DOI:
10.1039/D1FO01867A
(Review Article)
Food Funct., 2022,
13, 38-51
Epitope mapping and the effects of various factors on the immunoreactivity of main allergens in egg white
Received
13th June 2021
, Accepted 27th November 2021
First published on 29th November 2021
Abstract
Egg white has high protein content and numerous biological/functional properties. However, reported allergenicity for some of the proteins in egg white is an issue that needs to be paid exclusive attention. A consideration of the structure of IgE epitopes and their sequences, as well as a comprehensive understanding of the effects of various processes on epitopes and the impact of the gastrointestinal tract on them, can help target such issues. The current study focuses on the identified IgE epitopes in egg white proteins and evaluation of the effects of the gastrointestinal digestion, carbohydrate moiety, food matrix, microbial fermentation, recombinant allergen, heat treatment, Maillard reaction and combination of various processes and gastrointestinal digestion on egg white allergenicity. Although the gastrointestinal tract reduces the immunoreactivity of native egg white proteins, some of the IgE epitope-containing fragments remain intact during the digestion process. It has been found that the gastrointestinal tract can have both positive and negative impacts on the IgE binding activities of egg white proteins. Elimination of the carbohydrate moiety leads to a reduction in the immunoreactivity of ovalbumin. But, such effects from the carbohydrate parts in the IgE binding activity need to be explored further. In addition, the interaction between the egg white proteins and the food matrix leads to various effects from the gastrointestinal tract on the digestion of egg white proteins and their subsequent immunoreactivity. Further on this matter, studies have shown that both microbial fermentation and Maillard reaction can reduce the IgE binding activities of egg white proteins. Also, as an alternate approach, the thermal process can be used to treat the egg white proteins, which may result in the reduction or increase in their IgE binding activities depending on the conditions used in the process. Overall, based on the reported data, the allergenicity levels of egg white proteins can be mitigated or escalated depending on the conditions applied in the processing of the food products containing egg white. So far, no practical solutions have been reported to eliminate such allergenicity.
1. Introduction
An egg is known to be a well-balanced and nutritious food ingredient to be used in a variety of menus in human diets.1,2 Generally, egg white contributes to 60–63% of egg weight3 and contains numerous proteins components at different concentrations.4 However, quantitatively, ovalbumin (OVA) at 54% (w/w), ovotransferrin (OVT) at 12%, ovomucoid (OVM) at 11%, ovomucin (OVC) at 3.5% and lysozyme (LYS) at 3.5% are considered major proteins in the egg white.2 OVT is a glycoprotein whose peptides have been shown to have antioxidant, antimicrobial, iron transportation and anti-cancer properties inhibiting tumor growth in vitro.5,6 LYS is an antimicrobial agent and is used in the pharmaceutical industry.2 Several in vitro studies have shown that egg white protein isolates can also be used as antidiabetic agents.7 Egg white hydrolysates have also been reported to be effective for neuroprotection against neuropathic disorders (in the rats) induced by mercury.8
Despite the health benefits and functional properties mentioned for the egg white, reported allergenicity for its proteins is considered to be a major concern for its application in the food industry. The egg is the second most allergenic food after milk9 and one of the most common sources of food allergies among infants and children affecting up to 9% of children worldwide.10 Due to the occurrence of various egg products, it is difficult to entirely remove this commodity from the diet. As a consequence, unwanted use of egg products may cause serious dangers. In addition, egg allergy can also concurrently occur with other allergies from other food components, in which case, avoiding the consumption of these foods may result in the risk of a shortage in the nutritional compounds in the diet.11 Therefore, the production and development of egg products that are safe for allergic consumers are highly justifiable.
A part of protein known as epitope reacts with antibodies or receptors on T cells. IgE-binding epitopes in allergens are classified under two categories (sequential or linear and conformational). IgE identifies sequential epitopes by successive amino acids, while conformational epitopes are recognized by IgE due to amino acids that are lined up by a tertiary structure of the protein.12 Epitope mapping not only helps understand the mechanism and allergic potential of allergens but also helps develop the appropriate approach and method to reduce or eliminate food allergies.13
The destruction of sequential and conformational IgE epitopes by applying various processes is shown in Fig. 1. Of the major allergens reported for eggs, four are in egg whites, which include OVM, OVA, OVT and LYS. Protein YGP42 and α-livetin are present in the yolk. The current review focuses on the allergens in egg whites, since most of the allergens in eggs are found in egg white.14 Studies have reported on such treatments as caffeic acid cross-linking,15 irradiation,16 pulsed electric fields,17 heat,18,19 interactions between epigallo-catechin 3-gallate and allergens,20 high-intensity21 acid treatment,22 nitration,23 plastein reaction24 and proteolysis25,26 to reduce egg white allergenicity. In order to select appropriate methods to combat the allergenicity from egg white proteins, a correct understanding of the influential factors such as epitope mapping, epitope type (linear or conformational), the effect of process and gastrointestinal digestion has to be compiled and therefore the objectives of this study were to investigate the structure and amino acid sequences in IgE epitopes for the main allergens of egg white proteins and evaluate the effects of various processes and gastrointestinal digestion in this regard.
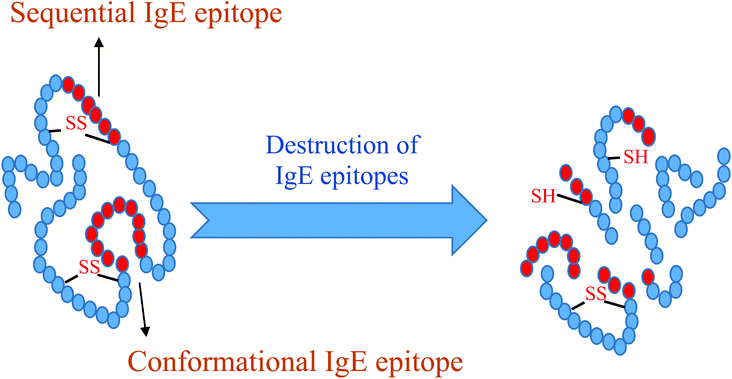 |
| Fig. 1 The schematic representation of the destruction in the sequential and conformational IgE epitopes applying any possible destruction process. | |
2. Sequence and structure of IgE epitopes
The following sections report on the findings related to epitopes and allergic properties of OVM, OVA, LYS and OVT. No studies have so far been reported on epitopes and allergic properties of OVC, which is suggested to be considered in future studies.
2.1. Ovalbumin
OVA is found at the highest content among the egg white proteins27 and with a molecular weight of ∼45 kDa, it has 385 amino acids, four thiol groups, one disulfide bond and an attached carbohydrate chain to asparagine 298 residue.27,28 Benedé et al.29 applied human and simulated gastrointestinal fluids for digestion of OVA and investigated the immunoreactivity levels of the produced peptides using human sera. One hundred and eighteen peptides were identified after the digestion of OVA by human sera. However, when simulated gastrointestinal fluids were applied, only 84 peptides were found in the hydrolysates. Among the identified peptides, 47 were found to be common for both media, among which seven peptides showed IgE binding properties. In another study, Mine and Rupa30 sequenced the IgE epitopes of OVA and identified five linear epitopes, some of which were exposed to the surface and had β-turn and β-sheet structures. Mine and Yang31 used the murine model for epitope mapping of OVA. The results showed that there were eight, two and five linear epitope regions for oral, intraperitoneal and subcutaneous entry routes, respectively. With oral sensitization, two epitopes were relatively hydrophobic and six epitopes were relatively hydrophilic. Among the amino acids in the epitope sequences, the highest levels were found for hydrophobic, polar and charged ones, including valine, alanine, phenylalanine, iso-leucine, serine and arginine amino acids. Table 1 shows some of the reported sequences for OVA linear IgE epitopes. Among the studies, the most frequent common sequences are 326–332, 375–384 and 323–332.
