DOI:
10.1039/D0SM02004D
(Communication)
Soft Matter, 2021,
17, 10-15
Recharging N95 masks using a van de Graaff generator for safe recycling†
Received
11th November 2020
, Accepted 24th November 2020
First published on 17th December 2020
Abstract
N95 respirators, used in the current COVID-19 pandemic, filter virus-containing aerosols using the static electricity of melt-blown polypropylene sheets. Their shortage at hospitals demands their recycling, but the standard sterilization methods, including alcohol spraying, washing, autoclaving, and heating in hot water, cannot be easily implemented because they compromise the electrostatic charges and thus their filtering effect. We report that a van de Graaff generator, commonly used for the demonstration of static electricity, can be used as a safe, cheap and quick method to recover the polypropylene electric charges that are lost during sterilization processes. We will show that this recharge also restores the masks’ filtering function.
The N95 respirator is a mask that health caretakers use at hospitals for taking care of COVID-19 patients. The classification “N95” shows that it filters out at least 95% of airborne particles, certified by the U.S. National Institute for Occupational Safety and Health (equivalent to FFP2 by European Union and DS2 from Japan) despite the fact that its pore size (∼10 μm) is larger than small aerosols (<1 μm). This is thanks to the static electricity of the melt-blown polypropylene fabric used in the mask,1–5 which captures small aerosols by electrostatic forces as most aerosols are electrically charged. Any contact with water, such as prolonged exposure to exhaling breaths or storage in humid places, could potentially remove the static electricity and lower its filtering efficiency. This is why these masks are, in a normal situation, for one time use for limited durations. However, during the pandemic, the pressure for recycling has surged at hospitals due to their shortage in supply,6 but the standard sterilization methods, including alcohol spraying, washing, autoclaving, and heating in hot water, cannot be easily implemented because they could affect the electrostatic charges and thus their filtering effect. In response to this challenge, scientific communities have proposed different methods to safely reuse masks.7–9 Ultraviolet radiation was employed at the University of Nebraska Medical Center in Lincoln.10,11 Fumigation, where the masks are exposed to vaporized hydrogen peroxide, was used at Duke University Hospitals.12 Baking was examined at Stanford University.13,14 Steeping in hot water (typically 60–80 °C) or autoclaving, followed by drying with a hair dryer to restore the electrostatic charges, was tested at Beijing University of Chemical Technology.15,16 Although they have provided important guidance to tackle the issue, to fulfill all the criteria (easy, safe, perfect sterilization efficiency, restored static electricity and filtering function, reproducibility etc.) remains a challenge. Recently, a method to “recharge” static electricity of masks by applying 1 kV potential via a DC voltage generator was demonstrated.17 Their direct approach to restore the charge is probably more reproducible and reliable than drying with a hair dryer, however, the necessity of the high-voltage generator limits the use outside of labs because of its high cost, safety issues, and the relatively long operation time (60 min) for the recharge.
In this work, we report a safe, easy and cheap method to recharge N95 masks at ∼100 kV for 3 min via a van de Graaff generator. This recharge is quick thanks to the used two-orders-of magnitude higher voltage. A van de Graaff generator is an electrostatic generator commonly used for the demonstration of static electricity at science museums or in science classes that can produce over 100 kV. Such a high voltage, if generated by a standard DC volage generator, requires specialized safety measures to handle, whereas a van de Graaff generator can even be touched due to the extremely low current as the metal electrode is electrically floating. In addition, the price range of van de Graaff generators (∼1000 USD) is an order of magnitude cheaper than the standard DC voltage generator that can generate above 1 kV. By combining autoclaving or heating in hot water, we will show that N95 masks can be sterilized without losing their filtering effect.
The amplitude, polarity and reproducibility of polypropylene static electricity depends on the manufacturer
First, we measured the static electricity of N95 or DS2 grade masks from four manufacturers by a voltage static meter. All of the masks consisted of three layers, where polypropylene is sandwiched with two protective layers (Fig. 1a). Since the used non-contact voltmeter is sensitive to the distance between the sample surface and the sensor, a home-made wooden clamp was used to fix this distance at 1 cm for reproducible measurements (Fig. 1b). Static electricity was measured on six surfaces as indicated in Fig. 1a for five masks per manufacturer. The polypropylene layers in manufacturer 1 had electrostatic charges, yet their amplitude and polarity were almost random (Fig. 1c, manufacturer 1). The polypropylene layers in manufacturer 2 and 3 had reproducible negative electrostatic charges (Fig. 1c, manufacturer 2 and 3). The polypropylene layer in manufacturer 4 had weak and non-reproducible charges (Fig. 1c, manufacturer 4). These data suggested that the amount of electrostatic charge varies among manufacturer and individual masks. This may be partially because of the ways these masks were stored, since the charge distribution was different even from the same manufacturer. Since the static electricity on the polypropylene from manufacturer 2 was the most reproducible, we will perform the rest of the experiments mainly with these masks.
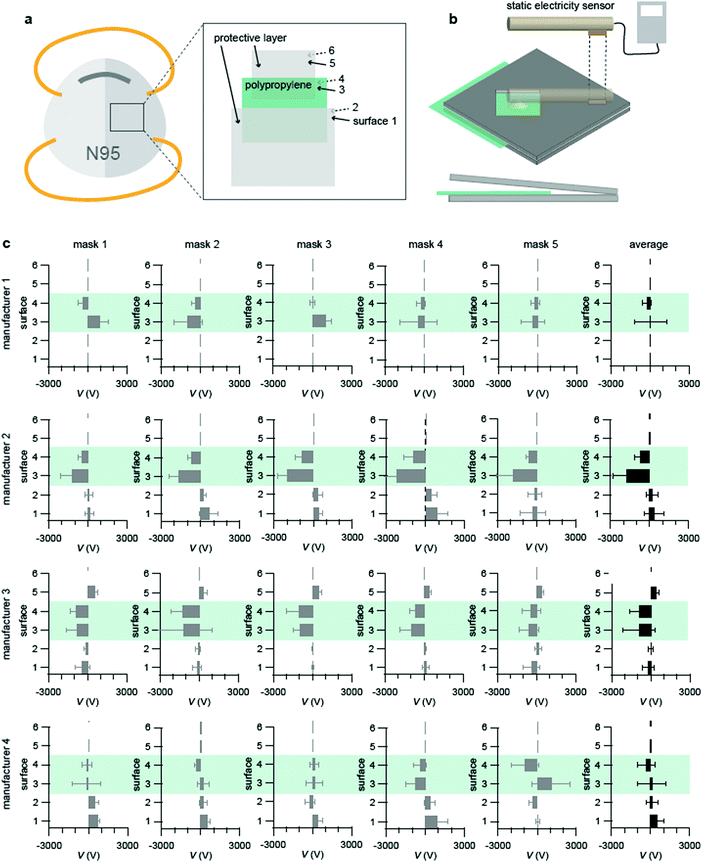 |
| Fig. 1 (a) A scheme of a N95 grade mask. (b) The setup of the voltage measurement. (c) Voltages measured at 6 surfaces indicated in (a). For each mask 10 locations were measured per surface and the averages and the standard deviations are shown. 5 masks were measured from 4 manufacturers. The “average” on the right side is the average of all of the 5 masks. | |
Washing with detergent or steeping in boiling water removed the static electricity of the masks
To study the effect of different sterilization techniques on the static electricity, the masks from manufacturer 2 were autoclaved, washed with house-hold detergent and bleach, or steeped in boiling water, and the resulting electrostatic charges were measured. Autoclaving one time reduced the static electricity by 54% for layer 4 and 51% for layer 3, respectively (Fig. 2a, autoclaved). These numbers are smaller than the ones, where only the polypropylene sheets (without the protective layers) were autoclaved once (60% for the layer 3 and 74% for the layer 4. Fig. 2a, autoclaved, “pp”), implying that the protective layers partially prevented the polypropylene from losing their charges. Additional autoclaving twice and three times further reduced the charges for layer 4 (Fig. 2a, autoclaved, “2 and 3”). Washing with detergent and color bleach in a washing machine diminished the charges by nearly 100% for both layers (Fig. 2a, washed with detergent). Heating in boiling water for 10 min also reduced the charges by 53% for layer 4 and 99% for layer 3 (Fig. 2a, heated in boiling water). Similar results were obtained with the masks from manufacturer 3 (Fig. S1, ESI†).
