DOI:
10.1039/D1QO01414E
(Research Article)
Org. Chem. Front., 2022,
9, 88-94
DBU-catalyzed dearomative annulation of 2-pyridylacetates with α,β-unsaturated pyrazolamides for the synthesis of multisubstituted 2,3-dihydro-4H-quinolizin-4-ones†
Received
20th September 2021
, Accepted 6th November 2021
First published on 9th November 2021
Abstract
The reaction of 2-pyridylacetates and α,β-unsaturated pyrazolamides with DBU as the catalyst has been developed. A range of unexplored multisubstituted 2,3-dihydro-4H-quinolizin-4-ones are obtained with satisfactory yields (up to 94%) and excellent diastereoselectivities (all cases >20
:
1 dr) via a dearomative [3 + 3] annulation process. This practical method also features transition metal free, mild reaction conditions, wide functional group tolerance, easy scale-up synthesis, and versatile further derivatization.
Functionalized heterocyclic scaffolds are attractive and interesting synthetic targets for the preparation of diversity-oriented compound libraries in medicinal and pharmaceutical applications.1 In particular, almost all of the small-molecule drugs approved by the FDA contain at least one heterocyclic moiety.2 The development of new synthetic strategies for the construction of functionalized heterocycles with structural diversification has always been a hot spot and main focus of research in organic synthesis. 4H-Quinolizin-4-one and its derivatives, as a type of N-containing heterocyclic compound, have been considered as important components in modern pharmaceuticals and versatile building blocks for drug and natural product synthesis.3 Consequently, an increasing number of creative methods have been developed for the preparation of 4H-quinolizin-4-one and its diverse derivatives.4 Despite the significant progress, inspired by their intriguing structural features and potential biological and pharmaceutical applications, the synthesis of diverse 4H-quinolizin-4-ones with innovative structures is not only of high value, but is also arousing much research attention from medical and synthetic organic chemists to develop novel and efficient methodologies.
Dearomatization of heteroarenes involving an annulation process represents an attractive strategy to access non-aromatic heterocycles from readily available raw materials in a very straightforward manner.5 In this research area, the possible involvement of 2-pyridylacetates for the construction of heterocyclic structures containing a dearomatized-pyridine moiety via a dearomative annulation process has been widely reported.6 Actually, from a reaction point of view, in these methods, the intrinsic 1,3-dinucleophilic character of 2-pyridylacetates makes them a type of C1–N3 synthon that reacts with appropriate 1,n-dielectrophilic partners to access aza-heterocycles via a dearomative [3 + n] annulation (Scheme 1). To the best of our knowledge, the majority of the existing reports on this aspect mainly focus on the formal [3 + 2] annulation to generate various indolizines and their derivatives (Scheme 1, left).7 However, the reaction involving 2-pyridylacetates as 1,3-dinucleophilic partners reacting with possible 1,3-dielectrophilic components for dearomative [3 + 3] annulation is relatively underdeveloped, and only a few scattered examples of the construction of 4H-quinolizin-4-one scaffolds have been reported (Scheme 1, right).8 Therefore, it is still highly desirable to develop feasible approaches for the synthesis of 4H-quinolizin-4-one derivatives with structural diversification.
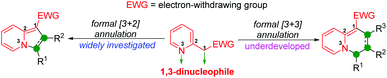 |
| Scheme 1 Profile of 2-pyridylacetates acting as 1,3-dinucleophiles for the dearomative annulation. | |
The employment of α,β-unsaturated pyrazolamides as 1,3-dielectrophilic reactants for the construction of various carbonyl-containing heterocycles has been reported.9 With comprehensive consideration of the 1,3-dinucleophilic character of 2-pyridylacetates and 1,3-dielectrophilic character of α,β-unsaturated pyrazolamides, we envisioned that the reaction of these two reactants would result in the formation of 4H-quinolizin-4-one scaffolds via a dearomative [3 + 3] annulation process (Scheme 2). As a continuation of our research interest in the development of new methodologies for the construction of various heterocycles,10 recently, we have discovered that one molecule of 2-pyridylacetate could react with two molecules of α,β-unsaturated pyrazolamide using DBU as the catalyst, affording a wide range of unexplored multisubstituted 2,3-dihydro-4H-quinolizin-4-ones with satisfactory yields and excellent diastereoselectivities (Scheme 2). Moreover, this practical method also features transition metal free, mild reaction conditions, wide functional group tolerance, easy scale-up synthesis, and versatile further functionalizations. Herein, we wish to report our research on this subject.
