DOI:
10.1039/D2MA00417H
(Paper)
Mater. Adv., 2022,
3, 7053-7060
Facile control of the surface property and hydrolytic degradation of poly(L-lactide) materials by coating poly(L-lactide)-based triblock copolymers with hydrophilic or hydrophobic block†
Received
13th April 2022
, Accepted 19th July 2022
First published on 21st July 2022
Abstract
Diversifying the surface property and hydrolytic degradation rate is crucial to widen the applications of poly(L-lactide) (PLLA). In the present study, PLLA films were coated with PLLA-poly(ethylene glycol) (PEG)-PLLA (PLLA-b-PEG-b-PLLA) and PLLA-polybutadiene (PB)-PLLA (PLLA-b-PB-b-PLLA) as typical examples of block copolymers to prepare biodegradable materials with controlled surface property and hydrolytic degradation rate. These block copolymers had outer blocks whose chemical structure is identical to that of the base material and a center block of hydrophilic PEG or hydrophobic PB. The contact angle of PLLA films with water (85°) was decreased and increased by coating with PLLA-b-PEG-b-PLLA (71°) and PLLA-b-PB-b-PLLA (115°), respectively, revealing the facile and effective hydrophilization or hydrophobization by coating. Due to increased hydroxy and carboxyl groups on the film surface, the contact angle values decreased monotonously with increasing alkaline hydrolytic degradation time, irrespective of the presence of the coated polymer or the type of polymer coating. The hydrolytic degradation was decelerated by coated PLLA-b-PEG-b-PLLA and PLLA-b-PB-b-PLLA and the decelerating effect was intense for hydrophobic PLLA-b-PB-b-PLLA than for hydrophilic PLLA-b-PEG-b-PLLA. After hydrolytic degradation, the contact angle difference between the PLLA-b-PEG-b-PLLA-coated and non-coated films remained unchanged, whereas that between the PLLA-b-PB-b-PLLA-coated and non-coated films became larger. This indicates a higher hydrolytic degradation-resistance of the coated PLLA-PB-PLLA than that of the coated PLLA-PEG-PLLA and thereby the hydrophobization effects of the coated PLLA-PB-PLLA lasted for a longer time. Coating triblock copolymers composed of outer blocks whose molecular structure is same as that of base polymeric materials and a center block with high or low hydrophilicity is a facile method to prepare fully biodegradable materials with controlled surface property and hydrolytic degradation rate.
1. Introduction
Poly(L-lactide) (PLLA) is a biobased biodegradable polymer synthesized from various renewable plant-based resources and is utilized in commodity, biomedical, pharmaceutical, and environmental applications.1–11 In biomedical, pharmaceutical, and environmental applications, manipulation of surface properties and hydrolytic degradation behavior and rate is crucial. For example, the surface hydrophilicity or hydrophobicity determines the cell or protein adsorption.12–14 The maximal cell or protein adhesion was observed on the polymer surfaces with a water contact angle around 70°.12–14 There are a wide variety of methods to control surface properties and hydrolytic degradation behavior and rate.11 For these purposes, copolymerization, polymer blending and composite formation are frequently utilized.10,11 However, in addition to surface properties and hydrolytic degradation behavior and rate, bulk physical properties such as mechanical and thermal properties are altered by these methods. Surface treatments such as polymer coating15–17 and alkali-treatment18–24 are promising and versatile methods to control surface properties and hydrolytic degradation behavior and rate without altering the bulk physical properties. Another advantage of surface treatments is their applicability on site after processing or molding of polymer materials, and thereby the surface property is controllable on site.