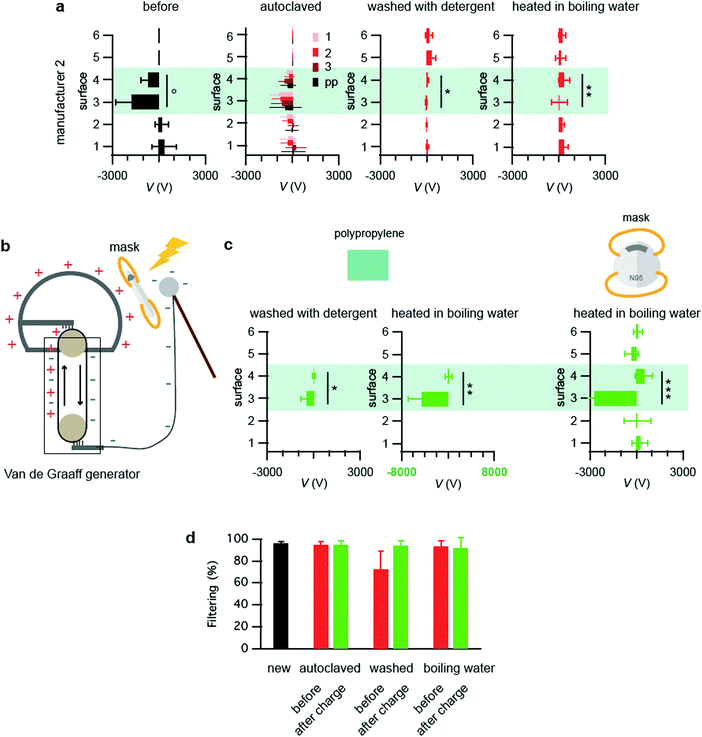 |
| Fig. 2 (a) Voltages from the masks’ static electricity measured before and after autoclaving, washing with detergent and color bleach, and steeping in boiling water. 10 locations were measured per surface, 3 masks were studied, and their average and the standard deviations are shown. (b) A scheme of a van de Graaff generator. (c) Voltages of the polypropylene and masks after being recharged. 10 locations were measured per surface, 3 polypropylene sheets or 3 masks were studied, and their average and the standard deviations are shown. (d) Filtering efficiency of the masks before and after sterilizing by different methods, and after recharging by a van de Graaff generator. 3 masks were tested per category and their averages and the standard deviations were plotted. | |
Van de Graaff generator recovers the static electricity in 3 min
To restore the static electricity that is reduced or lost during the sterilization procedures, these masks were recharged by a van de Graaff generator and the voltages were measured. A van de Graaff generator is an electrostatic generator, invented by Robert J. Van de Graaff around the 1930s.18 Positive and negative electrostatics formed by the triboelectric effect of the moving belt are stored in a large and a small metallic spherical shell (Fig. 2b). It is a cheap and safe way to generate up to 100 kV even with a table-top version, whereas a larger setup can create several MV. Applications of high voltages to polymers are known to inject electric charges, well-studied in the field of insulation materials for e.g. cable shielding.19,20 Although its exact mechanism is still under debate, trapping electrons or holes at defect sites or impurities causes these charges.21–23 A mask was attached to the larger metallic sphere of the van de Graaff generator and the smaller metallic sphere was hovered over the mask with a distance of several centimeter for 3 min, where corona discharge was occasionally observed. First as a control, only the polypropylene sheets without the protective layers were recharged via this method. The polypropylene after washing with detergent and color bleach recovered the charge back to 26% of the original charge (refer to Fig. 2a°, a* and c*). The polypropylene after steeping in hot water recovered the charge back to 255% (refer to Fig. 2a°, a** and c**. Note that the range of the x axis is different in Fig. 2c**). This suggests that the process before recharge affects the extent of the charge recovery. The polypropylene washed by detergent and color bleach recovered the charge less probably because house-hold detergents contain anti-static materials that remove charges and prevent them from reoccurring. In both cases, layer 4 did not recover charges significantly despite the fact that it was in the proximity to the larger metal sphere with positive charges, because polypropylene has a tendency to acquire negative charges by nature. Next, the entire masks were heated in hot water and recharged. Layer 3 regained the negative charge back to 153% of the original value, whereas layer 4 presented a slight positive voltage (refer to Fig. 2a°, a** and c***). This shows that the reduced charge by the sterilizing procedures could be restored via the van de Graaff generator.
Recharged masks after washing restored the filtering efficiency
Finally, as a first step to study the masks’ function after recharge, the filtering efficiency was measured by a mask fitting tester. A mask fitting tester measures the number of particles in and outside of masks by aspirating air for calculating the particle filtering efficiency by the following equation.