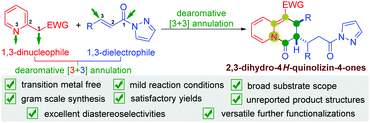 |
| Scheme 2 The strategy for the construction of multisubstituted 2,3-dihydro-4H-quinolizin-4-ones with 2-pyridylacetates and α,β-unsaturated pyrazolamides via dearomative [3 + 3] annulation. | |
Our optimization studies began with the reaction between 2-pyridylacetate 1a and α,β-unsaturated pyrazolamide 2a in DMF at room temperature. As shown in Table 1, in the presence of 1.0 equivalent of TMG as a base, it was found that one molecule of 1a actually reacted with two molecules of 2avia a dearomative [3 + 3] annulation process, leading to the formation of 3aa in 33% yield with a >20
:
1 diastereoselectivity ratio (dr) (entry 1). Using 1.0 equivalent of DBU resulted in product 3aa in 65% yield with a 16
:
1 dr (entry 2). DIPEA or TEA led to the failure of the reaction (entries 3 and 4). The inorganic base KOH could afford 3aa in 35% yield with an 8
:
1 dr (entry 5). After DBU was chosen as the optimal base, various solvents were examined (entries 6–10). It was found that the reaction did not occur in nonpolar solvents such as CH2Cl2, THF, and toluene (entries 6–8), but DMSO and MeCN could yield 3aa in moderate levels with a 12
:
1 dr (entries 9 and 10). However, relatively favorable results were still obtained in DMF (entry 2). On reducing the loading of DBU to 30 mol%, the reaction furnished 3aa in 69% yield with a >20
:
1 dr (entry 11), whereas 20 mol% of DBU promoted the reaction to generate 3aa in 71% yield with a >20
:
1 dr (entry 12). Increasing the reactant concentration led to a slight enhancement in the yield (entry 13). Ultimately, on changing the molar ratio of 1a and 2a from 1
:
2.5 to 1
:
3.5, product 3aa was obtained in 79% yield with a >20
:
1 dr (entry 14). Disappointingly, on changing the base from DBU to TBD or DBN, no improved results were obtained (entries 15 and 16).
Table 1 Optimization of reaction conditionsa
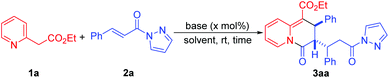
|
Entry |
Base |
x
|
Solvent |
Time (h) |
Yieldb (%) |
drc |
Unless otherwise noted, the reactions were carried out with 1a (0.4 mmol), 2a (1.0 mmol, 2.5 equiv.), and a base (x mol%) in 4.0 mL of solvent at room temperature.
Isolated yield.
Determined by 1H NMR analysis.
2.0 mL of DMF was used.
1a : 2a = 1 : 3.5. TMG = 1,1,3,3-tetramethylguanidine, DBU = 1,8-diazabicyclo[5.4.0]undec-7-ene, DIPEA = N,N-diisopropylethylamine, TEA = triethylamine, TBD = 1,5,7-triazabicyclo[4.4.0]dec-5-ene, DBN = 1,5-diazablcyclo[4.3.0]non-5-ene and nr = no reaction.
|
1 |
TMG |
100 |
DMF |
12 |
33 |
>20 : 1 |
2 |
DBU |
100 |
DMF |
12 |
65 |
16 : 1 |
3 |
DIPEA |
100 |
DMF |
12 |
nr |
— |
4 |
TEA |
100 |
DMF |
12 |
nr |
— |
5 |
KOH |
100 |
DMF |
12 |
35 |
8 : 1 |
6 |
DBU |
100 |
CH2Cl2 |
12 |
nr |
— |
7 |
DBU |
100 |
THF |
12 |
nr |
— |
8 |
DBU |
100 |
Toluene |
12 |
nr |
— |
9 |
DBU |
100 |
DMSO |
12 |
52 |
12 : 1 |
10 |
DBU |
100 |
MeCN |
12 |
38 |
12 : 1 |
11 |
DBU |
30 |
DMF |
24 |
69 |
>20 : 1 |
12 |
DBU |
20 |
DMF |
24 |
71 |
>20 : 1 |
13d |
DBU |
20 |
DMF |
24 |
76 |
>20 : 1 |
14d,e |
DBU |
20 |
DMF |
24 |
79 |
>20 : 1 |
14d,e |
TBD |
20 |
DMF |
24 |
12 |
18 : 1 |
15d,e |
DBN |
20 |
DMF |
24 |
33 |
19 : 1 |
With the optimized reaction conditions in hand, we explored the generality of the dearomative [3 + 3] annulation. Different 2-pyridylacetates were firstly examined by reacting with α,β-unsaturated pyrazolamide 2a under the standard conditions. As shown in Scheme 3, all of the reactions afforded their corresponding 2,3-dihydro-4H-quinolizin-4-one products with excellent diastereoselectivities (all cases >20
:
1 dr). The ester group of 1 could range from ethyl-ester to methyl-, propyl-, butyl-, or benzyl-ester, all of which reacted well with 2a to give their respective products 3ba–ea in good yields. On changing the ethyl-ester group of 1a to the sterically hindered isopropyl- or tertiary butyl-ester group, the corresponding products 3fa and 3ga were obtained in moderate yields. Moreover, cyclohexyl 2-(pyridin-2-yl)acetate also reacted with 2a to afford 3ha in 33% yield. In addition, trifluoro ethyl, allyl, and propargyl groups were also compatible with the reaction system to give the cycloadducts in good yields (for products 3ia–la). Furthermore, 2-((phenylsulfonyl)methyl)pyridine and 2-(pyridin-2-yl)acetonitrile were applicable to the reaction, providing the desired products 3ma and 3na in reasonable yields. Ultimately, it was found that substrates incorporating either an electron-donating or electron-withdrawing group at the C5-position of the pyridine ring also afforded the corresponding products but in low yields (for products 3oa–pa). Disappointedly, we also investigated the reaction of ethyl 2-(quinolin-2-yl)acetate, 1-phenyl-2-(pyridin-2-yl)ethan-1-one, 2-(pyridin-2-yl)acetaldehyde, and 2-(nitromethyl)pyridine with α,β-unsaturated pyrazolamide 2a under the standard conditions, respectively. It was found that these reactions got messy as monitored by TLC, and no main product could be obtained.
