Measurement of 87Sr/86Sr in limestones after acid leaching and direct injection in a liquid chromatograph coupled to a multicollector ICP-MS
Received
5th October 2021
, Accepted 7th December 2021
First published on 7th December 2021
Abstract
A procedure for the measurement of 87Sr/86Sr in carbonates without off-line strontium separation was developed, validated and applied to Normandy chalkstones. The method is based on the injection of the dissolved/leached sample into a high-performance liquid chromatography (HPLC) system directly coupled to a multicollector inductively coupled plasma mass spectrometer (MC-ICP-MS). Matrix and spectral (Ca, Rb) interferences were removed by an on-line separation procedure based on cation exchange chromatography using 1 M nitric acid as an eluent in the presence of 3.8 mM 18-crown-6. The main polyatomic interferent was identified as NO2Ca+, affecting mass 86 of strontium. Isotope ratios were calculated by multiple linear regression from the measured chromatographic peaks. The method was evaluated using a NIST 987 Sr isotope reference material and subsequently validated employing a JCp-1 coral reference material in comparison with the traditional off-line separation procedure. Finally, the method was applied to ten Normandy limestones (chalkstones) with results in agreement with the expected values based on geological, geochemical, and stratigraphic studies.
1. Introduction
The use of the 87Sr/86Sr isotope ratio as a tracer in marine sediments is widespread due to its correlation with the seawater 87Sr/86Sr ratio where the carbonate sediments precipitated millions of years ago.1–3 This fact enables the dating of carbonate sediments and allows its application in archaeological studies where the 87Sr/86Sr ratio in an archaeological sample (e.g. chalkstone used in a building) can be correlated with the quarry of origin of that chalkstone.
For accurate Sr isotope ratio determination in limestones, it is mandatory to isolate Sr from Rb and Ca due to isobaric and matrix interferences. The well-known isobaric interference between 87Rb and 87Sr needs to be eliminated by a chemical separation. In the same way, Ca must be separated from Sr as it may produce polyatomic interference at masses 86 and 88 due to the formation of Ca dimers (40Ca46Ca+, 42Ca44Ca+, 43Ca2+, 40Ca48Ca+, 42Ca46Ca+, and 44Ca2+) and argon–Ca molecular ions (38Ar48Ca+, 40Ar46Ca+, and 40Ar48Ca+).4
The traditional methodology for the isolation of target Sr is based on solid phase extraction by cation exchange or chelating resins. The commercially available DOWEX 50W-X8 and AG50W-X8 resins, based on sulfonic acid functional groups, recover Sr using HCl5,6 but a complete separation of Rb and Sr is difficult to achieve at high Rb concentrations.7 A chelating resin for specific separation of Sr is formed by 4,4′(5′)-di-t-butylcyclohexane 18-crown-6 (a crown ether) diluted in 1-octanol on an inert polymeric support and is commercially available as Eichrom's Sr Resin.8 This last resin is generally used in geochemistry studies due to the successful capability to selectively isolate Sr from many concomitant elements using HNO3 as an eluent.9 Once Sr is isolated, the 87Sr/86Sr ratio of the sample solution can be measured by Thermal Ionization Mass Spectrometry (TIMS) or Multicollector Inductively Coupled Plasma Mass Spectrometry (MC-ICP-MS) providing the precision and accuracy necessary to resolve the slight differences in Sr isotopic composition.
Although the main procedure to determine 87Sr/86Sr by MC-ICP-MS is solid phase extraction and continuous sample introduction,9 other alternative methods have been developed in order to reduce sample preparation time or to study heterogeneities in solid samples. Laser ablation MC-ICP-MS has been widely applied to carbonate materials allowing the determination of 87Sr/86Sr without prior chemical treatment.10–12 Unfortunately, this is not a very precise technique for Sr isotope ratio determination in samples with Sr concentrations under 30 μg g−1 (ref. 13) due to the fact that far fewer ions are measured due to a combination of low transmission and tiny sample size. Additionally, the process of mass dependent fractionation of Sr occurring during analysis14 and the polyatomic interference arising from the calcium matrix15 have hindered the application of laser ablation for the measurement of 87Sr/86Sr.
An alternative method for Sr isotope ratio measurement by MC-ICP-MS is the on-line separation of Sr from other elements by liquid chromatography. The separation of Rb and Sr by this technique was described previously7 but without an appropriate separation of Sr from Ca. García-Ruiz et al.16 described a successful separation of Sr from the interference in different sample matrixes with high precision Sr isotope ratio measurements and Rodriguez-Castrillón et al.17 applied this methodology in combination with multiple linear regression for isotope ratio measurement. However, no validation of the methodology or application to real samples was performed.16,17 This mathematical procedure allows the automatic correction of the baseline (e.g. krypton correction at masses 84 and 86 is not necessary) and does not require the integration of the chromatographic peaks obtaining isotope ratio precisions only a factor of two worse than those obtained by off-line isotope ratio measurement with continuous sample introduction.
