DOI:
10.1039/D2FO00099G
(Review Article)
Food Funct., 2022,
13, 6467-6483
Designing food for the elderly: the critical impact of food structure
Received
10th January 2022
, Accepted 21st May 2022
First published on 9th June 2022
Abstract
Ageing is an unavoidable progressive process causing many changes of the individual life. However, if faced in an efficient way, living longer in a healthy status could be an opportunity for all. In this context, food consumption and dietary patterns are pivotal factors in promoting active and healthy ageing. The development of food products tailored for the specific needs of the elderly might favour the fulfilment of nutritionally balanced diets, while reducing the consequences of malnutrition. To this aim, the application of a food structure design approach could be particularly profitable, being food structure responsible to the final functionalities of food products. In this narrative review, the physiological changes associated to food consumption occurring during ageing were firstly discussed. Then, the focus shifted to the possible role of food structure in delivering target functionalities, considering food acceptability, digestion of the nutrients, bioactive molecules and probiotic bacteria.
1 Introduction
For the first time in history, the elderly (with age > 65 years) represent worldwide the fastest growing segment of the population and the number of individuals over the age of 65 is predicted to increase from 703 million in 2019 to 1.2 billion in 2025 and to 2 billion by 2050.1,2 Such a perspective appears positive, as many individuals are expected to live longer. However, new aspects impacting our lives, from health care and social relationships to economic growth and fiscal sustainability, need to be tackled.3 The challenge is to maintain vitality and good quality of life for as long as possible, while reducing the morbidity and the years of disabilities. Thus, the boosting of policies dedicated to tackle ageing related issues is a priority for the international community.
Food consumption and dietary patterns, in association with physical activity, are recognized as pivotal factors in promoting an active and healthy ageing. These are respectively defined as “the process of optimizing opportunities for health, participation and security in order to enhance quality of life as people age” and as “the process of developing and maintaining the functional ability that enables well-being in older age”.2 Nutrition plays a critical role in modulating the development of many age-related physiological changes and diseases. While a number of chronic diseases are directly correlated to an excess of food intake (e.g. obesity, type II diabetes, hypertension, cancer), inadequate nutrient intake increases elderly frailty, worsening important functional abilities, such as immunity, bone health, and cognitive functions.
In this context, the design of foods tailored for the specific needs of the elderly might favour the fulfilment of nutritionally balanced diets, while reducing the consequences of malnutrition. To design foods for the elderly, food composition is only one side of the coin. In fact, it is widely accepted today that the practice of linking food composition to physiological outcomes generates inconsistent results between predicted and actual health benefits.4,5 The other and still underestimated side of the coin is how food components interact to generate a defined hierarchical organization at different scale lengths, from nano to macro dimensions, which is the food structure. The latter could significantly impact many food functionalities, comprising food stability, sensory acceptance, as well as oral processing and gastrointestinal behaviour. A complex series of food structure changes characterize the food lifespan from the transformation of raw materials into final products to their consumption and utilization in the human body.
In this narrative review, after examining the physiological changes associated to food consumption in old age, the possible role of food structure in delivering target functionalities has been critically discussed, considering food acceptability, digestion of the nutrients, bioactive molecules and probiotic bacteria. To this aim, literature from nutritional studies on elderly population was merged with those reporting the most recent finding on food structure and functionalities. Potentially relevant articles were identified through a computerized search in PubMed, Food Science and Technology Abstract, and Scopus.
2 Ageing and food consumption behaviour
Diet is a key factor to maintain health status at all ages. During ageing, the diet has an even more substantial impact on life quality and in turn on longevity. Nonetheless, many age-related factors could compromise the ability of individuals to attain the required nutritional needs. Physiological alterations associated with ageing are one of the main determinants affecting food consumption and utilization in the body. Table 1 summarizes the main physiological changes associated with ageing and their consequences on food consumption and utilization behaviours. With ageing, people feel less hungry and thirsty on average due to differences in satiety signals associated to hormonal changes.6,7 As a consequence, they eat and drink reduced quantities of food and beverages, which might lead to inadequate intake of total calories and water, unbalanced consumption of macronutrients, and insufficient ingestion of micronutrients and bioactive compounds.8 Additionally, the eating capability, defined as a combination of hand manipulation, oral processing, sensation, and cognition capacity, deeply changes.9 In fact, difficulties in the use of hands due to injuries, diseases, or distortions can hinder the ability to bring the food into the mouth.10,11 Also, the lack of teeth and the reduced chewing and swallowing capacity can alter food oral processing,12 with a consequent impact not only on perception and food acceptability, but also on food digestion and nutrient release.
Table 1 Physiological changes occurring during food consumption in the elderly and expected consequences
Food consumption stage |
Change |
Expected consequence |
Ref. |
Oral phase |
Reduced sensory perception |
Reduced appetite and desire to eat and drink, changes in food choice, dehydration |
23–33
|
Reduced capability of mastication |
Change in food choices, increased oral processing time, insufficient food disintegration and reduced digestion efficiency |
Dysphagia |
Increased risk of choking, insufficient moistening and food disintegration, reduced digestion efficiency |
Reduced salivary secretion |
Dry mouth and reduced bolus hydration |
Gastric phase |
Increased average pH, reduced levels of enzymes (e.g. pepsin, lipase) |
Reduced digestion efficiency, modified absorption and bioavailability of food components |
6, 14, 15, 34, 35 |
Decreased gastric emptying |
Increased transit time |
Intestinal phase |
Modification of bile and pancreatic juices composition, reduced concentrations of enzymes (e.g. pancreatin, lipase, α-amylase) |
Reduced of digestion efficiency, modified food components absorption and bioavailability |
6, 14, 15, 34, 36–38 |
Altered intestinal motility |
Increased transit time, constipation |
Altered intestinal microbiota |
Malabsorption of nutrient, increased incidence of gastrointestinal dysfunctions and infections |
Altered hormone responses (e.g.: cholecystokinin, ghrelin) |
Increased sensitivity to the satiating effects, reduced hunger and thirst |
After swallowing, the bolus transformation into utilizable components is influenced by the physiological modifications of the gastrointestinal functions (e.g. food transit and digestion rate, hormonal responses, enzyme output, nutrient absorption, and gut microbiota composition) occurring during ageing.13 For instance, the digestion rate of carbohydrates (mainly represented by starch) and the consequent rapid release of glucose is expected to be faster in the elderly.13,14 Moreover, as age increases, protein and lipid digestibility can change as a consequence of the declined production of pepsin and lipase.14,15
Finally, it should not be underestimated the role of the gut microbiota. Ageing and age-related diseases are frequently associated to a modification of the overall number and species diversity of the complex ecosystem of bacteria, fungal, and viral species living as symbiotic companions in our body from birth to death.16 Moreover, there is increasing awareness of the association between gut microbiota and brain functions, which has come to be known as the gut-brain axis,17,18 affecting cognitive outcomes and decline.19
All these physiological changes, which are associated with possible concomitant presence of chronic diseases (e.g. dementia, depression, cancer, stroke) and social constraints (e.g. lowliness, poor economic situations, difficulties in getting foods) further impair the food consumption of the elderly.14,20,21 As a consequence, malnutrition meant as the insufficient and unbalanced intake of macro- and micro-nutrients, is a common situation in older adults.14,22
3 Designing foods for the elderly
Based on these considerations and with the final aim to fight elderly malnutrition, there is the need to develop and supply foods specifically designed to promote the well-being and health of the elderly. To this aim, a rational design approach should be applied. This should starts by defining the target needs (i.e. contrast to unbalanced intake or deficiency or eating capability and sensory perceptions changes), followed by the identification of the food requirements to tackle inappropriate dietary patterns (Table 2). In this context, the definition of food (re)formulation requirements is needed. However, it is noteworthy that the assumption that food health effects are simply related to the content of specific nutrients represents an enormous gap in our knowledge, since not only “content” but also “functionality” of food components play a key role.39,40 The definition of the proper hierarchical organization of food elements, named food structure, is strategic at this stage to deliver the desired food functionalities.39–42 In fact, from one side, food structure affects food acceptability and sensory perception being associated to the macroscopic feature of foods as well as aroma release rate and taste from the matrix during eating.42,43 From the other side, food structure deeply influences nutritional and health performances of macro- and micro-nutrients and bioactive components during digestion.44–47 Hence, the design of food for the elderly might combine structure, formulation as well as approaches not only to deliver highly acceptable foods but also to steer food effecting on the body. It is worthy to note that the development of tailored foods for the elderly necessarily requires the application of an integrated approach by which the efficacy of technological interventions towards functionality are assessed, while guaranteeing the accomplishment of quality requirements.48
Table 2 Main issues in elderly dietary patterns, major expected outcomes, and relevant food requirements
Issue |
Major expected consequence |
Food requirements |
Impaired oral and digestion processing |
General malnutrition |
Easy to chew, easy to swallow, highly bioavailable food components |
High intake of sugar and highly digestible carbohydrates |
Hyperglycaemia and hyperinsulinemia, increase in triglyceride levels and reduction in high-density lipoprotein cholesterol levels, insulin resistance, inflammation, oxidative stress, muscle damage, decline of cognitive function, microvascular complications including renal damage |
Controlled carbohydrates digestion rate, reduced sugar content |
Low intake of fibre |
Constipation and other colon dysfunctions, microbiome dysbiosis and related consequences such as obesity and metabolic syndrome trigged by proinflammatory responses |
High content of soluble and/or insoluble fibres |
Low intake of proteins |
Loss of skeletal muscle mass, function, and strength, immune-senescence, sarcopenia and frailty |
Highly bioavailable proteins with good amino acid scoring |
Excessive intake of total lipids; unbalance intake of saturated/unsaturated fatty acids; low quantities of ω-3 long chain fatty acids |
Inflammation, oxidative stress, changes in microbiota, increased risk of cardiovascular diseases |
High content of polyunsaturated lipids, low content of saturated fats |
Deficiency of vitamins, especially vitamin D and B12, and minerals, especially calcium |
Reduction of muscle and bone health, decline of cognitive functions, immune-senescence |
Presence of highly bioavailable forms of vitamins and minerals |
Low intake of bioactive compounds |
Cell oxidative stress increase, impairment of cognitive functions |
Enrichment with bioactive components |
Low intake of probiotic bacteria |
Microbiota dysbiosis |
Enrichment with probiotics |
Excessive intake of salt |
Hypertension, impaired kidney and blood vessel functions, increased risk of stroke |
Low salt content |
Low intake of water |
Dehydration, impaired cognition |
High water content in solid/semisolid foods |
3.1 Technological interventions to steer food structure
Proteins, polysaccharides, and lipids can be regarded as the basic building blocks needed to develop food structures. These components are naturally present in food materials in their native structural organization, comprising, as not exhaustive list, oil bodies, cell walls, starch granules, fat crystals, and protein or polysaccharide stands.49 The application of food processing can lead to the de-structuring of this original organization followed by diverse structuring phases able to induce interactions among the building-blocks that finally generate manifold structures. Thus, the final product structure can be considered as the resultant of multiple structuring and de-structuring phases during food lifespan. The understanding of the multi-level organization of food components as well as the effect of food formulation and processing on this organization is today recognised as fundamental to design foods able to deliver target functionalities.5,39,41
Manifold of processing interventions can be applied to modify native food structures (Table 3). Milling, heat treatments, centrifugation, filtration, and enzymatic transformation can be regarded as the most widely and traditionally applied unit operations with a high de-structuring capacity, even if structuring phenomena are not excluded.