Table 1 Selected studies on the OVA linear IgE epitopes
Amino acid sequence |
Position |
Position in tertiary structure |
Secondary structure |
Method |
Ref. |
A and C represent alanine and cysteine; D and E represent aspartic and glutamic acids; F and G represent phenylalanine and glycine, respectively. H and M represent histidine and methionine; L, K and I represent leucine, lysine, and isoleucine, respectively. N, P and Q represent asparagine, proline and glutamine; R, S and T represent arginine, serine and threonine; V, W and Y represent valine, tryptophan and tyrosine, respectively. Not defined: ND. |
YRGGLEPINF |
125–134 |
ND |
ND |
Human |
29
|
ARELINSWVESQTN |
141–154 |
ND |
ND |
NSWVESQTNGIIRNVLQPSSVDSQTAMVLVN |
146–176 |
ND |
ND |
NVLQPSSVDSQTAM |
159–172 |
ND |
ND |
FKDEDTQAMPF |
188–198 |
ND |
ND |
AVHAAHAEINE |
326–336 |
ND |
ND |
HIATNAVLFFGRCVSP |
370–385 |
ND |
ND |
|
LAMVYLGAKDST |
38–49 |
Exposed to surface |
α-Helix & β-turn |
Human |
30
|
DVYSFSLA |
95–102 |
Exposed to surface |
β-Sheet & random |
EDTQAMPFRV |
191–200 |
Hidden in structure |
β-Sheet & β-turn |
VLLPDE |
243–248 |
Exposed to surface |
β-Turn |
GLEQLESIIN |
251–260 |
Exposed to surface |
β-Sheet & β-turn |
|
INKVVRFD |
53–60 |
Exposed to surface |
α-Helix |
BALB/c |
31
|
VNVHSSLR |
77–84 |
ND |
α-Helix & β-turn |
SRLYAE |
103–108 |
Exposed to surface |
Random |
GGLEPINFQT |
127–136 |
Exposed to surface |
α-Helix |
ERKIKV |
275–280 |
ND |
β-Turn & α-helix |
GITDVF |
301–306 |
ND |
α-Helix |
ISQAVHAAHA |
323–332 |
Exposed to surface |
β-Turn & random |
AVLFFGRCVS |
375–384 |
Hidden in structure |
β-Sheet & β-turn |
|
ISQAVHAAHAEINEAGT |
323–339 |
ND |
ND |
BALB/c |
32
|
|
AGVDAASVSEEFRADHPFLF |
347–366 |
ND |
ND |
Human |
33
|
AGVDAASVSEEFRADHPFLFCIKHIATNAVLFFGRCVSP |
347–385 |
ND |
ND |
EFRADHPFLF |
357–366 |
ND |
ND |
EFRADHPFLFCIKHIATNAV |
357–376 |
ND |
ND |
CIKHIATNAVLFFGRCVSP |
367–385 |
ND |
ND |
|
ISQAVHAAHAEINEAGT |
323–339 |
ND |
ND |
Murine model |
34
|
|
VYLGAKDSTRTQINKVVRFDKLPGFGDSIEAQCGTSVNVHSSLRDILNQITKPNDVYSFSLASRLYAEERYPILPEYLQCVKELYRGGLEPINFQTAADQARELINSWVESQTNGIIRNVLQPSSVDSQTAM |
41–172 |
ND |
ND |
Human |
35
|
GITDVFSSSANLSGISSAESLKISQAVHAAHAEINEAGTEVVGSAEAGVDAASVSEEFRADHPFLFCIKHIATNAVLFFGRCVSP |
301–385 |
ND |
ND |
2.2. Ovomucoid
OVM has been reported as the main allergen among egg white proteins.36 It has the highest content of carbohydrate (25%) in its structure, where the carbohydrate chains are attached to the protein moiety via asparagine.37,38 This protein has 186 amino acids, three domains (domain I is from residue 1 to 64, domain II from residue 65 to 130 and domain III from residue 131 to 186) and 9 disulfide bonds.3,39 There are three intra-domain disulfide bonds in each domain that leads to its heat or enzymatic stability.38,40 The tertiary structure of OVM is globular.41
Numerous studies have tried to identify the IgE epitopes of OVM. Martinez-Botas et al.42 used peptide microarray immunoassay for mapping sequential IgE epitopes. Among the 50 patients whose sera were investigated, 34% did not react with any sequential IgE peptides and 20% reacted with more than 10 sequential peptides. They introduced three epitopes in domains 1 and 2 as IgE epitopes that reacted with 26, 24 and 38% of the studied sera. Martinez-Botas et al.42 further reported that the efficacy of peptides with 20 amino acids was at higher levels than those with 15 amino acids. By altering the sequences of epitopes by replacing a new amino acid in each epitope, Mine and Zhang43 found three, four and two IgE epitopes in domains 1, 2 and 3, respectively. Altogether, 87 amino acids were present in 9 IgE epitopes of OVM. The IgE epitopes consisted of a higher proportion of charged hydrophobic and polar amino acids. Table 2 lists some related studies for IgE epitopes of OVM, where the most common sequences are 4–20, 51–56, 1–10, 101–104 and 121–124.42–46
Table 2 Reported linear IgE epitopes of OVM in human egg-allergic patients
Amino acid sequence |
Position |
Position in tertiary structure |
Method |
Ref. |
DCSRFPANTDKEGKDVL |
4–20 |
Domain 1 |
Human |
42
|
YSIEFGTNISKEHD |
46–59 |
Domain 1 |
FNPVCGTDGVTYDN |
91–104 |
Domain 2 |
|
TDGVTYTNDCL |
32–40 |
Domain 1 |
Human |
43
|
DCLLCAYSIEF |
40–50 |
Domain 1 |
KEHDGECKETV |
56–66 |
Domain 1 |
SSYAN |
71–75 |
Domain 2 |
DGKVMVLCNRA |
80–90 |
Domain 2 |
TYDNE |
101–105 |
Domain 2 |
KRHDGGCRKE |
121–131 |
Domain 2 |
KTYGNKCNFCNAVVES |
159–174 |
Domain 3 |
TLSHFGKC |
179–186 |
Domain 3 |
|
AEVDCSRFPN |
1–10 |
Domain 1 |
Human |
44
|
ATDKEGKDVL |
11–20 |
Domain 1 |
SIEFGTNISK |
47–56 |
Domain 1 |
VEQGASVDKRHD |
113–124 |
Domain 2 |
|
AEVDCSRFPANTDK |
1–14 |
Domain 1 |
Human |
45
|
NTDKEGKDVLVCNK |
11–24 |
Domain 1 |
GTDGVTYTNDCLLC |
31–44 |
Domain 1 |
GTNISKEHDGECKE |
51–64 |
Domain 1 |
ECKETVPMNCSSYA |
61–74 |
Domain 2 |
TYDNECLLCAHKVE |
101–114 |
Domain 2 |
KRHDGGCRKELAAV |
121–134 |
Domain 2 |
|
AEVDCSRFPANTDKEGKDVL |
1–20 |
Domain 1 |
Human |
46
|
FGTNISKE |
49–56 |
Domain 1 |
VLCNRAFNPVCG |
85–96 |
Domain 2 |
QGASVDKR |
115–122 |
Domain 2 |
NGTLTLSHFGKC |
175–186 |
Domain 3 |
2.3. Lysozyme
LYS is a monomeric enzyme with a molecular weight of 14.4 kDa and has 129 amino acid components and four disulfide bonds between cysteine amino acids (in positions 6–127, 30–115, 64–80 and 76–94).47–49 Two of the disulfide bonds in LYS are responsible for its high thermal resistance and the other two contribute to its enzymatic activity.3 Many studies have reported on the immunoreactivity of LYS but only a few have investigated structures and sequences of IgE epitopes in LYS. Jiménez-Saiz et al.49 investigated the type, immunoreactivity properties and biological activities of the derived peptides from simulated in vitro gastrointestinal digestion and reported that immunoreactivity of LYS is maintained due to the incomplete digestion of LYS in the stomach and intestine. They identified three IgE epitopes as follows in the LYS: 11–27, FLPLAALGKVFGRCELA; 57–83, NTQATNRNTDGSTDYGILQINSRWWCN and 108–122, ASVNCAKKIVSDGNG.
2.4. Ovotransferrin
OVT (conalbumin) is a glycoprotein with a molecular weight of ∼78 kDa containing 2.6% carbohydrates. It belongs to the family of iron-binding proteins with 686 amino acids and 15 disulfide bonds with no free sulfhydryl groups.50,51 The carbohydrate moiety in OVT consists of four residues of mannose and two residues of N-acetylglucosamine.52 The structure of OVT consists of an N lobe and C lobe.50 Each lobe has two domains and an iron-binding site.52 Generally, the secondary structure of OVT is composed of α-helix (17.9%), β-sheet (28.8%), β-turn (17.3%) and random coil (36.0%).53 So far, no experimental studies have been conducted to identify OVT IgE epitopes. However, Tong et al.53 used a web-based allergen database for predicting the IgE epitope mapping in OVT (Table 3).
Table 3 Predicted OVT IgE epitopes using a web-based allergen database in human
Amino acid sequence |
Position |
Amino acid sequence |
Position |
Method |
Ref. |
SSPEEK |
32–37 |
GVDTK |
294–298 |
Database |
53
|
NNLRD |
40–44 |
PVLKDL |
312–317 |
QQERISL |
47–53 |
KDEKSK |
371–376 |
TEGSTT |
104–109 |
VALDGGLV |
411–418 |
AVVKKGTEF |
115–123 |
TAGVC |
420–424 |
GKTSC |
130–134 |
PPNSRL |
512–517 |
TGLGRS |
136–141 |
GSGGI |
523–527 |
IESGSVE |
162–168 |
EKYFG |
538–542 |
GDPKTKC |
195–201 |
YRECNLA |
600–606 |
LKDGKG |
217–222 |
KANKIRD |
619–625 |
DQKDEY |
238–243 |
RFGVN |
633–637 |
SFLSK |
284–288 |
DLTKCL |
658–663 |
3. Relation between gastrointestinal digestion and the allergenicity of the protein
Protein digestion is dependent on several factors and varies from person to person. When passing through the gastrointestinal tract, proteins are exposed to different pH levels and several enzymes and as a consequence, the changes in the structure and sequence of the epitopes can influence the protein's allergenicity.54 For example, when food moves into the stomach, proteins are exposed to an acidic pH and undergo a certain level of denaturation and hydrolysis by the pepsin.54 Also, further down in the intestine, pancreatic enzymes (trypsin, chymotrypsin, carboxypeptidases A and B, enteropeptidases and elastase) can break down the proteins and change their allergenicity levels.55
According to Moreno,56 OVA is partially resistant to pepsin and gastrointestinal digestion. Intact OVA were detectable after 120 min of exposure to the stomach conditions [pH = 2.0, pepsin to substrate ratio of 1
:
20].57 However, unlike LYS, OVA can undergo duodenal digestion due to the bile salts.57 Duodenal digestion has a larger effect on the hydrolysis of OVA than gastric digestion but digestion by human gastric fluids leads to the production of shorter and less immunoreactive fragments compared to the simulated in vitro digestion.33 No major structural changes have been observed in the secondary structure of OVA due to the changes in the pH in the presence of bile salts and phosphatidylcholine but acidic pH can disrupt its tertiary structure.57 Generally, the IgE-binding activity of OVA is significantly reduced after gastrointestinal digestion, but due to the intact epitopes, some of its immunoreactivity properties are maintained.33,57,58
OVM is another important allergen found in egg white proteins. Among the three domains in OVM, the third one has more allergenicity levels than the other two.59–61 This protein is relatively resistant to heat and digestion by proteases.62 In a similar way, the antigenicity and allergenicity properties of OVM are resistant to heat and enzymatic digestion. Therefore, these properties are attributed to linear epitopes in OVM.62 The digestion rate of OVM in the stomach is partially dependent on the pepsin to protein ratio. The gastric digestion of this protein begins at the end of the first domain and then it is converted into smaller fragments.63 The produced fragments of pepsin digestion still show IgE-binding activity. Digestion with pepsin increases the sensitivity of OVM to trypsin and chymotrypsin but retains its trypsin inhibiting activity.64 Kovacs-Nolan et al.64 reported that the digestibility of OVM was increased by reducing S–S bridges, which further resulted in lower allergenicity levels.