It was found to be effective to coat the polymers with PLA blocks or chains on PLA materials to control surface properties, immobilize the biologically active substances, or confer reactive sites. For example, PLLA-b-PEG (α-acetal terminated) was synthesized, dissolved in toluene, and coated on silanized glass coated with PLLA.15 The water contact angles of PLLA-b-PEG coated PLLA decreased with increasing PEG length from 80° to 48°. Although PLLA-b-PEG-coating was effective in enhancing the surface hydrophilicity of PLLA, a toxic organic solvent was used for coating and the degradation behavior and rate and the retention characteristic of surface property were not investigated in the literature.15–17 Hydrophilic poly(vinyl alcohol) (PVA) coating in aqueous solution was effectively utilized to enhance the surface hydrophilicity of PLLA films using non-toxic water as a solvent.16 However, the decelerating effects on enzymatic degradation were small and insignificant for amorphous and crystallized PLLA films, respectively.16 In contrast, the decelerating effect on enzymatic degradation of poly(ε-caprolactone) (PCL) films was significant and can be manipulated by the concentration of PVA solution for coating.16 Polypyrrole coating increased the hydrophilicity of poly(L-lactic acid-co-ε-caprolactone)/silk fibroin nanofibers.17
Alkaline treatment with NaOH solution was applied to PLLA19,20,22–24 and poly(DL-lactide-co-glycolide) (85/15)18 films for controlling surface properties,19–22,24 cell adhesion,18 and enzymatic hydrolytic degradation.20 Alkali-treatment increased the surface hydrophilicity of PLLA films and accelerated their enzymatic hydrolytic degradation.20 However, too long treatment increased the surface roughness of PLLA.24 Such surface roughness is thought to increase the alkaline and enzymatic degradation rates, due to increased surface area per weight. Post treatment of alkali-treated films with citric acid further increased the hydrophilicity and roughness of PLLA films.22 Alkaline treatment with KOH solution increased the hydrophilicity of PLLA films with increasing concentration of KOH solution, whereas the live cell number increased when treated with 0.1 M KOH solution but decreased when treated with 0.4 M KOH solution.21 The decreased cell number for 0.4 M KOH solution can be ascribed to low local pH caused by hydrolysis-forming high density of carboxyl groups, resulting in a large deviation of surface local pH from the appropriate pH = 7. Aminolysis using the second generation polypropylene imine dendrimer (PPI-G2) greatly increased hydrophilicity and reduced the acidifying rate by hydrolytic degradation of PLLA films and nanofibers, resulting in promoted cell adhesion and viability.23 Alkaline treatment is effective only for hydrophilization of PLLA materials but not for hydrophobization.
One of the purposes of the present study was to show the effectiveness of coating block copolymers having outer blocks whose chemical structure is identical to that of the base biodegradable material and a center block of hydrophilic or hydrophobic chain to control the hydrophilicity or hydrophobicity of biodegradable polymeric materials. Another purpose was to investigate the effects of coating on hydrolytic degradation and the retention characteristic of surface hydrophilicity or hydrophobicity upon hydrolytic degradation. For these purposes, triblock copolymers, PLLA-b-poly(ethylene glycol) (PEG)-b-PLLA and PLLA-b-polybutadiene (PB)-b-PLLA (Fig. 1), were synthesized as typical examples with the blocks whose molecular structure is same as that of base materials and a hydrophilic or hydrophobic center block. Incorporated PLLA blocks in the triblock copolymers, whose chemical structure is identical to that of the base PLLA material, were designed to increase the affinity to base PLLA materials and induce the facile adsorption of triblock copolymers on to the surface of base PLLA materials. The PLLA films were coated with PLLA-b-PEG-b-PLLA and PLLA-b-PB-b-PLLA to prepare biodegradable materials with enhanced surface hydrophilicity or hydrophobicity (Fig. 2). The surface property, hydrolytic degradation behavior, and surface morphology change upon hydrolytic degradation were investigated by water contact angle measurements, gravimetry, and scanning electron microscopic observation. The surface coating of triblock copolymers was found to be effective in affording surface hydrophilicity or hydrophobicity and decelerating the hydrolytic degradation rate of biodegradable materials.
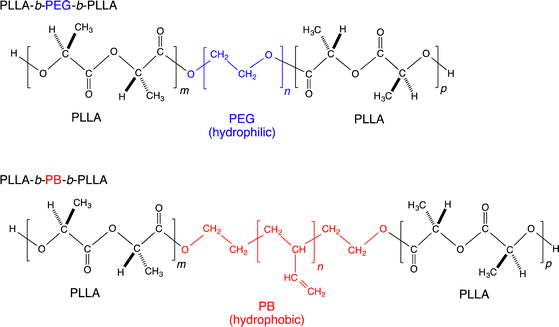 |
| Fig. 1 Molecular structures of PLLA-b-PEG-b-PLLA and PLLA-b-PB-b-PLLA used in this study. For simplicity, only 1,2-isomer units are shown in the structure of PLLA-b-PB-b-PLLA, although actually PB should contain 1,4-isomer units in addition to the main 1,2-isomer units. | |
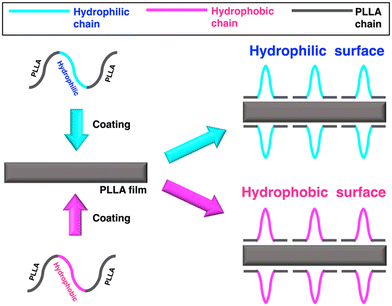 |
| Fig. 2 Schematic illustration of the strategy to control surface hydrophilicity or hydrophobicity by coating PLLA-b-PEG-b-PLLA and PLLA-b-PB-b-PLLA diblock copolymers having a hydrophilic or hydrophobic center block. | |
2. Experimental section
2.1. Materials
L-Lactide (PURASORB L®, Purac Biochem BV, Gorinchem, The Netherlands) was purified by recrystallization using ethyl acetate (JIS special grade, Nacalai Tesque, Inc.). Tin(II) 2-ethylhexanoate (Practical grade, Nacalai Tesque, Inc., Kyoto, Japan) was purified by distillation under reduced pressure. PEG (2.0 g, #6000, Nacalai Tesque), toluene (3 mL, Nacalai special grade, H2O < 30 ppm, Nacalai Tesque, Inc.), PB diol (1.0 g, G-3000, Nippon Soda, Co. Ltd., Tokyo, Japan), ethyl acetate, chloroform, acetone, methanol, dichloromethane (JIS special grade, Nacalai Tesque, Inc.), tetrahydrofuran [THF, dehydrated stabilizer free (Super Plus), Kanto Chemical Co. Inc., Tokyo, Japan], and distilled water (Special grade for HPLC, Nacalai Tesque, Inc.) were used as received.