Table 3 Main processing interventions that can be applied to modify native food structures
Technological interventions |
De-structuring and re-structuring effect |
HPH: high pressure homogenization; HPP: high pressure processing; PEF: pulsed electric fields; US: ultrasounds. |
Milling |
Native structure disruption; size reduction |
Centrifugation |
Component separation |
Filtration |
Component separation |
Heat treatments (cooking, baking, steaming, roasting) |
Chemical and/or physical changes due to heat transfer; water removal; new structure formation |
Enzymatic bioprocesses |
Hydrolysis; crosslinking |
Novel non-thermal technologies (HPH, HPP, PEF, US, cold plasma) |
Native structure disruption; new structure formation |
Besides, in the last years, a number of emerging non-thermal technologies have been proposed due to their “green” character and efficiency in favouring tissue softening and modification of biopolymer original structures due to their ability to breakdown native organization.50–52 Food ingredients obtained by de-structuring interventions of raw materials are then used to prepare multi-component and multi-phase foods. The re-assemble of these components is enabled through a precise sequence of process interventions that can be performed at industrial level or by consumers during domestic food preparation. Different structures can be finally obtained including, above all, gels, foams, emulsions, and dried porous materials, as summarised in Table 4.
Table 4 Main food structures generated by technological interventions
|
Structure |
Composition |
Microscopic appearance |
Technological intervention |
Light blue square: water phase; Yellow square: oil phase; Blu lines: hydrocolloid network; Orange lines: oleogelator network; Light blue cycles: water droplets; Yellow cycles: oil droplets; White cycles: air bubbles; Grey cycles: pores; Orange cylinder: dried gel. |
Gel |
Hydrogel |
Water phase |
|
Heating/cooling |
Hydrocolloid |
Mixing |
Oleogel |
Lipid phase |
|
|
Oleogelator |
|
Bigel |
Gelled lipid phase |
|
|
Gelled water phase |
|
Hydrocolloid |
|
Oleogelator |
|
|
Emulsion |
Microemulsion |
Water phase |
|
Rotor/stator mixing |
Nanoemulsion (water in oil; oil in water) |
Lipid phase |
High pressure homogenization |
|
Emulsifier |
Ultrasonication |
|
|
Microfluidization |
Multiple emulsion (water in oil in water; oil in water in oil) |
|
|
|
|
Foam |
Aqueous foam |
Water phase |
|
Rotor-stator mixing |
|
|
Air |
Turbulent mixing |
|
|
|
Steam injection |
|
|
|
Microfluidization |
|
Porous material |
Aerogel |
Dried gel |
|
Air drying |
Xerogel |
Air |
Freeze drying |
cryogel |
|
Supercritical CO2 drying |
|
|
|
|
Mixed structures |
Oleofoam |
Oleogel |
|
Rotor-stator mixing |
Air |
Turbulent mixing |
|
Steam injection |
|
Microfluidization |
Emulsion gel |
Gel |
|
Rotor/stator mixing |
Emulsion |
High pressure homogenization |
|
Ultrasonication |
|
Microfluidization |
|
Heating/cooling |
|
Mixing |
Each of these structures exists thanks to the ability of different molecules to organize themselves at different length scale from nano-, micro- and macro-level in a defined environment and under specific technological interventions. It should also be considered that they can be present alone in a food product or in combination with the others, generating the complex final food structure with related functionalities that should be preserved until food consumption. Interestingly, many recent literature reviews summarised the novel finding in the development of these structures53–59 demonstrating their high potentiality. The proper technological intervention to obtain the desired structure should be selected considering the modifications occurring in the food structure not only during processing, but also storage, consumer handling, and digestion. The latter consists in a huge number of enzymatic, mechanical, and chemical events that inevitably cause structuring and de-structuring phenomena impacting food functionalities in terms of release and adsorption of nutrients.5,39,41,60,61
4 Designing food structures to tackle elderly needs
To meet the elderly's needs reported in Table 2, different food structure design strategies can be applied depending on the intended purpose. The possible approaches proposed in the most recent literature are described below and summarized in Fig. 1.
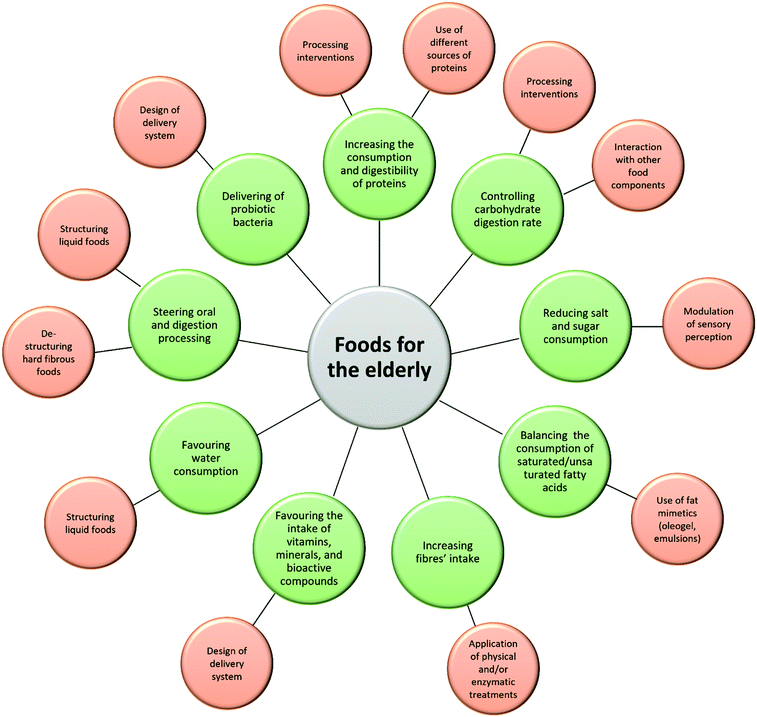 |
| Fig. 1 Food structure design approaches to tackle elderly needs. | |
4.1 Steering oral and digestion processing
As previously mentioned, the elderly could undergo a general malnutrition due to the changes associated to the oral processing, defined as the sequence of transformations that food undergoes from the first bite until swallowing including mastication, transportation, and bolus formation (Table 1).
An important aspect of food oral processing is the initiation of food digestion with the original structure breakdown and mixing with saliva. The food structure is firstly deformed, destabilized, and reduced into smaller fragments by forces applied by the teeth and tongue. The fragments formed upon chewing are then mixed with saliva, producing a cohesive bolus that can be safely swallowed.62 The extent of oral processing efforts needed to produce a swallowable bolus depends on the initial characteristics of the food structure as well as individuals’ eating capability.28 Liquid/viscous foods are easily processed and swallowed, while solid foods (e.g. hard, chewy, and less moist/lubricated structures) require a series of oral actions and longer periods in the oral cavity.63,64 Moreover, the continuous transformations of food structures during oral processing produces the multiple sensations that are processed by humans as sensory perception.65 At the beginning of oral processing, when the structure is deformed and fragmented, texture sensations are related to the initial mechanical/rheological properties of foods. At later stages of oral processing, the flow behaviour of the bolus and the interfacial properties are the main contributors to perception. Moreover, since in the bolus a part of the structures originally present are de-structured while some others still remain as well as new structures are formed,66,67 the study of bolus structure is believed to have pivotal importance in understanding the physiological responses of foods.67 As chewing efficiency and saliva characteristics change with age,32,68 the initial stages of digestion and consequently the release of nutrients can be compromised.
Due to the intimate connection between food structure and oral processing, the design of tailored food structures could contribute to facing the physiological changes occurring during ageing (Table 1) and be responsible for improving oral processing efficiency facilitating food consumption and increasing their acceptability by the elderly.
As aforementioned, combinations of proper formulation and process interventions could be applied to modify food structure, thus steering oral processing. To this regard, liquid foods, such as fruit juices, drinks, and soups, can be converted into soft materials to facilitate their swallowing.69 A variety of different ingredients, including thickeners, gelling agents, and emulsifiers, can be used to generate viscous dispersions, soft gels, foams, emulsions, etc. from liquid foods. From the other side, hard fibrous foods from plant or animal origin can be de-structured to confer them a softer consistency by applying the above mentioned conventional (milling, heating, baking, or steaming; freeze-thawing; enzymatic hydrolysis) and unconventional technologies (high-pressure processing, sonication, and pulsed-electric fields) (Table 3). A further strategy is represented by the application of proper formulation and technological interventions to induce the formation of structures, such as gels, foams, and emulsions (Table 4).
As already discussed, the bolus fate upon digestion is further influenced by the physiological modification of the gastrointestinal functions in terms of food transit, digestion rate, gastric emptying, gut microbiota composition, hormonal responses, and nutrient absorption (Table 1). All these alterations impair the digestibility and further utilization of macronutrients, micronutrients, bioactive molecules, and probiotic bacteria. Thus, specific actions should be developed depending of the intended component to be delivered, as described below.
4.2 Controlling carbohydrate digestion rate
Starch-rich foods, such as potatoes, rice, bread, pasta, cookies, and other bakery products, are staple foods being the major source of energy for our body. Modulating starch digestibility could allow targeting the physiological process reducing the negative impact of excessive glucose load, while satisfying the energy demand in the elderly. Several studies have reported efforts to control starch digestibility by modulating its structure.70–73 These include formulation and process interventions as well as the exploitation of the capability of glucose to interact with other molecules and carbohydrate digestive enzymes inhibiting approaches.
As known, in presence of water, starch granules undergo gelatinization resulting in a gel-like structure under processing. Gelatinized starch is used in foods to obtain defined rheological and mechanical properties. Gelatinized starch is more prone to the amylase activity leading to a faster glucose release than native starch.74 Thus, depending on the degree of starch gelatinization, the food might exhibit different glycemic index (GI). Controlled starch gelatinization can be obtained by means of physical treatments, including both conventional heat treatments (e.g. autoclaving, annealing, and heat-moisture treatment)74 and novel technologies, such as pressure-based technologies, electric field treatments, sonication or non-thermal plasma.75–78 These interventions can be applied to obtain partially gelatinized starch to be incorporated into a food as an ingredient with defined functionality.