Based on the reported studies,49 LYS hydrolysis can occur at both pH 1.2 and pH 2.0 conditions and produce peptides that are resistant to pepsin activity for at least two hours. At lower pH levels, LYS is hydrolyzed at a faster rate. In the stomach, the effect of pH on hydrolysis is higher than that of pepsin. The presence of bile salts can lead to the precipitation of LYS in the duodenum (due to the electrostatic interaction between the bile salts and LYS). On the other hand, phosphatidylcholine helps improve the partial solubility of LYS.49
According to Jiménez-Saiz et al.,65 the allergenicity of LYS was maintained even after gastrointestinal digestion. This was due to the incomplete hydrolysis of LYS in the gastrointestinal tract due to the precipitation with bile salts and also further due to the production of peptides with higher allergenicity levels.65 In a later study, Jiménez-Saiz et al.49 reported that even if the remaining LYS of gastric digestion were soluble in the absence of bile salts, the duodenal digestion on them was minimal and did not result in new or more abundant fragments. So far, no information has been provided on the effects of gastrointestinal digestion on allergenicity and allergenic epitopes of OVT and therefore such objectives should be considered in future studies.
4. The effect of the carbohydrate moiety on the allergenicity
Other than the proteins, some carbohydrates have been shown to be the sources of epitopes.66 Of the four major allergens in the egg white, three of them (OVA, OVM, OVT) are glycoproteins,67,68 which are types of compounds where proteins and carbohydrates are linked by covalent bonds.69 In this section, the effect of the carbohydrate moiety on the allergenicity of egg white protein is discussed. Studies show that the carbohydrate moiety in OVA has a significant contribution to its immunoreactivity.70,71 However, the terminal carbohydrate cleavage of N-glycan by N-acetylglucosaminidase reduces its IgE-binding activity.70,71 Hwang et al.70 reported a 40-fold reduction in the allergenicity of OVA by using N-acetylglucosaminidase extracted from Ascidian viscera. On the other hand, when using galactosidase and mannosidase enzymes, no changes were observed in the immunoreactivity of OVA.71 Investigating the secondary structure showed that the level of α-helix content increases after the deglycosylation, resulting in a possible decrease in the allergenicity of OVA.70
Considering the effects of carbohydrate part[s] on the allergenicity of OVM, two carbohydrate chains are attached to each of the domains 1 and 2 and one carbohydrate chain is attached to domain 3.72 In the study reported by Besler et al.,73 the deglycosylation of OVM was performed using a chemical process and the results showed no changes in immunoreactivity potency. It was concluded that the epitopes present in the protein structure can lead to immunoreactivity but the carbohydrate moiety does not have IgE-binding activity. Studies on the deglycosylated OVM (carried out by the effect of PNGase F enzyme) before and after the gastrointestinal digestion shows that before the digestion, 80% of the patients have fewer-allergic symptoms to the deglycosylated OVM, when compared to the intact OVM despite the fact that there were no apparent changes in the secondary and tertiary structures of OVM during the deglycosylation process.74 Therefore, it was concluded that some specific epitopes are located on the carbohydrate moiety of OVM.74 In the presence of α-amylase, both types of OVM were not affected by oral digestion. However, after the gastrointestinal digestion, the deglycosylated OVM was digested at higher levels than intact OVM and as a result, the produced fragments of the deglycosylated form indicated less allergic potential, which was related to the sensitivity of OVM to the proteolytic hydrolysis.74
5. Influence of food matrix on the allergenicity
An accurate assessment of the allergenic potential of foods can always help understand the allergenicity levels of proteins.75 To be active, allergens need to maintain their immunological forms during their stay in the entire digestive tract.76 The food matrix can impact the allergenicity levels of food proteins by interacting with allergens during a food process77 or by the digestion and absorption of food ingredients in the digestive tract.78 For example, chemical reactions (such as the Maillard reaction) between proteins and other food components can have a positive or negative impact on the allergenicity of proteins. Also, the formation of neoallergens during food processing can increase the allergenicity of the foods.78
Jiménez-Saiz et al.79 studied the effect of different types of polysaccharides on the simulated in vitro digestion and immunoreactivities of OVM and OVA. Before the digestion, the presence of polysaccharides (pectin, gum Arabic and xylan) in the protein solution did not show any differences in SDS-page bands of the proteins. But, the presence of a higher-molecular-weight protein resulted in some interactions between portions of proteins and polysaccharides in the mix samples. According to Jiménez-Saiz et al.,79 digestibility level of OVA was reduced in the presence of polysaccharides and such inhibitory effects from polysaccharides were at higher levels in the gastric digestion than in duodenal digestion. Regardless of the presence of polysaccharides, gastric and duodenal digestion can generally reduce allergic potencies of proteins. However, polysaccharides can increase the allergic potencies of OVA and OVM due to reduced digestibility by the formation of new complexes and/or enhancing the viscosity of stomach fluids.79 According to Wang et al.80 and Fraeye et al.,81 uronic acid, which is a part of gum Arabic and pectin structures, is responsible for anionic properties of these polysaccharides. In the duodenum, there is a possibility of the occurrence of electrostatic interactions between the positive regions of proteins and the negatively charged polysaccharides.79 As a subcategory of polysaccharides, pectin can also increase the viscosity of gastric fluids impacting the overall performance of proteins and their interactions with the surrounding environment.79
Martos et al.14 investigated the effect of egg yolk on digestibility and the immunoreactivity levels of egg white proteins and reported that at the end of the duodenal digestion, part of LYS, OVA and OVM remained intact in the absence of egg yolk and a large number of resistant fragments with a wide range of molecular weights were observed. According to Martos et al.,14 the highest levels of IgE binding activities were due to intact OVA and its four derived peptides as well as two fragments of LYS degradation. No peptides were found due to the OVM digestion. Although the presence of the egg yolk resulted in slight changes in the digestibility levels of egg white proteins, the amount of intact LYS was increased in the presence of egg yolk after duodenal digestion.14 Tong et al.82 reported that heat treatment (100 °C for 20 min) and egg addition (at 1
:
2 ratio, v/v) in custard formulations resulted in losses on allergenicity levels of the final product.
6. Effect of microbial fermentation on the allergenicity
Some studies have reported on the effects of bacterial fermentation on reducing the allergenicity levels of several food ingredients. Frias et al.83 showed that fermentation by Aspergillus oryzae led to a reduction in the allergic effects of soybean proteins. In a similar way, some bacteria from Lactobacillus and Streptococcus families have reportedly reduced the allergenic effects of milk, soybean and wheat.84–87 Li et al.88 fermented egg white using several strains of Lactobacillus and reported a reduction in the allergenicity due to L. delbrueckii, which was related to the possible cleavage of the OVM carbohydrate moiety. Similar results were reported by Li et al.89 when using Aspergillus oryzae (ATCC 16868).
7. Hypoallergenic egg white allergens
Studies have shown that the allergic potency can be changed by modifying the epitope sequences (e.g., by substituting an amino acid through a mutation process).90,91 One way to modify the sequence and also the structure of epitopes is to use a recombination process. Recombinant proteins are not entirely similar to their native analogues and some changes may occur in their structures.92 One of the important applications of recombinant allergens is their use in the treatment of some allergic diseases and skin prick.93,94 Several studies have reported on the food recombinant allergens such as soybeans, peanut, English walnut, hazelnut, wheat and fish.95 The recombinant allergens from egg white proteins are investigated in this section.
Dhanapala et al.96 produced four main egg white allergens using E. coli as an expression system. Three of these allergens (OVA, OVM and OVT) were IgE reactive and simulated the immune system but LYS lost its immunoreactivity properties. They did not provide a reason for reducing the immunoreactivity of LYS. Arita et al.97 used Saccharomyces cerevisiae AH 22 to produce two types of polymannosyl-attached LYS. They replaced glycine (position 49) with arsenic and also replaced arginine (position 21) with threonine to produce the potential N-linked glycosylation sites. The recombinant LYS had lower enzymatic activity than the natural ones. According to Arita et al.,97 the long polymannosyl chain (310 and 340 residues on 49 and 21 positions) resulted in the Steic's inhibition of the substrates around the enzyme's active sites. As a major improvement, the IgE- and IgG-binding of recombinant LYS were greatly suppressed. The carbohydrate chain could mask (hide) the epitopes due to its large and branched structure.
