DOI:
10.1039/D1RA08388K
(Paper)
RSC Adv., 2022,
12, 2145-2149
Synthesis of unprotected glyco-alkynones via molybdenum-catalyzed carbonylative Sonogashira cross-coupling reaction†
Received
15th November 2021
, Accepted 10th December 2021
First published on 13th January 2022
Abstract
Herein we report a novel Mo-catalyzed carbonylative Sonogashira cross-coupling between 2-iodoglycals and terminal alkynes. The reaction displays major improvements compared to a related Pd-catalyzed procedure previously published by our group, such as utilizing unprotected sugar derivatives as starting materials and tolerance to substrates bearing chelating groups. In this work we also demonstrate the utility of the glyco-alkynone products as platform for further functionalization by synthesizing glyco-flavones via Au-catalyzed 6-endo-dig cyclization.
Metal-catalyzed carbonylative reactions have a history of many decades of development that began in the second half of the last century1 The ubiquity of carbonyl-containing compounds and the efficiency of these reactions turned it into a popular transformation in many fields of chemistry, from natural products synthesis and drug development to industrial multi-ton production of commodity chemicals.2 This three-component reaction delivers carbonyl-containing compounds by connecting carbon electrophiles to carbon and heteroatom nucleophiles through a CO bridge in an atom-economical fashion. Some commonly employed nucleophiles are amines,3 alcohols,4 boronic acids,5 terminal alkynes6 and alkyl halides.7
While noble metals have been in the forefront of the development of carbonylative coupling reactions, significant advancements have been achieved more recently in the use of some Earth-abundant metals (Mn, Fe, Co, Ni and Cu).2h The reports in the field are much more scarce, however, when it comes to group VI metals, with transformations being restricted to amine and alcohol nucleophiles, described in reports first published by Yamane and Roberts (Scheme 1).8 These reactions take place at high temperatures and are believed to follow a classic carbonylation mechanism: oxidative addition, CO insertion with the formation of acyl-metal intermediates, nucleophile coordination and reductive elimination. Since then, carbonylative reactions mediated by group VI metals have only been applied to the synthesis of heterocyclic compounds in a handful of publications.9
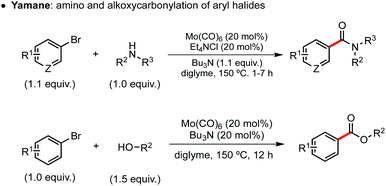 |
| Scheme 1 Mo-mediated amino- and alkoxycabonylation of aryl halides. | |
Inspired by this chemistry and continuing a research program in our laboratory devoted to the synthesis of biologically relevant C2-substituted glycals,10 we questioned whether Mo-catalyzed carbonylative coupling reactions could deliver carbonyl-containing glycals under Pd-free conditions from 2-iodoglycal electrophiles. Moreover, by eliminating palladium from the reaction medium, we wondered if unprotected 2-iodoglycals could be used as electrophiles,11 which would represent a major improvement from previous reports (Scheme 2A).10,12
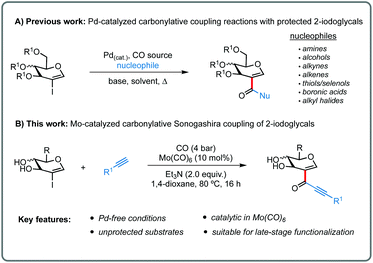 |
| Scheme 2 Metal-catalyzed carbonylative coupling reactions of 2-iodoglycals. | |
To test this idea, we chose the carbonylative Sonogashira coupling as our target reaction, as this is an unprecedented transformation under molybdenum catalysis, therefore synthetically relevant in its own right. Furthermore, the glyco-alkynone products are attractive molecules that can operate as platform for further functionalization in synthetic programs aiming complex sugar-containing targets.