2.2. Synthesis and purification of PLLA-PEG-PLLA and PLLA-PB-PLLA polymers
PLLA was synthesized by ring-opening polymerization of L-lactide (5.0 g) using tin(II) 2-ethylhexanoate (0.03 wt%) as the initiator at 140 °C for 10 h. PLLA-b-PEG-b-PLLA and PLLA-b-PB-b-PLLA were synthesized by ring-opening polymerization of L-lactide (0.6 and 1.0 g, respectively) in toluene using tin(II) 2-ethylhexanoate (0.3 wt%) as the initiator and PEG (2.0 g) and PB diol (1.0 g) as coinitiators, respectively, at 120 °C for 36 h. The synthesized PLLA-b-PEG-b-PLLA and PLLA-b-PB-b-PLLA were dissolved by adding chloroform after removal of toluene as much as possible and precipitated in excess amounts of diethyl ether and methanol, respectively, followed by drying under reduced pressure for at least 6 days. We have selected PEG and PB as a center block because PEG is a hydrophilic polymer which is biocompatible and approved for clinical use by the Food and Drug Administration (FDA),15 whereas PB diol is a rare commercially available hydrophobic polymer with hydroxy groups at both chain terminals. Because the molecular weight of PB diol was fixed (6.67 × 103 g mol−1), PEG was selected to have the molecular weight (9.06 × 103 g mol−1) similar to that of PB diol. Although PLLA-PEG and PLLA-PB diblock copolymers can be used for the similar purposes of this study, we used PLLA-PEG-PLLA and PLLA-PB-PLLA triblock copolymers expecting stronger adsorption of coating polymers to the material surface.
2.3. Specimen preparation
A PLLA film (thickness: 200 μm) was prepared by solvent-evaporation of a polymer solution (10 mg mL−1) using dichloromethane as the solvent, followed by drying on a glass Petri dish under reduced pressure for at least 6 days. PLLA-PEG-PLLA and PLLA-PB-PLLA coating for the PLLA films (10 × 15 × 0.2 mm3) was performed in 10 mg mL−1 (20 mL) PLLA-PEG-PLLA suspension (solvent: distilled water) and PLLA-PB-PLLA suspension [solvent: THF/distilled water (v/v) = 17/3] at 25 °C for 24 h. As blank non-coated specimens, PLLA films treated with only solvent (distilled water or the THF/distilled water mixture) in the absence of the triblock polymer were prepared. It should be noted that there are two types of blank non-coated specimens using distilled water and the THF/distilled water mixture for PLLA-PEG-PLLA and PLLA-PB-PLLA coating, respectively. The coated and non-coated films were rinsed thoroughly with distilled water for 3 min to remove non-coated free polymer chains, leaving the polymer chains coated on the film irreversibly. The rinsed films were dried under reduced pressure for at least 6 days.
2.4. Alkaline hydrolysis of PLLA films
The alkaline hydrolytic degradation of each of the PLLA films (10 × 15 × 0.2 mm3) was performed in a vial filled with 10 mL of 0.1 M NaOH solution (pH 13.0, Nacalai Tesque Inc.) at 37 °C for up to 24 h. The hydrolyzed films were rinsed thoroughly with distilled water for 3 min, followed by drying under reduced pressure for at least 6 days. After complete drying, the degradation of films was estimated by changes in weight loss, contact angle, and surface morphology. The weight loss (μg) was normalized by dividing by the film surface area (mm2) because alkaline hydrolytic degradation is reported to proceed via a surface erosion mechanism.10,11
2.5. Measurements and observation
The weight- and number-average molecular weights (Mw and Mn, respectively) of PLLA, coinitiator polymers, and triblock polymers were estimated at 40 °C in chloroform by using a Tosoh (Tokyo, Japan) gel permeation chromatography (GPC) system with a refractive index detector (RI-8020, Tosoh) and two TSK gel columns (GMHXL, Tosoh) using polystyrene standards (Tosoh). The 400 MHz 1H NMR spectra of the polymers and prepolymers were obtained in deuterated chloroform (Sigma-Aldrich Japan, K. K., 99.8%, containing 0.03 wt% tetramethylsilane) by using a Bruker BioSpin (Kanagawa, Japan) AVANCE III 400.
The cold crystallization and melting temperatures (Tcc and Tm, respectively) and enthalpies of cold crystallization and melting (ΔHcc and ΔHm, respectively) were obtained using a Shimadzu (Kyoto, Japan) DSC-60 differential scanning calorimeter under a nitrogen gas flow (50 mL min−1).25 The DSC measurements of the PLLA films (ca. 3 mg) were performed by heating at a rate of 10 °C min−1 from 0 to 200 °C.25 The contact angle formed between water drops and the surface of the films was measured by a Smart contact Mobile Entry 4 (Excimer Inc., Kanagawa, Japan). For contact angle measurements, the drops of water (5 μL) were mounted on 3 different positions of the film surface with a microsyringe. The contact angle values are mean values of three measurements on different parts of the films. The surface morphology of the PLLA films was studied using a Hitachi (Tokyo, Japan) SU3500 scanning electron microscope with an accelerating voltage of 10 kV without coating.
3. Results and discussion
3.1. Polymer synthesis
The 1H NMR spectra of PEG, PB diol, and synthesized PLLA-PEG-PLLA and PLLA-PB-PLLA polymers are shown in Fig. 3. The 1H NMR peaks of PEG and PB shown in Fig. 3 are ascribed based on the literature.26–28 The relatively large peak area of olefinic methylene (1,2-isomer unit) at 4.9 ppm compared to that at 2.0 ppm (1,4-cis- and 1,4-trans isomer units) in PB diol indicates that 1,2-isomer units rather than 1,4-isomer units are the main monomer units. In addition to the PEG and PB chain-derived peaks, the methine and methyl peaks of newly formed PLLA blocks appeared at 5.2 and 1.6 ppm, respectively, for both PLLA-PEG-PLLA and PLLA-PB-PLLA. Fig. S1 (ESI†) shows the GPC charts of PB diol, PLLA-PB-PLLA, PEG, and PLLA-PEG-PLLA. Comparing the GPC charts of PB diol and PLLA-PB-PLLA and those of PEG and PLLA-PEG-PLLA, molecular weight distribution curves of PB diol and PEG shifted to a higher molecular weight as a whole after L-lactide polymerization. The comparison between the molecular weights of PB diol and PLLA-PB-PLLA and those of PEG and PLLA-PEG-PLLA in Table 1 and the 1H NMR result clearly indicate the successful synthesis of PLLA-PEG-PLLA and PLLA-PB-PLLA.
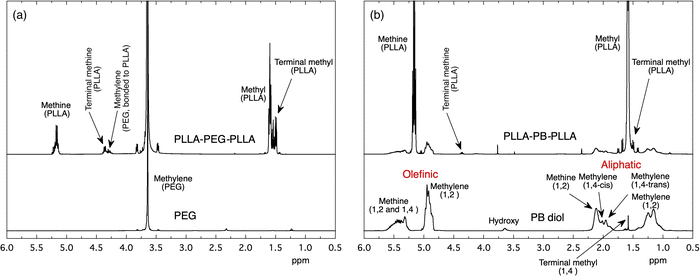 |
| Fig. 3
1H NMR spectra of PEG and PLLA-PEG-PLLA (a) and PB diol and PLLA-PB-PLLA (b). | |
Table 1 Molecular characteristics of polymers used in the present study
Code |
M
n
(g mol−1) |
M
w
/Mna |
Number-average molecular weight estimated by GPC measurements.
Weight-average molecular weight estimated by GPC measurements.
|
PLLA |
4.95 × 105 |
1.69 |
PEG |
9.06 × 103 |
1.13 |
PB diol |
6.67 × 103 |
1.36 |
PLLA-PEG-PLLA |
1.40 × 104 |
1.10 |
PLLA-PB-PLLA |
5.06 × 104 |
1.40 |
3.2. Surface coating
Fig. 4 and 5 show the average contact angles of PLLA films without coating and with PLLA-PEG-PLLA or PLLA-PB-PLLA coating. The average contact angle of PLLA films decreased from 85.1° to 71.2° and increased from 85.2° to 115.0° by the coating of PLLA-PEG-PLLA and PLLA-PB-PLLA, respectively (see the photos for 0 h). These results indicate that coating PLLA-PEG-PLLA with hydrophilic PEG blocks and PLLA-PB-PLLA with hydrophobic PB blocks is an effective method to increase the surface hydrophilicity and hydrophobicity of PLLA films, respectively.
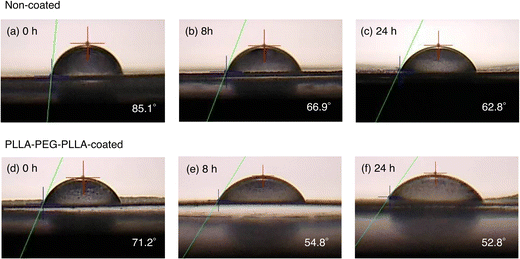 |
| Fig. 4 Water contact angles of non-coated PLLA films (a–c) and PLLA films coated with PLLA-PEG-PLLA (d–f) hydrolytically degraded for different periods of time. The shown contact angle values are the averaged ones and not specific ones for the respective photos. | |
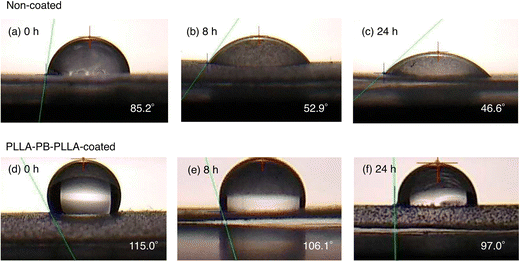 |
| Fig. 5 Water contact angles of non-coated PLLA films (a–c) and PLLA films coated with PLLA-PB-PLLA (d–f) hydrolytically degraded for different periods of time. The shown contact angle values are the averaged ones and not specific ones for the respective photos. | |
The DSC thermograms of PLLA films before and after coating are shown in Fig. S2 (ESI†) and the thermal properties of the films obtained from Fig. S2 (ESI†) are given in Table S1 (ESI†). Although a cold crystallization peak appeared for the PLLA films treated with distilled water, i.e., non-coated and PLLA-PEG-PLLA-coated PLLA films, the changes in Tm and ΔH(tot) (=ΔHcc + ΔHm) as an indicator of crystallinity were insignificant or very small, suggesting very small effects of removal of amorphous polymers and crystallization during coating.
3.3. Hydrolytic degradation
3.3.1. Weight loss.
The hydrolytic degradability of PLLA films was monitored by the weight loss caused by alkaline hydrolytic degradation (Fig. 6). The weight loss of all types of PLLA films increased linearly without an induction period. Such linear increase was observed when PLLA films were hydrolytically degraded in an alkaline solution due to a surface erosion mechanism.29 The weight loss rates of non-coated and PLLA-PEG-PLLA-coated PLLA films were 0.74 and 0.64 μg mm−2 h−1, respectively, whereas the weight loss rates of non-coated and PLLA-PB-PLLA-coated PLLA films were 1.69 and 1.37 μg mm−2 h−1, respectively. Both coatings are effective to significantly decelerate the hydrolytic degradation of PLLA films, irrespective of the hydrophilicity or hydrophobicity of the center block of coated polymers. Such a decelerating effect agrees with that of enzymatic degradation of PVA-coated PLLA films.16 The decelerating effect of coated polymers was slightly smaller for hydrophilic PLLA-PEG-PLLA (12% lower than for the non-coated PLLA film) than for hydrophobic PLLA-PB-PLLA (19% lower than for the non-coated PLLA film). This finding suggests that the amount of coated polymer is lower for PLLA-PEG-PLLA than for PLLA-PB-PLLA or the hydrophilic PEG block in PLLA-PEG-PLLA facilitated the catalytic hydroxyl ions in the NaOH aqueous solution to approach the PLLA film and thereby slightly reduced the decelerating effect of coated PLLA-PEG-PLLA. The weight loss rate of the non-coated films treated with the THF/distilled water mixture (1.69 μg mm−2 h−1) was higher than that of the non-coated film treated with distilled water (0.74 μg mm−2 h−1). This finding should be due to the porous structure of the non-coated film treated with the THF/distilled water mixture, which is shown Section 3.3.3. The porous structure should have increased the surface area per unit mass, resulting in the higher weight loss rate of the non-coated PLLA film treated with the THF/distilled water mixture. The remarkable alkaline hydrolytic degradation-accelerating effects of the porous structure are reported elsewhere for PCL films, wherein not only the pore fraction but also pore size is crucial to determine the degradation rate.30
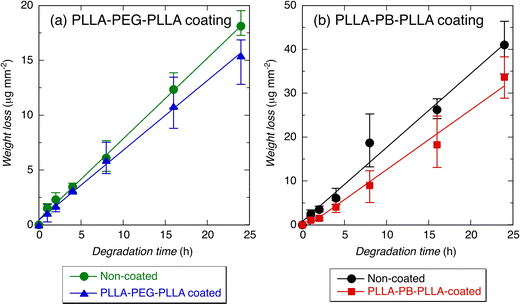 |
| Fig. 6 Weight loss per unit surface area of non-coated (a and b), PLLA-PEG-PLLA-coated (a), and PLLA-PB-PLLA-coated (b) films hydrolytically degraded for different periods of time. | |
3.3.2. Contact angle.
The numbers of hydrophilic hydroxy and carboxyl groups on the PLLA film surface are expected to increase by the hydrolysis of ester groups, resulting in the increased surface hydrophilicity and thereby decreased contact angle. To access the retention characteristic of surface hydrophilicity or hydrophobicity afforded by coating, the contact angle of PLLA films was measured during alkaline hydrolytic degradation [Fig. 7(a and b)]. The contact angles of the non-coated and PLLA-PEG-PLLA-coated PLLA films were decreased by 24 h degradation from 85.1° to 62.7° (22.4° decrease) and from 71.2° to 52.8° (18.4° decrease), respectively. The contact angles of the non-coated and PLLA-PB-PLLA-coated PLLA films were decreased by 24 h degradation from 85.2° to 46.6° (38.6° decrease) and from 115.0° to 97.0° (18.0° decrease), respectively. The contact angles of all types of PLLA films decreased gradually with degradation time, indicating the increased surface hydrophilicity upon hydrolytic degradation, due to the increased numbers of hydrophilic hydroxyl and carboxyl groups. It is interesting to note that contact angle difference between the non-coated and PLLA-PEG-PLLA-coated films was decreased by 24 h degradation from 13.9° to 9.9°, whereas surprisingly that between the non-coated and PLLA-PB-PLLA-coated films was increased by 1 h degradation from 29.8° to 50.5° and remained unchanged up to 24 h [Fig. 7(c)]. These results strongly suggest that the coated PLLA-PB-PLLA is more hydrolytic degradation-resistant than the coated PLLA-PEG-PLLA and thereby the remaining hydrophobic PLLA-PB-PLLA maintained the hydrophobicity of the PLLA film for a longer period.
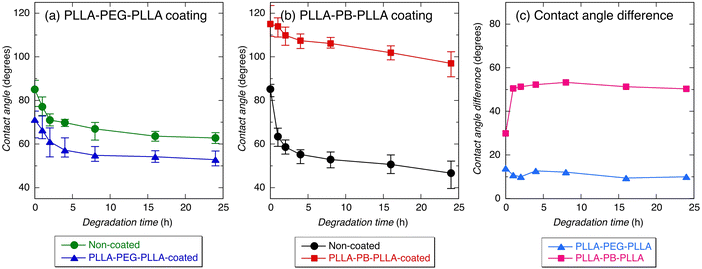 |
| Fig. 7 Surface contact angle of non-coated (a and b), PLLA-PEG-PLLA-coated (a), and PLLA-PB-PLLA-coated (b) films hydrolytically degraded for different periods of time and the contact angle difference between non-coated and coated films (c). | |
3.3.3. Scanning electron microscopy.
Fig. 8 and 9 show the scanning electron photomicrographs of the non-coated, PLLA-PEG-PLLA-coated, and PLLA-PB-PLLA-coated PLLA films before hydrolytic degradation and after hydrolytic degradation for 24 h. The surface of both non-coated and PLLA-PEG-PLLA-coated films treated with distilled water was smooth before and after hydrolytic degradation. The retained smooth surface is attributable to the low weight loss of PLLA films, wherein erosion occurred only on the surface of the films and, therefore, the internal structure could not be observed. Some circular morphologies observed after hydrolytic degradation are attributable to the hydrolysis-revealing spherulitic structures. In contrast, before hydrolytic degradation, spherulites and porous amorphous regions between the spherulites were observed on the surface of the non-coated and PLLA-PB-PLLA-coated films treated with the THF/distilled water mixture. Such a porous structure or surface roughness should have been formed by the plasticizing or swelling effect of THF and increased the surface area per unit weight, resulting in accelerated hydrolytic degradation of PLLA films treated with the THF/distilled water mixture compared to the hydrolytic degradation of PLLA films treated with distilled water (Fig. 6). After hydrolytic degradation, some spherulitic structures and cracks were formed on the non-coated film treated with the THF/distilled water mixture, whereas some spherulitic microspheres were observed on the porous surface of the PLLA-PB-PLLA coated film. These structures should have been formed by the selective removal of amorphous regions on the films by hydrolytic degradation. The temperature of 37 °C was too low and the degradation of 24 h was too short for the formation of spherulites by crystallization. So far, the reason for crack formation for the blank non-coated film is unknown.
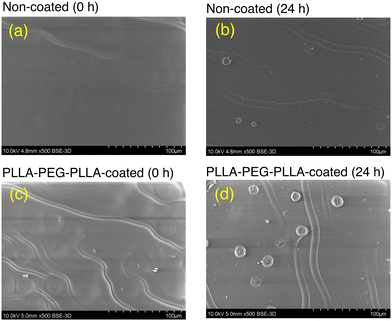 |
| Fig. 8 Scanning electron photomicrographs of non-coated (a and b) and PLLA-PEG-PLLA-coated (c and d) films before hydrolytic degradation (a and c) and after hydrolytic degradation for 24 h (b and d). | |
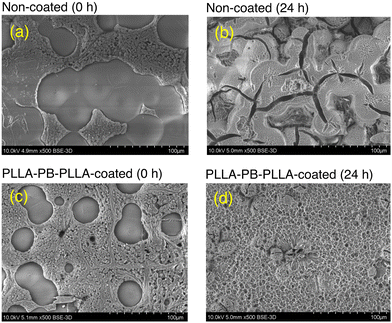 |
| Fig. 9 Scanning electron photomicrographs of non-coated (a and b) and PLLA-PB-PLLA-coated (c and d) films before hydrolytic degradation (a and c) and after hydrolytic degradation for 24 h (b and d). | |
Based on the results obtained in the present study, degradation mechanisms of PLLA-PEG-PLLA- and PLLA-PB-PLLA-coated films are proposed as follows. In the case of the PLLA-PEG-PLLA-coated film, some water-soluble PEG chains will be released into the hydrolysis medium by the hydrolytic scission of two ester groups adjoining the PEG block and their blocking effect on hydroxide ions to approach the PLLA material surface should become low. However, in the case of the PLLA-PB-PLLA-coated film, the water-insoluble PB chains will remain on the surface of PLLA materials even after the hydrolytic scission of two ester groups adjoining the PB block and their blocking effect on hydroxide ions to approach the PLLA material surface should remain.
In the present study, we showed that coating of PLLA-PEG-PLLA and PLLA-PB-PLLA polymers with a hydrophilic or hydrophobic center block as typical examples of block polymers was effective to hydrophilize and hydrophobize the biodegradable PLLA film and control the hydrolytic degradation rate of PLLA films. The type of hydrophilic or hydrophobic center block and the molecular weight of each block should be selected carefully depending on the property requirements for materials.
4. Conclusions
The synthesized triblock PLLA-PEG-PLLA and PLLA-PB-PLLA polymers with a hydrophilic or hydrophobic center block effectively hydrophilize and hydrophobize the biodegradable PLLA film. Both polymer coatings decelerated the hydrolytic degradation of the PLLA film. The distilled water used for PLLA-PEG-PLLA coating did not affect the surface morphology of PLLA films, whereas probably due to the plasticizing or swelling effect of THF used for PLLA-PB-PLLA coating induced the formation of spherulites and porous amorphous regions between spherulites. The porous structure should have increased the surface area per unit weight, resulting in accelerated hydrolytic degradation of PLLA films treated with the THF/distilled water mixture. The numbers of hydroxy and carboxyl groups per unit surface increased with alkaline degradation time, irrespective of the presence of coated polymer or the type of polymer coating. The coated PLLA-PB-PLLA had higher hydrolytic degradation-resistance than the coated PLLA-PEG-PLLA and thereby maintained the hydrophobicity of the PLLA film for a longer period. Coating block copolymers composed of outer blocks whose molecular structure is identical with that of the base biodegradable polymeric materials and a center block of hydrophilic or hydrophobic chain is a versatile method to produce biodegradable materials with manipulated surface property and hydrolytic degradation rate.
Author contributions
Hideto Tsuji: conceptualization, methodology, supervision, validation, formal analysis, data curation, visualization, writing – original draft preparation, reviewing and editing, project administration, funding acquisition. Narumi Tsukamoto: resources, investigation, formal analysis, writing – reviewing and editing. Yuki Arakawa: investigation, supervision (resources and investigation), writing – reviewing and editing.
Conflicts of interest
There are no conflicts to declare.
Acknowledgements
This research was supported by the research grant from The Hibi Science Foundation. The authors would like to thank Mr Masaki Ishikawa from Nippon Soda, Co. Ltd. (Tokyo, Japan) for providing them PB diol.
References
-
M. Vert, J. Feijen, A.-C. Albertsson, G. Scott and E. Chiellini, Biodegradable Polymers and Plastics, Royal Society of Chemistry: Cambridge, 1992 Search PubMed.
-
D. P. Mobley, Plastics from Microbes, Hanser Publishers: New York, 1994 Search PubMed.
- M. Vert, G. Schwarch and J. Coudane, J. Macromol. Sci., Pure Appl. Chem., 1995, A32, 787–796 CrossRef CAS.
-
A. J. Domb, J. Kost and D. M. Wieseman, Handbook of Biodegradable Polymers (Drug Targeting and Delivery, vol. 7), Harwood Academic Publishers; Amsterdam, Netherlands, 1997 Search PubMed.
-
D. L. Kaplan, Biopolymers from Renewable Resources, Springer; Berlin, Germany, 1998 Search PubMed.
- D. A. Garlotta, J. Polym. Environ., 2001, 9, 63–84 CrossRef CAS.
- A. Södergård and M. Stolt, Prog. Polym. Sci., 2002, 27, 1123–1163 CrossRef.
-
A.-C. Albertsson, Degradable Aliphatic Polyesters (Advances in Polymer Science, Vol.157), Springer; Berlin, Germany, 2002 Search PubMed.
-
Y. Doi and A. Steinbüchel, Polyesters I, II, III (Biopolymers, Vol. 3a, 3b, 4), Wiley-VCH; Weinheim, Germany, 2002 Search PubMed.
-
R. Auras, L.-T. Lim, S. E. M. Selke and H. Tsuji, Poly(lactic acid): Synthesis, structures, properties, processing, and applications (Wiley Series on Polymer Engineering and Technology), John Wiley & Sons, Inc.; NJ, 2010 Search PubMed.
-
H. Tsuji, in Poly(Lactic Acid). Bio-Based Plastics: Materials and Applications, ed. S. Kabasi, Wiley & Sons, Ltd, Chichester, UK, 2014, Chapter 8, pp. 171–239 Search PubMed.
- Y. Tamada and Y. Ikada, Polymer, 1993, 34, 2208–2212 CrossRef CAS.
- Y. Tamada and Y. Ikada, J. Colloid Interface Sci., 1993, 155, 334–339 CrossRef CAS.
- D. P. Dowling, I. S. Miller, M. Ardhaoui and W. M. Gallagher, J. Biomater. Appl., 2011, 26, 327–347 CrossRef CAS PubMed.
- H. Otsuka, Y. Nagasaki and K. Kataoka, Biomacromolecules, 2000, 1, 39–48 CrossRef CAS PubMed.
- H. Tsuji and T. Ishida, Macromol. Biosci., 2003, 3, 51–58 CrossRef CAS.
- B. Sun, T. Wu, J. Wang, D. Li, J. Wang, Q. Gao, M. A. Bhutto, H. El-Hamshary, S. S. Al-Deyab and X. Mo, J. Mater. Chem. B, 2016, 4, 6670–6679 RSC.
- Y. S. Nam, J. J. Yoon, J. G. Lee and T. G. Park, J. Biomater. Sci., Polym. Ed., 1999, 10, 1145–1158 CrossRef CAS PubMed.
- H. Tsuji and T. Ishida, J. Appl. Polym. Sci., 2002, 87, 1628–1633 CrossRef.
- H. Tsuji, T. Ishida and N. Fukuda, Polym. Int., 2003, 52, 843–852 CrossRef CAS.
- J. Harrison, S. Pattanawong, J. S. Forsythe, K. A. Gross, D. R. Nisbet, H. Beh, T. F. Scott, A. O. Trounson and R. Mollard, Biomaterials, 2004, 25, 4963–4970 CrossRef CAS PubMed.
- C. Guo, M. Xiang and Y. Dong, Mater. Lett., 2015, 140, 144–147 CrossRef CAS.
- A. Ganjalinia and S. Akbari, Appl. Surf. Sci., 2017, 394, 446–456 CrossRef CAS.
- I. R. Durán, S. Vanslambrouck, P. Chevallier, C. A. Hoesli and G. Laroche, Colloids Surf., B, 2020, 189, 110847 CrossRef PubMed.
- H. Tsuji, K. Osanai and Y. Arakawa, Cryst. Growth Des., 2018, 18, 6009–6019 CrossRef CAS.
- H. Asadi, K. Rostamizadeh and M. D. Salari, J. Microencapsulation, 2011, 28, 406–416 CrossRef CAS PubMed.
- F. Ziaee, M. Ronagh-Baghbani and M. R. Jozaghkar, Polym. Bull., 2020, 77, 2345–2365 CrossRef CAS.
- F. Ziaee, H. S. Mobarakeh, M. Nekoomanesh and H. ArabiIranian, Iran. Polym. J., 2008, 17, 379–388 CAS.
- H. Tsuji and Y. Ikada, J. Polym. Sci., Part A: Polym. Chem., 1998, 36, 59–66 CrossRef CAS.
- H. Tsuji and T. Ishizaka, J. Appl. Polym. Sci., 2001, 80, 2281–2291 CrossRef CAS.
Footnote |
† Electronic supplementary information (ESI) available: S1. GPC charts of PB diol, PLLA-PB-PLLA, PEG, and PLLA-PEG-PLLA (Fig. S1), S2. DSC thermograms of PLLA films before and after coating (Fig. S2), S3. Thermal properties of PLLA films before and after coating (Table S1). See DOI: https://doi.org/10.1039/d2ma00417h |
|
This journal is © The Royal Society of Chemistry 2022 |
Click here to see how this site uses Cookies. View our privacy policy here.