Mine et al.98 produced recombinant allergens from the third domain of OVM using E. coli as an expression system by four single-site mutations at glycine 32, phenylalanine 37, threonine 49, and lysine 55 and a double-site mutation at glycine 32 and phenylalanine 37. The data showed that the replacement of phenylalanine (position 37) with alanine and the simultaneous replacement of glycine (position 32) and phenylalanine (position 37) with methionine and alanine resulted in complete elimination of IgE and IgG binding but substitution of threonine at position 49, lysine at position 55, and glycine at position 32 did not indicate any changes in the immunoreactivity levels of the protein. Investigating the secondary structure of the protein showed that α-helix had decreased in the recombinant allergen when compared to the native protein. They suggested that phenylalanine (position 37), which is at the core of the α-helix structure, plays an important role in stimulating the immune system. But, glycine at position 32 (the core of the β-bend structure) shows a synergistic effect in the immunoreactivity of the third domain of OVM. Dhanapala et al.99 removed two disulfide bridges from 9 cysteine–cysteine bonds in the structure of OVM by replacing alanine at cysteine 192 and cysteine 210 using site-directed mutagenesis. The recombinant allergen showed less immunoreactivity than did the wild type. As Dhanapala et al.99 concluded, the structure and S–S bonds play a major role in the allergic reactivity of OVM.
Rupa and Mine100 produced recombinant OVA using Escherichia coli. The IgE- and IgG-binding activities of the recombinant OVA were higher in some of the sera samples from the patients when compared to the native protein. They attributed the increased immunoreactivity of recombinant OVA to the changes in the structure of the protein as the recombinant variant had a lower α-helix and β-structure, which was also less compacted.
8. Changes in the allergenicity due to the Maillard reaction
The Maillard reaction is a common phenomenon occurring in some foods containing both protein and carbohydrates when they are exposed to heat. A series of rearrangements such as condensation, oxidation and hydration (and dehydration) are taking place during this reaction.101,102 As a result, the allergenicity levels of proteins can change through the formation of new epitopes or destruction of the existing epitope(s) when they undergo the above rearrangements.103,104
Rupa et al.105 showed that the mannosylated egg white (produced by a 3-day Maillard reaction at a protein/mannose ratio of 1
:
1 at 55 °C) in BALB/c mice decreased the levels of such parameters as specific IgG, IgE, histamine and mouse mast cell protease that are associated with the allergenicity and antigenicity. In a different study,97 it was reported that the attachment of galactomannan to LYS by the Maillard reaction (at a protein to galactomannan ratio of 1
:
4, 60 °C and 65% relative humidity) leads to a reduction in the allergenicity and increase in the antigenicity.
Ma et al.106 investigated effects of various parameters (temperature: 60–100 °C; time: 10–120 min; pH: 2.0–10.0; glucose/lysine ratio: 1–100 and OVA concentration at 4.00, 2.40, 0.40, 0.24 and 0.04 mg mL−1) on the reduction of immunoreactivity of OVA. The results showed that depending on the conditions applied, the Maillard reaction can impose a positive or negative impact on IgE- and IgG-binding capacities of the proteins studied. According to this study, the temperature was the most effective parameter in reducing IgE-binding due to the Maillard reaction and, under optimal conditions, IgE- and IgG-binding can be reduced by 62 and 64%, respectively. Ma et al.106 reported that the immunoreactivity of OVA can be reduced by adjusting the conditions applied to the Maillard reaction. Studies have been reported on lysine 227 and lysine 323 as two reaction sites for the glycation of OVA with ribose leading to a reduction in the immunoreactivity of OVA due to masking of IgE and IgG epitopes.107
9. The effect of thermal processing on the allergenicity
Heat treatment is a conventional approach in food processing applied to reduce microbial load, which increases the shelf life and improves the quality of food products.108 However, proteins can undergo some changes due to the applied heat. Depending on the temperature, process time, structural properties of the proteins, environmental variables (such as moisture, and pH), the type and extent of changes are different.109 Thermal process can also impact the susceptibility levels of the proteins to gastrointestinal digestion, which ultimately leads to changes in the allergenicity levels.110
Stanciuc et al.111 investigated the impact of heat treatment (for 20 min at 80 or 100 °C) at various pH levels (4.5, 7.0 and 9.5) on the structure and antigenicity of OVM. The highest levels of hydrophobic groups were found at pH 7.0. But, the highest structural compression was observed at pH 9.5. However, such thermal treatments did not result in significant changes in the antigenicity of OVM at pH 4.5 and 7.0. However, when pH 9.5 was applied, a significant decrease (91%) was observed in the antigenicity of OVM.
According to Claude et al.,112 heating at 80 °C for 6 h under opposite electrostatic conditions can lead to the formation of small or large aggregates of OVA. The results showed that small aggregates had less allergic potency than large ones.112 Relatively similar results were reported by Claude et al.,113 who showed that the production of large aggregates of OVA by heat treatment reduces IgE- and IgG4-binding ability in humans and IgE- and IgG1,2a-binding ability in the mouse compared with the native OVA. They stated that the applied conditions (OVA solutions: 15 mg mL−1 in 0.8 M NaCl and pH 5.0 for 6 h at 80 °C) did not eliminate all IgE-binding epitopes but led to the loss or inaccessibility of some of them.113
Using the egg-allergic patients’ sera, Tong et al.11 examined the effects of thermal processing on the structure and allergenicity of OVT. The heat treatment was performed at 55–100 °C for 5–60 min at pH 7.3. The antigenicity was increased by heating at low temperatures (50, 60 and 70 °C for 5 min). Studying the secondary structure of heated OVT at 55 °C for 30 min showed that changes in the α-helix, β-sheets, β-turn and random coils lead to protein unfolding and expose some of the hidden epitopes to the outside environment. There were no major differences between the structures of the heated OVT (at 60 °C) and that of the native protein. However, the structural changes in the protein were at higher levels when heated at 80 °C and the number of sulfidryl groups was increased due to the cleavage of disulfide bonds.11 Consequently, the destruction of the structure due to the breakdown of disulfide bonds decreased the antigenicity level of OVA. Also, depending on the individuals, different levels of allergenicity were obtained for different patients.11
10. Simultaneous effects of various processes and gastrointestinal digestion on the allergenicity
As discussed in section 3 of this article, one of the most important factors in determining the allergenicity of egg white proteins is the possibility of changes in allergens during gastrointestinal digestion.54 It was found that allergenicity levels for proteins can be reduced using such methods as pulsed electric fields, which result in masking the allergen epitopes in the inner parts of aggregates.17 However, aggregates of proteins can be unfolded by various digestive enzymes in the gastrointestinal tract resulting in the higher exposure of its epitopes to IgE and an increase in the allergenicity, which is an adverse effect against what a process may have done. This section reviews the studies published on the effects of various processes in combination with gastrointestinal digestion on allergic potential.
Claude et al.19 applied two OVA aggregates at 50 nm and 65 μm sizes and two short and two long methods to investigate the gastric, intestinal and jejunal digestion. The immunoreactivity effects of the samples being studied were completely different. The native OVA showed the highest IgE binding activity, but the smaller aggregate showed less IgE binding activity. On the other hand, the impact of the gastric phase was higher on the aggregates than on the native OVA. But, the native OVA was more hydrolyzed at the intestinal phase. Jejunal digestion did not have significant impacts on the native OVA in the two methods under study but increased the degree of digestion of aggregates, especially after 5 h. Overall, the gastrointestinal phase led to the production of large peptides from the native OVA, which limited more hydrolysis in the jejunal phase. The results showed a positive effect of the thermal process on digestibility but the correlation between digestibility and IgE-binding capacity was opposite.19 These findings are in good agreement with other published studies on this matter. Jiménez-Saiz et al.114 reported that native OVA maintains ∼30% of its IgE-binding activity after duodenal digestion but OVA treated at 90 °C for 15 min showed a higher reduction in immunoreactivity when compared to the native one due to the higher susceptibility to gastrointestinal digestion. Fushiki et al.115 reported that mild heat treatment or acid denaturation can increase the effect of pepsin and pancreatic digestion on OVA. But, native OVA was hardly affected by such enzymatic treatments. In a similar study, Takagi et al.58 reported that 5 min heat pre-treatment at 100 °C could result in more effective hydrolysis of OVA in the gastrointestinal tract. However, milder heat treatments (60 °C for 30 min or 90 °C for 15 min) have not shown any major effect on the digestibility of OVM. Treatment at 90 °C for 15 min resulted in ∼50% reduction in the allergenicity of OVA.114
Lechevalier et al.18 investigated the effects of dry heat treatment (60–90 °C for 1–10 days) on immunoreactivity and the functional properties of egg white powder. A moderate dry heat treatment led to more protein digestion due to the unfolding or more flexibility of the protein structure, whereas severe dry heat treatment led to more resistance to digestion due to protein aggregation. Also, dry heat can impact the antigenicity of proteins through changes in their structures.18 According to Lechevalier et al.,18 greatest impacts on the antigenicity were observed when a severe thermal process was applied. Such effects were different for different proteins. For example, antigenicity for LYS was decreased and IgG-binding for OVT was increased while OVM was not affected by such processes.
The Maillard reaction at 50 °C for 96 h for a protein/glucose ratio of 20
:
1 (w/w) did not indicate any impact on the gastrointestinal digestibility of OVM but reduced digestibility of OVA, especially in the gastric phase. In general, the Maillard reaction exhibits adverse effects on the immunoreactivity of OVA and OVM after digestion.114 According to Jiménez-Saiz et al.,114 the immunoreactivity of OVM was increased by the formation of a new epitope or glucose reaction with IgE but that of OVA was reduced due to irreversible changes in the protein structure and the destruction of structural epitopes.
Ma et al.116 examined the effect of transglutaminase-mediated crosslinking on IgE binding of OVA. The samples previously subjected to high pressure were more affected by the enzyme. Simultaneous or sequential enzymatic treatment and high pressure resulted in the formation of high-molecular-weight polymers.116 In addition to new bonds formed by the enzyme, the new disulfide bonds occurring after high-pressure treatment contributed to the production of such polymers.116 Results showed that the digestibility and allergenicity levels of the polymers did not show significant differences with those of native proteins. But, minor changes were found in the digestibility of OVA. Table 4 lists some physical and chemical methods that can impact the allergenicity of egg white proteins.