In designing carbohydrate-rich foods with controlled digestibility, the presence of other components can be also used to modulate digestibility. In fact, the formation of chemical or physical interactions between starch and other molecules, such as proteins, hydrocolloids or lipids, could be effective in controlling starch digestibility by modulating the ability of α-amylase to reach the action sites.79,80 Soluble fibres (e.g. β-glucans, inulin) are one of the most common ingredients used to limit starch digestibility. These molecules would hinder the access of enzymes by interacting with starch granules at the surface, by forming a continuous hydrated network surrounding starch granules, or by increasing the viscosity of the digesta.81 Also the presence of lipids and proteins has been reported to affect the glycaemic response. Lipids contribute to starch digestion rate decrease by complexing amylose;71,82 while proteins can interact with starch generating a network surrounding the carbohydrate that acts as barrier to enzyme access.83,84 Finally, minor components, such as polyphenols, lecithin, organic acids, and salts seem to inhibit the digestive enzyme activity, reducing starch hydrolysis.85–87
Overall, this evidence suggests that carbohydrates structure as well as the whole food structure have a critical impact on the glycaemic response. Different possible food structure design strategies could be applied to control carbohydrate digestion rate. All these strategies should be pursued to generate a variety of foods targeted for people that need to control the glycaemic response, eventually allowing a reduction of pharmacological interventions. To reach this goal, however, in vivo studies are needed to validate to what extent food structures can be modulated to control the digestion rate of carbohydrates. Thus, research efforts should be strengthened in this direction.
4.3 Increasing fibres intake
As well known, high dietary fibre intake contributes to lowering the risk of several degenerative diseases, such as cardiovascular diseases, type 2 diabetes, and obesity.88,89 However, hard, fibrous, dry, sticky, or adhesive textural attributes of high fibre containing foods often discourage the consumption of food products by the elderly. Studies have shown that products that are instinctively judged “too hard”, “difficult to eat” or “not sure to swallow” are not perceived as desirable foods by the elderly.26,30,90 Thus, the possibility to design foods with high fibre content with acceptable textural outcomes may help to meet the elderly need to eat more fibrous foods. Bread and other cereal products (e.g. pasta, biscuits, cracker, breakfast cereals), which are good candidates for fibre enrichment, are widely consumed by the elderly. Dietary fibres that can be used for food incorporation include inulin, oligosaccharides, β-glucans deriving from flours of different cereals and pulses. In order to overcome a decrease in quality characteristics of fibre-containing foods, the structure of fibres and cell wall materials can be modified both enzymatically and through physical treatments in order to generate the desired texture.91,92 For instance, enzymatic hydrolyses can be used to improve insoluble fibres solubility. Alternatively, as already stated, many de-structuring process interventions can be applied to obtain foods more acceptable by elderly consumers (Table 3).
In conclusion, de-structuring interventions associated within proper formulation strategies could allow to overcome the negative impact of fibre inclusion into foods thus improving their overall quality and sensory acceptability by the target population (i.e. the elderly).
4.4 Reducing salt and sugar consumption
Salt and sugar reduction in foods is challenging since both ingredients play a pivotal role in food acceptability. For the elderly, this situation is much more impacting due to the reduction of taste perception which steers them to foods with high content of salt and sugar.
When a food product is eaten, the sodium and chloride ions or sugar molecules are released into the mouth. The release rate depends on the structure and composition of the food as well as on mastication and salivation.93 Thus, besides the use of salt or sugar replacers or boosters, an effective approach for salt/sugar reduction in foods is modulating their perception by designing food structure. To this aim, different approaches have been described, comprising the definition of the optimal crystal size and morphology; the development of inhomogeneous spatial distribution of sugar or salt in the food matrix; the increase in food porosity or the design of multilayer or emulsified structures.94–97 For instance, Sala and Stieger (2013)94 investigated the influence of changing the fracture mechanics of agar–gelatine–oil composite gels containing sugar. Results showed that the maximum sweetness intensity of the most brittle gel was twice as intense as the least brittle gel and reached maximum intensity in less than half the time. Enhancement of sweetness perception and reduction of sucrose content in solid food products can be achieved through modification of the serum or fluid released from solid food structures.98 In principle, a greater quantity of fluid containing solubilized sucrose/sugar released from a structure during mastication can increase the quantity of sucrose delivered to sweet taste receptors, as demonstrated by Sala, Stieger, and van de Velde (2010)98 for mixed whey protein isolate/gellan gum gels.
Based on the aforementioned considerations, a proper food structure design could help in the shift from the conventional approach of using salt/sugar substitutes with all their limitations to a more sustainable strategy associated to the “unconventional” structuring of foods to modulate sensory perceptions.
4.5 Balancing the consumption of saturated/unsaturated fatty acids
Fats rich in saturated fatty acids, including animal fats (e.g. butter and lard), tropical oils (e.g. palm oil, palm kernel oil, and coconut oil) as well as margarine and high saturated shortenings are multipurpose ingredients that are used to modulate food structure and sensory characteristics (taste, colour, flavour, crispiness, and creaminess). Due to their peculiarities, it is challenging from the consumer point of view to renounce saturated fat-rich foods as well as for food producers to substitute their unique technological functionalities to manufacture foods rich in healthy fats.99,100 From the other side, vegetable or fish oils, which are rich in unsaturated or polyunsaturated fatty acids, have many nutritional advantages.101,102 However, their susceptibility to oxidation during processing and storage has to be taken into account both from quality and health points of view. Additionally, their liquid state at ambient temperature limits the range of products in which they can be added without modifying the sensory food perception.
One of the most innovative and promising ways to face these issues is the development of oleogels obtained from mono and polyunsaturated fatty acids-rich liquid oils, which are structured into semi-solid materials.55,103 The interest in oleogels has dramatically increased in the last decade due to their potential application as replacers of common hard stock fat in different food products.55,100,104,105
More recently, oleogels have also been proposed as efficient tools to modulate lipid digestion as well as deliver nutrients and bioactive compounds.106–111These authors demonstrated the ability of oleogels to reduce the lipolysis extent, probably by hindering the lipase activity. Such an effect can be modulated by the proper selection of the gelator type and the relevant concentration. In fact, both oleogel firmness as well as microstructure seem to have a role in lipid digestion. Interestingly, also some in vivo studies highlighted the significant impact of lipid physical state on post-prandial plasma triglycerides, glycaemia, and appetite when comparing the co-ingestion of a carbohydrate-rich meal with ethylcellulose-oleogel instead of liquid oil.108–110
Besides oleogels, a wide variety of emulsion gels have been proposed in literature as possible fat substitutes. Emulsion gels are generally formed by an edible oil (from vegetable, marine, and other sources) entrapped in a gel network dispersed in a continuous water phase and structured by biopolymers (e.g. proteins, fibres, etc.) and/or amphiphilic molecules or stabilizers. For instance, monoglyceride-structured emulsions showed good performances in different bakery products, ice cream, and cheese;112,113 whereas k-carrageenan, locust bean gum, or inulin-based gelled emulsions were used to substitute animal fats in burgers, meat batters, and frankfurter.114–117 As for the case of oleogels, also the structure of emulsion gels could affect lipid digestion.
Moreover, the manipulation of gel strength and microstructure allows modifying the extent and rate of lipid digestion. For instance, Guo et al. (2017)118 reported that the lipid digestion rate decreased as the firmness of an emulsion gel increased. Similarly, Gu et al. (2017)119 showed that lipid droplets encapsulated within protein microgels were digested more slowly than free lipid droplets.
It can be summarised that food structure can play a dual role in designing lipid containing foods for the elderly. It allows not only to generate sensory acceptable products with a balanced nutrient content but also to control lipid digestion rate and extent. However, it is a matter of fact that the transition towards foods with higher content of unsaturated fatty acids needs considerations regarding food stability and the control of lipid oxidation during storage to avoid delivering unacceptable as well as hazardous foods.120,121
4.6 Increasing the consumption of proteins
A systematic review on macronutrient intakes in older adults in Western populations reported that 10% of adults aged over 60 years did not meet the estimated average requirement of protein set by the WHO (i.e. 0.66 g per kg body weight per day).122
To face nutritional deficiencies associated with proteins, there is the need to enlarge the protein sources considered in the diet. Besides it, the transition from a prevalent animal protein (such as meat, poultry, egg, and milk) consumption towards a prevalent plant-based diet should also be favoured. Examples of alternative sources of proteins are mainly pulses and cereals. However, plant proteins are characterised by reduced technological functionalities (e.g. water and oil binding capacity, emulsifying, gelling, and foaming capacity) and digestibility when compared to the animal-derived ones. Plant proteins also have a lower content of essential amino acids that may be responsible for the lower anabolic capacity of these proteins compared with animal-based ones.123
The possibility to increase plant-protein usage in food formulations is thus dependent on the identification of strategies to steer their technological and nutritional performances and the ability to efficiently blend them. Both functionalities are strictly dependent on protein structure (primary, secondary, and tertiary) and their ability (surface properties, water–oil binding capacity, exposure of hydrophilic or lipophilic sites) to interact with other food components.124
In the last five years, an increasing number of papers focussed on possible technological interventions that can be used to steer protein technological functionalities and digestibility, mainly aimed at modifying plant protein structure. Many conventional technological interventions, such as heating, dehulling, soaking, germination, fermentation, freeze–thaw cycles, and extrusion, have been demonstrated to improve the technological and nutritional quality of plant proteins thanks to their de-structuring ability.125 However, it is also recognised that over-processing could generate a further reorganization and re-aggregation of proteins leading to a decrease of their functionalities.126
In this context, the application of novel technologies (Table 3) appears particularly promising to reduce the impact of high energy processing.50,51,127 Protein modification via the creation of larger or smaller molecules or aggregates can lead to alterations in traditional protein functional properties and may give rise to new ones. For instance, HPH has been recently proposed as an effective tool to induce structural changes in proteins, enhancing their functional properties. HPH has been demonstrated to improve the functional properties of potato,128 lentil,129 faba bean,130 myofibrillar,131 soy,132,133 kidney beans,66 and pea.134 Similarly, PEF treatments were studied as feasible technique to change protein structure and functionality.135,136 At the moment, this is an open and hot topic being the final result not easily predictable due to the differences in protein structure and susceptibility to processing. What is clear from the literature is that for each protein type it is necessary to determine the best performing process to obtain the desired result in terms of functional properties. Finally, to face the lower amino acid scoring of plant proteins, combinations of various plant-based proteins or blends of the animal and plant-based proteins needs to be explored in order to provide properties that closely reflect those of animal-based proteins.
Nowadays, the use of plant-based proteins as food ingredients and its implications are in the spotlight. Food structure design appears pivotal in modifying protein technological and health functionalities to enlarge the possible application of novel protein sources. However, in many cases, their application is hindered due to a reduced sensory acceptability as a consequence of the presence of typical off-flavours. Strategies to modulate these unpleasant sensory attributes are needed and the research on this topic is still lacking.
4.7 Favouring the intake of vitamins, minerals, and bioactive compounds
Besides macronutrients, deficiency of micronutrients and low intake of bioactive compounds could cause severe health consequences in elderly people.
Fruits and vegetables are particularly rich in bioactive molecules, minerals, and vitamins. To exert their positive effects on health, these molecules must be released from the food matrix and absorbed by the human body (i.e. bioaccessibility and bioavailability). Bioaccessibility is defined as the amount of a compound that is released from the food matrix through the gastrointestinal tract and is available for absorption,137 whereas bioavailability represents the fraction of ingested bioactive compounds that end up in the systemic circulation reaching the active site in an active form and are metabolised.138 It is well recognised that the bioaccessibility of a compound is much more relevant than its content within the food matrix. Even if food processing can degrade bioactive compounds, depending on the product and processing conditions, it can also be exploited to induce structural modifications able to increase bioaccessibility and thus bioactivity of selected components. Besides the conventional heat treatments, non-thermal technologies (Table 3) have been proposed as alternatives to conventional thermal processing to improve the health-related properties of plant-based foods. Application of non-thermal process is expected not only to reduce the thermal damage but also to modify the structure in tissues, finally improving the bioactive compound's bioaccessibility. The application of non-thermal technologies, such as PEF, HPH, or US, promotes the leakage of cell content increasing the bioactive bioaccessibility.139 For instance, Alongi et al. (2019)140 reported that the phenolic content of apple juice subjected to thermal or ultrasound pasteurization was higher than that of the non-thermally treated one, due to the release of monomers and dimers upon heat induced hydrolysis of heat-labile molecule as well as of phenolic compounds from the vegetable matrix upon tissue disruption. Similarly, an increase in bioaccessibility of carotenoids, vitamin C, and phenolic compounds contained in fruit juices was reported after PEF and US treatments141–143
In addition, designing foods enriched with vitamins, minerals, and bioactive components might represent an additional way to face micronutrients deficiency in the elderly as well as reduce the burden of pills. There are several technological limitations in producing food products enriched with micronutrients and bioactive compounds.144–146 These include (a) degradation or undesirable changes of the added compounds during processing and storage of food products; (b) non-uniform distribution of micronutrients throughout the food because of the minor amounts added to the product; (c) lipophilic character of several natural bioactive compounds (e.g. vitamin A and D, carotenoids, curcuminoids, etc.), which may limit their direct addition to the food due to low solubility, crystallinity, chemical and biochemical instability.146
These issues can be overcome by the design of a proper structure embedding the bioactive molecules that is able to protect them during food processing, storage, and further transit in the gastrointestinal tract while improving their bioavailability.147,148 The most studied and high performing protective and delivery systems for oral administration of bioactive lipophilic compounds are lipid-based nanoparticles, comprising the wide category of emulsions (e.g. nanoemulsions, double emulsions, emulsion gels, particle filled emulsions, high internal phase emulsions), liposomes, solid lipid nanoparticles, among others.144,147 In these systems, the liposoluble bioactive molecules are generally embedded in the lipid core. The engineering of the particle characteristics (e.g. particle dimensions, lipid core physical state, structure of the shell, interfacial properties) allows improving the bioaccessibility and bioavailability of selected bioactive molecules.146–148,149 At the current stage of knowledge, the rational and reliable design of bioactive delivery systems goes through a deep understanding of the relationships among food nano-micro-macro structure and (i) food quality and sensory characteristics, (ii) bioactive stability as a consequence of processing and storage conditions as well as (iii) bioactive bioavailability in the human body. Only by integrating quantitative structure-bioavailability knowledge it would be possible to beget new tools for developing health-value-added foods.
4.8 Delivering of probiotic bacteria
As already pointed out, the elderly can benefit from dietary supplementation with probiotic bacteria.
To be effective in delivering health benefits, probiotic bacteria must arrive to the target host site in adequate amount: today, it is well recognized that a viability higher than 106–107 UFC g−1 is needed to promote the claimed health benefits.150 Their viability in food is strongly endangered by various factors, including environmental features of the food matrix, processing treatments applied, possible presence of starter microorganisms (in the case of fermented food), conditions and duration of storage, as well as gastrointestinal transit.151 Moreover, during the gastrointestinal transit, the strongly acidic conditions of the stomach, as well as the presence of enzymes and bile salts, are expected to significantly reduce their survival.152,153
Traditionally, probiotic microorganisms have been added to foods as free cells. However, to maintain probiotic bacteria viability during food processing, storage, and gastrointestinal transit, probiotic encapsulation has been widely applied.154–156 In particular, probiotic cells are segregated from the surrounding environment by embedding them into a shell of a selected material. In this context, probiotics are firstly dispersed in liquid matrices containing a structuring biopolymer or a combination of polymers and then the water is removed mainly by spray-drying or lyophilisation. A plethora of encapsulation strategies based on food biopolymer structuring capacity has been proposed.154–156 Polysaccharides, such as k-carrageenan, alginate, and resistant starch beads, or proteins, mainly whey proteins and caseins, are widely used because they are inexpensive, non-toxic, and biocompatible.155,157–160
An alternative strategy to introduce live cells of probiotics into foods is the use of structured emulsions.161–163 Gels appears particularly promising in this attempt due to their compatibility with the food matrix and the ability to protect bacteria against environmental stresses. For instance, Zhuang et al.163 used bigels made of soy lecithin-stearic acid oleogel in emulsion with a whey protein hydrogel to deliver the probiotic Lactobacillus acidophilus and Bifidobacterium lactis in yoghurt. Similarly, Calligaris et al. (2018)113 and Melchior et al. (2022)164 exploited monoglyceride structured emulsions to deliver Lactobacillus rhamnosus strain into ice-cream and ricotta cheese. The structure of these systems was able to protect the probiotic bacteria during food processing, storage, and further digestion.
It can be concluded that the advantages of consumption of foods rich in probiotics for the elderly are well recognised and their consumption should be favoured. Today, mainly dairy derivatives are available for the high compatibility of these products with probiotic survival. However, the enlargement of the available probiotic food categories could favour the consumption by the elderly. In this attempt delivery systems with a protective structure for bacteria should be designed and tested.
4.9 Favouring water consumption
The intake of water by the elderly could be critical causing inadequate hydration, which is associated with many hydration-related medical conditions.8 The elderly population is at increased risk for dehydration for a number of reasons, including decrease in the thirst sensation, decrease in renal perfusion, altered sensitivity to antidiuretic hormone and neurocognitive deficits.8 Moreover, impaired oral processing could compromise the swallowing of fluid foods.
Food structure design approach could also be useful for encouraging the ingestion of significant amounts of water thus maintaining an optimal fluid level. In particular, the conversion of fluid foods, such as water itself, beverages, soups, and milk, into viscous to soft materials could favour their consumption. For instance, the use of hydrocolloids to increase product viscosity or to obtain hydrogels could allow to generate materials with a viscous soft texture delivering high water contents. The possible addition of other nutrients/bioactive molecules as well as flavourings could increase the functionalities of the product.
5 Research needs and future trends
Despite the growing interest in developing foods specifically targeting the elderly needs, one of the main hurdles is represented by the limited tools to verify their effective functionality. Today, an ever increasing number of papers deals with food behaviour during digestion and further utilization by the human body. Many of these studies focused on linking food structure and functionality in the gastrointestinal tract. This issue is faced by internationally recognised methods for simulation of human oral and digestion processing up to the colon and microbiota functions.165 However, to date, there are no standardized protocols for the elderly13 and the literature on this topic is definitively reduced. Some pioneer studies have developed the first dynamic in vitro digestion model targeted for the elderly (70–75 years old) and dedicated to the study of protein digestibility.166 More recently, static in vitro digestion methods have been developed to mimic elderly conditions once again for the study of protein digestibility.167–169 For other nutrients, micronutrients, and bioactive compounds, there is a general lack of studies on their biological fate under elderly gastro-intestinal conditions, rendering urgent the development of dedicated research efforts. It is likely that the possibility to simulate elderly gastro-intestinal conditions would open the possibility to design foods successfully tailored for the specific needs of the ageing population.
Additionally, in the field of food structure, it is highly demanded the reinforcement of efforts in developing quantitative findings on the structure–function relation, covering the understanding of the entire lifespan of food considering the different structuring/de-structuring events from raw material up to the fate of the complex food product in the human body. To this aim novel and even more sophisticated analytical techniques are highly demanded.
Finally, reading the literature on food for the elderly, there is a general lack of targeted studies on sensory acceptance of food designed for the elderly. This aspect is particularly critical considering that elderly people are often looking for traditional foods without specific and novel tasting experiences. Therefore, in designing foods for the elderly, this aspect appears of fundamental importance.
6 Conclusions
Ageing is an inevitable and progressive process, but, if faced in an efficient way, living longer in a healthy status could be an opportunity for individuals. Today, there is increasing awareness that an appropriate and balanced diet may prevent the insurgence of diet-related pathologies and/or improve well-being and life expectancy, also reflecting on the ageing process. As discussed, many physiological changes occur with ageing impacting food consumption. Thus, there is the need to develop a new food category specifically designed for the needs of the elderly. As other food categories, such as baby foods, foods for the elderly should be engineered to meet the peculiar needs of this group of population. To this aim, the application of a food structure design approach could be particularly profitable being food structure responsible to the final functionalities of food products. Thus, the understanding of the relationships between food structure and related functionalities, in food as well as in the human body, can play a determining role in developing tailored foods for the elderly.
Notably, the described food structure design strategies to develop the next generation of food for the elderly are only one piece of the puzzle to tackle the multivariate challenges associated with population ageing. Coordinated interventions from different disciplines are needed to tackle elderly frailty from different points of view. In this context, it appears pivotal not only to boost an integrated collaboration of experts in medicine, nutrition, and food science, but also include the contribution of other specialists such as in sociology, psychology, and education. Only working all together, it would be possible to face elderly-related issues favouring active and healthy ageing.
Author contributions
All the authors contributed to the conceptualization and design of the proposed approach and the writing of the manuscript.
Conflicts of interest
There are no conflicts to declare.
Acknowledgements
This work was undertaken as a part of the project “Personalized Health management of physical, mental and social frailty in the elderly” granted by the Fondazione Friuli (Italy).
References
-
United Nations, Active Ageing Index Analytical Report, 2019 Search PubMed.
-
WHO, World report on ageing and health, 2015 Search PubMed.
-
European Commission, The 2021 Ageing Report. Economic & Budgetary Projections for the EU Member States (2019–2070), 2021, vol. 148 Search PubMed.
- T. K. Thorning, H. C. Bertram, J. P. Bonjour, L. De Groot, D. Dupont, E. Feeney, R. Ipsen, J. M. Lecerf, A. Mackie, M. C. McKinley, M. C. Michalski, D. Rémond, U. Risérus, S. S. Soedamah-Muthu, T. Tholstrup, C. Weaver, A. Astrup and I. Givens, Whole dairy matrix or single nutrients in assessment of health effects: Current evidence and knowledge gaps, Am. J. Clin. Nutr., 2017, 105, 1033–1045 CrossRef CAS PubMed.
- Q. Guo, A. Ye, H. Singh and D. Rousseau, Destructuring and restructuring of foods during gastric digestion, Compr. Rev. Food Sci. Food Saf., 2020, 19, 1658–1679 CrossRef PubMed.
- W. F. Nieuwenhuizen, H. Weenen, P. Rigby and M. M. Hetherington, Older adults and patients in need of nutritional support: Review of current treatment options and factors influencing nutritional intake, Clin. Nutr., 2010, 29, 160–169 CrossRef PubMed.
- A. Wysokiński, T. Sobów, I. Kłoszewska and T. Kostka, Mechanisms of the anorexia of aging—a review, Age, 2015, 37, 1–14 CrossRef PubMed.
- D. Picetti, S. Foster, A. K. Pangle, A. Schrader, M. George, J. Y. Wei and G. Azhar, Hydration health literacy in the elderly, Nutr. Healthy Aging, 2017, 4, 227–237 Search PubMed.
- L. Laguna and J. Chen, The eating capability: Constituents and assessments, Food Qual. Prefer., 2016, 48, 345–358 CrossRef.
- D. A. Nowak and J. Hermsdörfer, Selective deficits of grip force control during object manipulation in patients with reduced sensibility of the grasping digits, Neurosci. Res., 2003, 47, 65–72 CrossRef PubMed.
- B. Winder, K. Ridgway, A. Nelson and J. Baldwin, Food and drink packaging: Who is complaining and who should be complaining, Appl. Ergon., 2002, 33, 433–438 CrossRef PubMed.
- N. Kshetrimayum, C. V. K. Reddy, S. Siddhana, M. Manjunath, S. Rudraswamy and S. Sulavai, Oral health-related quality of life and nutritional status of institutionalized elderly population aged 60 years and above in Mysore City, India, Gerodontology, 2013, 30, 119–125 CrossRef PubMed.
- A. Mackie, A. I. Mulet-Cabero and A. Torcello-Gomez, Simulating human digestion: Developing our knowledge to create healthier and more sustainable foods, Food Funct., 2020, 11, 9397–9431 RSC.
- D. Rémond, D. R. Shahar, D. Gille, P. Pinto, J. Kachal, M. A. Peyron, C. N. Dos Santos, B. Walther, A. Bordoni, D. Dupont, L. Tomás-Cobos and G. Vergères, Understanding the gastrointestinal tract of the elderly to develop dietary solutions that prevent malnutrition, Oncotarget, 2015, 6, 13858–13898 CrossRef PubMed.
- S. Lee, K. Jo, H. G. Jeong, Y. S. Choi, H. I. Yong and S. Jung, Understanding protein digestion in infants and the elderly: Current in vitro digestion models, Crit. Rev. Food Sci. Nutr., 2021, 1–18 CrossRef PubMed.
- P. W. O'Toole and M. J. Claesson, Gut microbiota: Changes throughout the lifespan from infancy to elderly, Int. Dairy J., 2010, 20, 281–291 CrossRef.
-
M. G. Gareau, Cognitive Function and the Microbiome. In: International Review of Neurobiology, International Review of Neurobiology, Academic Press, 2016, vol. 131, pp. 227–246 Search PubMed.
- J. E. Beilharz, N. O. Kaakoush, J. Maniam and M. J. Morris, Cafeteria diet and probiotic therapy: Cross talk among memory, neuroplasticity, serotonin receptors and gut microbiota in the rat, Mol. Psychiatry, 2018, 23, 351–361 CrossRef CAS PubMed.
- M. Komanduri, S. Gondalia, A. Scholey and C. Stough, The microbiome and cognitive aging: a review of mechanisms, Psychopharmacology, 2019, 236, 1559–1571 CrossRef CAS PubMed.
- N. J. Cox, K. Ibrahim, A. A. Sayer, S. M. Robinson and H. C. Roberts, Assessment and treatment of the anorexia of aging: A systematic review, Nutrients, 2019, 11, 1–20 CrossRef PubMed.
- E. Agarwal, M. Miller, A. Yaxley and E. Isenring, Malnutrition in the elderly: A narrative review, Maturitas, 2013, 76, 296–302 CrossRef CAS PubMed.
- S. Brownie, Why are elderly individuals at risk of nutritional deficiency?, Int. J. Nurs. Pract., 2006, 12, 110–118 CrossRef PubMed.
- M. Assad-Bustillos, C. Tournier, C. Septier, G. Della Valle and G. Feron, Relationships of oral comfort perception and bolus properties in the elderly with salivary flow rate and oral health status for two soft cereal foods, Food Res. Int., 2019, 118, 13–21 CrossRef CAS PubMed.
- J. A. Y. Cichero, Adjustment of Food Textural Properties for Elderly Patients, J. Texture Stud., 2016, 47, 277–283 CrossRef.
- K. Field and L. M. Duizer, Food Sensory Properties and the Older Adult, J. Texture Stud., 2016, 47, 266–276 CrossRef.
- L. Laguna, M. M. Hetherington, J. Chen, G. Artigas and A. Sarkar, Measuring eating capability, liking and difficulty perception of older adults: A textural consideration, Food Qual. Prefer., 2016, 53, 47–56 CrossRef.
- H. S. Park, D. K. Kim, S. Y. Lee and K. H. Park, The effect of aging on mastication and swallowing parameters according to the hardness change of solid food, J. Texture Stud., 2017, 48, 362–369 CrossRef PubMed.
- A. Sarkar, Oral processing in elderly: Understanding eating capability to drive future food texture modifications, Proc. Nutr. Soc., 2019, 78, 329–339 CrossRef PubMed.
- C. Schwartz, M. Vandenberghe-Descamps, C. Sulmont-Rossé, C. Tournier and G. Feron, Behavioral and physiological determinants of food choice and consumption at sensitive periods of the life span, a focus on infants and elderly, Innovative Food Sci. Emerging Technol., 2018, 46, 91–106 CrossRef.
- C. M. Steele, W. A. Alsanei, S. Ayanikalath, C. E. A. Barbon, J. Chen, J. A. Y. Cichero, K. Coutts, R. O. Dantas, J. Duivestein, L. Giosa, B. Hanson, P. Lam, C. Lecko, C. Leigh, A. Nagy, A. M. Namasivayam, W. V. Nascimento, I. Odendaal, C. H. Smith and H. Wang, The Influence of Food Texture and Liquid Consistency Modification on Swallowing Physiology and Function: A Systematic Review, Dysphagia, 2015, 30, 2–26 CrossRef PubMed.
- L. J. Pereira and A. van der Bilt, The influence of oral processing, food perception and social aspects on food consumption: a review, J. Oral Rehabil., 2016, 43, 630–648 CrossRef CAS PubMed.
- M. Vandenberghe-Descamps, H. Labouré, A. Prot, C. Septier, C. Tournier, G. Feron and C. Sulmont-Rossé, Salivary Flow Decreases in Healthy Elderly People Independently of Dental Status and Drug Intake, J. Texture Stud., 2016, 47, 353–360 CrossRef.
- A. Forster, N. Samaras, G. Gold and D. Samaras, Oropharyngeal dysphagia in older adults: A review, Eur. Geriatr. Med., 2011, 2, 356–362 CrossRef.
- M. Boland, Human digestion - a processing perspective, J. Sci. Food Agric., 2016, 96, 2275–2283 CrossRef CAS PubMed.
- T. L. Russell, R. R. Berardi, J. L. Barnett, L. C. Dermentzoglou, K. M. Jarvenpaa, S. P. Schmaltz and J. B. Dressman, Upper Gastrointestinal pH in Seventy-Nine Healthy, Elderly, North American Men and Women, Pharm. Res., 1993, 10, 187–196 CrossRef CAS PubMed.
- J. E. Morley, Decreased Food
Intake With Aging, J. Gerontol., Ser. A, 2001, 56, 81–88 CrossRef PubMed.
- B. Vellas, D. Balas, J. Moreau, M. Bouisson, F. Senegas-Balas, M. Guidet and A. Ribet, Exocrine pancreatic secretion in the elderly, Int. J. Pancreatol., 1988, 3, 497–502 CrossRef CAS PubMed.
- R. I. Clark and D. W. Walker, Role of gut microbiota in aging-related health decline: insights from invertebrate models, Cell. Mol. Life Sci., 2018, 75, 93–101 CrossRef CAS PubMed.
- J. M. Aguilera, Food product engineering: building the right structures, J. Sci. Food Agric., 2006, 86, 1147–1155 CrossRef CAS.
- A. Mackie, Food: more than the sum of its parts, Curr. Opin. Food Sci., 2017, 16, 120–124 CrossRef.
- J. M. Aguilera, The food matrix: implications in processing, nutrition and health, Crit. Rev. Food Sci. Nutr., 2019, 59, 3612–3629 CrossRef CAS PubMed.
-
E. van der Linden, Assembly of Structures in Foods in Food Materials Science – Principles and Practice, ed. J. M. Aguilera and P. J. Lillford, Springer New York, 2008, pp. 145–167 Search PubMed.
- J. M. Aguilera, Why food microstructure?, J. Food Eng., 2005, 67, 3–11 CrossRef.
- D. Dupont, S. Le Feunteun, S. Marze and I. Souchon, Structuring food to control its disintegration in the gastrointestinal tract and optimize nutrient bioavailability, Innovative Food Sci. Emerging Technol., 2018, 46, 83–90 CrossRef CAS.
- M. Hiolle, V. Lechevalier, J. Floury, N. Boulier-Monthéan, C. Prioul, D. Dupont and F. Nau, In vitro digestion of complex foods: How microstructure influences food disintegration and micronutrient bioaccessibility, Food Res. Int., 2020, 128, 108817 CrossRef CAS PubMed.
