DOI:
10.1039/D2MA00316C
(Paper)
Mater. Adv., 2022,
3, 5796-5806
Photocatalytic dye degradation using BiVO4–paint composite coatings†
Received
19th March 2022
, Accepted 24th May 2022
First published on 14th June 2022
Abstract
Bismuth vanadate (BiVO4)–paint composites coated on the aluminium sheet surface were fabricated with different BiVO4 amounts (0, 20, and 40 wt%) in paint. The degradation capability of the methylene blue (MB) dye using the BiVO4–paint coated sample was explored. The impact of BiVO4 content on the dye degradation performance was analyzed. The fabricated materials were characterized using scanning electron microscopy (SEM) and X-ray diffraction (XRD). XRD confirmed the existence of the BiVO4 crystalline phase in paint. The elemental composition of the sample was determined using X-ray electron diffraction spectroscopy (EDS) inbuilt in SEM. The absorption spectra of BiVO4 was determined using an UV-Vis-NIR spectrophotometer. As the content of BiVO4 in the BiVO4–paint sample increases, there is an increase in the dye degradation efficiency. The 40 wt%-BiVO4–paint sample exhibited an ∼72% dye degradation efficiency as compared to the 0 wt%-BiVO4–paint sample (∼27%) in a duration of 240 min of the photocatalysis experiment. The photocatalytic MB dye degradation is in accordance with the pseudo-first-order kinetics attaining the largest k value of 0.00524 min−1. Photocatalytically treated water after the photocatalysis experiment falls in the moderate phytotoxicity level. A germination index study was done using Vigna radiata seeds.
1. Introduction
Clean and safe water is considered one of the most essential natural resources for the survival of living beings on earth.1,2 Due to rapid urbanization, there incurred an increase in water contamination due to effluents discharged directly to water bodies.3 Moreover, industries such as textile, pharmaceuticals, mining, etc. also discharge polluted water in enormous quantities directly to the environment.4 The release of dyes from the textile industry directly into the environment is a concern. Dyes are harmful pollutants for living beings because of their toxic and carcinogenic nature.5 So, getting safe water is a global concern at present. In this regard, various water treatment methods such as biological, physical, and chemical processes have been adopted to overcome these issues.6–10 The recalcitrant nature of a few pollutants limits the use of biological treatment processes,11,12 and at the same time chlorination, ozonation and high cost retard the usage of chemical processes for water treatment.12,13 Physical techniques including coagulation, absorption, ion exchange, and ultrafiltration cause secondary pollutant byproducts or simply transfer pollutants from one phase to another. So this may cause an increase in cost for further treatment processes.14–16 Photocatalysis is considered one of the efficient water treatment processes in recent years owing to its several advantages such as no waste byproducts, environmental friendliness, low cost, and complete mineralization.17–19 When the surface of a semiconductor photocatalyst material absorbs photon energy, there is the generation of electrons in the conduction band (CB) and holes in the valence band (VB). These generated electrons and holes further react with the water molecules generating highly active superoxide and hydroxyl radicals which further degrade the organic pollutants present in the contaminated water to carbon dioxide and water as end products.20,21 In recent years, several photocatalyst materials such as TiO2, ZnO, BiVO4, WO3, ZnS, CeO2, etc. have been reported.22–25 TiO2 displays excellent photocatalytic activity but its usage is restricted due to its wide bandgap (3.2 eV) which needs ultraviolet (UV) light as a source for activation.26 ZnO has a bandgap of 3.37 eV, the usage of which is limited by UV light irradiation.27,28 Visible light occupies ∼45% of solar light, while UV light occupies ∼3–5%.29 This led to the search for new photocatalytic materials that can be visible light active. In this context, BiVO4 would be a better option as a photocatalyst as it possesses the unique property of a narrow bandgap (2.4 eV), corrosion resistance, non-toxicity, stability, and good dispersibility.30–34 Based on density functional theory (DFT) calculations, researchers have claimed that the VB of monoclinic bismuth vanadate (m-BiVO4) constitutes Bi-6s and O-2p orbitals, while the CB constitutes V-3d orbitals.35–37 In the monoclinic scheelite structure, the 6s state of Bi3+ is situated above the O-2p orbital, resulting in reduced bandgap and viable transition from Bi-6s2 to V-3d. Walsh et al. used DFT calculations to demonstrate that hybridization among Bi-6s and O-2p states at the apex of the VB is the reason for the reduced bandgap for m-BiVO4. It showed that BiVO4 possesses a direct bandgap.38 The monoclinic scheelite structure is reported to give the best visible light photocatalytic degradation.39 BiVO4 can be synthesized through the hydrothermal method, microwave-assisted methods, co-precipitation, solid-state synthesis, and sono-chemical method.40–43
Paints are considered one of the important construction materials among steel, window glass, cement, insulation materials, etc. Engineered material usage is encouraged to improve existing conventional products or to improve or create products with new functions and applications. SiO2, Fe2O3, Al2O3, carbon-nanotubes (CNTs), and ZrO2 have been utilized to enhance the longevity and quality of structures.44,45 Incorporating materials such as Ag, CNTs, SiO2, ZnO, CeO2, Fe2O3, and TiO2 in paints can enhance properties like easy cleaning, UV-protection, scratch resistance, anti-corrosion, antibacterial activity, fire resistance, and wood preservation.46–49 Paints are applied to protect substrates from environmental effects and for appealing needs. It is an economical way to safeguard metals from getting corroded compared to other surface protection methods.50 Coatings are applied as thin layers over substrates like metals and wood as a protection against corrosion and for decoration.51 Incorporating different materials into paints have been reported to improve their UV resistance,52 rheological properties,53 coloring,54 and mechanical properties (SiO2).55 Paints consist of different components like binders, pigments, solvents, fillers, diluents, and several additives such as thickeners, antifoam agents, and dispersing additives.56 TiO2 embedded in paint has been reported to induce a photocatalytic effect for reducing nitrogen oxides and volatile organic compounds present as air pollutants in the environment.57–60 Photocatalytic paints have self-cleaning properties since they tend to remove unwanted substances from the surface using the photon energy of light.61 Paints usually use pigments such as TiO2 which are photocatalytically inactive, suppressing the possibility of photo-oxidization of the polymer binder, thereby causing photochalking. The paint pigments comprise non-active rutile titania particles which are surface-treated with silica or alumina, ensuring photocatalytic passivity. Such pigments stabilize paints to be used as an exterior coating, thereby blocking solar UV radiation.62,63 Baudys et al. have suggested exposing the sample containing acrylate or silicate binders for a certain weathering time, causing degradation of the polymer binder, and at the same time would unblock the photocatalyst surface to avail better photocatalytic activity. The stability of the polymer binder should be analyzed to obtain paints with photocatalytic activity and a reasonable lifetime.63 Islam et al. have recently reported a photocatalytic paint based on a photopolymer resin and TiO2 for degrading organic pollutants in water.64 Bonnefond et al. have reported stable photocatalytic paints incorporating TiO2 and an acrylic polymer for bacterial disinfection.65,66 Microspheres of TiO2 incorporated in acrylic paints have been reported for photocatalytic methylene blue (MB) dye degradation.67 Only a few reports are available regarding photocatalytic coatings and paints. Research on the use of paints for photocatalysis has not been much explored, which needs further investigation.
In this study, we intend to explore a photocatalyst material that can be visible light active, economical, and free from the quest to recover the catalyst and at the same time the support utilized should possess corrosion, abrasion, and acidic particle resistance. The simple preparation method, possibility of large-scale applicability on various substrates, and being devoid of hazardous chemicals and no need for the use of sophisticated instruments make this study remarkable. We explored MB dye degradation in visible light using BiVO4–paint composites coated on a rectangular aluminium sheet where the aluminium sheet acts as a support/substrate. Adhesion of paints incorporated with BiVO4 over the surface of the aluminium sheet as a coating is easy.68 The photocatalyst will be used in wastewater so corrosion of the substrate needs consideration. Aluminium is known to possess high anti-corrosion properties, abrasion resistance, and acidic particle resistance, so it has been selected as the substrate.69,70 Paint is a commonly used construction material and can also adhere to the substrate easily so it can be considered as an ideal material to load the photocatalyst. The usage of a paint-coated substrate for wastewater remediation through the photocatalysis experiment using visible light irradiation would be both economical as well as free from separation and filtration processes to recover the catalyst. The BiVO4–paint composite coated on an aluminium substrate for photocatalytic MB dye degradation has not been explored so far.
2. Materials and methods
2.1 Fabrication of BiVO4
Oxide powders of Bi2O3 and V2O5 in a stoichiometric molar ratio were homogeneously mixed manually through the use of an agate mortar pestle for approximately 30 minutes. Once the powders were homogeneously mixed, the mixture was subjected to calcination at 700 °C for 8 hours by placing in a furnace to get the pure BiVO4 (BiV) phase.
2.2 Fabrication of BiV-paint
BiV-paint samples were prepared by coating the catalyst on the rectangular aluminium sheet as shown in Scheme 1. The dimensions of the aluminium substrate used were 20 mm × 20 mm × 1 mm. The aluminium substrate was cleaned with distilled (DI) water and acetone thrice to eradicate any contamination present over its surface. Berger Luxol high gloss enamel paint was used as a coat on the substrate. For fabrication of the BiV-paint sample, BiVO4 and paint were mixed to form a slurry, where 0%, 20 wt%, and 40 wt% of BiVO4 was added to the paint. Here the calculation for the coating was done for 0.8 g in which specified wt% of the BiVO4 catalyst was mixed. The slurry was hand-mixed for approx. 10 minutes to obtain a homogenous mixture using a brush. The slurry of BiVO4 and paint was then applied on both sides of the rectangular aluminium sheets using a brush in order to obtain a uniform coating. The coated sample was covered and kept safely for drying in the environment for 5 days. The obtained dried samples with 0%, 20 wt%, and 40 wt% were named paint, 20BiV-paint, and 40BiV-paint, respectively.
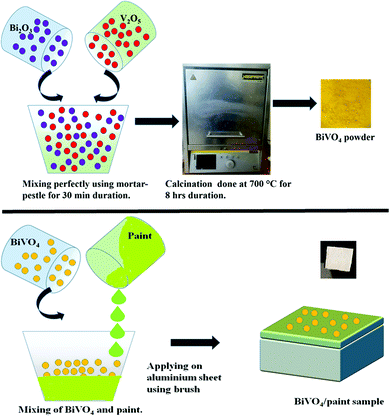 |
| Scheme 1 Schematic illustration showing the synthesis method adopted for BiV-paint. | |
2.3 Characterization of BiV-paint
The crystalline structural analysis of the prepared BiVO4–paint composite was conducted using an X-ray diffractometer (XRD) (Rigaku, Japan (9 kW Cu-Kα anode)). The sample was scanned at 3° min−1 over the 10–80° range. The surface morphology and microstructures of the paint sample were analyzed using a Nova Nano SEM-450 field emission scanning electron microscope (FE-SEM). The elemental chemical composition of the sample was analyzed using X-ray electron diffraction spectroscopy (EDS) which is inbuilt in SEM. The photoluminescence spectra of the samples were recorded using a LabRAM HR Evolution photoluminescence spectrometer (HORIBA, Japan) in the scan range of 350 to 650 nm. An excitation wavelength of 325 nm was used to record the spectra. The absorption spectra of BiVO4 were determined using a UV-Vis-NIR spectrophotometer (Shimadzu UV-3150a).
2.4 Photocatalytic experiment
Each of the obtained paint, 20BiV-paint, and 40BiV-paint samples was utilized to degrade MB dye solution under visible light illumination with the use of 2 Havells bulbs (15 W each). Further, 0.11 g of BiVO4 powder was subjected to the photocatalysis experiment and its degradation capability was analyzed. Prior to the start of the experiment, it was made sure to achieve adsorption–desorption saturation for the MB dye and the samples by employing continuous stirring (500 rpm) in a dark environment. On the attainment of the adsorption saturation, the used dye was replaced with a fresh 10 ml dye with an initial concentration of ∼5 mg L−1. After finishing 30 min of the photocatalysis experiment, each time a 1 mL solution was taken from the beaker in order to quantify the dye degradation, and on completion of the quantification, it was replenished back to the beaker so as to sustain the constant volume of dye in the beaker. The dye removal percentage was analyzed using eqn (1):71 |  | (1) |
where Co and C denote the initial dye concentration and that obtained after time duration ‘t’, respectively.
Diffuse reflectance spectroscopy (DRS) was conducted to obtain the absorption spectra of the BiVO4 powder which was further transformed into Tauc plots (plot of (αE)2vs. E) to obtain a direct band gap.72,73
2.5 Phytotoxicity test
Distilled (DI) water and sample water collected after 0 and 4 h of photocatalysis were used to conduct seed germination and root length tests. This test determines the sustainability and suitability of the degraded MB dye water post photocatalysis for seed (Vigna radiata) germination. This would help in understanding the reuse of the collected water after the photocatalysis experiment. For testing, 3 vials each containing 10 seeds of Vigna radiata were taken. The vials were sprinkled everyday with 0.5 ml of treated, untreated, and DI water. The test was conducted in Mandi, India where the environmental temperature was 25 °C. The results were analyzed 7 days after the test. The evaluation of the outcome was done after completion of the duration of 7 days. AFNOR ISO 17126 was followed to determine the root length and seed germination counts.74 The germination index was evaluated using eqn (2):75 |  | (2) |
3. Results and discussion
Fig. 1 presents the XRD plots of the BiV, paint, 20BiV-paint, and 40BiV-paint samples. The prepared samples are of a high crystalline quality which can be observed through the presence of sharp XRD peaks. The BiV, 20BiV-paint, and 40BiV-paint samples demonstrate very good agreement with the pure monoclinic phase of BiVO4 following the standard reference JCPDS 01-075-1866. The XRD results indicate the complete phase formation of the BiVO4 powder sample. All the major peaks of BiVO4 can be easily identified in 20BiV-paint and 40BiV-paint samples. The presence of additional peaks in 20BiV-paint and 40BiV-paint samples is due to other constituents of the paint. Paint may possess many metal oxides such as Al2O3, SiO2, CaO, TiO2, etc.76 As the exact constituents of paints are not readily available, unknown peaks have not been identified.
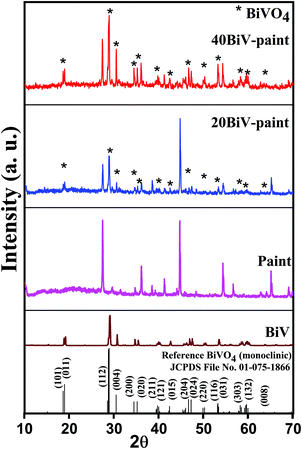 |
| Fig. 1 XRD plots of BiV, paint, 20BiV-paint, and 40BiV-paint samples. | |
The SEM images of BiVO4, paint, and 40BiV-paint were taken to demonstrate their surface morphology as shown in Fig. 2(a–c). Fig. 2(a) demonstrates the irregular shape morphology of the BiVO4 particles. Fig. 2(b and c) demonstrate the microstructures of the paint and 40BiV-paint samples. The presence of Bi, V, and O along with other elements in 40BiV-paint can be observed through the EDS elemental color map as shown in Fig. 2(d and e). The EDS spectrum shown in Fig. 2(f) confirms the presence of C, O, Al, Si, Bi, V, Ca, and Ti elements in the 40BiV-paint sample.
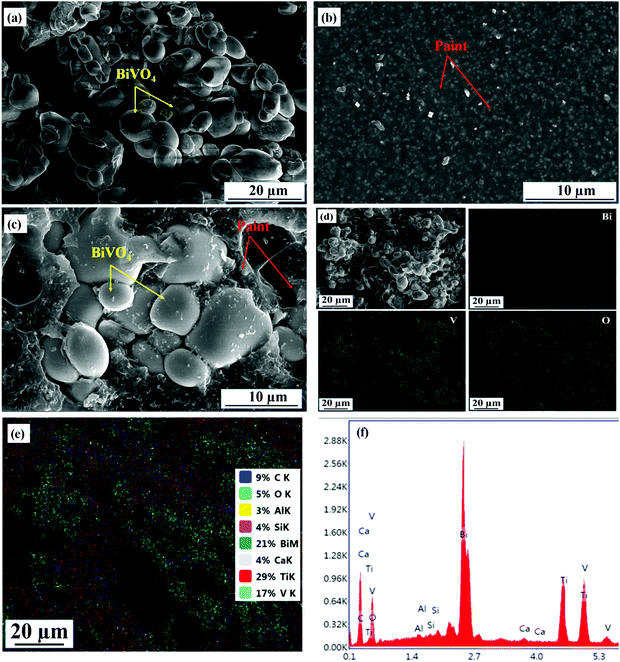 |
| Fig. 2 SEM micrographs of (a) BiV, (b) paint, and (c) 40BiV-paint; (d and e) EDS color mapping of 40BiV-paint; and (f) EDS spectrum obtained for the 40BiV-paint sample. | |
In order to determine the applicability of BiVO4 powder for the photocatalysis experiment in visible light, the DRS spectrum of the BiVO4 powder sample was recorded. The adsorption edge of the BiV powder and its explored bandgap (2.42 eV) are displayed in Fig. 3(a) and (b), respectively. The absorption edges of 40BiV-paint and paint are shown in the inset of Fig. 3(a). The inset of Fig. 3(b) shows the explored bandgaps of 40BiV-paint and paint as 2.1 eV and 2.07 eV, respectively. Paint may constitute many different elements so its bandgap value is low. The obtained bandgap lies in the visible region so this affirms that BiV powder is suitable for photocatalytic dye degradation experiments in visible light.
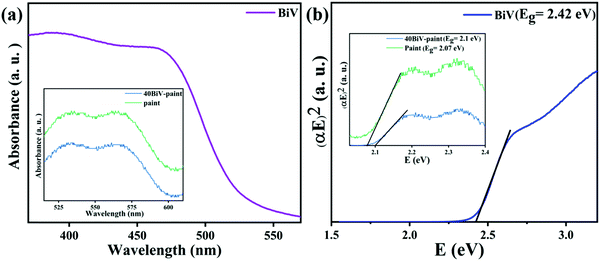 |
| Fig. 3 (a) UV-vis absorption spectrum of BiV powder (inset graph shows the absorption edge of 40BiV-paint and paint) and (b) bandgap of BiV using Tauc plots (inset graph shows the bandgap of 40BiV-paint and paint). | |
Fig. 4 shows the recorded photoluminescence spectra of BiV, 40BiV-paint, and paint using an excitation wavelength of 325 nm. A high PL intensity denotes a higher rate of electron–hole pair recombination.77 A lower PL intensity value indicates that there exists a higher electron transfer efficiency which increases the degradation of contaminants and thus enhances the photocatalytic efficiency.78,79 It can be seen that there is a gradual decrease in the PL intensity. The PL intensity of 40BiV-paint is lower than that of paint which suggests that electron–hole pair recombination is suppressed in the case of 40BiV-paint. A very low PL intensity of BiVO4 may be due to the formation of structural defects during calcination which traps the electrons.80 This can be viewed in the PL spectra of BiV.
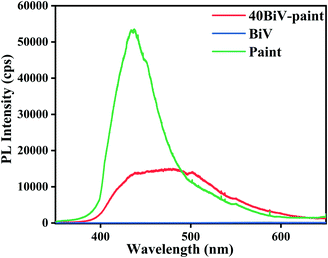 |
| Fig. 4 Photoluminescence spectra of 40BiV-paint, BiV, and paint. | |
The obtained BiV powder was used as a catalyst for degrading MB dye through the photocatalysis experiment and the obtained results are shown in Fig. 5(a). Prior to the start of the photocatalysis experiment, it was made sure that adsorption saturation was attained carefully. Fig. 5(a) reveals that as the irradiation time is elevated there occurs a substantial decrease in the peak intensity of the MB dye, which affirms the degradation of the MB dye. Fig. 5(b) shows the
vs. time plots attained with and without sample usage during the photocatalysis experiment. According to the results, without sample use (control) ∼27% dye degradation is attained in 240 min, while with BiV powder usage the attained degradation percentage raises to ∼53% within 240 min of visible light illumination. There is a 26% more degradation efficiency of MB dye as compared to that of the control sample. This approves the good photocatalytic ability of BiVO4.
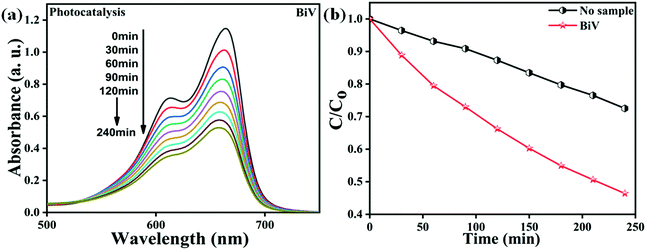 |
| Fig. 5 (a) The acquired absorption spectra from the photocatalysis using BiV powders and (b) vs. time plots acquired from the photocatalysis with and without any sample use. | |
The obtained 40BiV-paint sample was used as a catalyst for degrading MB dye through the photocatalysis experiment and the obtained results are shown in Fig. 6(a). Fig. 6(a) shows that as the irradiation time is elevated there occurs a substantial decrease in the peak intensity of the MB dye, which affirms MB dye degradation. Fig. 6(b) displays the
vs. time plots attained with paint, 20BiV-paint, and 40BiV-paint and without sample usage during the photocatalysis experiment. The degradation efficiency attained without the use of the sample (control) is ∼27%, while with the use of paint, 20BiV-paint, and 40BiV-paint samples it raises to 50%, 67%, and 72%, respectively, in 240 minutes of visible light irradiation. Here, 20BiV-paint and 40BiV-paint samples attain improvements of 40% and 45%, respectively, in the degradation efficiency as compared to that attained by the control sample. The crystalline peaks in the paints would have contributed to attain 23% more degradation of the dye than the control sample. Improvements of 17% and 22% attained by 20BiV-paint and 40BiV-paint samples, respectively, would be due to the BiVO4 catalyst loading. Fig. 6(c) shows the
vs. time plots for different MB dye concentrations (5, 10, and 12.5 mg L−1). In order to know the active radicals present during the photocatalysis experiment, isopropanol (IPA), ethylenediaminetetraacetic acid (EDTA), and p-benzoquinone (p-BQ) scavengers were appended individually in the MB dye solution with a view to seize the different active radicals like hydroxyl radicals (˙OH), holes (h+) and superoxide radicals (˙O2−), respectively.81Fig. 6(d) shows that the BQ scavenger that scavenges O2˙− radicals has a major influence on the photocatalytic dye degradation efficiency.81,82 This confirms O2˙− radicals as the major active and dominant species in the commencing photocatalytic dye degradation experiment.
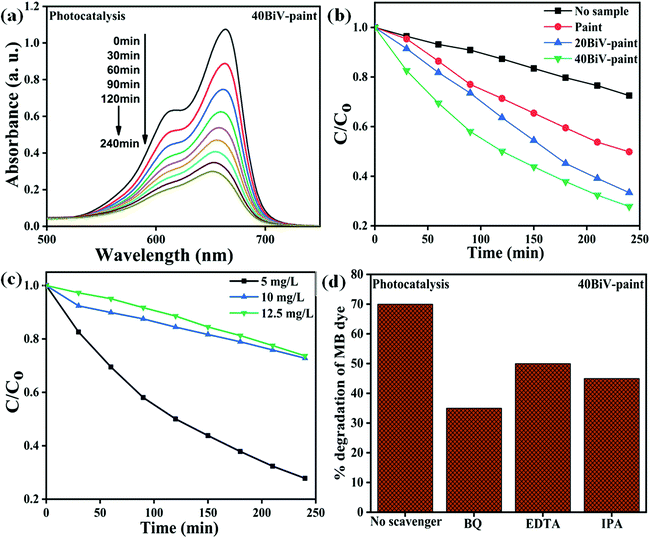 |
| Fig. 6 (a) Absorption spectra acquired from the photocatalysis using the 40BiV-paint sample, (b) vs. time plots acquired from photocatalysis with and without any sample use, (c) vs. time plots acquired from photocatalysis using the 40BiV-paint sample at different MB dye concentrations (5, 10, and 12.5 mg L−1), and (d) effect of different scavengers on MB dye degradation efficiency acquired from the photocatalysis experiment using the 40BiV-paint sample. | |
Fig. 7(a) shows the −
vs. time plots attained during the photocatalytic experiment with different MB dye concentrations using the 40BiV-paint sample. The MB dye degradation reaction during photocatalysis is in accordance with the pseudo first-order kinetics as given in eqn (3):83–85
|  | (3) |
where ‘
k’ represents the kinetic rate constant, which is calculated from the slope of the
vs. time ‘
t’ linear plot. The 40BiV-paint sample attains the largest
k value of 0.00524 min
−1 in 5 mg L
−1 dye and showed a decline in the
k value as the MB dye concentration is elevated.
Fig. 7(b) shows the plots of kinetic rate constant ‘
k’
vs. different dye concentrations (5, 10, and 12.5 mg L
−1).
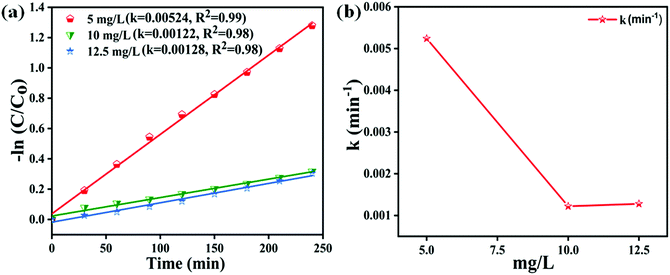 |
| Fig. 7 (a) − vs. time plots for various MB dye concentrations (mg L−1) and (b) plots of kinetic rate constant ‘k’ vs. varied dye concentrations during photocatalysis. | |
For conducting the germination index test, 3 vials each containing 10 seeds of Vigna radiata were taken. The vials were sprinkled everyday with 0.5 ml of treated, untreated, and DI water. Fig. 8(a–c) show the growth of seeds using dye water collected before photocatalysis, treated water collected after 4 h of photocatalysis, and distilled water. According to the results, 5 mg L−1 untreated dye water causes more hindrance for the seed growth as compared to the DI and treated water. Although the seed growth using treated water lies in the non-toxic approach, precaution still needs to be taken in the case of edible plants owing to the associated harmful adverse effects.74 A better suggestion would be to utilize the treated waste water for irrigating parks and playground which would surely decrease the burden on natural water need.86Fig. 8(d) displays the results of the phytotoxicity test. For a better understanding of the compounds on the basis of GI values, a major classification has been made: high phytotoxicity (GI < 50%), moderate phytotoxicity (
), and the absence of phytotoxicity (GI > 80%). This toxicity level classification adheres to the criteria as suggested by Emino et al.87 and Zucconi et al.75 The results suggest that untreated dye water falls in the level of high toxicity, while treated water after the photocatalysis experiment falls in moderate phytotoxicity.75 For the GI test treated water after the photocatalysis experiment were taken in use. According to the results, untreated dye water lies in high toxicity, while photocatalytically treated water lies at the level of moderate phytotoxicity. Treated water used for the GI test is the same dye water that attained 58% dye degradation efficiency after the photocatalysis experiment. Thus, further improvement in the efficiency to 100% clean wastewater is also attainable by an increase in catalyst load, an increase in degradation time duration and a decrease in dye concentration.88,89
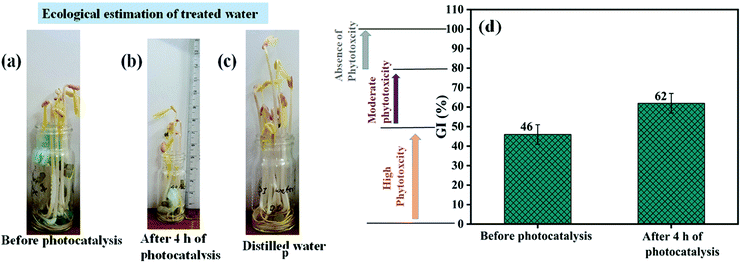 |
| Fig. 8 The impact of the MB dye on the growth of the Vigna radiata seeds explored for 7 days; the treatment is done using (a) 5 mg L−1 MB dye, (b) treated wastewater and (c) distilled water; (d) germination index test done on two samples after 0 and 4 h of photocatalysis. | |
Fig. 9 presents the schematic mechanism of the BiV-paint sample undergoing MB dye degradation during the photocatalysis experiment. The inset of Fig. 9 shows the optical transition of m-BiVO4. As visible light falls on BiVO4, electron–hole pairs are generated on the BiVO4 surface. This causes the excitation of VB electrons to the CB while leaving holes in the VB. There is an optical transition of m-BiVO4 from Bi 6s–O 2p hybrid orbitals to vacant V 3d orbitals which results in a reduced bandgap of 2.4 eV. Coupling among V 3d, O 2p, and Bi 6s causes a lowering of the conduction band minimum. These interactions form symmetric electron and hole masses and thus assist in efficient toxic carrier separation.38 The generated holes cause oxidation of the adsorbed water producing hydroxyl radicals (OH˙), while the generated electrons react with the adsorbed oxygen (O2) producing superoxide radicals (O2˙−). These (O2˙− and OH˙) radicals are further called as reactive oxygen species (ROS) which lead to MB dye degradation to harmless products.90–92 Thus, the BiV-paint sample demonstrates photocatalytic activity.
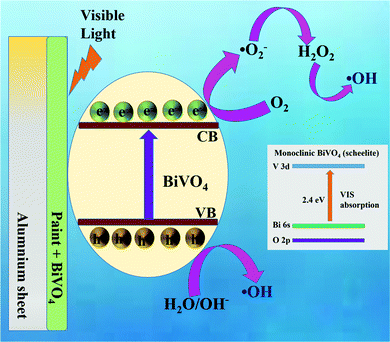 |
| Fig. 9 Schematic mechanism for photocatalytic MB dye degradation using the BiV-paint sample. | |
4. Conclusions
The dye degradation capability of bismuth vanadate mixed in paint during the photocatalysis experiment in visible light was studied. As the content of BiVO4 in the BiVO4–paint sample increases, there is an increase in the dye degradation efficiency. The 40 wt%-BiVO4–paint sample exhibited an ∼72% dye degradation efficiency as compared to the 0 wt%-BiVO4–paint sample (∼27%) in a duration of 240 min of the photocatalysis experiment. The photocatalytic MB dye degradation is in accordance with the pseudo-first-order kinetics with 0.00524 min−1 as the largest k value attained. Photocatalytically treated water after the photocatalysis experiment falls in the moderate phytotoxicity level. The usage of paint coated substrates for wastewater remediation through the photocatalysis experiment using visible light irradiation would be both economical as well as free from separation and filtration processes to recover the catalyst.
Data availability
All data are available within this manuscript.
Conflicts of interest
The authors proclaim of having no conflicts of interest.
References
- M. Bodzek and M. Rajca, Photocatalysis in the treatment and disinfection of water. Part I. Theoretical backgrounds/Fotokataliza w oczyszczaniu i dezynfekcji wody część i. podstawy teoretyczne, Ecol. Chem. Eng. S, 2012, 19(4), 489–512 CAS.
- S. S. Shinde, C. H. Bhosale and K. Y. Rajpure, Photocatalytic activity of sea water using TiO2 catalyst under solar light, J. Photochem. Photobiol., B, 2011, 103(2), 111–117 CrossRef CAS PubMed.
- F. Li, C. Yang, Q. Li, W. Cao and T. Li, The pH-controlled morphology transition of BiVO4 photocatalysts from microparticles to hollow microspheres, Mater. Lett., 2015, 145, 52–55 CrossRef CAS.
- C. P. Sajan, S. Wageh, A. Al-Ghamdi, J. Yu and S. Cao, TiO2 nanosheets with exposed {001} facets for photocatalytic applications, Nano Res., 2016, 9(1), 3–27 CrossRef CAS.
- V. P. Singh and R. Vaish, Cement-based diesel exhaust emission soot coatings for the removal of organic pollutants from water, Constr. Build. Mater., 2020, 234, 117377 CrossRef.
- T. Robinson, G. McMullan, R. Marchant and P. Nigam, Remediation of dyes in textile effluent: a critical review on current treatment technologies with a proposed alternative, Bioresour. Technol., 2001, 77(3), 247–255 CrossRef CAS.
- S. Ye, M. Yan, X. Tan, J. Liang, G. Zeng and H. Wu,
et al., Facile assembled biochar-based nanocomposite with improved graphitization for efficient photocatalytic activity driven by visible light, Appl. Catal., B, 2019, 250, 78–88 CrossRef CAS.
- S. Ye, G. Zeng, H. Wu, J. Liang, C. Zhang and J. Dai,
et al., The effects of activated biochar addition on remediation efficiency of co-composting with contaminated wetland soil, Resour., Conserv. Recycl., 2019, 140, 278–285 CrossRef.
- S. Ye, G. Zeng, H. Wu, C. Zhang, J. Dai and J. Liang,
et al., Biological technologies for the remediation of co-contaminated soil, Crit. Rev. Biotechnol., 2017, 37(8), 1062–1076 CrossRef CAS PubMed.
- S. Ye, G. Zeng, H. Wu, C. Zhang, J. Liang and J. Dai,
et al., Co-occurrence and interactions of pollutants, and their impacts on soil remediation—a review, Crit. Rev. Environ. Sci. Technol., 2017, 47(16), 1528–1553 CrossRef.
- K. Rajeshwar, M. E. Osugi, W. Chanmanee, C. R. Chenthamarakshan, M. V.-B. Zanoni and P. Kajitvichyanukul,
et al., Heterogeneous photocatalytic treatment of organic dyes in air and aqueous media, J. Photochem. Photobiol., C, 2008, 9(4), 171–192 CrossRef CAS.
- V. K. Gupta, I. Ali, T. A. Saleh, A. Nayak and S. Agarwal, Chemical treatment technologies for waste-water recycling—an overview, RSC Adv., 2012, 2(16), 6380–6388 RSC.
- H. M. Coleman, B. R. Eggins, J. A. Byrne, F. L. Palmer and E. King, Photocatalytic degradation of 17-β-oestradiol on immobilised TiO2, Appl. Catal., B, 2000, 24(1), L1–L5 CrossRef CAS.
- S. Esplugas, D. M. Bila, L. G.-T. Krause and M. Dezotti, Ozonation and advanced oxidation technologies to remove endocrine disrupting chemicals (EDCs) and pharmaceuticals and personal care products (PPCPs) in water effluents, J. Hazard. Mater., 2007, 149(3), 631–642 CrossRef CAS PubMed.
- P. R. Gogate and A. B. Pandit, A review of imperative technologies for wastewater treatment II: hybrid methods, Adv. Environ. Res., 2004, 8(3–4), 553–597 CrossRef CAS.
- H. K. Moo-Young, Pulp and paper effluent management, Water Environ. Res., 2007, 79(10), 1733–1741 CrossRef CAS.
- D. S. Bhatkhande, V. G. Pangarkar and A. A. C. M. Beenackers, Photocatalytic degradation for environmental applications–a review, J. Chem. Technol. Biotechnol., 2002, 77(1), 102–116 CrossRef CAS.
- W. Ma, J. Li, X. Tao, J. He, Y. Xu and J. C. Yu,
et al., efficient degradation of organic pollutants by using dioxygen activated by resin-exchanged iron(II) bipyridine under visible irradiation, Angew. Chem., 2003, 115(9), 1059–1062 CrossRef.
- M. Kumar, M. N.-M. Ansari, I. Boukhris, M. S. Al-Buriahi, Z. A. Alrowaili and N. Alfryyan,
et al., Sonophotocatalytic Dye Degradation Using rGO-BiVO4 Composites, Glob Challenges, 2022, 2100132 CrossRef.
- T. Banerjee and A. Mukherjee, Overall water splitting under visible light irradiation using nanoparticulate RuO2 loaded Cu2O powder as photocatalyst, Energy Procedia, 2014, 54, 221–227 CrossRef CAS.
- C. Regmi, B. Joshi, S. K. Ray, G. Gyawali and R. P. Pandey, Understanding mechanism of photocatalytic microbial decontamination of environmental wastewater, Front. Chem., 2018, 6, 33 CrossRef PubMed.
- A. R. Khataee and M. B. Kasiri, Photocatalytic degradation of organic dyes in the presence of nanostructured titanium dioxide: Influence of the chemical structure of dyes, J. Mol. Catal. A: Chem., 2010, 328(1–2), 8–26 CrossRef CAS.
- Y. Guo, L. Chen, F. Ma, S. Zhang, Y. Yang and X. Yuan,
et al., Efficient degradation of tetrabromobisphenol A by heterostructured Ag/Bi5Nb3O15 material under the simulated sunlight irradiation, J. Hazard. Mater., 2011, 189(1–2), 614–618 CrossRef CAS PubMed.
- S. Janitabar-Darzi and A. R. Mahjoub, Investigation of phase transformations and photocatalytic properties of sol–gel prepared nanostructured ZnO/TiO2 composites, J. Alloys Compd., 2009, 486(1–2), 805–808 CrossRef CAS.
- S. Chakrabarti and B. K. Dutta, Photocatalytic degradation of model textile dyes in wastewater using ZnO as semiconductor catalyst, J. Hazard. Mater., 2004, 112(3), 269–278 CrossRef CAS PubMed.
- H. Golmojdeh and M. A. Zanjanchi, A facile approach for synthesis of BiVO4 nano-particles possessing high surface area and various morphologies, Cryst. Res. Technol., 2012, 47(9), 1014–1025 CrossRef CAS.
- L. Järup, Hazards of heavy metal contamination, Br. Med. Bull., 2003, 68(1), 167–182 CrossRef PubMed.
- A. Kołodziejczak-Radzimska and T. Jesionowski, Zinc oxide—from synthesis to application: a review, Materials, 2014, 7(4), 2833–2881 CrossRef PubMed.
- X. Liu, F. Wang and Q. Wang, Nanostructure-based WO3 photoanodes for photoelectrochemical water splitting, Phys. Chem. Chem. Phys., 2012, 14(22), 7894–7911 RSC.
- A. Kudo, K. Omori and H. A. Kato, Phosphate Doping Into Monoclinic BiVO4 for Enhanced Photoelectrochemiczd Water Oxidation Activity, J. Am. Chem. Soc., 1999, 121(49), 11459–11467 CrossRef CAS.
- M. Guo, Y. Wang, Q. He, W. Wang, W. Wang and Z. Fu,
et al., Enhanced photocatalytic activity of S-doped BiVO4 photocatalysts, RSC Adv., 2015, 5, 58633–58639 RSC.
- Y. Hu, J. Fan, C. Pu, H. Li, E. Liu and X. Hu, Facile synthesis of double cone-shaped Ag4V2O7/BiVO4 nanocomposites with enhanced visible light photocatalytic activity for environmental purification, J. Photochem. Photobiol., A, 2017, 337, 172–183 CrossRef CAS.
- G. Singh, M. Kumar and R. Vaish, Promising multicatalytic and adsorption capabilities in V2O5/BiVO4 composite pellets for water-cleaning application, Surf. Interfaces, 2021, 23(November 2020), 100924, DOI:10.1016/j.surfin.2021.100924 , available from: .
- M. Kumar, G. Singh and R. Vaish, A reduced graphene oxide/bismuth vanadate composite as an efficient piezocatalyst for degradation of organic dye, Mater. Adv., 2021, 2(12), 4093–4101 RSC.
- J. K. Cooper, S. Gul, F. M. Toma, L. Chen, Y.-S. Liu and J. Guo,
et al., Indirect bandgap and optical properties of monoclinic bismuth vanadate, J. Phys. Chem. C, 2015, 119(6), 2969–2974 CrossRef CAS.
- Y. Hermans, A. Klein, K. Ellmer, R. van de Krol, T. Toupance and W. Jaegermann, Energy-band alignment of BiVO4 from photoelectron spectroscopy of solid-state interfaces, J. Phys. Chem. C, 2018, 122(36), 20861–20870 CrossRef CAS.
- J. K. Cooper, S. Gul, F. M. Toma, L. Chen, P. A. Glans and J. Guo,
et al., Electronic structure of monoclinic BiVO4, Chem. Mater., 2014, 26(18), 5365–5373 CrossRef CAS.
- A. Walsh, Y. Yan, M. N. Huda, M. M. Al-Jassim and S.-H. Wei, Band edge electronic structure of BiVO4: elucidating the role of the Bi s and V d orbitals, Chem. Mater., 2009, 21(3), 547–551 CrossRef CAS.
- M. Kumar, R. Vaish and S. ben Ahmed, Piezo-photocatalytic activity of mechanochemically synthesized BiVO4 for dye cleaning, J. Am. Ceram. Soc., 2022 DOI:10.1111/jace.18233.
- Q. Luo, L. Zhang, X. Chen, O. K. Tan and K. C. Leong, Mechanochemically synthesized m-BiVO4 nanoparticles for visible light photocatalysis, RSC Adv., 2016, 6(19), 15796–15802 RSC.
- M. Han, X. Chen, T. Sun, O. K. Tan and M. S. Tse, Synthesis of mono-dispersed m-BiVO4 octahedral nano-crystals with enhanced visible light photocatalytic properties, CrystEngComm, 2011, 13(22), 6674–6679 RSC.
- M. Hofmann, M. Rainer, S. Schulze, M. Hietschold and M. Mehring, Nonaqueous Synthesis of a Bismuth Vanadate Photocatalyst By Using Microwave Heating: Photooxidation versus Photosensitized Decomposition in Visible-Light-Driven Photocatalysis, ChemCatChem, 2015, 7(8), 1357–1365 CrossRef CAS.
- M. C. Neves and T. Trindade, Chemical bath deposition of BiVO4, Thin Solid Films, 2002, 406(1–2), 93–97 CrossRef CAS.
- I. Hincapié, A. Caballero-Guzman, D. Hiltbrunner and B. Nowack, Use of engineered nanomaterials in the construction industry with specific emphasis on paints and their flows in construction and demolition waste in Switzerland, Waste Manage., 2015, 43, 398–406 CrossRef PubMed.
- P. Van Broekhuizen, F. van Broekhuizen, R. Cornelissen and L. Reijnders, Use of nanomaterials in the European construction industry and some occupational health aspects thereof, J. Nanopart. Res., 2011, 13(2), 447–462 CrossRef.
- J. Teizer, M. Venugopal, W. Teizer and J. Felkl, Nanotechnology and its impact on construction: bridging the gap between researchers and industry professionals, J. Constr. Eng. Manag., 2012, 138(5), 594–604 CrossRef.
- J. Lee, S. Mahendra and P. J.-J. Alvarez, Nanomaterials in the construction industry: a review of their applications and environmental health and safety considerations, ACS Nano, 2010, 4(7), 3580–3590 CrossRef CAS PubMed.
- J.-P. Kaiser, S. Zuin and P. Wick, Is nanotechnology revolutionizing the paint and lacquer industry? A critical opinion, Sci. Total Environ., 2013, 442, 282–289 CrossRef CAS PubMed.
- M. J. Hanus and A. T. Harris, Nanotechnology innovations for the construction industry, Prog. Mater. Sci., 2013, 58(7), 1056–1102 CrossRef CAS.
- M. Saxena and L. K. Dhimole, Utilization and value addition of copper tailing as an extender for development of paints, J. Hazard. Mater., 2006, 129(1–3), 50–57 CrossRef CAS PubMed.
- J. Pospıšil and S. Nešpurek, Photostabilization of coatings. Mechanisms and performance, Prog. Polym. Sci., 2000, 25(9), 1261–1335 CrossRef.
- H. Ye, L. Zhu, W. Li, G. Jiang, H. Liu and H. Chen, Anchoring CeO2 nanoparticles on monodispersed SiO2 spheres to construct hydrophobic polymer coating with enhanced UV absorption ability, Chem. Eng. J., 2017, 321, 268–276 CrossRef CAS.
- Q. Yu, X. Xu, C. Wang, Y. Ma, D. Hui and Z. Zhou, Remarkably improvement in antibacterial activity by synergistic effect in n-Cu@T-ZnO nanocomposites, Composites, Part B, 2017, 110, 32–38 CrossRef CAS.
- A. Zhang, B. Mu, Z. Luo and A. Wang, Bright blue halloysite/CoAl2O4 hybrid pigments: preparation, characterization and application in water-based painting, Dyes Pigm., 2017, 139, 473–481 CrossRef CAS.
- E. Huajaikaew, T. Piroonpan, K. Booncharoen and W. Pasanphan, Comb-like poly(dodecyl methacrylate) modified SiO2 nanoparticles as nanohybrid coatings: Electron beam grafting and tuning superhydrophobic/water-repellent surface studies, Prog. Org. Coat., 2022, 163, 106658 CrossRef CAS.
- D. Truffier-Boutry, B. Fiorentino, V. Bartolomei, R. Soulas, O. Sicardy and A. Benayad,
et al., Characterization of photocatalytic paints: a relationship between the photocatalytic properties–release of nanoparticles and volatile organic compounds, Environ. Sci.: Nano, 2017, 4(10), 1998–2009 RSC.
- A. Gandolfo, L. Rouyer, H. Wortham and S. Gligorovski, The influence of wall temperature on NO2 removal and HONO levels released by indoor photocatalytic paints, Appl. Catal., B, 2017, 209, 429–436 CrossRef CAS.
- A. L. da Silva, D. N.-F. Muche, S. Dey, D. Hotza and R. H.-R. Castro, Photocatalytic Nb2O5-doped TiO2 nanoparticles for glazed ceramic tiles, Ceram. Int., 2016, 42(4), 5113–5122 CrossRef CAS.
- C. Toro, B. T. Jobson, L. Haselbach, S. Shen and S. H. Chung, Photoactive roadways: Determination of CO, NO and VOC uptake coefficients and photolabile side product yields on TiO2 treated asphalt and concrete, Atmos. Environ., 2016, 139, 37–45 CrossRef CAS.
- E. Boonen and A. Beeldens, Recent photocatalytic applications for air purification in Belgium, Coatings, 2014, 4(3), 553–573 CrossRef CAS.
- S. M. Amorim, J. Suave, L. Andrade, A. M. Mendes, H. J. Jose and R. F. P. M. Moreira, Towards an efficient and durable self-cleaning acrylic paint containing mesoporous TiO2 microspheres, Prog. Org. Coat., 2018, 118, 48–56 CrossRef CAS.
- R. E. Day, The role of titanium dioxide pigments in the degradation and stabilisation of polymers in the plastics industry, Polym. Degrad. Stab., 1990, 29(1), 73–92 CrossRef CAS.
- M. Baudys, J. Krýsa and A. Mills, Smart inks as photocatalytic activity indicators of self-cleaning paints, Catal. Today, 2017, 280, 8–13 CrossRef CAS.
- M. T. Islam, A. Dominguez, R. S. Turley, H. Kim, K. A. Sultana and M. A.-I. Shuvo,
et al., Development of photocatalytic paint based on TiO2 and photopolymer resin for the degradation of organic pollutants in water, Sci. Total Environ., 2020, 704, 135406 CrossRef CAS PubMed.
- A. Bonnefond, E. González, J. M. Asua, J. R. Leiza, E. Ieva and G. Brinati,
et al., Stable photocatalytic paints prepared from hybrid core-shell fluorinated/acrylic/TiO2 waterborne dispersions, Crystals, 2016, 6(10), 136 CrossRef.
- A. Bonnefond, E. González, J. M. Asua, J. R. Leiza, J. Kiwi and C. Pulgarin,
et al., New evidence for hybrid acrylic/TiO2 films inducing bacterial inactivation under low intensity simulated sunlight, Colloids Surf., B, 2015, 135, 1–7 CrossRef CAS PubMed.
- S. M. D. Amorim, J. C. Sapatieri, D. E. Moritz, M. D. Domenico, L. A. D. C. Laqua, C. D. Moura-Nickel and R. D. F. P. M. Moreira, Antifungal and photocatalytic activity of smart paint containing porous microspheres of TiO2, Mater. Res., 2020, 22(6) DOI:10.1590/1980-5373-MR-2019-0470.
- L. M. Farrier and S. L. Szaruga, Sample preparation and characterization of artificially aged aircraft coatings for microstructural analysis, Mater. Charact., 2005, 55(3), 179–189 CrossRef CAS.
- Y. Zhu, L. Zhang, L. Wang, R. Tan and L. Cao, Interface diffusion and reaction between TiO2 film photocatalyst and aluminium alloy substrate, Surf. Interface Anal., 2001, 32(1), 218–223 CrossRef CAS.
- N. Hassan, S. Lu, W. Xu, H. Ge and A. Sultana, Fabrication of stable superhydrophobic bismuth material on the aluminum substrate with high photocatalytic activity, J. Nanopart. Res., 2021, 23(4), 1–15 CrossRef.
- J.-G. Nam, E.-S. Lee, W.-C. Jung, Y.-J. Park, B.-H. Sohn and S.-C. Park,
et al., Photovoltaic enhancement of dye-sensitized solar cell prepared from [TiO2/ethyl cellulose/terpineol] paste employing TRITONTM X-based surfactant with carboxylic acid group in the oxyethylene chain end, Mater. Chem. Phys., 2009, 116(1), 46–51 CrossRef CAS.
- M. Meinert and G. Reiss, Electronic structure and optical band gap determination of NiFe2O4, J. Phys.: Condens. Matter, 2014, 26(11), 115503 CrossRef PubMed.
- Z. Shao, X. Meng, H. Lai, D. Zhang, X. Pu and C. Su,
et al., Coralline-like Ni2P decorated novel tetrapod-bundle Cd0.9Zn0.1SZB/WZ homojunctions for highly efficient visible-light photocatalytic hydrogen evolution, Chin. J. Catal., 2021, 42(3), 439–449 CrossRef CAS.
- A. Priac, P.-M. Badot and G. Crini, Treated wastewater phytotoxicity assessment using Lactuca sativa: focus on germination and root elongation test parameters, C. R. Biol., 2017, 340(3), 188–194 CrossRef PubMed.
- H. Zeghioud, N. Khellaf, A. Amrane, H. Djelal, M. Bouhelassa and A. A. Assadi,
et al., Combining photocatalytic process and biological treatment for Reactive Green 12 degradation: optimization, mineralization, and phytotoxicity with seed germination, Environ. Sci. Pollut. Res., 2021, 28(10), 12490–12499 CrossRef CAS PubMed.
- E. Ozel, G. Unluturk and S. Turan, Production of brown pigments for porcelain insulator applications, J. Eur. Ceram. Soc., 2006, 26(4–5), 735–740 CrossRef CAS.
- Q. I. Zwane, S. Moeno and L. N. Dlamini, A multiphase BiVO4 with the potential of being an environmental photocatalyst, Appl. Nanosci., 2019, 9(4), 539–555 CrossRef CAS.
- K. Anwar, F. K. Naqvi and S. Beg, Synthesis of tetragonally stabilized lanthanum doped bismuth vanadium oxide nanoparticles and its enhanced visible light induced photocatalytic performance, Phase Transitions, 2022, 95(1), 64–79 CrossRef CAS.
- M. M. Sajid, S. B. Khan, N. A. Shad and N. Amin, Synthesis of Zn3(VO4)2/BiVO4 heterojunction composite for the photocatalytic degradation of methylene blue organic dye and electrochemical detection of H2O2, RSC Adv., 2018, 8(62), 35403–35412 RSC.
- P. Praus, J. Lang, A. Martaus, L. Svoboda, V. Matějka and M. Kormunda,
et al., Composites of BiVO4 and g-C3N4: synthesis, properties and photocatalytic decomposition of Azo Dye AO7 and nitrous oxide, J. Inorg. Organomet. Polym. Mater., 2019, 29(4), 1219–1234 CrossRef CAS.
- U. Alam, A. Khan, D. Ali, D. Bahnemann and M. Muneer, Comparative photocatalytic activity of sol-gel derived rare earth metal (La, Nd, Sm and Dy)-doped ZnO photocatalysts for degradation of dyes, RSC Adv., 2018, 8(31), 17582–17594 RSC.
- J. H. Shah, M. Fiaz, M. Athar, J. Ali, M. Rubab, R. Mehmood and R. Djellabi, Facile synthesis of N/B-double-doped Mn2O3 and WO3 nanoparticles for dye degradation under visible light, Environ. Technol., 2019, 41(18), 2372–2381 CrossRef PubMed.
- E. Prabakaran and K. Pillay, Synthesis of N-doped ZnO nanoparticles with cabbage morphology as a catalyst for the efficient photocatalytic degradation of methylene blue under UV and visible light, RSC Adv., 2019, 9(13), 7509–7535 RSC.
- B. Vellaichamy and P. Periakaruppan, Ag nanoshell catalyzed dedying of industrial effluents, RSC Adv., 2016, 6(38), 31653–31660 RSC.
- C. Yang, W. Dong, G. Cui, Y. Zhao, X. Shi and X. Xia,
et al., Highly efficient photocatalytic degradation of methylene blue by P2ABSA-modified TiO2 nanocomposite due to the photosensitization synergetic effect of TiO2 and P2ABSA, RSC Adv., 2017, 7(38), 23699–23708 RSC.
- A. Singh, A. Bhati, P. Khare, K. M. Tripathi and S. K. Sonkar, Soluble graphene nanosheets for the sunlight-induced photodegradation of the mixture of dyes and its environmental assessment, Sci. Rep., 2019, 9(1), 1–12 CrossRef PubMed.
- E. R. Emino and P. R. Warman, Biological assay for compost quality, Compost Sci. Util., 2004, 12(4), 342–348 CrossRef.
- S. Tu, Y. Guo, Y. Zhang, C. Hu, T. Zhang and T. Ma,
et al., Piezocatalysis and Piezo-Photocatalysis: Catalysts Classification and Modification Strategy, Reaction Mechanism, and Practical Application, Adv. Funct. Mater., 2020, 30(48), 2005158 CrossRef CAS.
- A. Kumar and G. Pandey, A review on the factors affecting the photocatalytic degradation of hazardous materials, Mater. Sci. Eng., 2017, 1(3), 1–10 Search PubMed.
- Y. Zhang, Z. Yang, R. Li, H. Geng and C. Dong, Investigation of fine chalk dust particles’ chemical compositions and toxicities on alveolar macrophages in vitro, Chemosphere, 2015, 120, 500–506 CrossRef CAS PubMed.
- H. Lei, H. Zhang, Y. Zou, X. Dong, Y. Jia and F. Wang, Synergetic photocatalysis/piezocatalysis of bismuth oxybromide for degradation of organic pollutants, J. Alloys Compd., 2019, 809, 151840 CrossRef CAS.
- G. Tezcanli-Güyer and N. H. Ince, Individual and combined effects of ultrasound, ozone and UV irradiation: a case study with textile dyes, Ultrasonics, 2004, 42(1–9), 603–609 CrossRef PubMed.
|
This journal is © The Royal Society of Chemistry 2022 |
Click here to see how this site uses Cookies. View our privacy policy here.