Table 4 Studies on physical and chemical methods related to allergenicity levels of egg white proteins
Protein |
Processing method |
Results |
Ref. |
Egg white |
Irradiation with UV (0.0–29.1 W m−2) for up to 30 min at 8 °C |
In all cases, a decrease in immunoreactivity was detected |
16
|
Acid treatment |
- Decreasing in IgE binding of OVA (at pH's 2.0 and 3.0) and IgG (at pH 2.0) |
22
|
- No difference in IgE and IgG binding of OVM and OVT |
Dynamic high-pressure microfluidization |
Dynamic high-pressure microfluidization treatment from 80–200 MPa significantly reduced the allergenicity of egg white proteins, especially at 120 MPa due to reduction in α-helix content. |
117
|
Double enzyme hydrolysis by pepsin and chymotrypsin |
IgE- and IgG-binding of peptide less than 3 kDa was significantly lower than native proteins |
118
|
Ovalbumin |
Covalent interaction between OVA and chlorogenic acid (CHA) |
OVA–CHA conjugates reduced the allergenicity capacity of OVA due to structural changes of protein and covering of IgE epitopes by CHA |
119
|
Polyphenol oxidase-mediated covalent cross-linking of OVA with caffeic acid |
Antigenicity and allergenicity of OVA considerably reduced after cross-linking |
120
|
Covalent interaction between OVA and quercetin |
In vivo study showed that conjugation with quercetin decreased the allergenicity and antigenicity of OVA |
121
|
Ovomucoid |
Nitration |
No effect |
23
|
Electrolysis |
The allergic action of OVM was reduced after electrolysis on both the anode and cathode sides due to S–S bond destruction |
122
|
Ovotransferrin |
Iron-chelation |
Iron-chalated OVA made the immune system less stimulated than iron-free OVA |
123
|
11. Considerations for future studies
1- LYS and OVT are among the major egg allergens with little information about sequences and structures of their epitopes. In order to select a proper process to remove allergenicity from egg white proteins, a complete understanding of the structures and sequences of allergic epitopes is needed. Therefore, more studies have to be conducted in this field on all egg white allergens, especially on LYS and OVT.
2- It should also be investigated whether the allergenicity reduction is associated with any possible structural changes of the epitopes.
3- Food matrix can impact the allergenicity levels of the proteins by affecting their gastrointestinal digestion behavior.77,78 Therefore, some studies are needed to identify possible effects of different types of food matrices on the allergen proteins during different food processes
4- A comprehensive study is needed to identify the effects of all steps of the digestive tract on the allergenicity levels of egg white proteins, especially that of OVM.
5- The fermentation process is an easy, inexpensive and practical approach that has shown good results in reducing the allergenicity activities of egg white proteins.89,90 Therefore, further studies on the effects of different bacterial and fungal species on allergenicity reduction should be considered.
6- Although various processes for the allergenicity reduction may cause some changes in the structure of the proteins, they may also impact their functional properties that need to be investigated.104 One ultimate goal for eliminating the allergenicity of egg white proteins is to use them in various applications without any concerns for allergic consumers.
12. Conclusion
In order to produce diverse products from egg white, elimination/mitigation of allergenicity from this commodity has to be evaluated. This review considered investigations on the changes in the structures and sequences of IgE epitopes from the main allergens in egg white and the effect of various factors on allergenicity levels of egg white proteins. According to the studies, although different processes may result in reducing the allergenicity levels of egg white proteins, so far, not a single procedure has been proposed to eliminate the allergenicity in egg white proteins. Regardless of that, both positive and negative effects of such processes have been reported on structural changes in the allergens during gastrointestinal digestion. Such changes in the structure can in turn increase or decrease the allergenicity level of the product. Therefore, optimizations of processes may lead to reducing allergenicity levels of egg white proteins. But, studies are needed to investigate the quality, safety, functional and biological properties of proteins after such changes.
Author contributions
This review article has been jointly prepared by Behzad Gazme, a Ph.D. student at the University of Tehran, and his supervisory committee, Karamatollah Rezaei from the University of Tehran, Iran, and Chibuike C. Udenigwe from the University of Ottawa, Canada.
Conflicts of interest
The authors declare no conflict of interest.
Acknowledgements
The authors would like to acknowledge the assistance provided by the Research Council of the College of Agriculture and Natural Resources at the University of Tehran (grant number: 7106016/6/40) as well as those obtained from Natural Sciences and Research Council of Canada (NSERC: RGPIN-2018-06839) and the University of Ottawa Faculty of Health Sciences.
References
- D. J. McNamara, The fifty year rehabilitation of the egg, Nutrients, 2015, 7, 8716–8722 CrossRef PubMed.
- J. M. Miranda, X. Anton, C. Redondo-Valbuena, P. Roca-Saavedra, J. A. Rodriguez, A. Lamas, C. M. Franco and A. Cepeda, Egg and egg-derived foods: effects on human health and use as functional foods, Nutrients, 2015, 7, 706–729 CrossRef PubMed.
- Y. Zhu, S. K. Vanga, J. Wang and V. Raghavan, Impact of food processing on the structural and allergenic properties of egg white, Trends Food Sci. Technol., 2018, 78, 188–196 CrossRef CAS.
- J. Wang, Y. Liang, D. A. Omana, N. N. Kav and J. Wu, Proteomics analysis of egg white proteins from different egg varieties, J. Agric. Food Chem., 2011, 60, 272–282 CrossRef PubMed.
- E. Abeyrathne, H. Lee and D. Ahn, Egg white proteins and their potential use in food processing or as nutraceutical and pharmaceutical agents—a review, Poult. Sci., 2013, 92, 3292–3299 CrossRef CAS PubMed.
- F. F. Machado, J. S. Coimbra, E. E. G. Rojas, L. A. Minim, F. C. Oliveira and S. S. Rita de Cássia, Solubility and density of egg white proteins: Effect of pH and saline concentration, LWT–Food Sci. Technol., 2007, 40, 1304–1307 CrossRef.
- B. Gazme, K. Rezaei and C. C. Udenigwe, Anti-diabetic properties of hydrolysates from egg white proteins using immobilized enzymes followed by in vitro gastrointestinal digestion, Appl. Food Biotechnol., 2020, 7, 235–249 CAS.
- D. A. Rizzetti, F. Fernandez, S. Moreno, J. A. U. Ocio, F. M. Peçanha, G. Vera, D. V. Vassallo, M. M. Castro and G. A. Wiggers, Egg white hydrolysate promotes neuroprotection for neuropathic disorders induced by chronic exposure to low concentrations of mercury, Brain Res., 2016, 1646, 482–489 CrossRef CAS PubMed.
- F. Jahandideh, S. Chakrabarti, K. Majumder, Q. Li, S. Panahi, J. S. Morton, S. T. Davidge and J. Wu, Egg white protein hydrolysate reduces blood pressure, improves vascular relaxation and modifies aortic angiotensin II receptors expression in spontaneously hypertensive rats, J. Funct. Foods, 2016, 27, 667–673 CrossRef CAS.
- J. Čelakovská, J. Bukač, R. Vaňková, J. Krejsek, I. Krcmova and C. Andrýs, Egg allergy in atopic dermatitis patients-analysis of results of specific extract IgE and specific IgE to molecular components. Bird-egg syndrome, Food Agric. Immunol., 2021, 32, 265–282 CrossRef.
- P. Tong, J. Gao, H. Chen, X. Li, Y. Zhang, S. Jian, H. Wichers, Z. Wu, A. Yang and F. Liu, Effect of heat treatment on the potential allergenicity and conformational structure of egg allergen ovotransferrin, Food Chem., 2012, 131, 603–610 CrossRef CAS.
- J. Lin and H. A. Sampson, The role of immunoglobulin E-binding epitopes in the characterization of food allergy, Curr. Opin. Allergy Clin. Immunol., 2009, 9, 357–363 CrossRef CAS PubMed.
- C. Liu and S. K. Sathe, Food allergen epitope mapping, J. Agric. Food Chem., 2018, 66, 7238–7248 CrossRef CAS PubMed.
- G. Martos, R. López-Fandiño and E. Molina, Immunoreactivity of hen egg allergens: influence on in vitro gastrointestinal digestion of the presence of other egg white proteins and of egg yolk, Food Chem., 2013, 136, 775–781 CrossRef CAS PubMed.
- P. Tong, S. Chen, J. Gao, X. Li, Z. Wu, A. Yang, J. Yuan and H. Chen, Caffeic acid-assisted cross-linking catalyzed by polyphenol oxidase decreases the allergenicity of ovalbumin in a Balb/c mouse model, Food Chem. Toxicol., 2018, 111, 275–283 CrossRef CAS PubMed.
- L. Manzocco and M. C. Nicoli, Macromolecular crowding affects protein photosensitivity: The case of egg white immunoreactivity, Food Chem., 2012, 132, 982–988 CrossRef CAS.
- W. Yang, Z. Tu, H. Wang, L. Zhang, Y. Gao, X. Li and M. Tian, Immunogenic and structural properties of ovalbumin treated by pulsed electric fields, Int. J. Food Prop., 2017, 20, S3164–S3176 CrossRef CAS.
- V. Lechevalier, C. Guérin-Dubiard, M. Anton, V. Beaumal, E. D. Briand, A. Gillard, Y. Le Gouar, N. Musikaphun, M. Pasco and D. Dupont, Effect of dry heat treatment of egg white powder on its functional, nutritional and allergenic properties, J. Food Eng., 2017, 195, 40–51 CrossRef CAS.
- M. Claude, R. Lupi, G. Picariello, M. Drouet, C. Larré, S. Denery-Papini and C. Brossard, Digestion differently affects the ability of native and thermally aggregated ovalbumin to trigger basophil activation, Food Res. Int., 2019, 118, 108–114 CrossRef CAS PubMed.
- J. Ognjenović, M. Stojadinović, M. Milčić, D. Apostolović, J. Vesić, I. Stambolić, M. Atanasković-Marković, M. Simonović and T. C. Velickovic, Interactions of epigallo-catechin 3-gallate and ovalbumin, the major allergen of egg white, Food Chem., 2014, 164, 36–43 CrossRef PubMed.
- W. H. Yang, Z. C. Tu, H. Wang, X. Li and M. Tian, High–intensity ultrasound enhances the immunoglobulin (Ig) G and IgE binding of ovalbumin, J. Sci. Food Agric., 2017, 97, 2714–2720 CrossRef CAS PubMed.
- J. O. Lee, D. Sung, S. H. Park, J. Lee, J. Kim, D. H. Shon, K. Ahn and Y. Han, Effect of acid treatment on allergenicity of peanut and egg, J. Sci. Food Agric., 2017, 97, 2116–2121 CrossRef CAS PubMed.
- S. C. Diesner, C. Schultz, C. Ackaert, G. J. Oostingh, A. Ondracek, C. Stremnitzer, J. Singer, D. Heiden, F. Roth-Walter and J. Fazekas, Nitration of β-lactoglobulin but not of ovomucoid enhances anaphylactic responses in food allergic mice, PLoS One, 2015, 10, e0126279 CrossRef PubMed.
- X. Sun, C. Acquah, B. Gazme, R. T. Boachie, I. D. Nwachukwu and C. C. Udenigwe, Mechanisms of plastein formation influence the IgE-binding activity of egg white protein hydrolysates after simulated static digestion, Food Chem., 2021, 345, 128783 CrossRef CAS PubMed.
- A. Yang, C. Long, J. Xia, P. Tong, Y. Cheng, Y. Wang and H. Chen, Enzymatic characterisation of the immobilised Alcalase to hydrolyse egg white protein for potential allergenicity reduction, J. Sci. Food Agric., 2017, 97, 199–206 CrossRef CAS PubMed.
- B. Gazme, K. Rezaei and C. C. Udenigwe, Effect of enzyme immobilization and in vitro digestion on the immune-reactivity and sequence of IgE epitopes in egg white proteins, Food Funct., 2020, 11, 6632–6642 RSC.
- M. M. Pereira, R. A. Cruz, M. R. Almeida, Á. S. Lima, J. A. Coutinho and M. G. Freire, Single-step purification of ovalbumin from egg white using aqueous biphasic systems, Process Biochem., 2016, 51, 781–791 CrossRef CAS PubMed.
- Z. Xiong, M. Zhang and M. Ma, Emulsifying properties of ovalbumin: Improvement and mechanism by phosphorylation in the presence of sodium tripolyphosphate, Food Hydrocolloids, 2016, 60, 29–37 CrossRef CAS.
- S. Benedé, I. N. López-Expósito, R. López-Fandiño and E. Molina, Identification of IgE-binding peptides in hen egg ovalbumin digested in vitro with human and simulated gastroduodenal fluids, J. Agric. Food Chem., 2013, 62, 152–158 CrossRef PubMed.
- Y. Mine and P. Rupa, Fine mapping and structural analysis of immunodominant IgE allergenic epitopes in chicken egg ovalbumin, Protein Eng., 2003, 16, 747–752 CrossRef CAS PubMed.
- Y. Mine and M. Yang, Epitope characterization of ovalbumin in BALB/c mice using different entry routes, Biochim. Biophys. Acta, Proteins Proteomics, 2007, 1774, 200–212 CrossRef CAS PubMed.
- L. Z. Sun, S. Elsayed, T. Aasen, T. Van Do, N. Aardal, E. Florvaag and K. Vaali, Comparison between Ovalbumin and Ovalbumin Peptide 323–339 Responses in Allergic Mice: Humoral and Cellular Aspects, Scand. J. Immunol., 2010, 71, 329–335 CrossRef CAS PubMed.
- K. Honma, Y. Kohno, K. Saito, N. Shimojo, T. Horiuchi, H. Hayashi, N. Suzuki, T. Hosoya, H. Tsunoo and H. Niimi, Allergenic epitopes of ovalbumin (OVA) in patients with hen's egg allergy: inhibition of basophil histamine release by haptenic ovalbumin peptide, Clin. Exp. Immunol., 1996, 103, 446–453 CrossRef CAS PubMed.
- E. M. Janssen, M. H. Wauben, E. H. Jonker, G. Hofman, W. Van Eden, F. P. Nijkamp and A. J. Van Oosterhout, Opposite effects of immunotherapy with ovalbumin and the immunodominant T-cell epitope on airway eosinophilia and hyperresponsiveness in a murine model of allergic asthma, Am. J. Respir. Cell Mol. Biol., 1999, 21, 21–29 CrossRef CAS PubMed.
- H. Kahlert, A. Petersen, W.-M. Becker and M. Schlaak, Epitope analysis of the allergen ovalbumin (Gal d II) with monoclonal antibodies and patients’ IgE, Mol. Immunol., 1992, 29, 1191–1201 CrossRef CAS PubMed.
- E. Abeyrathne, H. Lee, C. Jo, J. Suh and D. Ahn, Enzymatic hydrolysis of ovomucoid and the functional properties of its hydrolysates, Poult. Sci., 2015, 94, 2280–2287 CrossRef CAS PubMed.
- E. Abeyrathne, H. Lee and D. Ahn, Separation of ovotransferrin and ovomucoid from chicken egg white, Poult. Sci., 2014, 93, 1010–1017 CrossRef CAS PubMed.
- C. Giosafto, N. Rigby, A. Sorrentino, F. Mulholland and C. Mills, Optimization of In Vitro N-Deglycosylation of Ovomucoid Protein, MOJ Food Process. Technol., 2016, 2, 00058 Search PubMed.
- R. Porta, C. V. L. Giosafatto, P. di Pierro, A. Sorrentino and L. Mariniello, Transglutaminase-mediated modification of ovomucoid: effects on its trypsin inhibitory activity and antigenic properties, Amino Acids, 2013, 44, 285–292 CrossRef CAS PubMed.
- A. Winiarska-Mieczan and M. Kwiecień, Avian egg's white ovomucoid as food-allergen for human, Postepy Biochem., 2007, 53, 212–217 CAS.
- J. Kido and T. Matsumoto, Attenuated Allergenic Activity of Ovomucoid After Electrolysis, Allergy, Asthma Immunol. Res., 2015, 7, 599–604 CrossRef PubMed.
- J. Martinez-Botas, I. Cerecedo, J. Zamora, C. Vlaicu, M. C. Dieguez, D. Gómez-Coronado, V. De Dios, S. Terrados and B. De La Hoz, Mapping of the IgE and IgG4 sequential epitopes of ovomucoid with a peptide microarray immunoassay, Int. Arch. Allergy Immunol., 2013, 161, 11–20 CrossRef CAS PubMed.
- Y. Mine and J. W. Zhang, Identification and fine mapping of IgG and IgE epitopes in ovomucoid, Biochem. Biophys. Res. Commun., 2002, 292, 1070–1074 CrossRef CAS PubMed.
- K. M. Järvinen, K. Beyer, L. Vila, L. Bardina, M. Mishoe and H. Sampson, Specificity of IgE antibodies to sequential epitopes of hen's egg ovomucoid as a marker for persistence of egg allergy, Allergy, 2007, 62, 758–765 CrossRef PubMed.
- E. Holen, B. Bolann and S. Elsayed, Novel B and T cell epitopes of chicken ovomucoid (Gal d 1) induce T cell secretion of IL–6, IL–13, and IFN–γ, Clin. Exp. Allergy, 2001, 31, 952–964 CrossRef CAS PubMed.
- S. K. Cooke and H. A. Sampson, Allergenic properties of ovomucoid in man, J. Immunol., 1997, 159, 2026–2032 CAS.
- A. A. L. Tribst, M. A. B. de Morais, C. Y. Tominaga, A. F. Z. Nascimento, M. T. Murakami and M. Cristianini, How high pressure pre-treatments affect the function and structure of hen egg-white lysozyme, Innovative Food Sci. Emerging Technol., 2018, 47, 195–203 CrossRef CAS.
-
T. Silvetti, S. Morandi, M. Hintersteiner and M. Brasca, in Egg Innovations and Strategies for Improvements, Elsevier, 2017, pp. 233–242 Search PubMed.
- R. Jiménez-Saiz, S. Benedé, B. Miralles, I. López-Expósito, E. Molina and R. López-Fandiño, Immunological behavior of in vitro digested egg–white lysozyme, Mol. Nutr. Food Res., 2014, 58, 614–624 CrossRef PubMed.
- C. Le Floch-Fouéré, S. Pezennec, M. Pasco, G. Paboeuf, A. Renault and S. Beaufils, Moderate conformational impact of citrate on ovotransferrin considerably increases its capacity to self-assemble at the interface, J. Colloid Interface Sci., 2015, 437, 219–226 CrossRef PubMed.
- S. H. Moon, J. H. Lee, D. U. Ahn and H. D. Paik, In vitro antioxidant and mineral–chelating properties of natural and autocleaved ovotransferrin, J. Sci. Food Agric., 2015, 95, 2065–2070 CrossRef CAS PubMed.
- F. Giansanti, L. Leboffe, F. Angelucci and G. Antonini, The nutraceutical properties of ovotransferrin and its potential utilization as a functional food, Nutrients, 2015, 7, 9105–9115 CrossRef CAS PubMed.
- P. Tong, J. Gao, H. Chen, X. Li, Y. Zhang and S. Jian, Preparation and Immunological Reactions of a Purified Egg Allergen Ovotransferrin, Int. J. Food Prop., 2014, 17, 293–308 CrossRef CAS.
- J. Pekar, D. Ret and E. Untersmayr, Stability of allergens, Mol. Immunol., 2018, 100, 14–20 CrossRef CAS PubMed.
- J. Keller and P. Layer, Human pancreatic exocrine response to nutrients in health and disease, Gut, 2005, 54, 1–28 CrossRef PubMed.
- F. J. Moreno, Gastrointestinal digestion of food allergens: effect on their allergenicity, Biomed. Pharmacother., 2007, 61, 50–60 CrossRef CAS PubMed.
- G. Martos, P. Contreras, E. Molina and R. López-Fandiño, Egg white ovalbumin digestion mimicking physiological conditions, J. Agric. Food Chem., 2010, 58, 5640–5648 CrossRef CAS PubMed.
- K. Takagi, R. Teshima, H. Okunuki and J. I. Sawada, Comparative study of in vitro digestibility of food proteins and effect of preheating on the digestion, Biol. Pharm. Bull., 2003, 26, 969–973 CrossRef CAS PubMed.
- P. Rupa and Y. Mine, Ablation of ovomucoid–induced allergic response by desensitization with recombinant ovomucoid third domain in a murine model, Clin. Exp. Immunol., 2006, 145, 493–501 CrossRef CAS PubMed.
- J. Hirose, N. Kitabatake, A. Kimura and H. Narita, Recognition of native and/or thermally induced denatured forms of the major food allergen, ovomucoid, by human IgE and mouse monoclonal IgG antibodies, Biosci. Biotechnol. Biochem., 2004, 68, 2490–2497 CrossRef CAS PubMed.
- H. Lemon-Mulé, H. A. Sampson, S. H. Sicherer, W. G. Shreffler, S. Noone and A. Nowak-Wegrzyn, Immunologic changes in children with egg allergy ingesting extensively heated egg, J. Allergy Clin. Immunol., 2008, 122, 977–983 CrossRef PubMed.
- Y. Mine and P. Rupa, Immunological and biochemical properties of egg allergens, World's Poult. Sci. J., 2004, 60, 321–330 CrossRef.
- K. Takagi, R. Teshima, H. Okunuki, S. Itoh, N. Kawasaki, T. Kawanishi, T. Hayakawa, Y. Kohno, A. Urisu and J.-I. Sawada, Kinetic analysis of pepsin digestion of chicken egg white ovomucoid and allergenic potential of pepsin fragments, Int. Arch. Allergy Immunol., 2005, 136, 23–32 CrossRef CAS PubMed.
- J. Kovacs-Nolan, J. W. Zhang, S. Hayakawa and Y. Mine, Immunochemical and structural analysis of pepsin-digested egg white ovomucoid, J. Agric. Food Chem., 2000, 48, 6261–6266 CrossRef CAS PubMed.
- R. Jiménez-Saiz, G. Martos, W. Carrillo, R. López-Fandiño and E. Molina, Susceptibility of lysozyme to in vitro digestion and immunoreactivity of its digests, Food Chem., 2011, 127, 1719–1726 CrossRef.
- S. P. Commins and T. A. Platts-Mills, Allergenicity of carbohydrates and their role in anaphylactic events, Curr. Allergy Asthma Rep., 2010, 10, 29–33 CrossRef CAS PubMed.
- M. I. Petrosino, A. Scaparrotta, M. L. Marcovecchio, D. Panichi, D. Rapino, M. Attanasi, P. Di Filippo, S. Di Pillo and F. Chiarelli, Usefulness of molecular diagnosis in egg allergic children, Arch. Med. Sci., 2018, 14, 132 CrossRef CAS PubMed.
- E. Peñas, C. di Lorenzo, F. Uberti and P. Restani, Allergenic proteins in enology: A review on technological applications and safety aspects, Molecules, 2015, 20, 13144–13164 CrossRef PubMed.
- J.-T. Yang, C.-E. Wu, Y.-Y. Li, S.-Q. Jia, G. J. Fan and F.-R. Peng, Identification and purification of an allergic glycoprotein from Ginkgo biloba Kernel, Agric. Sci. China, 2011, 10, 631–641 CrossRef CAS.
- H. S. Hwang, J. Y. Kim, H. Park, J. Jeong, H. Hyun, T. J. Yoon, H.-Y. Park, H.-D. Choi and H. H. Kim, Cleavage of the terminal N-acetylglucosamine of egg-white ovalbumin N-glycans significantly reduces IgE production and Th2 cytokine secretion, Biochem. Biophys. Res. Commun., 2014, 450, 1247–1254 CrossRef CAS PubMed.
- H. S. Hwang, H. Park, J. Kim, J. Y. Choi, Y. K. Lee, H.-Y. Park, H.-D. Choi and H. H. Kim, Significant reduction in allergenicity of ovalbumin from chicken egg white following treatment with ascidian viscera N-acetylglucosaminidase, Biochem. Biophys. Res. Commun., 2016, 475, 107–112 CrossRef CAS PubMed.
- Y. Mine and M. Yang, Recent advances in the understanding of egg allergens: basic, industrial, and clinical perspectives, J. Agric. Food Chem., 2008, 56, 4874–4900 CrossRef CAS PubMed.
- M. Besler, H. Steinhart and A. Paschke, Allergenicity of hen's egg–white proteins: IgE binding of native and deglycosylated ovomucoid, Food Agric. Immunol., 1997, 9, 277–288 CrossRef CAS.
- S. Benedé, R. López-Fandiño, M. Reche, E. Molina and I. López-Expósito, Influence of the carbohydrate moieties on the immunoreactivity and digestibility of the egg allergen ovomucoid, PLoS One, 2013, 8, e80810 CrossRef PubMed.
- S. J. Maleki, Food processing: effects on allergenicity, Curr. Opin. Allergy Clin. Immunol., 2004, 4, 241–245 CrossRef CAS PubMed.
- F. Van Wijk, S. Nierkens, I. Hassing, M. Feijen, S. Koppelman, G. De Jong, R. Pieters and L. Knippels, The effect of the food matrix on in vivo immune responses to purified peanut allergens, Toxicol. Sci., 2005, 86, 333–341 CrossRef CAS PubMed.
- A. Nowak-Wegrzyn and A. Fiocchi, Rare, medium, or well done? The effect of heating and food matrix on food protein allergenicity, Curr. Opin. Allergy Clin. Immunol., 2009, 9, 234–237 CrossRef CAS PubMed.
- V. Schulten, I. Lauer, S. Scheurer, T. Thalhammer and B. Bohle, A food matrix reduces digestion and absorption of food allergens in vivo, Mol. Nutr. Food Res., 2011, 55, 1484–1491 CrossRef CAS PubMed.
- R. Jiménez-Saiz, I. López-Expósito, E. Molina and R. López-Fandiño, IgE-binding and in vitro gastrointestinal digestibility of egg allergens in the presence of polysaccharides, Food Hydrocolloids, 2013, 30, 597–605 CrossRef.
- S.-P. Nie, C. Wang, S. W. Cui, Q. Wang, M.-Y. Xie and G. O. Phillips, A further amendment to the classical core structure of gum Arabic (Acacia senegal), Food Hydrocolloids, 2013, 31, 42–48 CrossRef CAS.
- I. Fraeye, I. Colle, E. Vandevenne, T. Duvetter, S. Van Buggenhout, P. Moldenaers, A. Van Loey and M. Hendrickx, Influence of pectin structure on texture of pectin–calcium gels, Innovative Food Sci. Emerging Technol., 2010, 11, 401–409 CrossRef CAS.
- P. Tong, L. Xiong, J. Gao, X. Li, Z. Wu, A. Yang, J. Yuan, Y. Wu and H. Chen, Influence of heat treatment and egg matrix on the physicochemical and allergenic properties of egg custard, J. Food Sci., 2020, 85, 789–799 CrossRef CAS PubMed.
- J. Frias, Y. S. Song, C. Martínez-Villaluenga, E. G. l. De Mejia and C. Vidal-Valverde, Immunoreactivity and amino acid content of fermented soybean products, J. Agric. Food Chem., 2007, 56, 99–105 CrossRef PubMed.
- S.-H. Seo and S.-J. Cho, Changes in allergenic and antinutritional protein profiles of soybean meal during solid-state fermentation with Bacillus subtilis, LWT–Food Sci. Technol., 2016, 70, 208–212 CrossRef CAS.
- N. Kleber, U. Weyrich and J. Hinrichs, Screening for lactic acid bacteria with potential to reduce antigenic response of β-lactoglobulin in bovine skim milk and sweet whey, Innovative Food Sci. Emerging Technol., 2006, 7, 233–238 CrossRef CAS.
- Y.-S. Song, J. Frías, C. Martinez-Villaluenga, C. Vidal-Valdeverde and E. G. de Mejia, Immunoreactivity reduction of soybean meal by fermentation, effect on amino acid composition and antigenicity of commercial soy products, Food Chem., 2008, 108, 571–581 CrossRef CAS PubMed.
- J. Leszczyńska, A. Diowksz, A. Łącka, M. Bryszewska, K. Wolska and W. Ambroziak, Decrease of wheat flour allergenicity via lactic acid fermentation, Food Agric. Immunol., 2009, 20, 139–145 CrossRef.
- S. Li, M. Offengenden, M. Fentabil, M. G. Gänzle and J. Wu, Effect of egg white fermentation with lactobacilli on IgE binding ability of egg white proteins, Food Res. Int., 2013, 52, 359–366 CrossRef CAS.
- S. Li, M. Offengenden, M. G. Gänzle and J. Wu, Aspergillus oryzae reduces IgE binding ability of allergenic egg white proteins, Front. Agric. Sci. Eng., 2018, 5, 373–381 CrossRef.
- P. Sharma, S. N. Gaur and N. Arora, In silico identification of IgE-binding epitopes of osmotin protein, PLoS One, 2013, 8, e54755 CrossRef CAS PubMed.
- X. Ren, X. Zhang, Y. Li and Z. Wang, Epitope mapping and immunological characterization of a major allergen TBa in tartary buckwheat, Biotechnol. Lett., 2010, 32, 1317–1324 CrossRef CAS PubMed.
- R. Valenta, K. Niespodziana, M. Focke-Tejkl, K. Marth, H. Huber, A. Neubauer and V. Niederberger, Recombinant allergens: what does the future hold?, J. Allergy Clin. Immunol., 2011, 127, 860–864 CrossRef CAS PubMed.
- R. Valenta, B. Linhart, I. Swoboda and V. Niederberger, Recombinant allergens for allergen–specific immunotherapy: 10 years anniversary of immunotherapy with recombinant allergens, Allergy, 2011, 66, 775–783 CrossRef CAS PubMed.
- U. Nurmatov, G. Devereux, A. Worth, L. Healy and A. Sheikh, Effectiveness and safety of orally administered immunotherapy for food allergies: a systematic review and meta-analysis, Br. J. Nutr., 2014, 111, 12–22 CrossRef CAS PubMed.
- A. Nowak-Wegrzyn, New perspectives for use of native and engineered recombinant food proteins in treatment of food allergy, Immunol. Allergy Clin. North Am., 2007, 27, 105–127 CrossRef PubMed.
- P. Dhanapala, T. Doran, M. L. Tang and C. Suphioglu, Production and immunological analysis of IgE reactive recombinant egg white allergens expressed in Escherichia coli, Mol. Immunol., 2015, 65, 104–112 CrossRef CAS PubMed.
- K. Arita, E. E. Babiker, H. Azakami and A. Kato, Effect of chemical and genetic attachment of polysaccharides to proteins on the production of IgG and IgE, J. Agric. Food Chem., 2001, 49, 2030–2036 CrossRef CAS PubMed.
- Y. Mine, E. Sasaki and J. W. Zhang, Reduction of antigenicity and allergenicity of genetically modified egg white allergen, ovomucoid third domain, Biochem. Biophys. Res. Commun., 2003, 302, 133–137 CrossRef CAS.
- P. Dhanapala, D. Withanage-Dona, M. L. Tang, T. Doran and C. Suphioglu, Hypoallergenic variant of the major egg white allergen gal d 1 produced
by disruption of cysteine bridges, Nutrients, 2017, 9, 171 CrossRef PubMed.
- P. Rupa and Y. Mine, Immunological comparison of native and recombinant egg allergen, ovalbumin, expressed in Escherichia coli, Biotechnol. Lett., 2003, 25, 1917–1924 CrossRef CAS PubMed.
- M. Iwan, Y. M. Vissers, E. Fiedorowicz, H. Kostyra, E. Kostyra, H. F. Savelkoul and H. J. Wichers, Impact of Maillard reaction on immunoreactivity and allergenicity of the hazelnut allergen Cor a 11, J. Agric. Food Chem., 2011, 59, 7163–7171 CrossRef CAS PubMed.
- A. Ilchmann, S. Burgdorf, S. Scheurer, Z. Waibler, R. Nagai, A. Wellner, Y. Yamamoto, H. Yamamoto, T. Henle and C. Kurts, Glycation of a food allergen by the Maillard reaction enhances its T-cell immunogenicity: role of macrophage scavenger receptor class A type I and II, J. Allergy Clin. Immunol., 2010, 125, 175–183 CrossRef CAS PubMed.
- M. Toda, M. Heilmann, A. Ilchmann and S. Vieths, The Maillard reaction and food allergies: is there a link?, Clin. Chem. Lab. Med., 2014, 52, 61–67 CAS.
- E. C. Mills, A. I. Sancho, N. M. Rigby, J. A. Jenkins and A. R. Mackie, Impact of food processing on the structural and allergenic properties of food allergens, Mol. Nutr. Food Res., 2009, 53, 963–969 CrossRef CAS PubMed.
- P. Rupa, S. Nakamura, S. Katayama and Y. Mine, Effects of ovalbumin glycoconjugates on alleviation of orally induced egg allergy in mice via dendritic–cell maturation and T–cell activation, Mol. Nutr. Food Res., 2014, 58, 405–417 CrossRef CAS PubMed.
- X. Ma, J. Gao, P. Tong, H. Yang, Q. Zu, X. Meng, J. Lu and H. Chen, Effects of Maillard reaction conditions on in vitro immunoglobulin G binding capacity of ovalbumin using response surface methodology, Food Agric. Immunol., 2015, 26, 835–847 CrossRef CAS.
- Z.-W. Liao, Y.-H. Ye, H. Wang, Y. Chen, X.-M. Sha, L. Zhang, T. Huang, Y.-M. Hu and Z.-C. Tu, The mechanism of decreased IgG/IgE-binding of ovalbumin by preheating treatment combined with glycation identified by liquid chromatography and high-resolution mass spectrometry, J. Agric. Food Chem., 2018, 66, 10693–10702 CrossRef CAS PubMed.
- A. K. Verma, S. Kumar, M. Das and P. D. Dwivedi, Impact of thermal processing on legume allergens, Plant Foods Hum. Nutr., 2012, 67, 430–441 CrossRef CAS PubMed.
- A. Paschke and M. Besler, Stability of bovine allergens during food processing, Ann. Allergy, Asthma, Immunol., 2002, 89, 16–20 CrossRef CAS.
- T. Rahaman, T. Vasiljevic and L. Ramchandran, Effect of processing on conformational changes of food proteins related to allergenicity, Trends Food Sci. Technol., 2016, 49, 24–34 CrossRef CAS.
- N. Stanciuc, A. A. Creţu, I. Banu and I. Aprodu, Advances on the impact of thermal processing on structure and antigenicity of chicken ovomucoid, J. Sci. Food Agric., 2018, 98, 3119–3128 CAS.
- M. Claude, G. G. Bouchaud, R. Lupi, L. Castan, O. Tranquet, S. Denery-Papini, M. Bodinier and C. Brossard, How proteins aggregate can reduce allergenicity: Comparison of ovalbumins heated under opposite electrostatic conditions, J. Agric. Food Chem., 2017, 65, 3693–3701 CrossRef CAS PubMed.
- M. Claude, R. Lupi, G. Bouchaud, M. Bodinier, C. Brossard and S. Denery-Papini, The thermal aggregation of ovalbumin as large particles decreases its allergenicity for egg allergic patients and in a murine model, Food Chem., 2016, 203, 136–144 CrossRef CAS PubMed.
- R. Jiménez-Saiz, J. Belloque, E. Molina and R. López-Fandino, Human immunoglobulin E (IgE) binding to heated and glycated ovalbumin and ovomucoid before and after in vitro digestion, J. Agric. Food Chem., 2011, 59, 10044–10051 CrossRef PubMed.
- T. Fushiki, N. Yamamoto and K. Iwai, Investigation of digestion and absorption of a dietary protein by using its antigenicity as an index: gastrointestinal digestion of ovalbumin, Agric. Biol. Chem., 1985, 49, 1335–1342 CAS.
- X. Ma, D. Lozano-Ojalvo, H. Chen, R. Lopez-Fandiño and E. Molina, Effect of high pressure-assisted crosslinking of ovalbumin and egg white by transglutaminase on their potential allergenicity, Innovative Food Sci. Emerging Technol., 2015, 29, 143–150 CrossRef CAS.
- Y. Chi, Y. Li and Y. Zhao, Effect of Dynamic High-pressure Microfluidization on Egg White Protein Allergenicity and Digestibility, Trans. Chin. Soc. Agric. Mach., 2017, 6, 41 Search PubMed.
- J. Yuan, Y. Zheng, Y. Wu, H. Chen, P. Tong and J. Gao, Double enzyme hydrolysis for producing antioxidant peptide from egg white: Optimization, evaluation, and potential allergenicity, J. Food Biochem., 2020, 44, e13113 CrossRef PubMed.
- Y. Lu, S. Li, H. Xu, T. Zhang, X. Lin and X. Wu, Effect of covalent interaction with chlorogenic acid on the allergenic capacity of ovalbumin, J. Agric. Food Chem., 2018, 66, 9794–9800 CrossRef CAS PubMed.
- K. Liu, S. Chen, H. Chen, P. Tong and J. Gao, Cross-linked ovalbumin catalyzed by polyphenol oxidase: Preparation, structure and potential allergenicity, Int. J. Biol. Macromol., 2018, 107, 2057–2064 CrossRef CAS PubMed.
- T. Zhang, Z. Hu, Y. Cheng, H. Xu, T. C. Velickovic, K. He, F. Sun, Z. He, Z. Liu and X. Wu, Changes in Allergenicity of Ovalbumin in Vitro and in Vivo on Conjugation with Quercetin, J. Agric. Food Chem., 2020, 68, 4027–4035 CrossRef CAS PubMed.
- J. Kido and T. Matsumoto, Attenuated Allergenic Activity of Ovomucoid After Electrolysis, Allergy, Asthma Immunol. Res., 2015, 7, 599–604 CrossRef PubMed.
- P. Tong, L. Gao, J. Gao, X. Li, Z. Wu, A. Yang and H. Chen, Iron-induced chelation alleviates the potential allergenicity of ovotransferrin in a BALB/c mouse model, Nutr. Res., 2017, 47, 81–89 CrossRef CAS PubMed.
|
This journal is © The Royal Society of Chemistry 2022 |
Click here to see how this site uses Cookies. View our privacy policy here.