We initiated our studies by carrying out an optimization campaign based on the reaction of 2-iodo-D-glucal (1a) and 4-ethynyltoluene (2a) (Table 1). We identified Mo(CO)6 as the best choice of catalyst, even though the reaction still takes place with other sources of molybdenum, such as Na2MoO4 (entry 2), presumably by the in situ formation of the metal carbonyl complex. As expected, the reaction still proceeds in reasonable yield with PdCl2 (entry 3), but not with other metals such as NiCl2 or FeCl2. The use of DIPEA as base resulted in diminished yield, while inorganic bases completely shut down the reaction (entries 5 and 6). A solvent screening showed that 3a can be obtained in a series of solvents, albeit in lower yields (26% with MeCN, 40% with THF and 32% with DMF) (entry 7). Finally, a control experiment demonstrated that CO(g) is needed in this reaction, presumably to regenerate the catalytic species Mo(CO)6 (entry 8).
Table 1 Optimization of reaction conditionsa
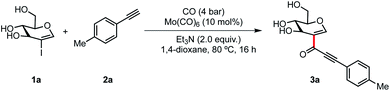
|
|
Variation from standard conditions |
Yieldb |
Reaction conditions: 1a (0.5 mmol), 2a (1.5 mmol), catalyst (10 mol%), base (2.0 equiv.), CO (4 bar), solvent (5 mL), 80 °C, 16 h. Isolated yield. |
1 |
None |
78% |
2 |
Na2MoO4 instead of Mo(CO)6 |
58% |
3 |
PdCl2 instead of Mo(CO)6 |
64% |
4 |
NiCl2 or FeCl2 instead of Mo(CO)6 |
0% |
5 |
DIPEA instead of Et3N |
27% |
6 |
Cs2CO3, K2CO3 instead of Et3N |
0% |
7 |
MeCN, THF of DMF instead of 1,4-dioxane |
26–40% |
8 |
Co CO(g) |
0% |
Having established good reaction conditions, we next explored the generality of this method (Table 2).
Table 2 Mo-catalyzed carbonylative Sonogashira cross-coupling of 2-iodoglycals with terminal alkynesa
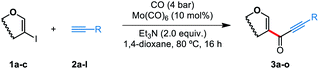
|
Reaction conditions: 2-iodoglycal (0.5 mmol), terminal alkyne (1.5 mmol), Mo(CO)6 (10 mol%), Et3N (2.0 equiv.), CO (4 bar), 1,4-dioxane (5 mL), 80 °C, 16 h. |
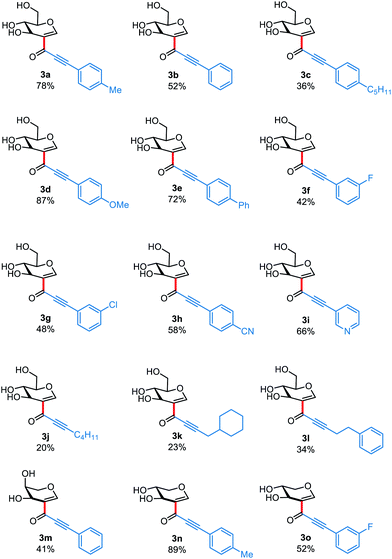 |
Using 2-iodo-D-glucal as electrophile, terminal alkynes bearing electron-rich aromatic rings delivered the desired products in moderate to good yields (3a–3e), while electron-poor groups gave alkynones 3f–i in slightly diminished yields. It is noteworthy that coordinating groups such as those present in 2h and 2i were tolerated by our methodology. This result combined with the fact that this reaction proceeds well with unprotected glycals indicates that the catalyst is less prone to be deactivated by coordination, a major improvement from the Pd-catalyzed coupling previously reported by our group.10a Terminal alkynes bearing alkyl groups were also amenable to this transformation, however, products 3j–3l were obtained only in low yields. Finally, we wished to test how different sugar derivatives perform under the standard reaction conditions, thus 2-iodo-L-arabinal and 2-iodo-D-xylal were reacted with terminal alkynes 2m and 2n, delivering 3m–o in yields comparable to those obtained with 2-iodo-D-glucal.
The structure of compound 3a was unambiguously confirmed by single-crystal X-ray analysis. The ORTEP view is shown in Fig. 1 (details in the ESI†).