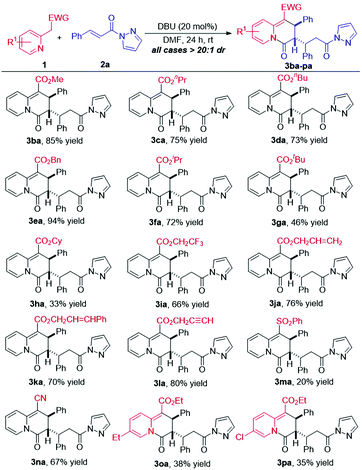 |
| Scheme 3 Substrate scope of 2-pyridylacetates. Reaction conditions: the reactions were carried out with 1 (0.4 mmol), 2a (1.4 mmol), and DBU (20 mol%) in 2.0 mL of DMF at room temperature for 24 h. The yield refers to the isolated yield after column chromatography. The dr was determined by 1H NMR analysis. | |
Next, various substituted α,β-unsaturated pyrazolamides were examined by their reactions with 2-pyridylacetate 1a under the standard conditions. As illustrated in Scheme 4, all of the reactions provided the corresponding cycloadducts with excellent diastereoselectivities (all cases >20
:
1 dr). On installing a chlorine atom at the m-position of the phenyl ring, product 3ab was smoothly obtained in 80% yield. Moreover, substrates with electron-withdrawing substituents, such as Cl–, F–, Br–, I–, CN–, and CF3– at the p-position of the phenyl ring, were also tolerated in the dearomative [3 + 3] annulation, affording 3ac–ah in moderate to good yields with excellent diastereoselectivities. Likewise, on incorporating an electron-donating substituent (Me– or MeO–) into the phenyl ring, the reactions proceeded smoothly to give 3ai and 3aj in good yields. In addition, the bulkier naphthyl group was also tolerated in the reaction with 1a to furnish 3ak in 67% yield with a >20
:
1 dr. Moreover, the heteroaromatic substituted α,β-unsaturated pyrazolamide substrate also could react effectively with 1a, delivering 3al in 70% yield with a >20
:
1 dr. Disappointingly, using β-methyl substituted α,β-unsaturated pyrazolamide or β-unsubstituted N-pyrazole acrylic amide failed to afford the desired products, probably due to the lower reactivity than their aryl-substituted congeners.
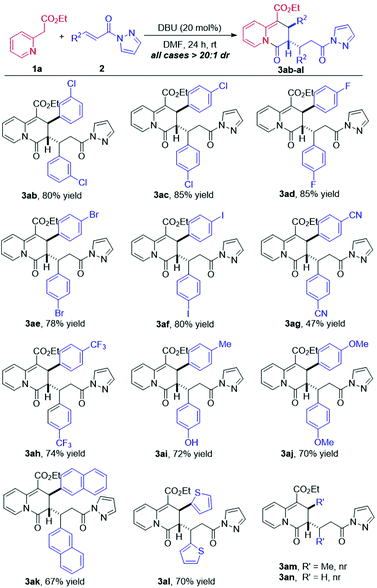 |
| Scheme 4 Substrate scope of α,β-unsaturated pyrazolamides. Reaction conditions: the reactions were carried out with 1a (0.4 mmol), 2 (1.4 mmol), and DBU (20 mol%) in 2.0 mL of DMF at room temperature for 24 h. The yield refers to the isolated yield after column chromatography. The dr was determined by 1H NMR analysis. | |
To evaluate the synthetic potential of the methodology, we conducted a gram-scale reaction of 1a and 2a under the standard conditions. As shown in Scheme 5, the reaction proceeded smoothly to give 3aa in 71% yield with a >20
:
1 dr. This suggests that the developed catalytic system has good scalability. In addition, the structure and relative configuration of product 3aa was further confirmed by single crystal X-ray crystallographic study.11 Then, different transformations of 3aa were carried out to showcase the synthetic utility of the products 2,3-dihydro-4H-quinolizin-4-ones.12 Treating 3aa with DDQ in CH2Cl2 gave the product 4H-quinolizin-4-one 4 in 54% yield. The corresponding ester derivative 5 could be easily obtained in 90% yield with a >20
:
1 dr through the cleavage of the pyrazole moiety and then an esterification process. Similarly, on treating 3aa with BnNH2 in THF at 70 °C, the corresponding amide product 6 was obtained in 60% yield without any loss in the diastereoselectivity. The reaction of 3aa with BnNH2 in the presence of DBU led to the formation of product 7 in 77% yield with a 53
:
47 dr. Finally, 3aa was conveniently conversed into the thioesterification product 8 in 61% yield with a >20
:
1 dr.