- J. Norton, Y. Gonzalez Espinosa, R. L. Watson, F. Spyropoulos and I. Norton, Functional food microstructures for macronutrient release and delivery, Food Funct., 2015, 6, 663–678 RSC.
- G. Somaratne, M. J. Ferrua, A. Ye, F. Nau, J. Floury, D. Dupont and J. Singh, Food material properties as determining factors in nutrient release during human gastric digestion: a review, Crit. Rev. Food Sci. Nutr., 2020, 1–17 Search PubMed.
- M. Alongi and M. Anese, Re-thinking functional food development through a holistic approach, J. Funct. Foods, 2021, 81, 104466 CrossRef.
- I. Heertje, Structure and function of food products: A, review, Food Struct., 2014, 1, 3–23 CrossRef.
- R. N. Pereira and A. A. Vicente, Environmental impact of novel thermal and non-thermal technologies in food processing, Food Res. Int., 2010, 43, 1936–1943 CrossRef.
- L. Picart-Palmade, C. Cunault, D. Chevalier-Lucia, M. P. Belleville and S. Marchesseau, Potentialities and limits of some non-thermal technologies to improve sustainability of food processing, Front. Nutr., 2019, 5, 1–18 Search PubMed.
- D. Knorr and H. Watzke, Food processing at a crossroad, Front. Nutr., 2019, 6, 1–8 CrossRef PubMed.
- D. J. McClements, Recent progress in hydrogel delivery systems for improving nutraceutical bioavailability, Food Hydrocolloids, 2017, 68, 238–245 CrossRef CAS.
- Y. Lu, L. Mao, Z. Hou, S. Miao and Y. Gao, Development of Emulsion Gels for the Delivery of Functional Food Ingredients: from Structure to Functionality, Food Eng. Rev., 2019, 11, 245–258 CrossRef.
- A. R. Patel and K. Dewettinck, Edible oil structuring: An overview and recent updates, Food Funct., 2016, 7, 20–29 RSC.
- L. Mao, Y. Lu, M. Cui, S. Miao and Y. Gao, Design of gel structures in water and oil phases for improved delivery of bioactive food ingredients, Crit. Rev. Food Sci. Nutr., 2020, 60, 1651–1666 CrossRef CAS PubMed.
- Y. Lu, Y. Zhang, F. Yuan, Y. Gao and L. Mao, Emulsion gels with different proteins at the interface: Structures and delivery functionality, Food Hydrocolloids, 2021, 116, 106637 CrossRef CAS.
- C. Tan and D. J. McClements, Application of advanced emulsion technology in the food industry: A review and critical evaluation, Foods, 2021, 10, 1–25 Search PubMed.
- L. Manzocco, K. S. Mikkonen and C. A. García-González, Aerogels as porous structures for food applications: Smart ingredients and novel packaging materials, Food Struct., 2021, 28, 100188 CrossRef CAS.
- S. L. Turgeon and L. E. Rioux, Food matrix impact on macronutrients nutritional properties, Food Hydrocolloids, 2011, 25, 1915–1924 CrossRef CAS.
- H. Singh, A. Ye and M. J. Ferrua, Aspects of food structures in the digestive tract, Curr. Opin. Food Sci., 2015, 3, 85–93 CrossRef.
- J. Chen, Food oral processing: Some important underpinning principles of eating and sensory perception, Food Struct., 2014, 1, 91–105 CrossRef.
- M. G. Aguayo-Mendoza, E. C. Ketel, E. van der Linden, C. G. Forde, B. Piqueras-Fiszman and M. Stieger, Oral processing behavior of drinkable, spoonable and chewable foods is primarily determined by rheological and mechanical food properties, Food Qual. Prefer., 2019, 71, 87–95 CrossRef.
- M. S. M. Wee, A. T. Goh, M. Stieger and C. G. Forde, Correlation of instrumental texture properties from textural profile analysis (TPA) with eating behaviours and macronutrient composition for a wide range of solid foods, Food Funct., 2018, 9, 5301–5312 RSC.
- J. Chen, Food oral processing-A review, Food Hydrocolloids, 2009, 23, 1–25 CrossRef CAS.
- Z. Guo, Z. Huang, Y. Guo, B. Li, W. Yu, L. Zhou, L. Jiang, F. Teng and Z. Wang, Effects of high-pressure homogenization on structural and emulsifying properties of thermally soluble aggregated kidney bean (Phaseolus vulgaris L.) proteins, Food Hydrocolloids, 2021, 119, 106835 CrossRef CAS.
- A. I. Mulet-Cabero, A. R. Mackie, A. Brodkorb and P. J. Wilde, Dairy structures and physiological responses: a matter of gastric digestion, Crit. Rev. Food Sci. Nutr., 2020, 60, 3737–3752 CrossRef CAS PubMed.
- L. Laguna, A. Sarkar, G. Artigas and J. Chen, A quantitative assessment of the eating capability in the elderly individuals, Physiol. Behav., 2015, 147, 274–281 CrossRef CAS PubMed.
- J. E. Norton, G. A. Wallis, F. Spyropoulos, P. J. Lillford and I. T. Norton, Designing food structures for nutrition and health benefits, Annu. Rev. Food Sci. Technol., 2014, 5, 177–195 CrossRef CAS PubMed.
- M. K. Lal, B. Singh, S. Sharma, M. P. Singh and A. Kumar, Glycemic index of starchy crops and factors affecting its digestibility: A review, Trends Food Sci. Technol., 2021, 111, 741–755 CrossRef CAS.
- J. Parada and J. L. Santos, Interactions between Starch, Lipids, and Proteins in Foods: Microstructure Control for Glycemic Response Modulation, Crit. Rev. Food Sci. Nutr., 2016, 56, 2362–2369 CrossRef CAS PubMed.
- J. Singh, L. Kaur and H. Singh, Food Microstructure and Starch Digestion, Adv. Food Nutr. Res., 2013, 70, 137–179 CAS.
- M. Alongi, S. Calligaris and M. Anese, Fat concentration and high-pressure homogenization affect chlorogenic acid bioaccessibility and α-glucosidase inhibitory capacity of milk-based coffee beverages, J. Funct. Foods, 2019, 58, 130–137 CrossRef CAS.
- J. Singh, A. Dartois and L. Kaur, Starch digestibility in food matrix: a review, Trends Food Sci. Technol., 2010, 21, 168–180 CrossRef CAS.
- D. Peressini, S. Melchior, M. Berlese and S. Calligaris, Application of high-pressure homogenization to tailor the functionalities of native wheat starch, J. Sci. Food Agric., 2020, 1–8 Search PubMed.
- F. Zhu, Modifications of starch by electric field based techniques, Trends Food Sci. Technol., 2018, 75, 158–169 CrossRef CAS.
- F. Zhu, Plasma modification of starch, Food Chem., 2017, 232, 1–7 CrossRef PubMed.
- F. Zhu, Impact of ultrasound on structure, physicochemical properties, modifications, and applications of starch, Trends Food Sci. Technol., 2015, 43, 1–17 CrossRef CAS.
- C. Chi, X. Li, S. Huang, L. Chen, Y. Zhang, L. Li and S. Miao, Basic principles in starch multi-scale structuration to mitigate digestibility: A review, Trends Food Sci. Technol., 2021, 109, 154–168 CrossRef CAS.
- N. Pellegrini, E. Vittadini and V. Fogliano, Designing food structure to slow down digestion in starch-rich products, Curr. Opin. Food Sci., 2020, 32, 50–57 CrossRef.
- A. Yuris, K. K. T. Goh, A. K. Hardacre and L. Matia-Merino, Understanding the interaction between wheat starch and Mesona chinensis polysaccharide, LWT – Food Sci. Technol., 2017, 84, 212–221 CrossRef CAS.
- J. Holm, I. Björck, S. Ostrowska, A.-C. Eliasson, N.-G. Asp, K. Larsson and I. Lundquist, Digestibility of Amylose–Lipid Complexes in vitro and in vivo, Starch – Stärke, 1983, 35, 294–297 CrossRef CAS.
- C. Yang, F. Zhong, H. Douglas Goff and Y. Li, Study on starch-protein interactions and their effects on physicochemical and digestible properties of the blends, Food Chem., 2019, 280, 51–58 CrossRef CAS PubMed.
- D. J. A. Jenkins, M. J. Thorne, T. M. S. Wolever, A. V. Rao and L. U. Thompson, The effect of starch-protein interaction in wheat on the glycemic response and rate of in vitro digestion, Am. J. Clin. Nutr., 1987, 45, 946–951 CrossRef CAS PubMed.
- H. G. M. Liljeberg and I. M. E. Björk, Delayed gastric emptying rate as a potential mechanism for lowered glycemia after eating sourdough bread: Studies in humans and rats using test products with added organic acids or an organic salt, Am. J. Clin. Nutr., 1996, 64, 886–893 CrossRef CAS PubMed.
- H. Liljeberg and I. Björck, Delayed gastric emptying rate may explain improved glycaemia in healthy subjects to a starchy meal with added vinegar, Eur. J. Clin. Nutr., 1998, 52, 368–371 CrossRef CAS PubMed.
- E. Barber, M. J. Houghton and G. Williamson, Flavonoids as human intestinal α-glucosidase inhibitors, Foods, 2021, 10(1939), 1–22 Search PubMed.
- B. Grube, P. Chong, K. Lau and H. Orzechowski, A natural fiber complex reduces body weight in the overweight and obese: A double–blind, randomized, placebo–controlled study, Obesity, 2013, 21, 58–64 CrossRef CAS PubMed.
- M. Havrlentová, Z. Petruláková, A. Burgárová, F. Gago, A. Hlinková and E. Šturdík, Cereal β-glucans and their significance for the preparation of functional foods - A review, Czech J. Food Sci., 2011, 29, 1–14 CrossRef.
- L. Laguna, A. Sarkar, G. Artigas and J. Chen, A quantitative assessment of the eating capability in the elderly individuals, Physiol. Behav., 2015, 147, 274–281 CrossRef CAS PubMed.
- R. J. Redgwell and M. Fischer, Dietary fiber as a versatile food component: An industrial perspective, Mol. Nutr. Food Res., 2005, 49, 521–535 CrossRef PubMed.
- D. Dhingra, M. Michael, H. Rajput and R. T. Patil, Dietary fibre in foods: A review, J. Food Sci. Technol., 2012, 49, 255–266 CrossRef CAS PubMed.
- E. Neyraud, J. Prinz and E. Dransfield, NaCl and sugar release, salivation and taste during mastication of salted chewing gum, Physiol. Behav., 2003, 79, 731–737 CrossRef CAS PubMed.
- G. Sala and M. Stieger, Time to first fracture affects sweetness of gels, Food Hydrocolloids, 2013, 30, 73–81 CrossRef CAS.
- A. C. Mosca, F. van de Velde, J. H. F. Bult, M. A. J. S. van Boekel and M. Stieger, Taste enhancement in food gels: Effect of fracture properties on oral breakdown, bolus formation and sweetness intensity, Food Hydrocolloids, 2015, 43, 794–802 CrossRef CAS.
- Z. Wang, K. Yang, T. Brenner, H. Kikuzaki and K. Nishinari, The influence of
agar gel texture on sucrose release, Food Hydrocolloids, 2014, 36, 196–203 CrossRef CAS.
- J. D. Rios-Mera, M. M. Selani, I. Patinho, E. Saldaña and C. J. Contreras-Castillo, Modification of NaCl structure as a sodium reduction strategy in meat products: An overview, Meat Sci., 2021, 174, 108417 CrossRef CAS PubMed.
- G. Sala, M. Stieger and F. van de Velde, Serum release boosts sweetness intensity in gels, Food Hydrocolloids, 2010, 24, 494–501 CrossRef CAS.
- A. I. Blake and A. G. Marangoni, Factors affecting the rheological properties of a structured cellular solid used as a fat mimetic, Food Res. Int., 2015, 74, 284–293 CrossRef CAS PubMed.
- F. C. Wang, A. J. Gravelle, A. I. Blake and A. G. Marangoni, Novel trans fat replacement strategies, Curr. Opin. Food Sci., 2016, 7, 27–34 CrossRef.
- S. Du, J. Jin, W. Fang and Q. Su, Does fish oil have an anti-obesity effect in overweight/obese adults? A meta-analysis of randomized controlled trials, PLoS One, 2015, 10, 1–20 Search PubMed.
- J. Orsavova, L. Misurcova, J. V. Ambrozova, R. Vicha and J. Mlcek, Fatty acids composition of vegetable oils and its contribution to dietary energy intake and dependence of cardiovascular mortality on dietary intake of fatty acids, Int. J. Mol. Sci., 2015, 16, 12871–12890 CrossRef CAS PubMed.
- E. D. Co and A. G. Marangoni, Organogels: An alternative edible oil-structuring method, J. Am. Oil Chem. Soc., 2012, 89, 749–780 CrossRef.
- A. Singh, F. I. Auzanneau and M. A. Rogers, Advances in edible oleogel technologies – A decade in review, Food Res. Int., 2017, 97, 307–317 CrossRef CAS PubMed.
- G. Fayaz, S. A. H. Goli, M. Kadivar, F. Valoppi, L. Barba, S. Calligaris and M. C. Nicoli, Potential application of pomegranate seed oil oleogels based on monoglycerides, beeswax and propolis wax as partial substitutes of palm oil in functional chocolate spread, LWT, 2017, 86, 523–529 CrossRef CAS.
- C. M. O'Sullivan, M. Davidovich-Pinhas, A. J. Wright, S. Barbut and A. G. Marangoni, Ethylcellulose oleogels for lipophilic bioactive delivery-effect of oleogelation on: In vitro bioaccessibility and stability of beta-carotene, Food Funct., 2017, 8, 1438–1451 RSC.
- A. Ashkar, S. Laufer, J. Rosen-Kligvasser, U. Lesmes and M. Davidovich-Pinhas, Impact of different oil gelators and oleogelation mechanisms on digestive lipolysis of canola oil oleogels, Food Hydrocolloids, 2019, 97, 105218 CrossRef CAS.
- S. Y. Tan, E. W.-Y. Peh, A. G. Marangoni and C. J. Henry, Effects of liquid oil vs. oleogel co-ingested with a carbohydrate-rich meal on human blood triglycerides, glucose, insulin and appetite, Food Funct., 2017, 8, 241–249 RSC.
- S. Y. Tan, E. Peh, P. C. Siow, A. G. Marangoni and C. J. Henry, Effects of the physical-form and the degree-of-saturation of oil on postprandial plasma triglycerides, glycemia and appetite of healthy Chinese adults, Food Funct., 2017, 8, 4433–4440 RSC.
- S. Y. Tan, E. Peh, E. Lau, A. G. Marangoni and C. J. Henry, Physical form of dietary fat alters postprandial substrate utilization and glycemic response in healthy Chinese men, J. Nutr., 2017, 147, 1138–1144 CrossRef CAS PubMed.
- S. Calligaris, M. Alongi, P. Lucci and M. Anese, Effect of different oleogelators on lipolysis and curcuminoid bioaccessibility upon in vitro digestion of sunflower oil oleogels, Food Chem., 2020, 314, 126146 CrossRef CAS PubMed.
- S. Calligaris, L. Manzocco, F. Valoppi and M. C. Nicoli, Effect of palm oil replacement with monoglyceride organogel and hydrogel on sweet bread properties, Food Res. Int., 2013, 51, 596–602 CrossRef CAS.
- S. Calligaris, M. Marino, M. Maifreni and N. Innocente, Potential application of monoglyceride structured emulsions as delivery systems of probiotic bacteria in reduced saturated fat ice cream, LWT, 2018, 96, 329–334 CrossRef CAS.
- T. Pintado, I. Muñoz-González, M. Salvador, C. Ruiz-Capillas and A. M. Herrero, Phenolic compounds in emulsion gel-based delivery systems applied as animal fat replacers in frankfurters: Physico-chemical, structural and microbiological approach, Food Chem., 2021, 340, 128095 CrossRef CAS PubMed.
- A. M. Herrero and C. Ruiz-Capillas, Novel lipid materials based on gelling procedures as fat analogues in the development of healthier meat products, Curr. Opin. Food Sci., 2021, 39, 1–6 CrossRef.
- C. de S. Paglarini, G. de Figueiredo Furtado, A. R. Honório, L. Mokarzel, V. A. da Silva Vidal, A. P. B. Ribeiro, R. L. Cunha and M. A. R. Pollonio, Functional emulsion gels as pork back fat replacers in Bologna sausage, Food Struct., 2019, 20, 100105 CrossRef.
- C. de S. Paglarini, G. de F. Furtado, J. P. Biachi, V. A. S. Vidal, S. Martini, M. B. S. Forte, R. L. Cunha and M. A. R. Pollonio, Functional emulsion gels with potential application in meat products., J. Food Eng., 2018, 222, 29–37 CrossRef CAS.
- Q. Guo, A. Ye, N. Bellissimo, H. Singh and D. Rousseau, Modulating fat digestion through food structure design, Prog. Lipid Res., 2017, 68, 109–118 CrossRef CAS PubMed.
- L. Gu, Y. Su, Z. Zhang, B. Zheng, R. Zhang, D. J. McClements and Y. J. Yang, Modulation of Lipid Digestion Profiles Using Filled Egg White Protein Microgels, Agric. Food Chem., 2017, 65, 6919–6928 CrossRef CAS PubMed.
- M. Alongi, P. Lucci, M. L. Clodoveo, F. P. Schena and S. Calligaris, Oleogelation of extra virgin olive oil by different oleogelators affects the physical properties and the stability of bioactive compounds, Food Chem., 2022, 368, 130779 CrossRef CAS PubMed.
- K. Gutiérrez-luna, D. Ansorena and I. Astiasarán, Use of hydrocolloids and vegetable oils for the formulation of a butter replacer: Optimization and oxidative stability, LWT, 2022, 153, 112538 CrossRef.
- S. Ter Borg, S. Verlaan, D. M. Mijnarends, J. M. G. A. Schols, L. C. P. G. M. De Groot and Y. C. Luiking, Macronutrient Intake and Inadequacies of Community-Dwelling Older Adults, a Systematic Review, Ann. Nutr. Metab., 2015, 66, 242–255 CrossRef CAS PubMed.
- S. H. M. Gorissen, J. J. R. Crombag, J. M. G. Senden, W. A. H. Waterval, J. Bierau, L. B. Verdijk and L. J. C. van Loon, Protein content and amino acid composition of commercially available plant-based protein isolates, Amino Acids, 2018, 50, 1685–1695 CrossRef CAS PubMed.
- F. U. Akharume, R. E. Aluko and A. A. Adedeji, Modification of plant proteins for improved functionality: A review, Compr. Rev. Food Sci. Food Saf., 2021, 20, 198–224 CrossRef CAS PubMed.
- A. G. A. Sá, Y. M. F. Moreno and B. A. M. Carciofi, Food processing for the improvement of plant proteins digestibility, Crit. Rev. Food Sci. Nutr., 2019, 60, 3367–3386 CrossRef PubMed.
- E. Capuano, T. Oliviero, V. Fogliano and N. Pellegrini, Role of the food matrix and digestion on calculation of the actual energy content of food, Nutr. Rev., 2018, 76, 274–289 CrossRef PubMed.
- L. H. Fasolin, R. N. Pereira, A. C. Pinheiro, J. T. Martins, C. C. P. Andrade, O. L. Ramos and A. A. Vicente, Emergent food proteins – Towards sustainability, health and innovation, Food Res. Int., 2019, 125, 108586 CrossRef CAS PubMed.
- R. Levy, Z. Okun, M. Davidovich-Pinhas and A. Shpigelman, Utilization of high-pressure homogenization of potato protein isolate for the production of dairy-free yogurt-like fermented product, Food Hydrocolloids, 2021, 113, 106442 CrossRef CAS.
- F. T. Saricaoglu, Application of high-pressure homogenization (HPH) to modify functional, structural and rheological properties of lentil (Lens culinaris) proteins, Int. J. Biol. Macromol., 2020, 144, 760–769 CrossRef CAS PubMed.
- J. Yang, G. Liu, H. Zeng and L. Chen, Effects of high pressure homogenization on faba bean protein aggregation in relation
to solubility and interfacial properties, Food Hydrocolloids, 2018, 83, 275–286 CrossRef CAS.
- F. Wu, X. Shi, H. Zou, T. Zhang, X. Dong, R. Zhu and C. Yu, Effects of high-pressure homogenization on physicochemical, rheological and emulsifying properties of myofibrillar protein, J. Food Eng., 2019, 263, 272–279 CrossRef CAS.
- G. Fayaz, S. Plazzotta, S. Calligaris, L. Manzocco and M. C. Nicoli, Impact of high pressure homogenization on physical properties, extraction yield and biopolymer structure of soybean okara, LWT, 2019, 113, 108324 CrossRef CAS.
- S. Plazzotta, M. Moretton, S. Calligaris and L. Manzocco, Physical, chemical, and techno-functional properties of soy okara powders obtained by high pressure homogenization and alkaline-acid recovery, Food Bioprod. Process., 2021, 128, 95–101 CrossRef CAS.
- S. Melchior, M. Moretton, S. Calligaris, L. Manzocco and M. C. Nicoli, High pressure homogenization shapes the techno-functionalities and digestibility of pea proteins, Food Bioprod. Process., 2021, 131, 77–85 CrossRef.
- S. G. Giteru, I. Oey and M. A. Ali, Feasibility of using pulsed electric fields to modify biomacromolecules: A review, Trends Food Sci. Technol., 2018, 72, 91–113 CrossRef CAS.
- S. Melchior, S. Calligaris, G. Bisson and L. Manzocco, Understanding the impact of moderate-intensity pulsed electric fields (MIPEF) on structural and functional characteristics of pea, rice and gluten concentrates, Food Bioprocess Technol., 2020, 13, 2145–2155 CrossRef CAS.
- M. G. Ferruzzi, The influence of beverage composition on delivery of phenolic compounds from coffee and tea, Physiol. Behav., 2010, 100, 33–41 CrossRef CAS PubMed.
- W. Stahl, H. Van Den Berg, J. Arthur, A. Bast, J. Dainty, R. M. Faulks, C. Gärtner, G. Haenen, P. Hollman, B. Holst, F. J. Kelly, M. C. Polidori, C. Rice-Evans, S. Southon, T. Van Vliet, J. Viña-Ribes, G. Williamson and S. B. Astley, Bioavailability and metabolism, Mol. Aspects Med., 2002, 23, 39–100 CrossRef CAS PubMed.
- F. J. Barba, L. R. B. Mariutti, N. Bragagnolo, A. Z. Mercadante, G. V. Barbosa-Cánovas and V. Orlien, Bioaccessibility of bioactive compounds from fruits and vegetables after thermal and nonthermal processing, Trends Food Sci. Technol., 2017, 67, 195–206 CrossRef CAS.
- M. Alongi, G. Verardo, A. Gorassini, M. A. Lemos, G. Hungerford, G. Cortella and M. Anese, Phenolic content and potential bioactivity of apple juice as affected by thermal and ultrasound pasteurization, Food Funct., 2019, 10, 7366–7377 RSC.
- M. Buniowska, J. M. Carbonell-Capella, A. Frigola and M. J. Esteve, Bioaccessibility of bioactive compounds after non-thermal processing of an exotic fruit juice blend sweetened with Stevia rebaudiana, Food Chem., 2017, 221, 1834–1842 CrossRef CAS PubMed.
- M. J. Rodríguez-Roque, B. De Ancos, R. Sánchez-Vega, C. Sánchez-Moreno, M. P. Cano, P. Elez-Martínez and O. Martín-Belloso, Food matrix and processing influence on carotenoid bioaccessibility and lipophilic antioxidant activity of fruit juice-based beverages, Food Funct., 2016, 7, 380–389 RSC.
- M. J. Rodríguez-Roque, B. de Ancos, C. Sánchez-Moreno, M. P. Cano, P. Elez-Martínez and O. Martín-Belloso, Impact of food matrix and processing on the in vitro bioaccessibility of vitamin C, phenolic compounds, and hydrophilic antioxidant activity from fruit juice-based beverages, J. Funct. Foods, 2015, 14, 33–43 CrossRef.
- D. J. McClements, E. A. Decker, Y. Park and J. Weiss, Structural design principles for delivery of bioactive components in nutraceuticals and functional foods, Crit. Rev. Food Sci. Nutr., 2009, 49, 577–606 CrossRef CAS PubMed.
- C. Acquah, G. Ohemeng-Boahen, K. A. Power and S. M. Tosh, The Effect of Processing on Bioactive Compounds and Nutritional Qualities of Pulses in Meeting the Sustainable Development Goal 2, Front. Sustainable Food Syst., 2021, 5, 681662 CrossRef.
- D. J. McClements, Recent developments in encapsulation and release of functional food ingredients: delivery by design, Curr. Opin. Food Sci., 2018, 23, 80–84 CrossRef.
- D. J. McClements and S. F. Peng, Current status in our understanding of physicochemical basis of bioaccessibility, Curr. Opin. Food Sci., 2020, 31, 57–62 CrossRef.
- R. F. S. Gonçalves, J. T. Martins, C. M. M. Duarte, A. A. Vicente and A. C. Pinheiro, Advances in nutraceutical delivery systems: From formulation design for bioavailability enhancement to efficacy and safety evaluation, Trends Food Sci. Technol., 2018, 78, 270–291 CrossRef.
- S. Boostani and S. M. Jafari, A comprehensive review on the controlled release of encapsulated food ingredients; fundamental concepts to design and applications, Trends Food Sci. Technol., 2021, 109, 303–321 CrossRef CAS.
- V. S. Jayamanne and M. R. Adams, Determination of survival, identity and stress resistance of probiotic bifidobacteria in bio-yoghurts, Lett. Appl. Microbiol., 2006, 42, 189–194 CrossRef CAS PubMed.
-
M. Marino, N. Innocente, S. Melchior, S. Calligaris and M. Maifreni, Main Technological Challenges Associated With the Incorporation of Probiotic Cultures into Foods in Advances in Probiotics Microorganisms in Food and Health, ed. D. Dhanasekaran and A. Sankaranarayanan, Academic Press, 2021, pp. 479–495 Search PubMed.
- C. S. Ranadheera, S. K. Baines and M. C. Adams, Importance of food in probiotic efficacy, Food Res. Int., 2010, 43, 1–7 CrossRef.
- I. Sumeri, L. Arike, J. Stekolštšikova, R. Uusna, S. Adamberg, K. Adamberg and T. Paalme, Effect of stress pretreatment on survival of probiotic bacteria in gastrointestinal tract simulator, Appl. Microbiol. Biotechnol., 2010, 86, 1925–1931 CrossRef CAS PubMed.
- A. Terpou, A. Papadaki, I. K. Lappa, V. Kachrimanidou, L. A. Bosnea and N. Kopsahelis, Probiotics in food systems: significance and emerging strategies towards improved viability and delivery of enhanced beneficial value, Nutrients, 2019, 11, 1591 CrossRef CAS PubMed.
- J. Burgain, C. Gaiani, M. Linder and J. Scher, Encapsulation of probiotic living cells: From laboratory scale to industrial applications, J. Food Eng., 2011, 104, 467–483 CrossRef CAS.
- L. K. Sarao and M. Arora, Probiotics, prebiotics, and microencapsulation: A review, Crit. Rev. Food Sci. Nutr., 2017, 57, 344–371 CrossRef CAS PubMed.
- S. Gouin, Microencapsulation: Industrial appraisal of existing technologies and trends, Trends Food Sci. Technol., 2004, 15, 330–347 CrossRef CAS.
- L. F. Calinoiu, B. E. Ştefanescu, I. D. Pop, L. Muntean and D. C. Vodnar, Chitosan coating applications in probiotic microencapsulation, Coatings, 2019, 9, 1–21 CrossRef.
- S. B. Doherty, M. A. Auty, C. Stanton, R. P. Ross, G. Fitzgerald and A. Brodkorb, Application of whey protein micro-bead coatings for enhanced strength and probiotic protection during fruit juice storage and gastric incubation, J. Microencapsulation, 2012, 29, 713–728 CrossRef CAS PubMed.
- L. G. Gómez-Mascaraque, R. C. Morfin, R. Pérez-Masiá, G. Sanchez and A. Lopez-Rubio, Optimization of electrospraying conditions for the microencapsulation of probiotics and evaluation of their resistance during storage and in vitro digestion, LWT – Food Sci. Technol., 2016, 69, 438–446 CrossRef.
- M. Marino, N. Innocente, S. Calligaris, M. Maifreni, A. Marangone and M. C. Nicoli, Viability of probiotic Lactobacillus rhamnosus in structured emulsions containing saturated monoglycerides, J. Funct. Foods, 2017, 35, 51–59 CrossRef CAS.
- S. Melchior, M. Marino, F. D′este, N. Innocente, M. C. Nicoli and S. Calligaris, Effect of the formulation and structure of monoglyceride-based gels on the viability of probiotic Lactobacillus rhamnosus upon in vitro digestion, Food Funct., 2021, 12, 351–361 RSC.
- X. Zhuang, S. Clark and N. Acevedo, Bigels—oleocolloid matrices—as probiotic protective systems in yogurt, J. Food Sci., 2021, 86, 4892–4900 CrossRef CAS PubMed.
- S. Melchior, S. Calligaris, M. Marino, F. D'Este, G. Honsell, M. C. Nicoli and N. Innocente, Digestive protection of probiotic Lacticaseibacillus rhamnosus in Ricotta cheese by monoglyceride structured emulsions, Int. J. Food Sci. Technol., 2022, 57, 3106–3115 CrossRef CAS.
- A. Brodkorb, L. Egger, M. Alminger, P. Alvito, R. Assunção, S. Ballance, T. Bohn, C. Bourlieu-Lacanal, R. Boutrou, F. Carrière, A. Clemente, M. Corredig, D. Dupont, C. Dufour, C. Edwards, M. Golding, S. Karakaya, B. Kirkhus, S. Le Feunteun, U. Lesmes, A. Macierzanka, A. R. Mackie, C. Martins, S. Marze, D. J. McClements, O. Ménard, M. Minekus, R. Portmann, C. N. Santos, I. Souchon, R. P. Singh, G. E. Vegarud, M. S. J. Wickham, W. Weitschies and I. Recio, INFOGEST static in vitro simulation of gastrointestinal food digestion, Nat. Protoc., 2019, 14, 991–1014 CrossRef CAS PubMed.
- C. S. Levi and U. Lesmes, Bi-compartmental elderly or adult dynamic digestion models applied to interrogate protein digestibility, Food Funct., 2014, 5, 2402–2409 RSC.
- K. Aalaei, B. Khakimov, C. De Gobba and L. Ahrné, Digestion patterns of proteins in pasteurized and ultra-high temperature milk using in vitro gastric models of adult and elderly, J. Food Eng., 2021, 292, 110305 CrossRef CAS.
- E. Hernández-Olivas, S. Muñoz-Pina, J. Sánchez-García, A. Andrés and A. Heredia, Understanding the role of food matrix on the digestibility of dairy products under elderly gastrointestinal conditions, Food Res. Int., 2020, 137, 109454 CrossRef PubMed.
- E. Hernández-Olivas, S. Muñoz-Pina, A. Andrés and A. Heredia, Impact of elderly gastrointestinal alterations on in vitro digestion of salmon, sardine, sea bass and hake: Proteolysis, lipolysisand bioaccesibilityt of calcium and vitamins, Food Chem., 2020, 326, 127024 CrossRef PubMed.
|
This journal is © The Royal Society of Chemistry 2022 |
Click here to see how this site uses Cookies. View our privacy policy here.