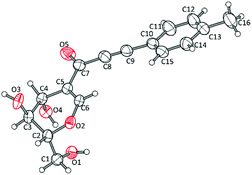 |
| Fig. 1 Thermal ellipsoid representation of compound 3a. | |
In order to demonstrate the synthetic utility of the glyco-alkynone products, we next tested their reactivity toward Au-catalyzed 6-endo-dig cyclization.13 Our goal was to display the internal alkyne as a valuable platform for further modification and, at the same time, obtain biologically relevant flavones.14
Overall, this Au-catalyzed cyclization occurred smoothly with a range aromatic glyco-alkynones, which gave glyco-flavones 4a–f in moderate to good yields. Electron-deficient and aliphatic alkynones (3h, 3i and 3k), on the other hand, failed to deliver the expected product, possibly due to a lower affinity towards gold coordination (Scheme 3).
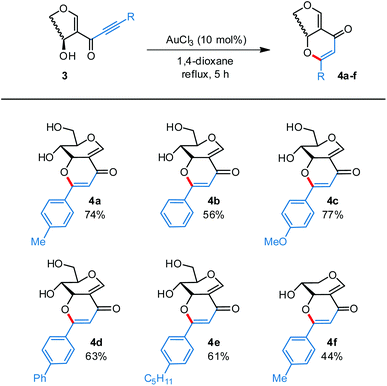 |
| Scheme 3 Au-catalyzed 6-endo-dig cyclization of glyco-alkynones. Reaction conditions: glyco-alkynone 3 (0.2 mmol), AuCl3 (10 mol%), 1,4-dioxane (2 mL), 80 °C, 3 h. | |
To shed light on the reaction mechanism of this transformation, a control experiment with the addition of the radical-trapping reagent TEMPO (2,2,6,6-tetramethyl-1-piperidinyloxy) was carried out. The reaction proceeded in good yield with no suppression being observed, which rules out the intermediacy of radical species (Scheme 4).15
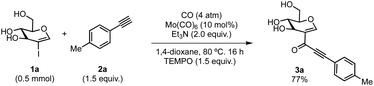 |
| Scheme 4 Radical-trapping experiment with TEMPO. | |
Based on this result and on literature precedents, we propose the reaction mechanism depicted in Fig. 2. Oxidative addition of the 2-iodoglycal to molybdenum generates complex (ii). Insertion of carbon monoxide leads to acylmolybdenum (iii), as previously proposed by Yamane and others.8,16 Coordination of a terminal alkyne to this complex gives (iv) which, after reductive elimination, delivers the alkynone product (v) and regenerates the active Mo(0) species.
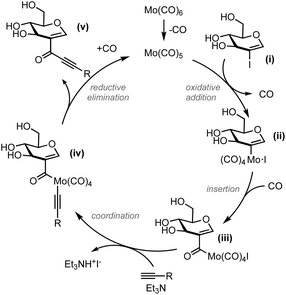 |
| Fig. 2 Proposed catalytic cycle for the Mo-catalyzed carbonylative Sonogashira reaction. | |
Conclusions
In summary, we developed a Pd-free carbonylative coupling reaction between 2-iodoglycals and terminal alkynes that rely on inexpensive Mo(CO)6 as catalyst and tolerates unprotected sugar derivatives as substrate. The products obtained are also prone to further functionalization as was demonstrated by the synthesis of six glyco-flavones via gold catalysis. An in-depth investigation of the reaction mechanism and new applications of this newly developed method are currently in progress in our laboratory.
Author contributions
MPD and AASP carried out the synthetic work. RAB collected X-ray crystallography data. MPD, HAE, HAS designed and wrote the manuscript. All authors approved the final version of the manuscript before submission.
Conflicts of interest
The authors declare no competing financial interest.
Notes and references
-
(a) V. W. Reppe, Justus Liebigs Ann. Chem., 1953, 582, 1 CrossRef;
(b) A. Schoenberg, I. Bartoletti and R. F. Heck, J. Org. Chem., 1974, 39, 3318 CrossRef CAS;
(c) A. Schoenberg and R. F. Heck, J. Org. Chem., 1974, 39, 3327 CrossRef CAS;
(d) A. Schoenberg and R. F. Heck, J. Am. Chem. Soc., 1974, 96, 7761 CrossRef CAS;
(e) N. Miyaura and A. Suzuki, Chem. Lett., 1981, 879 CrossRef CAS;
(f) S. Cacchi, E. Morera and G. Ortar, Tetrahedron Lett., 1985, 26, 1109 CrossRef CAS;
(g) S. Cacchi, P. G. Ciattini, E. Morera and G. Ortar, Tetrahedron Lett., 1986, 33, 3931 CrossRef.