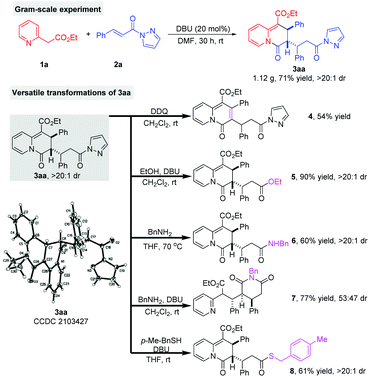 |
| Scheme 5 Gram-scale experiment and versatile transformations of 3aa. | |
We further conducted some control experiments to verify the importance of the N-acylpyrazole moiety of the α,β-unsaturated pyrazolamides for the above DBU-catalyzed dearomative [3 + 3] annulation (Scheme 6). As mentioned earlier, the reaction of 1a and 2a smoothly provided 3aa in 79% yield with a >20
:
1 dr. Under the standard conditions, the reaction of 1a and α,β-unsaturated pyrazolamide 11 gave the cycloadduct 12 only in a trace amount, but 12 was obtained in 34% yield with a >20
:
1 dr on raising the reaction temperature to 40 °C for 48 h. These two experiments revealed that the pyrazole moiety in α,β-unsaturated pyrazolamides is more conducive to the dearomative [3 + 3] annulation process than the 3,5-dimethyl pyrazole moiety. In addition, it was also found that both α,β-unsaturated ester 9 and α,β-unsaturated amide 10 failed to react with 1a under the standard conditions. These control experiments revealed that the N-acylpyrazole moiety in α,β-unsaturated pyrazolamides is crucial for the dearomative annulation reaction.
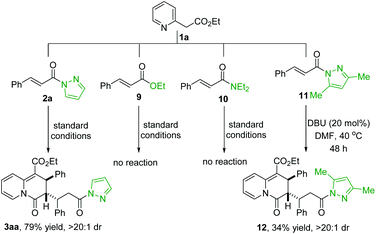 |
| Scheme 6 Control experiments. | |
In conclusion, we have developed a dearomative [3 + 3] annulation of 2-pyridylacetates and α,β-unsaturated pyrazolamides with DBU as the catalyst. A wide range of unexplored multisubstituted 2,3-dihydro-4H-quinolizin-4-ones were obtained in satisfactory yields (up to 94%) with excellent diastereoselectivities (all cases >20
:
1 dr). The developed protocol takes full advantage of the 1,3-dinucleophilic character of 2-pyridylacetates and 1,3-dielectrophilic character of α,β-unsaturated pyrazolamides to realize the dearomative annulation process. The N-acylpyrazole moiety of the α,β-unsaturated pyrazolamides was demonstrated to be crucial for the reaction. This practical method also features transition metal free, mild reaction conditions, wide functional group tolerance, easy scale-up synthesis, and versatile further derivatization.
Conflicts of interest
There are no conflicts to declare.
Acknowledgements
We are grateful to the National Natural Science Foundation of China (No. 22171029, 21901024, 21871252, 21801024, and 21801026), the Sichuan Science and Technology Program (2021YFS0315), and the Talent Program of Chengdu University (2081919035 and 2081921038).
Notes and references
-
(a) S. Hanessian, G. McNaughton-Smith, H.-G. Lombart and W. D. Lubell, Design and Synthesis of Conformationally Constrained Amino Acids as Versatile Scaffolds and Peptide Mimetics, Tetrahedron, 1997, 53, 12789–12854 CrossRef CAS;
(b) R. E. Dolle, Comprehensive Survey of Combinatorial Library Synthesis: 2000, J. Comb. Chem., 2001, 3, 477–517 CrossRef CAS;
(c) A. Facchetti, π-Conjugated Polymers for Organic Electronics and Photovoltaic Cell Applications, Chem. Mater., 2011, 23, 733–758 CrossRef CAS;
(d) E. Vitaku, D. T. Smith and J. T. Njardarson, Analysis of the Structural Diversity, Substitution Patterns, and Frequency of Nitrogen Heterocycles among U.S. FDA Approved Pharmaceuticals, J. Med. Chem., 2014, 57, 10257–10274 CrossRef CAS PubMed;
(e) U. H. F. Bunz, The Larger Linear N-Heteroacenes, Acc. Chem. Res., 2015, 48, 1676–1686 CrossRef CAS.
-
(a) R. D. Taylor, M. MacCoss and A. D. G. Lawson, Rings in Drugs, J. Med. Chem., 2014, 57, 5845–5859 CrossRef CAS;
(b) T. Y. Zhang, The Evolving Landscape of Heterocycles in Drugs and Drug Candidates, Adv. Heterocycl. Chem., 2017, 121, 1–12 CrossRef CAS.
-
(a) Y.-S. Xu, C.-C. Zeng, Z.-G. Jiao, L.-M. Hu and R.-G. Zhong, Design, Synthesis and Anti-HIV Integrase Evaluation of 4-Oxo-4H-quinolizine-3-carboxylic Acid Derivatives, Molecules, 2009, 14, 868–883 CrossRef CAS;
(b) S. D. Kuduk, R. K. Chang, C. N. Di Marco, W. J. Ray, L. Ma, M. Wittmann, M. A. Seager, K. A. Koeplinger, C. D. Thompson, G. D. Hartman and M. T. Bilodeau, Quinolizidinone Carboxylic Acids as CNS Penetrant, Selective M1 Allosteric Muscarinic Receptor Modulators, ACS Med. Chem. Lett., 2010, 1, 263–267 CrossRef CAS PubMed;
(c) T. Fujii, Y. Shindo, K. Hotta, D. Citterio, S. Nishiyama, K. Suzuki and K. Oka, Design and Synthesis of a FlAsH-Type Mg2+ Fluorescent Probe for Specific Protein Labeling, J. Am. Chem. Soc., 2014, 136, 2374–2381 CrossRef CAS;
(d) Y. Lu, S. Alujas-Burgos, C. Oliveras-González, L. Vázquez-Jiménez, P. Rojo, Á. Álvarez-Larena, P. Bayón and M. Figueredo, Enantioselective approach to indolizidine and quinolizidine scaffolds. Application to the synthesis of peptide mimics, Tetrahedron, 2018, 74, 104–116 CrossRef CAS.
- For a leading review, see:
(a) M. R. Kulkarni and N. D. Gaikwad, Recent advances towards the synthesis of 4H-quinolizin-4-one, Tetrahedron, 2020, 76, 131409 CrossRef CAS. For selected examples, see:
(b) R. den Heeten, L. J. P. van der Boon, D. L. J. Broere, E. Janssen, F. J. J. de Kanter, E. Ruijter and R. V. A. Orru, Concise Synthesis of Highly Substituted Benzo[a]quinolizines by a Multicomponent Reaction/Allylation/Heck Reaction Sequence, Eur. J. Org. Chem., 2012, 275–280 CrossRef CAS;
(c) H. Yu, G. Zhang and H. Huang, Palladium-Catalyzed Dearomative Cyclocarbonylation by C-N Bond Activation, Angew. Chem., Int. Ed., 2015, 54, 10912–10916 CrossRef CAS;
(d) M. J. James, N. D. Grant, P. O'Brien, R. J. K. Taylor and W. P. Unsworth, Catalytic Dearomatization Approach to Quinolizidine Alkaloids: Five Step Total Synthesis of (±)-Lasubine II, Org. Lett., 2016, 18, 6256–6259 CrossRef CAS;
(e) P. S. Shinde, A. C. Shaikh and N. T. Patil, Efficient access to alkynylated quinalizinones via the gold(I)-catalyzed aminoalkynylation of alkynes, Chem. Commun., 2016, 52, 8152–8155 RSC;
(f) J. Li, Y. Yang, Z. Wang, B. Feng and J. You, Rhodium(III)-Catalyzed Annulation of Pyridinones with Alkynes via Double C–H Activation: A Route to Functionalized Quinolizinones, Org. Lett., 2017, 19, 3083–3086 CrossRef CAS PubMed;
(g) R. Yang, J.-T. Yu, S. Sun and J. Cheng, Palladium-catalyzed CO-free cyclizative carbonylation
of 2-benzylpyridines leading to pyridoisoquinolinones, Org. Chem. Front., 2018, 5, 962–966 RSC;
(h) C.-C. Dong, J.-F. Xiang, L.-J. Xu and H.-Y. Gong, From CO2 to 4H-Quinolizin-4-ones: A One-Pot Multicomponent Approach via Ag2O/Cs2CO3 Orthogonal Tandem Catalysis, J. Org. Chem., 2018, 83, 9561–9567 CrossRef CAS;
(i) X.-L. Min, C. Sun and Y. He, Synthesis of 1-Amino-2H-quinolizin-2-one Scaffolds by Tandem Silver Catalysis, Org. Lett., 2019, 21, 724–728 CrossRef CAS PubMed;
(j) Z. Chen, T. Liu, X. Ma, P. Liang, L. Long and M. Ye, A One-Pot Sonogashira Coupling and Annulation Reaction: An Efficient Route toward 4H-Quinolizin-4-ones, Synlett, 2019, 863–867 CAS;
(k) X. Zhou, A. Chen, W. Du, Y. Wang, Y. Peng and H. Huang, Palladium-Catalyzed Hydrocarbonylative Cyclization Enabled by Formal Insertion of Aromatic C=N Bonds into Pd–Acyl Bonds, Org. Lett., 2019, 21, 9114–9118 CrossRef CAS PubMed.
- For selected reviews, see:
(a) W.-T. Wu, L. Zhang and S.-L. You, Recent Progress on Gold-catalyzed Dearomatization Reactions, Acta Chim. Sin., 2017, 75, 419–438 CrossRef CAS;
(b) G. Huang and B. Yin, Recent Developments in Transition Metal-Catalyzed Dearomative Cyclizations of Indoles as Dipolarophiles for the Construction of Indolines, Adv. Synth. Catal., 2019, 361, 405–425 CrossRef CAS;
(c) C. Zheng and S.-L. You, Catalytic asymmetric dearomatization (CADA) reaction-enabled total synthesis of indole-based natural products, Nat. Prod. Rep., 2019, 36, 1589–1605 RSC;
(d) Z.-L. Xia, Q.-F. Xu-Xu, C. Zheng and S.-L. You, Chiral phosphoric acid-catalyzed asymmetric dearomatization reactions, Chem. Soc. Rev., 2020, 49, 286–300 RSC;
(e) F.-T. Sheng, J.-Y. Wang, W. Tan, Y.-C. Zhang and F. Shi, Progresses in organocatalytic asymmetric dearomatization reactions of indole derivatives, Org. Chem. Front., 2020, 7, 3967–3998 RSC;
(f) Z. Zhang, H. Han, L. Wang, Z. Bu, Y. Xie and Q. Wang, Construction of bridged polycycles through dearomatization strategies, Org. Biomol. Chem., 2021, 19, 3960–3982 RSC.
- For selected examples, see:
(a) M. Hagimori, S. Matsui, N. Mizuyama, K. Yokota, J. Nagaoka and Y. Tominaga, Novel Synthesis of 4H-Quinolizine Derivatives Using Sulfonyl Ketene Dithioacetals, Eur. J. Org. Chem., 2009, 5847–5853 CrossRef CAS;
(b) C. W. Muir, A. R. Kennedy, J. M. Redmond and A. J. B. Watson, Synthesis of functionalised 4H-quinolizin-4-ones via tandem Horner–Wadsworth–Emmons olefination/cyclisation, Org. Biomol. Chem., 2013, 11, 3337–3340 RSC;
(c) Z.-P. Yang, Q.-F. Wu and S.-L. You, Direct Asymmetric Dearomatization of Pyridines and Pyrazines by Iridium-Catalyzed Allylic Amination Reactions, Angew. Chem., Int. Ed., 2014, 53, 6986–6989 CrossRef CAS PubMed;
(d) Z.-P. Yang, Q.-F. Wu, W. Shao and S.-L. You, Iridium-Catalyzed Intramolecular Asymmetric Allylic Dearomatization Reaction of Pyridines, Pyrazines, Quinolines, and Isoquinolines, J. Am. Chem. Soc., 2015, 137, 15899–15906 CrossRef CAS PubMed;
(e) J. Ma, F. Strieth-Kalthoff, T. Dalton, M. Freitag, J. L. Schwarz, K. Bergander, C. Daniliuc and F. Glorius, Direct Dearomatization of Pyridines via an Energy-Transfer-Catalyzed Intramolecular [4+2] Cycloaddition, Chem, 2019, 5, 2854–2864 CrossRef CAS;
(f) H.-J. Zhang, Z.-P. Yang, Q. Gu and S.-L. You, Tandem Pd-Catalyzed Intermolecular Allylic Alkylation/Allylic Dearomatization Reaction of Benzoylmethyl pyridines, Pyrazines, and Quinolines, Org. Lett., 2019, 21, 3314–3318 CrossRef CAS PubMed;
(g) L. Chen, R. Huang, K. Li, X.-H. Yun, C.-L. Yang and S.-J. Yan, An environmentally benign cascade reaction of chromone-3-carboxaldehydes with ethyl 2-(pyridine-2-yl)acetate
derivatives for highly site-selective synthesis of quinolizines and quinolizinium salts in water, Green Chem., 2020, 22, 6943–6953 RSC;
(h) L. Chang, N. Fischer-Durand, G. Gontard, B. Bertrand, S. Thorimbert and L. Dechoux, A Solvent-free, Catalyst-free Formal [3+3] Cycloaddition Dearomatization Strategy: Towards New Fluorophores for Biomolecules Labelling, ChemSusChem, 2021, 14, 1821–1824 CrossRef CAS PubMed.
- For selected examples, see:
(a) L. Xiang, Y. Yang, X. Zhou, X. Liu, X. Li, X. Kang, R. Yan and G. Huang, I2-Mediated Oxidative Cyclization for Synthesis of Substituted Indolizines, J. Org. Chem., 2014, 79, 10641–10647 CrossRef CAS;
(b) R.-R. Liu, J.-J. Hong, C.-J. Lu, M. Xu, J.-R. Gao and Y.-X. Jia, Indolizine Synthesis via Oxidative Cross-Coupling/Cyclization of Alkenes and 2-(Pyridin-2-yl)acetate Derivatives, Org. Lett., 2015, 17, 3050–3053 CrossRef CAS;
(c) D. C. Mohan, C. Ravi, V. Pappula and S. Adimurthy, Synthesis of Indolizines through Oxidative Linkage of C–C and C–N Bonds from 2-Pyridylacetates, J. Org. Chem., 2015, 80, 6846–6855 CrossRef PubMed;
(d) H. Li, X. Li, Y. Yu, J. Li, Y. Liu, H. Li and W. Wang, Synthesis of Indolizines via Reaction of 2-Substitued Azaarenes with Enals by an Amine-NHC Relay Catalysis, Org. Lett., 2017, 19, 2010–2013 CrossRef CAS PubMed;
(e) Y. Liu, Y. Yu, Y. Fu, Y. Liu, L. Shi, H. Li and W. Wang, Transition-metal-free synthesis of indolizines via, [3+2] annulation from α-bromoenals and 2-substitued azaarenes, Org. Chem. Front., 2017, 4, 2119–2123 RSC;
(f) T. Wu, M. Chen and Y. Yang, Synthesis of Indolizines via Palladium Catalyzed Annulation of Propargyl Carbonates and 2-(Pyridin-2-yl)acetonitrile Derivatives, J. Org. Chem., 2017, 82, 11304–11309 CrossRef CAS;
(g) D. Yang, Y. Yu, Y. Wu, H. Feng, X. Li and H. Cao, One-Pot Regiospecific Synthesis of Indolizines: A Solvent-Free, Metal-Free, Three-Component Reaction of 2-(Pyridin-2-yl)acetates, Ynals, and Alcohols or Thiols, Org. Lett., 2018, 20, 2477–2480 CrossRef CAS;
(h) Z. Chen, P. Liang, X. Ma, H. Luo, G. Xu, T. Liu, X. Wen, J. Zheng and H. Ye, Catalyst-Free Annulation of 2-Pyridylacetates and Ynals with Molecular Oxygen: An Access to 3-Acylated Indolizines, J. Org. Chem., 2019, 84, 1630–1639 CrossRef CAS PubMed;
(i) Z. Chen, P. Liang, F. Xu, Z. Deng, L. Long, G. Luo and M. Ye, Metal-Free Aminothiation of Alkynes: Three-Component Tandem Annulation toward Indolizine Thiones from 2-Alkylpyridines, Ynals, and Elemental Sulfur, J. Org. Chem., 2019, 84, 12639–12647 CrossRef CAS PubMed;
(j) L. He, Y. Yang, X. Liu, G. Liang, C. Li, D. Wang and W. Pan, Iodine-Mediated Oxidative Cyclization of 2-(Pyridin-2-yl)acetate Derivatives with Alkynes: Condition-Controlled Selective Synthesis of Multisubstituted Indolizines, Synthesis, 2020, 52, 459–470 CrossRef;
(k) Y. Fang, F. Li, Y. Yang, X. Liu and W. Pan, Iodine Mediated Base-Controlled Regio-Selective Annulation of 2-(Pyridin-2-yl)acetate Derivatives with Acrylic Esters for the Synthesis of Indolizines, Adv. Synth. Catal., 2020, 362, 1333–1344 CrossRef CAS.
-
(a) G. R. Newkome, J. M. Robinson and N. S. Bhacca, Chemistry of heterocyclic compounds. 8. One-step synthesis of 2-hydroxy-4H-quinolizin-4-ones, J. Org. Chem., 1973, 38, 2234–2236 CrossRef CAS;
(b) P. Cebasek, D. Bevk, S. Pirc, B. Stanovnik and J. Svete, Parallel Synthesis of 3-Amino-4H-Quinolizin-4-ones, Fused 3-Amino-4H-Pyrimidin-4-ones, and Fused 3-Amino-2H-Pyran-2-ones, J. Comb. Chem., 2006, 8, 95–102 CrossRef CAS;
(c) H. He, C. Qi, Y. Ou, W. Xiong, X. Hu, Y. Ren and H. Jiang, Base-promoted annulation of α-hydroxy ketones and dimethyl but-2-ynedioate: straightforward access to pyrano[4,3-a]quinolizine-1,4,6(2H)-triones and 2H-pyran-2,5(6H)-diones, Org. Biomol. Chem., 2014, 12, 8128–8131 RSC;
(d) J.-W. Fang, F.-J. Liao, Y. Qian, C.-C. Dong, L.-J. Xu and H.-Y. Gong, One-Pot Synthesis of 3-Substituted 4H-Quinolizin-4-ones via Alkyne Substrate Control Strategy, J. Org. Chem., 2021, 86, 3648–3655 CrossRef CAS PubMed.
- For selected examples, see:
(a) K. Itoh, M. Hasegawa, J. Tanaka and S. Kanemasa, Enantioselective Enol Lactone Synthesis under Double Catalytic Conditions, Org. Lett., 2005, 7, 979–981 CrossRef CAS PubMed;
(b) Y. Zhang, Y. Liao, X. Liu, Q. Yao, Y. Zhou, L. Lin and X. Feng, Catalytic Michael/Ring-Closure Reaction of α,β-Unsaturated Pyrazoleamides with Amidomalonates: Asymmetric Synthesis of (-)-Paroxetine, Chem. – Eur. J., 2016, 22, 15119–15124 CrossRef CAS;
(c) S. Meninno, C. Volpe and A. Lattanzi, Catalytic Enantioselective Synthesis of Protecting-Group-Free 1,5-Benzothiazepines, Chem. – Eur. J., 2017, 23, 4547–4550 CrossRef CAS PubMed;
(d) G. Wang, Y. Tang, Y. Zhang, X. Liu, L. Lin and X. Feng, Enantioselective Synthesis of N-H-Free 1,5-Benzothiazepines, Chem. – Eur. J., 2017, 23, 554–557 CrossRef CAS PubMed;
(e) S. Meninno, I. Quaratesi, C. Volpe, A. Mazzanti and A. Lattanz, Catalytic enantioselective one-pot approach to cis- and trans-2,3-diaryl substituted 1,5-benzothiazepines, Org. Biomol. Chem., 2018, 16, 6923–6934 RSC;
(f) J. Xian, L. Chen, L. Ye, Y. Sun, Z. Shi, Z. Zhao and X. Li, Enantioselective synthesis of fused dihydropyranones via squaramide-catalyzed Michael addition/lactonization cascade reaction, Tetrahedron, 2019, 75, 2350–2356 CrossRef CAS;
(g) C.-W. Lei, C.-B. Zhang, Z.-H. Wang, K.-X. Xie, J.-Q. Zhao, M.-Q. Zhou, X.-M. Zhang, X.-Y. Xu and W.-C. Yuan, Coumarin-3-formylpyrazoles as 3-carbon synthons in cyclocondensation for the synthesis of spiro-fused pentacyclic spirooxindoles, Org. Biomol. Chem., 2020, 18, 845–850 RSC.
-
(a) J.-Q. Zhao, L. Yang, X.-J. Zhou, Y. You, Z.-H. Wang, M.-Q. Zhou, X.-M. Zhang, X.-Y. Xu and W.-C. Yuan, Organocatalyzed Dearomative Cycloaddition of 2-Nitrobenzofurans and Isatin-Derived Morita–Baylis–Hillman Carbonates: Highly Stereoselective Construction of Cyclopenta[b]benzofuran Scaffolds, Org. Lett., 2019, 21, 660–664 CrossRef CAS PubMed;
(b) X.-M. Chen, C.-W. Lei, D.-F. Yue, J.-Q. Zhao, Z.-H. Wang, X.-M. Zhang, X.-Y. Xu and W.-C. Yuan, Organocatalytic Asymmetric Dearomatization of 3-Nitroindoles and 3-Nitrobenzothiophenes via Thiol-Triggered Diastereo- and Enantioselective Double Michael Addition Reaction, Org. Lett., 2019, 21, 5452–5456 CrossRef CAS PubMed;
(c) Z.-Z. Ge, L. Yang, Y. You, Z.-H. Wang, K.-X. Xie, M.-Q. Zhou, J.-Q. Zhao and W.-C. Yuan, Asymmetric dearomatization of 2-nitrobenzofurans by organocatalyzed one-step Michael addition to access 3,3′-disubstituted oxindoles, Chem. Commun., 2020, 56, 2586–2589 RSC;
(d) W.-C. Yuan, X.-J. Zhou, J.-Q. Zhao, Y.-Z. Chen, Y. You and Z.-H. Wang, Catalytic Enantioselective Dearomatization/Rearomatization of 2-Nitroindoles to Access 3-Indolyl-3′-Aryl-/Alkyloxindoles: Application in the Formal Synthesis of Cyclotryptamine Alkaloids, Org. Lett., 2020, 22, 7088–7093 CrossRef CAS PubMed;
(e) P.-H. Dou, Y. Chen, Y. You, Z.-H. Wang, J.-Q. Zhao, M.-Q. Zhou and W.-C. Yuan, Organocatalyzed Asymmetric
Dearomative [3+2] Annulation of Electron-Deficient 2-Nitrobenzo Heteroarenes with 3-Isothiocyanato Oxindoles, Adv. Synth. Catal., 2021, 363, 4047–4053 CrossRef CAS.
- CCDC 2103427 (3aa) contains the supplementary crystallographic data for this paper. †.
- For details, see the ESI.†.
Footnote |
† Electronic supplementary information (ESI) available: Experimental procedures, spectral data of new compounds, and crystallographic data. CCDC 2103427. For ESI and crystallographic data in CIF or other electronic format see DOI: 10.1039/d1qo01414e |
|
This journal is © the Partner Organisations 2022 |
Click here to see how this site uses Cookies. View our privacy policy here.