The objective of this study was to develop and validate a fast methodology for 87Sr/86Sr determination in limestones based on the on-line chromatographic separation of Ca, Sr and Rb16 coupled with an MC-ICP-MS as a detector and final data treatment by multiple linear regression.17 The on-line chromatographic separation is proposed as an alternative to solid phase extraction, a time-consuming process that can introduce contamination during sample handling. To validate our methodology, two certified reference materials were used, NIST SRM 987, a strontium isotopic reference material and JCp-1, a calcium carbonate Porites sp. coral.18 Finally, the method was applied to ten Normandy limestones (chalkstones) and the results were compared with the expected values based on previous geological, geochemical, and stratigraphic studies.19
2. Experimental
2.1. Instrumentation
The chromatographic separation of Ca, Sr and Rb was performed using a Thermo Finnigan Surveyor HPLC system (Thermo Scientific, Bremen, Germany). The optimization of the chromatographic conditions was carried out in a quadrupole ICP-MS (HP7500ce, Agilent Technologies, Manchester, UK). The column from the chromatographic system was connected through polyetheretherketone (PEEK) tubing (Supelco, Pennsylvania, USA) to the sample introduction system consisting of a Conikal nebulizer (1 ml min−1 flow) and a quartz Scott Spray Chamber (Glass expansion, Melbourne, Australia). Data acquisition was carried out in time resolved analysis mode with 0.1 s acquisition time per isotope. The monitored isotopes were 43Ca, 85Rb, 86Sr, 87Rb–87Sr and 88Sr.
Method evaluation and validation was performed in a multicollector double focusing magnetic sector field MC-ICP-MS (Neptune Plus, Thermo Scientific, Bremen, Germany) with a forward Nier–Johnson geometry and provided with nine collectors: eight mobile and a fixed central Faraday cup. The chromatographic system was coupled with PEEK tubing to the sample introduction system consisting of a Conikal nebulizer (1 ml min−1 flow) (Glass Expansion, Melbourne, Australia) and a cyclonic spray chamber (Thermo Scientific, Bremen, Germany). The Faraday cup configuration was as follows: 82(L4), 83(L3), 84(L2), 85(L1), 86(C), 87(H1), 88(H2) and 90(H3). Data acquisition was performed over 1200 cycles with 1.049 s integration time.
2.2. Reagents and materials
The chromatographic mobile phase was prepared with ultra-pure water obtained from a Purelab flex water purification system (Elga Labwater, Paris, France), nitric acid from Merck (Darmstadt, Germany), analytical grade, purified by sub-boiling distillation and ≥99.0% purity 18-crown-6 ether from Sigma Aldrich (Missouri, USA). Glacial acetic acid, used for sample leaching, was from VWR Chemicals (Pennsylvania, USA). Rb standard from High-Purity Standards (North Charleston, USA) was added to the calcite sample during the optimization procedure. Validation of the procedure was carried out by analysis of certified reference materials NIST SRM 987 strontium carbonate (NIST, Gaithersburg, MD, USA) and JCp-1 calcium carbonate, a Porites sp. coral (GSJ, Tsukuba, Japan).
2.3. Sample preparation procedure
For chromatographic optimization, 0.1 g of ground crystalline calcite (Las Caldas, Oviedo, Spain) was dissolved in HNO3 and 500 μg l−1 of Rb standard was added to the calcite solution to obtain a relatively large Rb signal after the chromatography separation. The same sample preparation procedure was initially applied to Normandy limestones to check the chromatographic separation procedure and optimization of the measurement conditions. For method validation, a strontium carbonate isotopic standard, NIST SRM 987, and a geochemical reference material, Porites sp. coral JCp-1, were measured. NIST SRM 987 was diluted in HNO3 to a final concentration of 250 μg l−1.
Limestone sample preparation was based on Bailey et al.20 applying a sequential leaching procedure. In brief, ca. 0.1 g of sample was wetted with two ml of ultrapure water, and 300 μL of 20% w/w acetic acid was added. To avoid contamination, limestone sample preparation was carried out in a laminar flow cabinet (MSC Advantage, Thermo Scientific, Bremen, Germany). After reaction the solid was separated by centrifugation and a second addition of water and 20% w/w acetic acid performed. The supernatant of the second addition was isolated by centrifugation and was filtered through a 0.45 μm filter prior to its introduction into the chromatography system. For JCp-1 the same dissolution procedure was applied; however, due to its purity, a sequential leaching was not necessary and the first extracted solution was injected into the chromatographic system.
2.4. Off-line sample preparation procedure and measurement conditions for JCp-1
This new methodology has been compared with the results obtained for the reference material JCp-1 in the Geochronology and Isotopic Geochemistry Unit – SGIker of the University of the Basque Country. Sample preparation at this facility was done in polypropylene Class-A (ISO-5) laminar-flow benches within an ISO-7 clean lab, employing the same materials and reagents of similar quality as those described above. About 0.05 g of the JCp-1 reference material was dissolved in 1.5 ml of 7 M HNO3, and Sr purification from this solution was performed through a solid extraction phase with commercial Sr-resin (TrisKem International, Bruz, France). The purification process is based on Pin et al.,21 but employing 7 M HNO3 instead of 2 M HNO3 as the initial sample loading solution to avoid Rb extraction by the resin. After Sr back-extraction from the resin in 0.05 M HNO3, each sample solution was conveniently diluted in 3% HNO3 to obtain a final concentration of about 200 ng Sr per g solution. The samples were introduced as wet aerosols into a Thermo Scientific Neptune MC-ICP-MS using an ESI 100 μl min−1 PFA nebulizer and a dual cyclonic-Scott double pass spray chamber. The spectrometric method comprised a static multi-collection routine of 3 blocks of 25 cycles with an integration time of 8 s per cycle. Baselines of the amplifiers were reset at the beginning of each block. Instrumental mass bias and Kr interference were corrected following Balcaen et al.22
2.5. Liquid chromatographic separation
The separation of Ca, Sr and Rb was achieved working in isocratic mode, using 1 M HNO3 and 3.8 mM 18-crown-6 ether combined with a Dionex cation exchange IonPac CS12A analytical column (4 mm × 250 mm) with carboxylic acid functional groups. Two different perfluoroalkoxy (PFA) sample loops (Omnifit, Cambridge, England) were used, 100 μl and 50 μl, depending on Sr concentration in the samples.