-
(a) A. Brennführer, H. Neumann and M. Beller, ChemCatChem, 2009, 1, 28 CrossRef;
(b) X.-F. Wu, H. Neumann and M. Beller, Chem. Rev., 2013, 113, 1 CrossRef CAS PubMed;
(c) P. Guatam and B. C. Bhanage, Catal. Sci. Technol., 2015, 5, 4663 RSC;
(d) P. H. Gehrtz, V. Hirschbeck, B. Ciszek and I. Fleischer, Synthesis, 2016, 48, 1573 CrossRef CAS;
(e) Y. Bai, D. C. Davis and M. Dai, J. Org. Chem., 2017, 82, 2319 CrossRef CAS PubMed;
(f) Y. Li, Y. Hu and X.-F. Wu, Chem. Soc. Rev., 2018, 47, 172 RSC;
(g) K. Ma, B. S. Martin, X. Yin and M. Dai, Nat. Prod. Rep., 2019, 36, 174 RSC;
(h) J.-B. Peng, F.-P. Wu and X.-F. Wu, Chem. Soc. Rev., 2019, 119, 2090 CrossRef CAS PubMed;
(i) Z. Chen, L.-C. Wang and X.-F. Wu, Chem. Commun., 2020, 56, 6016 RSC;
(j) Z. Yin, J.-X. Xu and X.-F. Wu, ACS Catal., 2020, 10, 6510 CrossRef CAS;
(k) S. Zhang, H. Neumann and M. Beller, Chem. Soc. Rev., 2020, 49, 3187 RSC;
(l) D. Das and B. M. Bhanage, Adv. Synth. Catal., 2020, 362, 3022 CrossRef CAS.
-
(a) S. Fuse, R. Takahashi and T. Takahashi, Eur. J. Org. Chem., 2015, 3430 CrossRef CAS;
(b) R. Shi, H. Zhang, L. Lu, P. Gan, Y. Sha, H. Zhang, Q. Liu, M. Beller and A. Lei, Chem. Commun., 2015, 51, 3247 RSC;
(c) Y. Li, F. Zhu, Z. Wang and X.-F. Wu, ACS Catal., 2016, 6, 5561 CrossRef CAS;
(d) R. S. Mane and B. M. Bhanage, Adv. Synth. Catal., 2017, 359, 2621 CrossRef CAS;
(e) Q. Xing, H. Lv, C. Xia and F. Li, Chem. Commun., 2017, 53, 6914 RSC.
-
(a) M. Beller, W. Mägerlein, A. F. Indolese and C. Fischer, Synthesis, 2001, 1098 CAS;
(b) R. H. Munday, J. R. Martinelli and S. L. Buchwald, J. Am. Chem. Soc., 2008, 130, 2754 CrossRef CAS PubMed;
(c) B. T. Sargent and E. J. Alexanian, J. Am. Chem. Soc., 2016, 138, 7520 CrossRef CAS PubMed;
(d) Y. Wang and V. Gevorgyan, Angew. Chem., Int. Ed., 2017, 56, 3191 CrossRef CAS PubMed;
(e) H. Minami, K. Nogi and H. Yorimitsu, Org. Lett., 2019, 21, 2518 CrossRef CAS PubMed.
-
(a) P. Gautam and B. M. Bhanage, J. Org. Chem., 2015, 80, 7810–7815 CrossRef CAS PubMed;
(b) X. Qi, L.-B. Jiang, H.-P. Li and X.-F. Wu, Chem.–Eur. J., 2015, 21, 17650 CrossRef CAS PubMed;
(c) P. Gautam, M. Dhiman, V. Polshettiwar and B. M. Bhanage, Green Chem., 2016, 18, 5890 RSC;
(d) Y. Wang, Y. Wu, Y. Li and Y. Tang, Chem. Sci., 2017, 8, 3852 RSC;
(e) P. Gautam, R. Gupta and B. M. Bhanage, Eur. J. Org. Chem., 2017, 3431 CrossRef CAS;
(f) D. