DOI:
10.1039/D1MO00257K
(Research Article)
Mol. Omics, 2022,
18, 83-91
4D-quantitative proteomics signature of asthenozoospermia and identification of extracellular matrix protein 1 as a novel biomarker for sperm motility†
Received
28th July 2021
, Accepted 30th October 2021
First published on 24th November 2021
Abstract
Asthenozoospermia (AZS), diagnosed by reduced sperm motility, is one of the major causes of male infertility. However, AZS has no effective therapeutic treatment and the underlying molecular mechanism remains largely unclear. In this study, state-of-the-art 4D-quantitative proteomics analysis was used to compare the protein profiling between 7 normozoospermic and 11 asthenozoospermic sperm samples. Overall, 4718 proteins were identified and 1430 differential abundant proteins were found in the two groups. The differentially expressed proteins were analyzed by GO and KEGG. The core deregulated proteins and pathways associated sperm motility dysfunction included energy metabolism and the sperm structure. Integrative analysis further identified extracellular matrix protein 1 (ECM1) as a novel biomarker related to AZS. Our study could provide new insights into the molecular basis of low sperm motility. The mass spectrometric data are available via ProteomeXchange with identifier PXD027637.
Introduction
Infertility is a worldwide problem affecting about 15% of couples trying to conceive. Asthenozoospermia (AZS) is a common cause of human male infertility, which is characterized by reduced forward motility with progressive motility <32% (World Health Organization, 2010). There are two types of AZS, isolated AZS (approximately accounts for 20% of infertile men) and AZS associated with oligo- and/or teratozoospermia (approximately accounts for 60% of infertile men).1,2 The molecular mechanism underlying AZS remains largely unknown. It has become clear that identifying precise proteins and pathways involved in sperm motility is needed.
The highly specialized sperm is a kind of transcriptional and translational silenced cell, which makes it particularly suitable for proteomics studies.3 In fact, several groups have tried to explore the detailed molecular basis of AZS using proteomics technologies, including initially two-dimensional (2D) gel electrophoresis4–8 and more recently three-dimensional (3D) proteomics.9–12 However, comparison of the published proteomics data on AZS has shown that none of the proteins have been identified by all studies. This great divergent might be attributable to the small dynamic range, lack of sensitivity to detect low abundance proteins, and high heterogeneity of AZS.
Mass spectrometry technology has greatly improved in recent years. The state-of-the-art 4D proteomics constitutes a fourth dimension, ion mobility, in addition to mass over charge (m/z), retention time, and signal intensity.13 Ion mobility spectrometry (IMS) mainly separates molecules in the gas phase by their collisional cross section (CCS), and can distinguish peptides with very small m/z differences. In this way, low-abundance protein signals can be distinguished and identified by 4D proteomics analysis, which has high depth, high throughput, and high sensitivity.14,15
In the present study, we initially quantified the proteome of AZS patients and NZS subjects by 4D proteomics utilizing a trapped ion mobility spectrometry (TIMS) device operated with the parallel accumulation-serial fragmentation (PASEF) scan mode. In total, 1430 differentially abundant proteins were identified, and previously reported protein markers of AZS were also presented in our list. Various pathways including energy metabolism and axonemal dynein complex were enriched according to bioinformatics analysis. By integration of various proteomics datasets, ECM1 was chosen as a potential up-regulated marker in AZS sperm.
Materials and methods
Human semen sample collection
This human study was approved by the Ethics Committee of Peking University Shenzhen Hospital. All the participants have signed the consent. Semen samples were collected by masturbation after 3–7 days of sexual abstinence. After liquefaction, all the semen samples were evaluated using a computer-assisted semen analyzer (CASA, Suijia Software Co., Ltd, Beijing, China). The motility of each spermatozoon was graded according to the World Health Organization laboratory manual 2010. In total, 7 normozoospermic men with normal sperm quality and 11 asthenozoospermic men (sperm concentration ≥15 million cell per ml, progressive motility <32%, total motility <40%, morphologically normal forms ≥4.0%) were recruited in this study. The basic semen parameters in patients and controls are described in Table 1. Compared with normozoospermic controls, the asthenozoospermic samples presented a significant difference in sperm motility when all other parameters were normal. All of the participants were also checked to be free of other common diseases including mumps, mycoplasma infection, varicosity, prostatitis, epididymitis and orchitis. The possibility of chromosal aneuploidy was also excluded in all of the participants.
Table 1 Basic semen parameters in asthenozoospermic patients and controls
|
Asthenozoospermia |
Normozoospermia |
P value |
Values are presented as the mean ± SD. |
Age (year) |
36 ± 3.83 |
33 ± 6.05 |
0.36 |
Sperm count (× 106 sperm per ml) |
92.05 ± 43.90 |
224.15 ± 135.96 |
0.09 |
Volume (ml) |
4.13 ± 1.51 |
4.24 ± 1.41 |
0.96 |
Total motility (%) |
24.14 ± 6.72 |
69.57 ± 11.03 |
9.21 × 10−7 |
Progressive motility (%) |
15.00 ± 5.39 |
60.29 ± 10.97 |
2.22 × 10−6 |
Protein extraction and digestion
Sperm were separated from semen samples by 60% Percoll (Sigma Chemical, MO, USA) as previously described.16 The sperm pellets were resuspended in lysis buffer (1% Triton X-100, 1% protease inhibitor cocktail, 3 μM TSA, 50 mM NAM) and sonicated on ice using a Focused-Ultrasonicator (Covaris Inc., MA, USA). The remaining debris was removed by centrifugation at 12
000 g at 4 °C for 10 min. Finally, the supernatant was collected and protein concentration was determined by BCA kit (Thermo Fisher, China).
Equal amount of protein from each sample was subjected to enzymatic digestion and lysis buffer was used to adjust the volume of samples to the same. TCA was added to samples slowly at a final concentration of 20%. The samples were vortexed to mix well and settle at 4 °C for 2 hours. The samples were centrifuged at 4500 g for 5 min and the pellets were resuspended and precipitated with pre-cooled acetone 2–3 times. After drying the pellet, triethylammonium bicarbonate (TEAB) was added at a final concentration of 200 mM and the pellets were ultrasonically dispersed. The samples were digested with trypsin at a ratio of 1
:
50 (protease: protein, m/m) overnight. The proteins were reduced with dithiothreitol (DTT) at a final concentration of 5 mM (56 °C for 30 min), then alkylated with iodoacetamide (IAA) at a final concentration of 11 mM (15 min at room temperature in the dark).
4D-quantitative proteomic analysis
The prepared sperm proteins from the same group were pooled together and subjected to state-of-the-art 4D-quantitative proteomic analysis in PTM Biolabs (Hangzhou, China) as described in a previous study.15 The tests were repeated three times. Briefly, tryptic peptides were dissolved in solvent A (0.1% formic acid and 2% acetonitrile in water) and separated with solvent B (0.1% formic acid in acetonitrile) on a nanoElute high-performance liquid chromatography (UHPLC) system (Bruker Daltonics Inc., USA). A liquid gradient was set as follows: 0–70 min, 6%–24% B; 70–84 min, 24%–35% B; 84–87 min, 35%–80% B; 87–90 min, 80% B, all at a constant flow rate of 450 nL min−1.
The peptides were subjected to a capillary source followed by the timsTOF Pro (Bruker Daltonics Inc., USA) mass spectrometry. The electrospray voltage applied was 1.75 kV. Precursors and fragments were analyzed at the TOF detector, with a MS/MS scan range from 400 to 1500 m/z. The timsTOF Pro was operated in parallel accumulation serial fragmentation (PASEF) mode. Precursors with charge states 0 to 5 were selected for fragmentation, and 10 PASEF-MS/MS scans were acquired per cycle. The dynamic exclusion was set to 30 s.
The resulting MS/MS data were processed using a Maxquant search engine (v.1.6.15.0). Tandem mass spectra were searched against the Homo_sapiens_9606_SP_20201214.fasta database (20
395 sequences). Trypsin/P was specified as a cleavage enzyme allowing up to two missing cleavages. The mass tolerance for precursor ions was set as 20 ppm in both the first search and main search. Spectra, peptide and protein false discovery rate (FDR) were adjusted to <1%. The mass spectrometry proteomics data have been deposited to the ProteomeXchange Consortium via the PRIDE partner repository with the dataset identifier PXD027637.
Bioinformatics analysis
Principal component analysis (PCA),10 volcano plots of differentially abundant sperm proteins,11 enrichment analysis of Gene Ontology (GO) and pathway were performed as previously reported.15
Western blotting
Sperm proteins extracted as described above were separated by 10% SDS–PAGE and electrotransferred onto PVDF membranes (Millipore, Bedford, MA, USA). After blocking with 5% (w/v) non-fat milk in TBST (20 mM Tris–HCl pH 7.5, 150 mM NaCl, 0.1% Tween 20), the membranes were incubated with anti-ECM1 (11521-1-AP, Proteintech, China) overnight at 4 °C. Anti-GAPDH antibody (60004-1-Ig, Proteintech, China) was used as the internal control. The membranes were incubated with corresponding HRP-labeled secondary antibody for 1 h at room temperature. Positive bands were detected using an ECL kit (Thermo Scientific, Waltham, USA) and quantified by ImageJ (version 1.58).
ATP measurement
The ATP concentrations in sperm were analyzed by a bioluminescent ATP assay kit (Beyotime Biotechnology, China) according to the manufacturer's instructions.17 In brief, sperm samples were collected and lysed to release ATP. The detection reagents containing firefly luciferase were mixed with samples or standards, and the luminescence values were detected using a multimode detector (DTX880, Beckman, CA, USA). The experimental values were calculated according to the equation of ATP standard curve. Values were normalized by protein content (nmol mg−1).
Statistical analysis
A protein with fold change >1.5 and an FDR value <1% was considered differentially abundant. All values are expressed as mean ± standard deviation of the mean. Statistical analysis was performed using GraphPad Prism software (version 5.0; La Jolla, CA). Comparison of the two groups was assessed by a two tailed unpaired Student t test. All experiments were repeated at least 3 times, and P < 0.05 was considered significant.
Results
The protein landscape significantly differs between AZS and NZS
11 asthenozoospermia patients and 7 normal controls were enrolled and their basic semen parameters are shown in Table 1. The sperm proteins from the same group were pooled together and subjected to 4D label-free quantitative proteomics analysis. Three experimental repetitions were performed to evaluate technical variation. After mass spectrometry analysis, a total of 4718 proteins were identified, of which 3753 proteins were quantified (Table S1, ESI†).
We then evaluated the distributions of unique peptide numbers and found that 90% of proteins were identified with more than 2 unique peptides (Fig. 1B). Principal component analysis (PCA) of all samples revealed clear separation of AZS and NZS (Fig. 1C).
 |
| Fig. 1 (A) 4D-proteomics detects more differentially abundant proteins. (B) Distribution of the number of peptides per protein. (C) The PCA analysis of the protein profile between NZS and AZS sperm. The two groups are presented in a different clustering pattern. Principal coordinate (PC) 1 and PC2 are the x axis and y axis, respectively, and have been scaled on the basis of percent variance. (D) Volcano plots of differentially abundant sperm proteins. Proteins with log2 (fold change) in expression compared with −log10 (P value). 729 downregulated proteins and 701 upregulated proteins were identified in asthenozoospermic sperm. | |
Globally, 1430 proteins were found to be differentially abundant (FDR < 1%, Fold change >1.5), with 701 up-regulated and 729 down-regulated in AZS sperm (Fig. 1D; Table S1, ESI†). When compared with published datasets,9–12 our study identified the largest number of differentially abundant proteins, about 4–10 fold that found in those studies (Fig. 1A).
Comprehensive functional annotation of sperm proteins
Gene Ontology (GO) overview based on the second-highest level GO terms, categorized into Biological Process, Cellular Components and Molecular Functions. In each GO term, a group of genes with the same function are listed together. Biological Process clusters mainly include the cell wall macromolecule catabolic process, cell wall macromolecule metabolic process, cillium-dependent cell motility, monosaccharide biosynthetic process, and cellular respiration (Fig. S1, ESI†). Molecular Functions clusters mainly consist of lysozyme activity, fatty-acyl–CoA binding, acyl–CoA dehydrogenase activity, and 3-hydroxyacyl–CoA dehydrogenase activity (Fig. S2, ESI†).
The top 5 enriched terms in cellular components are axonemal dynein complex, dynein complex, respiratory chain complex I, and mitochonrial respiratory chain complex I (Fig. 2A).
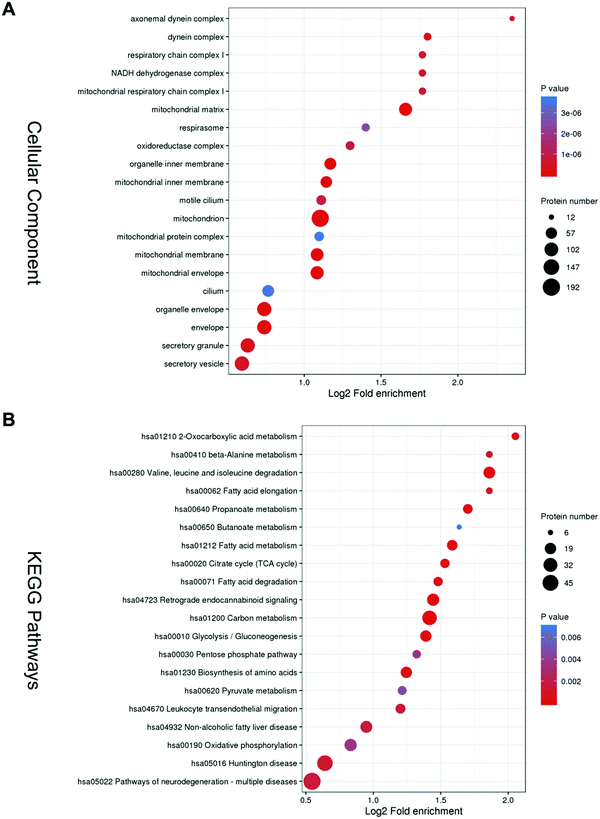 |
| Fig. 2 Functional annotation and pathway analysis of differentially abundant sperm proteins. Cellular function of differentially abundant proteins based on enrichment analysis of (A) Gene Ontology (GO) and (B) KEGG pathway. A strongly enriched term indicates comparably more proteins related to this function are present in the observed data. | |
KEGG analysis was performed and it was mainly enriched in energy metabolism. The top five enriched pathways are oxocarboxylic acid metabolism, beta-Alanine metabolism, valine, leucine and isoleucine degradation, fatty acid elongation, and propanoate metabolism (Fig. 2B).
These bioinformatics results are comparable to previous ones, suggesting that asthenozoospermia may be caused by deficiencies in sperm structure or energy production.
Previously reported biomarkers of AZS are also present in our study
To better integrate our findings with what is already known about AZS pathogenesis, we examined the expression of protein markers that have been implicated in previous studies. Glucose-6-phosphate isomerase (GPI), phosphoglycerate mutase 1 (PGAM1) and PGAM2, cytosolic malate dehydrogenase (MDH1), and tubulin polymerization-promoting protein family member 2 (TPPP2) are the most recent markers validated by Guo et al.11 In our study, GPI, an important enzyme involved in the aldehyde catabolic process, was significantly reduced to 0.4521 fold in AZS sperm (Table 2). PGAM1 and PGAM2, which play a role in canonical glycolysis, were significantly reduced to 0.8009 and 0.5801 fold in AZS sperm (Table 2). MDH1, which is important in mitochondrial NADH supply for oxidative phosphorylation, was significantly reduced to 0.5051 fold in AZS sperm (Table 2). TPPP2, which participates in microtubule bundle formation, was significantly reduced to 0.4729 fold in AZS sperm (Table 2).
Table 2 Previously validated protein markers of AZS are also present in our study
Protein ID |
Protein name |
Gene symbol |
Fold change |
Function |
Fold change: AZS vs. NZS. The fold change reported in published studies is indicated in brackets. |
A0A2U3TZU2 |
Glucose-6-phosphate isomerase |
GPI |
0.4521(0.536710/0.7449) |
Aldehyde catabolic process |
P18669 |
Phosphoglycerate mutase 1 |
PGAM1 |
0.8009(0.530210) |
Canonical glycolysis |
P15259 |
Phosphoglycerate mutase 2 |
PGAM2 |
0.5801(0.567210) |
Canonical glycolysis |
P40925 |
Cytosolic malate dehydrogenase |
MDH1 |
0.5051(0.654810) |
Gluconeogenesis |
P59282 |
Tubulin polymerization-promoting protein family member 2 |
TPPP2 |
0.4729(0.360110) |
Microtubule bundle formation |
|
|
|
|
|
These results demonstrate that the previously validated biomarkers of AZS are also included in our list of differentially expressed proteins. It confirms that our 4D-quantitative proteomics data are of high confidence.
Integrative analysis of proteomic studies in AZS
We then integrated our results with published proteomic data of AZS, but none of the proteins have been identified by all studies. Extracellular matrix protein 1 (ECM1, Protein ID_Q16610) is the only protein simultaneously present in our study as well as two other ones (Fig. 3A and B). The direct relationship between ECM1 and sperm motility has not been reported before. The protein interaction network of ECM1 was analyzed by STING (https://www.string-db.org/) (Fig. 3C). It was suggested that metalloproteinase inhibitor 3 (TIMP3) warranted to be noticed (Fig. 3C).
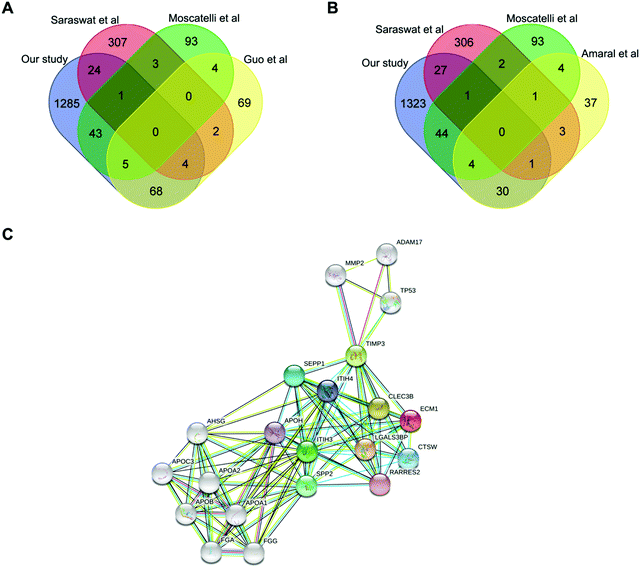 |
| Fig. 3 Comparison with the published proteomes. (A and B) The overlap among our sperm proteome and previously published data. The results are shown as a Venn diagram. (C) Protein–protein interaction analysis of ECM1 by STRING (https://www.string-db.org/). | |
ECM1 is a 61 kD intracellular protein,18 or a 85 kD secreted glycoprotein.19 According to the Human Protein Atlas (http://www.proteinatlas.org), ECM1 highly expresses in epididymis, esophagus and lymphoid tissues. It has been reported that ECM1 could be released into seminal plasma from epididymis.20 In our study, the expression level of ECM1 in AZS sperm is 2.18 fold of that in NZS (Fig. 4A). Meanwhile, the expression level of TIMP3 in AZS sperm is 1.43 fold that in NZS (Fig. 4B).
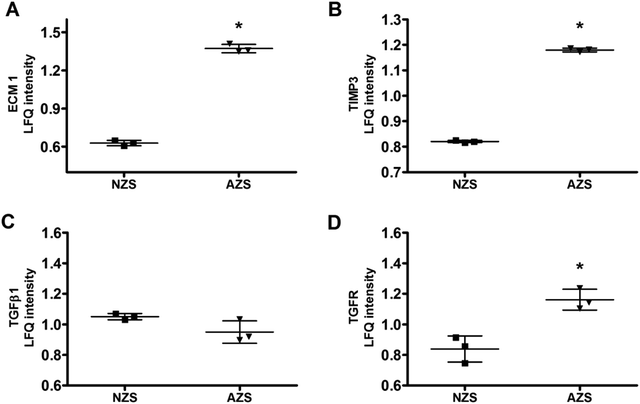 |
| Fig. 4 Protein expression levels of (A) ECM1, (B) TIMP3, (C) TGFβ1, and (D) TGFR in NZS and AZS sperm. *, p < 0.05, AZS vs. NZS, n = 3. | |
ECM1 prevents activation of transforming growth factor beta (TGFβ).19,21 Thus, we also checked the expression levels of TGFβ1 and TGF receptor (TGFR). As shown in Fig. 4C and D, the expression of TGFβ1 didn't change, while TGFR increased slightly but significantly.
Validation of ECM1 expression by Western blotting
High-throughput techniques are very effective in elucidating the differential abundant proteins in the clinical samples. To confirm the proteomic data, we analyzed both sets of samples using Western blotting. The results showed higher abundances of ECM1 in AZS sperm (Fig. 5A and B), which is in agreement with quantitative proteomic results.
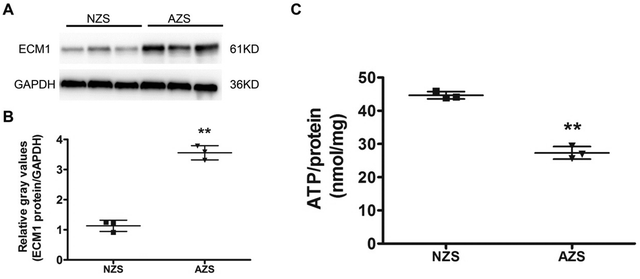 |
| Fig. 5 Western blotting verification of ECM1 and ATP measurement. (A) Western blotting analysis performed with antibodies against ECM1. GAPDH was used as a loading control. (B) Intensity analysis of Western blotting results for ECM1 normalized against GAPDH. **, p < 0.01, AZS vs. NZS, n = 3. (C) ATP concentrations were determined by a commercial kit. **, p < 0.01, AZS vs. NZS, n = 3. | |
Decreased ATP production in AZS sperm
The coordinated beating of sperm flagellum is regulated by axonemal heavy chain dynein ATPase via ATP hydrolysis.22 Indeed, lower ATP content has been demonstrated to be associated with reduced progressive motility.17,22 As described above, proteins related to energy metabolism are key cellular components identified by our proteomics analysis. Thus, we set out to compare intracellular ATP production between NZS and AZS sperm using an ATP assay kit. It was shown that the ATP concentration in AZS sperm was significantly reduced by ∼50% (Fig. 5C).
Discussion
There have been a few studies trying to identify biomarkers of AZS by proteomics, but due to an extremely low level of overlap between the results of these studies, more research is still needed. In this study we have used, for the first time, state-of-art 4D proteomics to analyze the differentially abundant proteins between NZS and AZS sperm. 1430 proteins were detected as either increased or decreased candidates in the AZS group. GO and KEGG analysis showed the top enriched terms in down-regulated genes involved in energy production and metabolic processes, cilium motility and axonemal structure. Furthermore, our study identified ECM1 as a new biomarker of AZS.
Although the underlying mechanism remains unknown, AZS is probably associated with deficiencies in sperm structure, energy metabolism or seminal plasma, or by a combination of these. Our results are consistent with published studies, suggesting malfunction of energy metabolism and axonemal structure in AZS sperm. In our study, we found that GPI, MDHI, PGAM2 and TPPP2 showed similar changes in AZS to those previously observed.9,11 Furthermore, studies on Tppp2 knockout mice demonstrated that deletion of TPPP2 resulted in male subfertility with significantly lower ATP levels, decreased sperm count and motility.23
Moreover, integrative analysis helped us to pick ECM1 out of 1430 protein deposits. We have detected an increased amount of ECM1 in AZS sperm. ECM1 has been reported to be a potential biomarker to distinguish between non-obstructive azoospermia (NOA) and obstructive azoospermia (OA). They found that the levels of ECM1 were notably decreased in seminal plasma of OA samples (∼1 μg ml−1), when compared to NZS (∼40 μg ml−1) and NOA (∼20 μg ml−1) samples.20,24 However, the relationship between ECM1 and asthenozoospermia is still unclear. ECM1 may have great potential as a novel marker among the differential abundant proteins between AZS and NZS.
ECM1 is one component of extracellular matrix, which directly affects cell fate, tissue organization, and function. Su et al.21 and Fan et al.19 showed that ECM1 may interact with αv integrins and thus inhibit latent TGFβ activation. ECM1 is required to maintain tissue homeostasis and decreased ECM1 expression was associated with more severe fibrosis progression.19 In this study, the expression of TGFβ1 didn't change, while TGFR increased significantly in AZS sperm. But the mechanism by which this phenomenon occurs remains unknown.
Seminal plasma contains up to 3200 proteins that are secreted by testes, epididymis, prostate, seminal vesicles, and Cowper's glands.25 Proteomic technology has rapidly been developed as a powerful tool to identify potential novel biomarkers from seminal-specific proteins.26 Determining the seminal plasma level of ECM1 in AZS may help to identify the original cell/tissue responsible for ECM1 production. During sperm maturation in epididymis, the specific secretory and absorptive activities of the epididymis epithelial cells makes the exchange of proteins between sperm and seminal plasma thorough. The upregulated ECM1 in sperm may be caused by an abnormal concentration of ECM1 in seminal plasma, which may be due to secretion and/or absorption dysfunction of the epididymis.
In conclusion, 4D-quantitative proteomics analysis of AZS samples identified 1430 differentially abundant proteins associated with sperm motility. Of note, upregulation of ECM1 in sperm may be a newly found cause of low sperm motility in AZS patients. The higher level of ECM1 in sperm may be due to an abnormal sperm maturation process. This study can help to elucidate the molecular mechanism underlying sperm motility and provide new therapeutic targets for AZS.
Data availability
The mass spectrometric data are available via ProteomeXchange with identifier PXD027637. The datasets supporting this article have also been uploaded as part of the supplementary material.
Author contributions
Performing experiments, J. Y., sample collection, Q. L. L and B. H. Y., data analysis and interpretation, B. Y. and B. Q. H, writing original draft, B. Y. and B. H. Y., financial support, B. Y., revising manuscript, B. Y. and B. Q. H.
Conflicts of interest
The authors declare no conflicts of interest.
Acknowledgements
This work was supported by grants from the Shenzhen Project of Science and Technology (KQJSCX20180327124222443) and the Guangdong Natural Science Foundation (2018A030313669).
References
- S. M. Curi, J. I. Ariagno, P. H. Chenlo, G. R. Mendeluk, M. N. Pugliese, L. M. Sardi Segovia, H. E. Repetto and A. M. Blanco, . Asthenozoospermia: analysis of a large population, Arch. Androl., 2003, 49(5), 343–349 CrossRef CAS PubMed.
- Z. Heidary, K. Saliminejad, M. Zaki-Dizaji and H. R. Khorram Khorshid, Genetic aspects of idiopathic asthenozoospermia as a cause of male infertility, Hum. Fertil., 2020, 23(2), 83–92 CrossRef PubMed.
- R. Oliva, S. de Mateo and J. M. Estanyol, Sperm cell proteomics, Proteomics, 2009, 9(4), 1004–1017 CrossRef CAS PubMed.
- C. Zhao, R. Huo, F. Q. Wang, M. Lin, Z. M. Zhou and J. H. Sha, Identification of several proteins involved in regulation of sperm motility by proteomic analysis, Fertil. Steril., 2007, 87(2), 436–438 CrossRef CAS PubMed.
- J. Martinez-Heredia, S. de Mateo, J. M. Vidal-Taboada, J. L. Ballesca and R. Oliva, Identification of proteomic differences in asthenozoospermic sperm samples, Hum. Reprod., 2008, 23(4), 783–791 CrossRef CAS PubMed.
- A. B. Siva, D. B. Kameshwari, V. Singh, K. Pavani, C. S. Sundaram, N. Rangaraj, M. Deenadayal and S. Shivaji, . Proteomics-based study on asthenozoospermia: differential expression of proteasome alpha complex, Mol. Hum. Reprod., 2010, 16(7), 452–462 CrossRef CAS.
- S. Shen, J. Wang, J. Liang and D. He, Comparative proteomic study between human normal motility sperm and idiopathic asthenozoospermia, World J. Urol., 2013, 31(6), 1395–1401 CrossRef CAS PubMed.
- M. Hashemitabar, S. Sabbagh, M. Orazizadeh, A. Ghadiri and M. Bahmanzadeh, A proteomic analysis on human sperm tail: comparison between normozoospermia and asthenozoospermia, J. Assist. Reprod. Genet., 2015, 32(6), 853–863 CrossRef PubMed.
- A. Amaral, C. Paiva, C. Attardo Parrinello, J. M. Estanyol, J. L. Ballesca, J. Ramalho-Santos and R. Oliva, Identification of proteins involved in human sperm motility using high-throughput differential proteomics, J. Proteome Res., 2014, 13(12), 5670–5684 CrossRef CAS PubMed.
- M. Saraswat, S. Joenvaara, T. Jain, A. K. Tomar, A. Sinha, S. Singh, S. Yadav and R. Renkonen, Human Spermatozoa Quantitative Proteomic Signature Classifies Normo- and Asthenozoospermia, Mol. Cell. Proteomics, 2017, 16(1), 57–72 CrossRef CAS.
- Y. Guo, W. Jiang, W. Yu, X. Niu, F. Liu, T. Zhou, H. Zhang, Y. Li, H. Zhu, Z. Zhou, J. Sha, X. Guo and D. Chen, Proteomics analysis of asthenozoospermia and identification of glucose-6-phosphate isomerase as an important enzyme for sperm motility, J. Proteomics, 2019, 208, 103478 CrossRef CAS PubMed.
- N. Moscatelli, P. Lunetti, C. Braccia, A. Armirotti, F. Pisanello, M. De Vittorio, V. Zara and A. Ferramosca, Comparative Proteomic Analysis of Proteins Involved in Bioenergetics Pathways Associated with Human Sperm Motility, Int. J. Mol. Sci., 2019, 20(12), 3000 CrossRef CAS.
- F. Meier, A. D. Brunner, S. Koch, H. Koch, M. Lubeck, M. Krause, N. Goedecke, J. Decker, T. Kosinski, M. A. Park, N. Bache, O. Hoerning, J. Cox, O. Rather and M. Mann, Online Parallel Accumulation-Serial Fragmentation (PASEF) with a Novel Trapped Ion Mobility Mass Spectrometer, Mol. Cell. Proteomics, 2018, 17(12), 2534–2545 CrossRef CAS.
- C. G. Vasilopoulou, K. Sulek, A. D. Brunner, N. S. Meitei, U. Schweiger-Hufnagel, S. W. Meyer, A. Barsch, M. Mann and F. Meier, Trapped ion mobility spectrometry and PASEF enable in-depth lipidomics from minimal sample amounts, Nat. Commun., 2020, 11(1), 331 CrossRef CAS PubMed.
- M. Wu, Y. Chen, H. Xia, C. Wang, C. Y. Tan, X. Cai, Y. Liu, F. Ji, P. Xiong, R. Liu, Y. Guan, Y. Duan, D. Kuang, S. Xu, H. Cai, Q. Xia, D. Yang, M. W. Wang, I. M. Chiu, C. Cheng, P. P. Ahern, L. Liu, G. Wang, N. K. Surana, T. Xia and D. L. Kasper, Transcriptional and proteomic insights into the host response in fatal COVID-19 cases, Proc. Natl. Acad. Sci. U. S. A., 2020, 117(45), 28336–28343 CrossRef CAS PubMed.
- Y. Du, M. Li, J. Chen, Y. Duan, X. Wang, Y. Qiu, Z. Cai, Y. Gui and H. Jiang, Promoter targeted bisulfite sequencing reveals DNA methylation profiles associated with low sperm motility in asthenozoospermia, Hum. Reprod., 2016, 31(1), 24–33 CrossRef CAS.
- J. M. Castaneda, R. Hua, H. Miyata, A. Oji, Y. Guo, Y. Cheng, T. Zhou, X. Guo, Y. Cui, B. Shen, Z. Wang, Z. Hu, Z. Zhou, J. Sha, R. Prunskaite-Hyyrylainen, Z. Yu, R. Ramirez-Solis, M. Ikawa, M. M. Matzuk and M. Liu, TCTE1 is a conserved component of the dynein regulatory complex and is required for motility and metabolism in mouse spermatozoa, Proc. Natl. Acad. Sci. U. S. A., 2017, 114(27), E5370–E5378 CrossRef CAS PubMed.
- H. Cai, S. Qi, Q. Yan, J. Ling, J. Du and L. Chen, Global proteome profiling of human livers upon ischemia/reperfusion treatment, Clin. Proteomics, 2021, 18(1), 3 CrossRef CAS PubMed.
- W. Fan, T. Liu, W. Chen, S. Hammad, T. Longerich, I. Hausser, Y. Fu, N. Li, Y. He, C. Liu, Y. Zhang, Q. Lian, X. Zhao, C. Yan, L. Li, C. Yi, Z. Ling, L. Ma, X. Zhao, H. Xu, P. Wang, M. Cong, H. You, Z. Liu, Y. Wang, J. Chen, D. Li, L. Hui, S. Dooley, J. Hou, J. Jia and B. Sun, ECM1 Prevents Activation of Transforming Growth Factor beta, Hepatic Stellate Cells, and Fibrogenesis in Mice, Gastroenterology, 2019, 157(5), 1352–1367 e13 CrossRef CAS.
- A. P. Drabovich, A. Dimitromanolakis, P. Saraon, A. Soosaipillai, I. Batruch, B. Mullen, K. Jarvi and E. P. Diamandis, Differential diagnosis of azoospermia with proteomic biomarkers ECM1 and TEX101 quantified in seminal plasma, Sci. Transl. Med., 2013, 5(212), 212ra160 Search PubMed.
- P. Su, S. Chen, Y. H. Zheng, H. Y. Zhou, C. H. Yan, F. Yu, Y. G. Zhang, L. He, Y. Zhang, Y. Wang, L. Wu, X. Wu, B. Yu, L. Y. Ma, Z. Yang, J. Wang, G. Zhao, J. Zhu, Z. Y. Wu and B. Sun, Novel Function of Extracellular Matrix Protein 1 in Suppressing
Th17 Cell Development in Experimental Autoimmune Encephalomyelitis, J. Immunol., 2016, 197(4), 1054–1064 CrossRef CAS PubMed.
- G. W. Dougherty, K. Mizuno, T. Nothe-Menchen, Y. Ikawa, K. Boldt, A. Ta-Shma, I. Aprea, K. Minegishi, Y. P. Pang, P. Pennekamp, N. T. Loges, J. Raidt, R. Hjeij, J. Wallmeier, H. Mussaffi, Z. Perles, O. Elpeleg, F. Rabert, H. Shiratori, S. J. Letteboer, N. Horn, S. Young, T. Strunker, F. Stumme, C. Werner, H. Olbrich, K. Takaoka, T. Ide, W. K. Twan, L. Biebach, J. Grosse-Onnebrink, J. A. Klinkenbusch, K. Praveen, D. C. Bracht, I. M. Hoben, K. Junger, J. Gutzlaff, S. Cindric, M. Aviram, T. Kaiser, Y. Memari, P. P. Dzeja, B. Dworniczak, M. Ueffing, R. Roepman, K. Bartscherer, N. Katsanis, E. E. Davis, I. Amirav, H. Hamada and H. Omran, CFAP45 deficiency causes situs abnormalities and asthenospermia by disrupting an axonemal adenine nucleotide homeostasis module, Nat. Commun., 2020, 11(1), 5520 CrossRef CAS.
- F. Zhu, P. Yan, J. Zhang, Y. Cui, M. Zheng, Y. Cheng, Y. Guo, X. Yang, X. Guo and H. Zhu, Deficiency of TPPP2, a factor linked to oligoasthenozoospermia, causes subfertility in male mice, J. Cell. Mol. Med., 2019, 23(4), 2583–2594 CrossRef CAS PubMed.
- D. Korbakis, C. Schiza, D. Brinc, A. Soosaipillai, T. D. Karakosta, C. Legare, R. Sullivan, B. Mullen, K. Jarvi, E. P. Diamandis and A. P. Drabovich, Preclinical evaluation of a TEX101 protein ELISA test for the differential diagnosis of male infertility, BMC Med., 2017, 15(1), 60 CrossRef.
- I. Batruch, C. R. Smith, B. J. Mullen, E. Grober, K. C. Lo, E. P. Diamandis and K. A. Jarvi, Analysis of seminal plasma from patients with non-obstructive azoospermia and identification of candidate biomarkers of male infertility, J. Proteome Res., 2012, 11(3), 1503–1511 CrossRef CAS.
- D. Milardi, G. Grande, F. Vincenzoni, I. Messana, A. Pontecorvi, L. De Marinis, M. Castagnola and R. Marana, Proteomic approach in the identification of fertility pattern in seminal plasma of fertile men, Fertil. Steril., 2012, 97(1), 67–73 e1 CrossRef CAS PubMed.
Footnote |
† Electronic supplementary information (ESI) available. See DOI: 10.1039/d1mo00257k |
|
This journal is © The Royal Society of Chemistry 2022 |
Click here to see how this site uses Cookies. View our privacy policy here.