate-copper(II) acetate12 and Y(III) nitrate bismuth(III) nitrate-copper(II) acetate.13 The practical utility of these reagents is limited by the requirement for stoichiometric amounts of corrosive acids or high reaction temperatures. On the other hand, reports on oxidation reactions using diaryltellurium dicarboxylates remain elusive.
We investigated a model oxidation reaction of benzoin to benzil using various diaryltellurium dicarboxylates. For that purpose, CH2Cl2 solutions of benzoin and diaryltellurium dicarboxylates were stirred for 24 h in an open flask at room temperature. After the reaction, benzil was isolated by column chromatography; yields are summarized in Table 4. The activity of the diaryltellurium dicarboxylates with trifluoroacetates (2a and 2b) was higher than that of the other diaryltellurium dicarboxylates (Table 4, entries 5 and 6).
Table 4 Oxidation of benzoin to benzil using diaryltellurium dicarboxylatesa
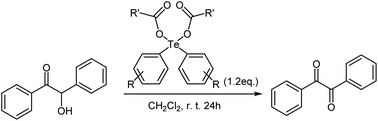
|
Entry |
Diaryltellurium dicarboxylates |
Yieldb (%) |
Conditions: benzoin (0.25 mmol) diaryltellurium dicarboxylates (0.3 mmol) and CH2Cl2 (3 mL), under aerobic conditions at room temperature for 24. Isolated yield. |
1 |
1a |
19 |
2 |
1b |
9 |
3 |
1c |
21 |
4 |
1d |
8 |
5 |
2a |
97 |
6 |
2b |
99 |
Subsequently, we investigated the scope of applicable benzoin derivatives under the previously established optimal reaction conditions and the results are summarized in Table 4. Overall, the reactions proceeded rapidly and furnished the expected oxide in good to excellent yield (Table 5, entries 1–5). This oxidation reaction, based on diaryltellurium dicarboxylates, proceeds under mild conditions and does not require the use of acids or bases.
Table 5 Substrate scope of the oxidation reaction using diaryltellurium dicarboxylatesa
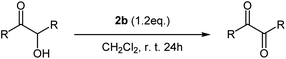
|
Entry |
R |
Yieldb (%) |
Conditions: benzoin (0.25 mmol) diaryltellurium dicarboxylates (0.3 mmol) and CH2Cl2 (3 mL), under aerobic conditions at room temperature for 24. Isolated yield. |
1 |
Phenyl |
97 |
2 |
n-Butyl |
96 |
3 |
4-Methoxyphenyl |
Quant. |
4 |
4-Methylphenyl |
91 |
5 |
2-Furan |
98 |
Conclusions
We have developed a one-pot synthesis of a series of diaryl tellurium dicarboxylates under safe and mild reaction conditions using atmospheric oxygen and inexpensive carboxylic acids. Furthermore, we have structurally characterized multiple diaryl tellurium dicarboxylates, and we discovered that these diaryltellurium dicaboxylates can be used to oxidaze benzoin under mild reaction conditions. The findings presented herein can be expected to lead to new research areas that employ diaryl tellurium dicarboxylates in the future.
Conflicts of interest
There are no conflicts to declare.
Notes and references
- S. Koguchi, Y. Shibuya, Y. Igarashi and H. Takemura, Synlett, 2019, 30, 99 CrossRef CAS.
- M. Oba, K. Nishiyama, S. Koguchi, S. Shimada and W. Ando, Organometallics, 2013, 32, 6620 CrossRef CAS.
- A. Mihoya, Y. Shibuya, A. Ito, A. Toyoda, M. Oba and S. Koguchi, Synlett, 2020, 31, 2043 CrossRef CAS.
- A. Mihoya, S. Koguchi, Y. Shibuya, M. Mimura and M. Oba, Catalysts, 2020, 10, 398 CrossRef CAS.
- Z. Liu and Z. Chen, Heteroat. Chem., 1992, 3, 559 CrossRef CAS.
- T. Kataoka and S. Watanabe, Sci. Synth., 2007, 31, 1159 Search PubMed.
- V. Chandrasekhar, A. Kumar, M. D. Pandey and R. K. Metre, Polyhedron, 2013, 52, 1362 CrossRef CAS.
- V. Chandrasekhar and R. Thirumoorthi, Eur. J. Inorg. Chem., 2008, 4578 CrossRef CAS.
- K. Kobayashi, H. Izawa, K. Yamaguchi, E. Hornc and N. Furukawa, Chem. Commun., 2001, 1428 RSC.
- M. Oba, M. Endo, K. Nishiyama, A. Ouchi and W. Ando, Chem. Commun., 2004, 1672 RSC; M. Oba, Y. Okada, K. Nishiyama, S. Shimada and W. Ando, Chem. Commun., 2008, 537 Search PubMed; M. Oba, Y. Okada, K. Nishiyama and W. Ando, Org. Lett., 2009, 11, 1879 CrossRef CAS PubMed; Y. Okada, M. Oba, A. Arai, K. Tanaka, K. Nishiyama and W. Ando, Inorg. Chem., 2010, 49, 383 CrossRef PubMed.
- V. Chandrasekhar and A. Kumar, J. Organomet. Chem., 2009, 694, 2628 CrossRef CAS.
- S. Tymonko, B. Nattier and R. Mohan, Tetrahedron Lett., 1999, 40, 765 CrossRef.
- A. McKillop, B. Swann, M. Ford and E. Taylor, J. Am. Chem. Soc., 1973, 95, 3641 CrossRef CAS.
|
This journal is © The Royal Society of Chemistry 2021 |
Click here to see how this site uses Cookies. View our privacy policy here.