2.6. Calculation procedure: multiple linear regression
The 87Sr/86Sr determination was carried out by multiple linear regression after measuring all Sr and Rb isotopes simultaneously by MC-ICP-MS. Linear regression applied to transient signals and afterwards to chromatographic data was described previously.23,24 Recently, multiple linear regression was applied to the chromatographic separation of Rb–Sr, as described by Rodriguez-Castrillón et al.17 In brief, in a Sr solution where Rb is not present, the plot of the signals obtained by time resolved analysis of two isotopes of Sr (e.g.87Sr and 86Sr) should provide a straight line, indicating the simple linear regression between both isotopes, following eqn (1):where S87 and S86 are the signals obtained by MC-ICP-MS measurement after chromatographic separation, a is the intercept and b is the slope of the straight line that corresponds to the 87Sr/86Sr signal ratio that, after mass bias correction, represents the 87Sr/86Sr isotope ratio. In the same way, in a Rb solution where Sr is not present, 87Rb and 85Rb can be correlated by simple linear regression.
In a solution where both Sr and Rb are present, the correlation between mass 87 from Sr and Rb and masses 86Sr and 85Rb follows eqn (2):
which is the equation of a plane in the 85–86–87 (
x–
y–
z) space. In this situation,
a is the intercept,
b the
87Rb/
85Rb signal ratio and
c the
87Sr/
86Sr signal ratio. In the case that Rb and Sr were not separated,
eqn (2) has infinite solutions and the
87Sr/
86Sr ratio cannot be determined by this method. However, when Rb and Sr are separated, even partially,
25eqn (2) has one solution that can be obtained by multiple linear regression allowing the determination of the
87Sr/
86Sr isotope ratio after internal mass bias correction.
Fig. 1 shows the 3D-plot of the signal at mass 87
vs. the signals at masses 85 and 86 for the chromatographic separation of Sr NIST 987 doped with a natural abundance Rb standard. As can be observed, the plot of the signals for the whole duration of the chromatogram (1000 data points) fits a plane whose slopes correspond to the
87Rb/
85Rb and
87Sr/
86Sr signal ratios. The equation of the plane (
eqn (2)) can be easily obtained with the LINEST function in Microsoft Excel.
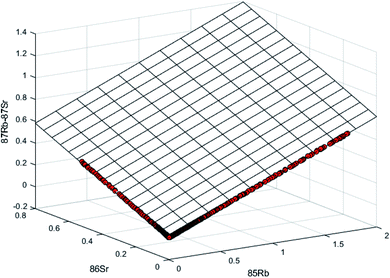 |
| Fig. 1 3D plot of signals (V) for 85Rb (x), 86Sr (y) and 87Rb–87Sr (z) for the separation of Sr NIST 987 and a natural abundance Rb standard (1000 data points). The plane follows the least squares solution: z = −0.000047 + 0.404699x + 0.727734y. | |
The ratio 88Sr/86Sr, used for internal mass bias correction, is obtained using the analogue of eqn (1) for the signals corresponding to 88Sr and 86Sr as there is no isobaric overlap from rubidium. Please note that the isobaric interference from 84Kr and 86Kr, corrected normally by measuring 83Kr and subtracting the contribution at masses 84 and 86 by an iterative procedure,22 does not need to be corrected here as the contribution appears at the intercept of the linear regression. The Russell equation was employed for internal mass bias correction.
3. Results and discussion
3.1. Optimization of the liquid chromatographic separation
Due to the high concentration of CaCO3 in limestones samples, dimers of Ca and ArCa molecular ions can be formed during the chemical and physical processes occurring in the ICP resulting in polyatomic interference4 mainly at masses 86 and 88. In order to avoid this interference, as well as the isobaric interference of 87Rb on 87Sr, the chromatographic separation of Ca, Sr and Rb is required. For the optimisation of the separation conditions, a pure crystallized calcite sample was dissolved in nitric acid and the solution, containing ca. 1000 μg ml−1 of Ca and ca. 1 μg ml−1 of Sr, was doped with 0.5 μg ml−1 of a Rb standard and injected into the chromatographic system. In the initial studies a quadrupole ICP-MS model Agilent 7500ce was employed using isotopes 43 (Ca), 85 and 87 (Rb) and 86, 87 and 88 (Sr) for detection as indicated in the Experimental section.
Based on previous studies,7,16 an Ion Pac CS12A column (Dionex) was used with HNO3 and 18-crown-6 ether as the mobile phase. It was indicated that the addition of 18-crown-6 ether to the mobile phase increases the retention times for alkali and alkaline earth ions26,27 improving the separation efficiency. After the evaluation of several mobile phases, 3.8 mM 18-crown-6 ether and 1 M HNO3 was selected as the eluent. Under these conditions, Ca elutes at ca. 100 s, Sr at ca. 200 s and Rb at ca. 700 s, respectively, as shown in Fig. 2 for masses 43, 85, 87 and 88, respectively.
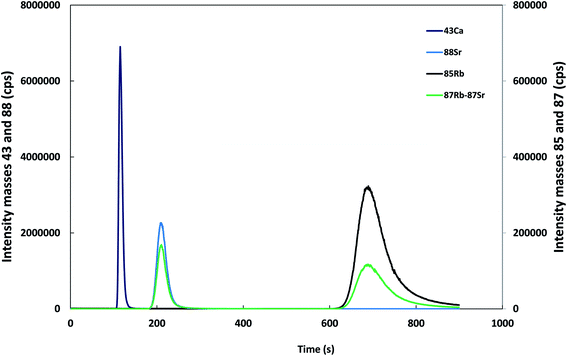 |
| Fig. 2 Separation of calcium, strontium and rubidium under the optimized chromatographic conditions. Masses 43 and 88, left axis. Masses 85 and 87, right axis (10-fold enlargement for clarity). | |
3.2. Coupling of the liquid chromatograph to the multicollector ICP-MS
Once the separation conditions were selected, the liquid chromatograph was coupled to the multicollector ICP-MS and a blank and a real sample of Normandy limestone, digested in acetic acid and diluted to ca. 1000 μg g−1 Ca, were injected. No strontium peak was detected in the injected blank so blank correction was not necessary. Fig. 3A shows the chromatogram obtained for the real sample focusing on the signal of Sr while in Fig. 3B the scale was enlarged to observe the spectral interference which appears at the calcium retention time and the small peak of rubidium obtained. Based on the isotopic profile of the calcium interference peak which appears in Fig. 3B the spectral interference was tentatively assigned to the polyatomic ion NO2Ca+ (main interference at mass 86) and not to Ca–Ca dimers or Ar–Ca polyatomic ions which would have a completely different isotopic profile with very low contribution at mass 86. The fact that we are using a mobile phase containing 1 M nitric acid could explain the spectral interference profile observed. It is clear that, for the multiple linear regression calculations, the first data points where the calcium peaks appear (ca. the first 150 seconds) need to be removed from the calculations but the rest of the chromatogram is included.
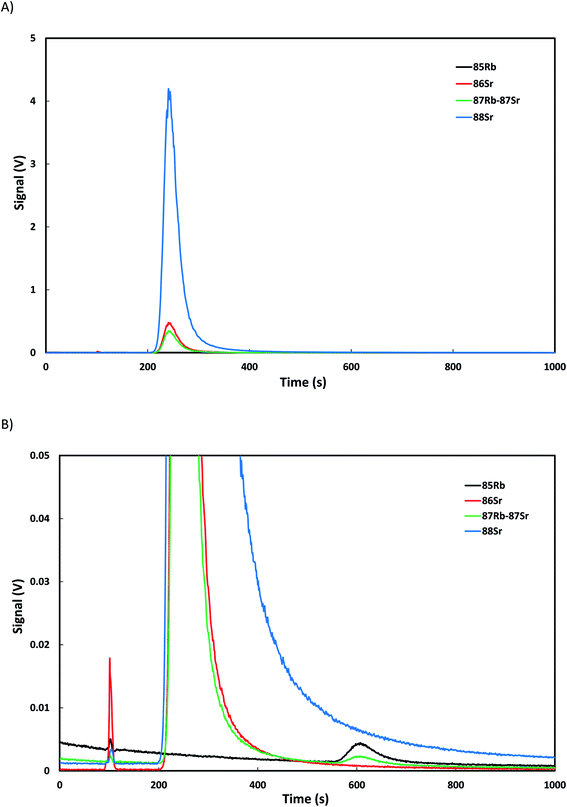 |
| Fig. 3 Separation of Ca, Sr and Rb and detection by multicollector ICP-MS in a real chalkstone sample. (A) Signals obtained in the full scale showing the Sr peak. (B) Enlargement of the chromatogram to show the Ca spectral interference and the small Rb peak present in the limestone. | |
3.3. Validation of the methodology: NIST 987 and JCp-1 coral reference materials
Method validation was carried out by measuring two certified reference materials, SRM NIST 987 and JCp-1, a Porites sp. coral. A solution of 5 mg l−1 NIST 987 was mixed with a 5 mg l−1 Rb standard and injected into the chromatographic system. The obtained chromatograms were treated by multiple linear regression as shown in Fig. 1 and mass bias was corrected using 8.37521 as a reference value for the 88Sr/86Sr ratio.28–32 Uncertainty propagation was carried out using the Kragten procedure.33Table 1 shows the results for 87Sr/86Sr for 25 sequential analyses by the proposed methodology obtained during 9 measuring sessions on different days. As can be observed, the average 87Sr/86Sr (n = 25) of 0.71026 ± 0.00007 is in very good agreement with the certified value of 87Sr/86Sr = 0.71034 ± 0.00026 and with the accepted value by the geochemical community.28–32
Table 1
87Sr/86Sr values for 25 measurements of NIST SRM 987 for the on-line procedure in the course of nine days of analysis (n = number of replicates per day). Uncertainty expressed as 2 times the standard deviation (2SD, n = 25)
Day |
n
|
87Sr/86Sr |
Uncertainty |
1 |
4 |
0.71032 |
|
2 |
3 |
0.71022 |
|
3 |
2 |
0.71022 |
|
4 |
3 |
0.71027 |
|
5 |
3 |
0.71027 |
|
6 |
3 |
0.71027 |
|
7 |
3 |
0.71029 |
|
8 |
2 |
0.71020 |
|
9 |
2 |
0.71025 |
|
Average |
25 |
0.71026 |
±0.00007 |
Certified |
— |
0.71034 |
±0.00026 |
Subsequently the certified reference material, JCp-1, was measured to validate the methodology in real samples and to study the performance of the chromatographic system in a calcium carbonate matrix. In contrast to NIST 987, JCp-1 is not a reference material for 87Sr/86Sr determination, but this material was studied previously for this purpose by 15 laboratories12,34–42 and the Sr isotope ratio results have been accepted and published in the GeoReM database.43 Sample preparation was carried out in two ways, as indicated in the procedures. The off-line sample preparation procedure was performed at the University of the Basque Country while the on-line procedure was carried out at the University of Oviedo by extraction with acetic acid and direct injection in the chromatographic system after adequate dilution. Table 2 shows the values obtained for 87Sr/86Sr from 25 replicates measured during five different sessions in the on-line procedure and for 4 replicates measured during four measurement sessions in the off-line procedure. The 87Sr/86Sr ratios were normalized to the 88Sr/86Sr ratio of 8.37521 as is customary in the geochemical literature.28–32,44 A mean value of 0.709157 ± 0.000034 (2SD) was obtained by the on-line procedure while a mean value of 0.709166 ± 0.000016 (2SD) was obtained by the off-line procedure. As can be observed, the agreement between the two procedures can be considered satisfactory. Also, these data are in agreement with the literature values ranging from 0.70913 ± 0.00008 to 0.709182 ± 0.000064.43
Table 2
87Sr/86Sr values for 25 measurements of the JCp-1 coral, in the course of 5 days of analysis (n: number of replicates per day) for the on-line procedure and values for 4 measurements over 4 days for the off-line procedure. Uncertainty of each measurement is expressed as 2 times the standard error (2SE) for the off-line procedure (n = 1) and as 2 times the standard deviation for the on-line procedure (n = 5). Uncertainty of the mean of all measurements is given as 2 times the standard deviation (2SD) in both cases
Day |
On-line procedure |
Off-line procedure |
n
|
87Sr/86Sr |
Uncertainty |
n
|
87Sr/86Sr |
Uncertainty |
1 |
5 |
0.709154 |
±0.000028 |
1 |
0.709157 |
±0.000011 |
2 |
5 |
0.709152 |
±0.000031 |
1 |
0.709167 |
±0.000011 |
3 |
5 |
0.709151 |
±0.000032 |
1 |
0.709176 |
±0.000011 |
4 |
5 |
0.709164 |
±0.000034 |
1 |
0.709165 |
±0.000012 |
5 |
5 |
0.709164 |
±0.000042 |
— |
— |
— |
Average |
25
|
0.709157
|
±0.000034
|
4
|
0.709166
|
±0.000016
|
A brief discussion of the advantages and disadvantages of the on-line procedure in comparison with the off-line procedure is worth including here. It is clear that sample preparation is simpler in the on-line procedure as, after digestion, the sample is injected directly into the LC system without any further treatment. For limestone samples this is possible thanks to the elevated concentration of strontium which makes preconcentration, for MC-ICP-MS measurement, not necessary. For other lower concentration samples, it may be necessary to apply the off-line strontium isolation procedure. One disadvantage of the on-line procedure is that the final uncertainty is ca. two-fold in comparison with that using off-line sample preparation and continuous sample introduction (see Table 2) due to the transient nature of the signals. On the other hand, data treatment is simpler in the on-line procedure as neither krypton correction, at masses 84 and 86, nor rubidium correction, at mass 87, is necessary. The rubidium correction used in the off-line procedure assumes that the fractionation factor calculated for 88Sr/86Sr can also be applied to 87Rb/85Rb which is not the case, and this correction works only for very low 85Rb signals. In the on-line procedure the magnitude of the 85Rb signal is of no concern. Finally, the fact that the 87Rb/85Rb ratio is measured along with the 87Sr/86Sr ratio (Fig. 1) opens the way for alternative mass bias correction procedures using 87Rb/85Rb as a reference instead of 88Sr/86Sr. This could be of interest when the 88Sr/86Sr ratio needs to be measured as well.
3.4. Application in the determination of Sr isotope ratios in Normandy chalkstones
Once the methodology was validated, its robustness was evaluated by measuring the Sr isotope ratios in ten limestone samples (chalkstones) as part of the project ATP (Archéometériaux: Territoire, Patrimonie) that studies the spatial distribution of Normandy chalkstone in Eastern Normandy, nominated as a Global Heritage Stone Resource due to the role it has played throughout history.45,46 Chalkstone, a special limestone considered as a “soft” rock due to its low cohesiveness and durability caused by its 20–40% porosity,47,48 dominated the construction of churches, castles, abbeys, and cathedral buildings in Eastern Normandy during medieval ages. The study of Sr isotope ratios is a part of a global study of this rock that implies archaeological criteria and geological techniques with the final objective of identifying and characterizing the medieval quarrying places of Normandy chalkstone and their diffusion areas between the 10th and 14th centuries.45,46
Sequential leaching procedures with acetic acid were performed and all extracts injected into the chromatographic system. No significant differences between the first and second extracts were found ruling out the existence of diagenetic contamination in the samples. Anyway, the procedure described by Bailey et al.20 was followed and the final results were based on the data collected from the second acetic acid extract. Table 3 shows the results of 87Sr/86Sr for chalkstones collected in three medieval quarries (Caumont, Vernon and Fécamp) and their comparison with the results of the analysis of four buildings (the castle remains at Lyons-la-Forêt, Maulèvrier-Sainte-Gertrude and Hautot-sur-Mer and the church at La Trinité-de-Thouberville). The observed isotope ratios are in agreement with the expected values based on strontium isotope stratigraphy according to McArthur et al.49 which defined the Upper Cretaceous 87Sr/86Sr isotope curve in the London–Paris basin. Unfortunately, the 87Sr/86Sr ratios alone do not offer a clear correlation between the chalkstone from quarries and buildings due to their small differences and the variability within the quarries themselves (as in the case of the Caumont quarry where three samples were measured). Only the sample at the Fécamp quarry is clearly different from the rest and it would help to discard this quarry as the origin of the chalkstone employed for the buildings. Obviously, the measurement of strontium isotope ratios is only one parameter in the geological and geochemical study of these materials where additional optical microscopy and whole rock geochemistry provided additional data interpretation capabilities.45
Table 3 Measured 87Sr/86Sr in chalkstones from different medieval quarries and buildings in Normandy (France)
|
Sample |
87Sr/86Sr |
2SD |
Quarries
|
Caumont |
C-CAU-04 |
0.707358 |
0.000022 |
Caumont |
C-CAU-06 |
0.707351 |
0.000021 |
Caumont |
C-CAU-10 |
0.707349 |
0.000018 |
Vernon |
C-NVD-02 |
0.707376 |
0.000030 |
Fécamp |
C-FEC-01 |
0.707548 |
0.000026 |
![[thin space (1/6-em)]](https://www.rsc.org/images/entities/char_2009.gif) |
Buildings
|
Lyons-la-Forêt (castle) |
LYO-27-1 |
0.707361 |
0.000037 |
Maulèvrier-Sainte-Gertrude (castle) |
MAU-101-1 |
0.707340 |
0.000017 |
Maulèvrier-Sainte-Gertrude (castle) |
MAU-127-5 |
0.707337 |
0.000032 |
Hautot-sur-Mer (castle) |
HAU-36-761 |
0.707320 |
0.000031 |
La Trinité-de-Thouberville (church) |
TRI-1-27-2 |
0.707406 |
0.000014 |
4. Conclusions
An on-line methodology for the determination of 87Sr/86Sr in limestones was developed based on weak acid leaching and the direct injection of the extracted samples into a HPLC system coupled to a MC-ICP-MS. In this way we can integrate the separation of spectral interference from Ca and Rb and the determination of the Sr isotope ratio in a unique process, which simplifies sample handling, avoiding time consuming off-line sample separation procedures. The method was validated with two reference materials and the results in real samples were in agreement with the expected values for Normandy chalkstones.48 The proposed technique can be applied in combination with other geological and geochemical techniques for archaeological research as is the case of the Normandy chalkstone.45
Conflicts of interest
There are no conflicts to declare.
Acknowledgements
Financial support through grant number PGC2018-097961-B-100 (Agencia Estatal de Investigación, Madrid, Spain) is gratefully acknowledged.
References
- F. E. Wickman, Isotope Ratios: A Clue to the Age of Certain Marine Sediments, J. Geol., 1948, 56, 61–66 CrossRef CAS.
- P. W. Gast, Abundance of Sr87 during geologic time, Bull. Geol. Soc. Am., 1955, 66, 1449–1454 CrossRef CAS.
- C. E. Hedge and F. G. Walthall, Radiogenic Strontium-87 as an Index of Geologic Processes, Science, 1963, 140, 1214–1217 CrossRef CAS PubMed.
- D. De Muynck, G. Huelga-Suarez, L. Van Heghe, P. Degryse and F. Vanhaecke, Systematic evaluation of a strontium-specific extraction chromatographic resin for obtaining a purified Sr fraction with quantitative recovery from complex and Ca-rich matrices, J. Anal. At. Spectrom., 2009, 24, 1498–1510 RSC.
- S. Chassery, F. E. Grousset, G. Lavaux and C. R. Quétel,
87Sr/86Sr measurements on marine sediments by inductively coupled plasma-mass spectrometry, Fresenius' J. Anal. Chem., 1998, 360, 230–234 CrossRef CAS.
- F. Vanhaecke, G. De Wannemacker, L. Moens and P. Van Den Haute, The use of sector field ICP-mass spectrometry for Rb-Sr geochronological dating, Anal. Bioanal. Chem., 2001, 371, 915–920 CAS.
- C. Latkoczy, T. Prohaska, M. Watkins, M. Teschler-Nicola and G. Stingeder, Strontium isotope ratio determination in soil and bone samples after on-line matrix separation by coupling ion chromatography (HPIC) to an inductively coupled plasma sector field mass spectrometer (ICP-SFMS), J. Anal. At. Spectrom., 2001, 16, 806–811 RSC.
- E. P. Horwitz, M. L. Dietz and R. Chiarizia, The application of novel extraction chromatographic materials to the characterization of radioactive waste solutions, J. Radioanal. Nucl. Chem., 1992, 161, 575–583 CrossRef CAS.
- A. N. Halliday, D. C. Lee, J. N. Christensen, A. J. Walder, P. A. Freedman, C. E. Jones, C. M. Hall, W. Yi and D. Teagle, Recent developments in inductively coupled plasma magnetic sector multiple collector mass spectrometry, Int. J. Mass Spectrom. Ion Processes, 1995, 146–147, 21–33 CrossRef.
- J. N. Christensen, A. N. Halliday, D. C. Lee and C. M. Hall, In situ Sr isotopic analysis by laser ablation, Earth Planet. Sci. Lett., 1995, 136, 79–85 CrossRef CAS.
- M. Bizzarro, A. Simonetti, R. K. Stevenson and S. Kurszlaukis, In situ 87Sr/86Sr investigation of igneous apatites and carbonates using laser-ablation MC-ICP-MS, Geochim. Cosmochim. Acta, 2003, 67, 289–302 CrossRef CAS.
- M. Weber, J. A. Wassenburg, K. P. Jochum, S. F. M. Breitenbach, J. Oster and D. Scholz, Sr-isotope analysis of speleothems by LA-MC-ICP-MS: high temporal resolution and fast data acquisition, Chem. Geol., 2017, 468, 63–74 CrossRef CAS.
- K. P. Jochum, B. Stoll, U. Weis, D. V. Kuzmin and A. V. Sobolev, In situ Sr isotopic analysis of low Sr silicates using LA-ICP-MS, J. Anal. At. Spectrom., 2009, 24, 1237–1243 RSC.
- Y. S. Liu, Z. C. Hu, M. Li and S. Gao, Applications of LA-ICP-MS in the elemental analyses of geological samples, Chin. Sci. Bull., 2013, 58, 3863–3878 CrossRef CAS.
- T. Waight, J. Baker and D. Peate, Sr isotope ratio measurements by double-focusing MC-ICPMS: techniques, observations, and pitfalls, Int. J. Mass Spectrom., 2002, 221, 229–244 CrossRef CAS.
- S. García-Ruiz, M. Moldovan and J. I. García Alonso, Large volume injection in ion chromatography. Separation of rubidium and strontium for on-line inductively coupled plasma mass spectrometry determination of strontium isotope ratios, J. Chromatogr., A, 2007, 1149, 274–281 CrossRef PubMed.
- J. A. Rodriguez-Castrillón, S. Garcıa-Ruiz, M. Moldovan and J. I. Garcia Alonso, Multiple linear regression and on-line ion exchange chromatography for alternative Rb–Sr and Nd–Sm MC-ICP-MS isotopic measurements, J. Anal. At. Spectrom., 2012, 27, 611–618 RSC.
- T. Okai, A. Suzuki, H. Kawahata, S. Terashima and N. Imai, Preparation of a new Geological Survey of Japan
geochemical reference material: coral JCp-1, Geostand. Newsl., 2002, 26, 95–99 CrossRef CAS.
-
J. M. McArthur, M. F. Thirlwall, A. S. Gale, W. J. Kennedy, J. A. Burnett, D. Mattey and A. R. Lord, Strontium Isotope Stratigraphy for the Late Cretaceous: A New Curve, Based on the English Chalk, Geological Society Special Publication, 1993, vol. 70, pp. 195–209 Search PubMed.
- T. R. Bailey, J. M. McArthur, H. Prince and M. F. Thirlwall, Dissolution methods for strontium isotope stratigraphy: whole rock analysis, Chem. Geol., 2000, 167, 313–319 CrossRef CAS.
- C. Pin, D. Briot, C. Bassin and F. Poitrasson, Concomitant separation of strontium and samarium-neodymium for isotopic analysis in silicate samples, based on specific extraction chromatography, Anal. Chim. Acta, 1994, 298, 209–217 CrossRef CAS.
- L. Balcaen, I. De Schrijver, L. Moens and F. Vanhaecke, Determination of the 87Sr/86Sr isotope ratio in USGS silicate reference materials by multi-collector ICP-mass spectrometry, Int. J. Mass Spectrom., 2005, 242, 251–255 CrossRef CAS.
- J. Fietzke, V. Liebetrau, D. Günther, K. Gürs, K. Hametner, K. Zumholz, T. H. Hansteen and A. Eisenhauer, An alternative data acquisition and evaluation strategy for improved isotope ratio precision using LA-MC-ICP-MS applied to stable and radiogenic strontium isotopes in carbonates, J. Anal. At. Spectrom., 2008, 23, 955–961 RSC.
- V. N. Epov, S. Berail, M. Jimenez-Moreno, V. Perrot, C. Pecheyran, D. Amouroux and O. F. X. Donard, Approach to measure isotopic ratios in species using multicollector-ICPMS coupled with chromatography, Anal. Chem., 2010, 82, 5652–5662 CrossRef CAS PubMed.
- A. Reguera-Galan, M. Moldovan and J. I. Garcia Alonso, The combined measurement of Sr-87/Sr-86 isotope ratios and Sr-88/Rb-85 elemental ratios using laser ablation MC-ICP-MS and its application for food provenance studies: the case for Asturian beans, J. Anal. At. Spectrom., 2018, 33, 867–875 RSC.
- A. Haidekker and C. G. Huber, Ion chromatography on chelating stationary phases: separation of alkali metals, J. Chromatogr., A, 2001, 921, 217–226 CrossRef CAS PubMed.
- D. A. Richens, D. Simpson, S. Peterson, A. McGinn and J. D. Lamb, Use of mobile phase 18-crown-6 to improve peak resolution between mono- and divalent metal and amine cations in ion chromatography, J. Chromatogr., A, 2003, 1016, 155–164 CrossRef CAS PubMed.
- Y. Di, E. Krestianinov, S. Zink and Y. Amelin, High-precision multidynamic Sr isotope analysis using thermal ionization mass spectrometer (TIMS) with correction of fractionation drift, Chem. Geol., 2021, 582, 120411 CrossRef CAS.
- U. Hans, T. Kleine and B. Bourdon, Rb–Sr chronology of volatile depletion in differentiated protoplanets: BABI, ADOR and ALL revisited, Earth Planet. Sci. Lett., 2013, 374, 204–214 CrossRef CAS.
- D. A. Papanastassiou and G. J. Wasserburg, Initial strontium isotopic abundances and the resolution of small time differences in the formation of planetary objects, Earth Planet. Sci. Lett., 1968, 5, 361–376 CrossRef.
- M. F. Thirlwall, Long-Term Reproducibility of Multicollector Sr and Nd Isotope Ratio Analysis, Chem. Geol., 1991, 94, 85–104 CrossRef CAS.
- D. Weis, B. Kieffer, C. Maerschalk, J. Barling, J. de Jong, G. A. Williams, D. Hanano, W. Pretorius, N. Mattielli, J. S. Scoates, A. Goolaerts, R. M. Friedman and J. B. Mahoney, High-precision isotopic characterization of USGS reference materials by TIMS and MC-ICP-MS, Geochem., Geophys., Geosyst., 2006, 7, Q08006 CrossRef.
- J. Kragten, Calculating Standard Deviations and Confidence Intervals with a Universally Applicable Spreadsheet Technique, Anal. Chim. Acta, 1994, 11, 2161–2165 Search PubMed.
- M. Weber, F. Lugli, K. P. Jochum, A. Cipriani and D. Scholz, Calcium Carbonate and Phosphate Reference Materials for Monitoring Bulk and Microanalytical Determination of Sr Isotopes, Geostand. Geoanal. Res., 2018, 42, 77–89 CrossRef CAS.
- K. P. Jochum, D. Garbe-Schönberg, M. Veter, B. Stoll, U. Weis, M. Weber, F. Lugli, A. Jentzen, R. Schiebel, J. A. Wassenburg, D. E. Jacob and G. H. Haug, Nano-Powdered Calcium Carbonate Reference Materials: Significant Progress for Microanalysis?, Geostand. Geoanal. Res., 2019, 43, 595–609 CrossRef.
- T. Ohno and T. Hirata, Simultaneous determination of mass-dependent isotopic fractionation and radiogenic isotope variation of strontium in geochemical samples by multiple collector-ICP-mass spectrometry, Anal. Sci., 2007, 23, 1275–1280 CrossRef CAS PubMed.
- Y. Sano, K. Shirai, N. Takahata, H. Amakawa and T. Otake, Ion microprobe Sr isotope analysis of carbonates with about 5 μm spatial resolution: an example from an ayu otolith, Appl. Geochem., 2008, 23, 2406–2413 CrossRef CAS.
- A. Krabbenhöft, J. Fietzke, A. Eisenhauer, V. Liebetrau, F. Böhm and H. Vollstaedt, Determination of radiogenic and stable strontium isotope ratios (87Sr/86Sr; δ88/86Sr) by thermal ionization mass spectrometry applying an 87Sr/84Sr double spike, J. Anal. At. Spectrom., 2009, 24, 1267–1271 RSC.
- M. Hori, T. Ishikawa, K. Nagaishi, K. Lin, B. S. Wang, C. F. You, C. C. Shen and A. Kano, Prior calcite precipitation and source mixing process influence Sr/Ca, Ba/Ca and 87Sr/86Sr of a stalagmite developed in southwestern Japan during 18.0-4.5 ka, Chem. Geol., 2013, 347, 190–198 CrossRef CAS.
- H. Vollstaedt, A. Eisenhauer, K. Wallmann, F. Böhm, J. Fietzke, V. Liebetrau, A. Krabbenhöft, J. Farkaš, A. Tomašových, J. Raddatz and J. Veizer, The Phanerozoic δ88/86Sr record of seawater: new constraints on past changes in oceanic carbonate fluxes, Geochim. Cosmochim. Acta, 2014, 128, 249–265 CrossRef CAS.
- J. Voigt, E. C. Hathorne, M. Frank, H. Vollstaedt and A. Eisenhauer, Variability of carbonate diagenesis in equatorial Pacific sediments deduced from radiogenic and stable Sr isotopes, Geochim. Cosmochim. Acta, 2015, 148, 360–377 CrossRef CAS.
- N. Fruchter, A. Eisenhauer, M. Dietzel, J. Fietzke, F. Böhm, P. Montagna, M. Stein, B. Lazar, R. Rodolfo-Metalpa and J. Erez, 88Sr/86Sr fractionation in inorganic aragonite and in corals, Geochim. Cosmochim. Acta, 2016, 178, 268–280 CrossRef CAS.
- K. P. Jochum, U. Nohl, K. Herwig, E. Lammel, B. Stoll and A. W. Hofmann, GeoReM: a new geochemical database for reference materials and isotopic standards, Geostand. Geoanal. Res., 2005, 29, 333–338 CrossRef CAS.
- A. O. Nier, The Isotopic Constitution of Strontium, Barium, Bismuth, Thallium and Mercury, Phys. Rev., 1938, 5, 275–278 CrossRef.
-
D. Ballesteros, A. Painchault, B. Puente-Berdasco, C. Nehme, D. Todisco, J. I. Garcia Alonso, M. Varano and D. Mouralis, Sourcing of the Chalkstone Used for Medieval Building in Eastern Duchy of Normandy (10th-14th Centuries), submitted for publication Search PubMed.
- D. Ballesteros, A. Painchault, C. Nehme, D. Todisco, M. Varano and D. Mouralis, Normandy chalkstone (France): geology and historical uses from quarries to monuments, Episodes, 2021, 44, 31–42 CrossRef.
- E. Vasanelli, M. Sileo, A. Calia and M. A. Aiello, Non-destructive Techniques to Assess Mechanical and Physical Properties of Soft Calcarenitic Stones, Procedia Chem., 2013, 8, 35–44 CrossRef.
- M. Al Heib, C. Duval, F. Theoleyre, J. M. Watelet and P. Gombert, Analysis of the historical collapse of an abandoned underground chalk mine in 1961 in Clamart (Paris, France), Bull. Eng. Geol. Environ., 2015, 74, 1001–1018 CrossRef CAS.
-
J. M. McArthur, R. J. Howarth and G. A. Shields, Strontium isotope stratigraphy, in The Geologic Time Scale, ed.F. M. Gradstein, G. G. Ogg, M. Chmitz and G. Ogg, Elsevier, Amsterdam, 2012, pp. 127–144 Search PubMed.
|
This journal is © The Royal Society of Chemistry 2022 |
Click here to see how this site uses Cookies. View our privacy policy here.