Masks were folded into two with one of the tubes inside, sealed with duct tape for eliminating leakages, and placed in a box with the other tube that measures the particles outside the mask. These masks were challenged by aerosols created by a humidifier that contains water with NaCl, which is also placed in the box. Particles in the range of 0.3–0.5 μm were counted for 20 second and the filtering efficiency was calculated. Note that the accuracy of this instrument is lower than the filter tester used in industry24 (e.g. Automated Filter Tester Model 8130A, TSI) and detects no particles below 0.3 μm. Therefore, the results presented here should be regarded as rather qualitative data. Brand new masks presented the filtering efficiency of 96% ± 1%, whereas those of three-times autoclaved, detergent-and-color-bleach washed, and hot-water steeped masks were 94% ± 3%, 72% ± 17%, 93% ± 5% respectively (Fig. 2d). Considering the error bars, the reduction in the filtering efficiency was detected only with the washed masks probably because the used instrument was not sensitive enough especially for smaller particles. Next, these masks were recharged by the van de Graaff generator and the filtering efficiency was studied again (Fig. 2d). The efficiency came back to 94% ± 4% for the washed masks. This result supports that the recharge by van de Graaff recovers the masks’ filtering efficiency.
In conclusion, we report that N95 grade masks can be sterilized and recharged by a van de Graaff generator. The filtering efficiency recovered after recharge, supporting the importance of the static electricity in their functions. Nevertheless, the use of new masks will always be preferable as we noticed the deformation of the masks during the sterilization procedures, which could hinder the suitable fitting of the masks to the face, thus inducing leakages. The demonstrated cheap, safe and quick method to recharge polypropylene sheets may be also applied for other filtering applications such as industrial and house-hold air purifiers.
Materials and methods
Masks
8210 (3M, USA), 8210J (3M Japan, JP), High-Luck (KOKEN, JP), and X-1702 (Vilene, JP) were purchased and used as delivered.
Static electricity measurements
Digital low voltage static meter KSD-3000 (Kasuga, JP) was combined with a home-made mask clamp. The clamp was made of wood, which holds little electrostatics by nature, to avoid any influence of the electrostatics of the clamp on the measurement. To measure different surfaces, masks were cut open and each layer was placed in the clamp to keep the distance to the sensor at 1 cm. The measurement was performed at room temperature (25 °C) at relative humidity of around 50%–60%. 10 locations were measured per surface and their average and the standard deviation are shown in figures.
Sterilizing procedures
Autoclaving was performed at 120 °C under 0.12 MPa for 10 min, while including the temperature ramp the total program was around 1 h. Washing was performed by house-hold detergent and color bleach in a washing machine with the standard program that takes around 1 h. Heating in hot water was performed by steeping masks in boiling water for 10 min. All of the masks were naturally dried and used for the experiments.
Recharging with a van de Graaff generator
The used van de Graaff generator (Gakurinsha Co., Ltd, JP) is equipped with a motor for moving the belt to cause the triboelectric effect. Masks were fixed to the larger metal shell with the bands of the masks, so that the face-side (layer 6) is in contact with the metal. The motor was run for 3 min, while the smaller metallic sphere was hovered over the mask with a distance of several cm. Corona discharge was occasionally observed.
Filtering efficiency measurement
Mask fitting tester MT-03 (Sibata, JP) is equipped with two tubes connected to an aspirator, where they count particles in and outside of masks for estimating the filtering efficiency. Masks were folded into two with a tube placed inside and sealed with duct tape, whereas the other tube was fixed nearby outside of the masks. These masks with tubes were placed in a box with a humidifier with salt water (150 mM) for simulating aerosols. The particle count was performed for 20 s with the particle size mode of 0.3 μm–0.5 μm. The first 3 measurements were abandoned as they could be influenced by the left-over particles inside the masks, and the following 3 measurements were averaged and presented in Fig. 2 with standard deviations.
Conflicts of interest
There are no conflicts to declare.
Acknowledgements
Part of the research leading to these results received funding from the FY 2020 University of Tokyo Excellent Young Researcher, the Female Faculty Startup Grant, the special fund of Institute of Industrial Science, the University of Tokyo, JSPS KAKENHI Grant Number JP20K22324, Inoue Science Research Award, the Naito Foundation and Kanamori Foundation. We thank Prof. Yoshiho Ikeuchi (The University of Tokyo) for the access to the autoclave and the workshop at Institute of Industrial Science, the University of Tokyo.
References
- H. Ding, Proc. SPIE, 1991, 1519, 847–856 CrossRef CAS.
- P. P. Tsai, H. Schreuder-Gibson and P. Gibson, J. Electrost., 2002, 54, 333–341 CrossRef CAS.
- R. L. Shambaugh, Ind. Eng. Chem. Res., 1988, 27, 2363–2372 CrossRef CAS.
- K. H. Lee, O. Ohsawa, K. Watanabe, I. S. Kim, S. R. Givens, B. Chase and J. F. Rabolt, Macromolecules, 2009, 42, 5215–5218 CrossRef CAS.
- A. Kilic, E. Shim and B. Pourdeyhimi, Aerosol Sci. Technol., 2015, 49, 666–673 CrossRef CAS.
- N. Sharma, Z. Hasan, A. Velayudhan, M. A. Emil, D. K. Mangal and S. D. Gupta, Chin. J. Health Manage., 2020, 22, 157–168 CrossRef.
- D. Mackenzie, Engineering, 2020, 6, 593–596 CrossRef CAS PubMed.
- P. S. C. Juang and P. Tsai, World J. Emerg. Med., 2020, 58, 817–820 CrossRef PubMed.
- J. Wang, L. J. Pan, S. Tang, J. S. Ji and X. M. Shi, Environ. Pollut., 2020, 266, 115099 CrossRef CAS PubMed.
-
G. Kolata, As coronavirus looms, mask shortage gives rise to promising approach [internet], New York Times, New York, 2020. Available from: https://www.nytimes.com/2020/03/20/health/coronavirusmasks-reuse.html.
-
J. L. Lowe, K. D. Paladino, J. D. Farke, K. Boulter, K. Cawcutt and M. Emodi, et al., N95 filtering facepiece respirator ultraviolet germicidal irradiation (UVGI) process for decontamination and reuse [Internet], University of Nebraska, Lincoln, 2020. Available from https://www.nebraskamed.com/sites/default/files/documents/covid-19/n-95-decon-process.pdf.
- A. Schwartz, M. Stiegel, N. Greeson, A. Vogel, W. Thomann and M. Brown,
et al., Decontamination and reuse of N95 respirators with hydrogen peroxide vapor to address worldwide personal protective equipment shortages during the SARS-CoV-2 (COVID-19) pandemic, Appl. Biosaf., 2020, 25(2), 67–70 CrossRef.
-
L. Liao, X. Wang, X. Yu, H. Wang, M. Zhao and Q. Wang, et al., Can N95 facial masks be used after disinfection? And for how many times? [Internet]. Stanford: LearnlyAnesthesia/Stanford AIM Lab COVID-19 Evidence Service, 2020. Available from: http://stanfordmedicine.app.box.com/v/covid19-PPE-1-2.
-
A. Price and L. F. Chu, Addressing COVID-19 face mask shortages (v 1.3), March 2020. [Internet]. Stanford: Learnly Anesthesia/Stanford AIM Lab COVID-19 Evidence Service, 2020. Available from: http://stanfordmedicine.app.box.com/v/covid19-PPE-1-2.
-
W. Cheng, Can’t buy mask? It can be reused after regeneration treatment! [Internet], ScienceNet, Beijing. Available from http://news.sciencenet.cn/htmlnews/2020/2/435754.shtm.
-
Z. Zhang and Y. Xing, New reusable face masks in production [Internet], Hong Kong, China, 2020. Available from: https://www.chinadailyhk.com/article/122864.
- E. Hossain, S. Bhadra, H. Jain, S. Das, A. Bhattacharya, S. Ghosh and D. Levine, Phys. Fluids, 2020, 32, 093304 CrossRef CAS PubMed.
- R. J. de Graaff, K. T. Compton and L. C. van Atta, Phys. Rev., 1933, 43, 149–157 CrossRef.
- Y. L. Chong, G. Chen, H. Miyake, K. Matsui, Y. Tanaka and T. Takada, J. Phys. D: Appl. Phys., 2006, 39, 1658–1666 CrossRef CAS.
- J. N. Marat-Mendes, R. M. Neagu and E. R. Neagu, J. Phys. D: Appl. Phys., 2004, 37, 343–347 CrossRef CAS.
- L. H. Catalani, G. Collins and M. Jaffe, Macromolecules, 2007, 40, 1693–1697 CrossRef CAS.
- G. Collins, J. Federici, Y. Imura and L. H. Catalani, J. Appl. Phys., 2012, 111, 044701 CrossRef.
- J. Lee and J. Kim, Polymers, 2020, 12, 721 CrossRef CAS PubMed.
- H. M. Xiao, J. Y. Gui, G. J. Chen and C. P. Xiao, J. Appl. Polym. Sci., 2015, 132, 42807 Search PubMed.
Footnote |
† Electronic supplementary information (ESI) available. See DOI: 10.1039/d0sm02004d |
|
This journal is © The Royal Society of Chemistry 2021 |
Click here to see how this site uses Cookies. View our privacy policy here.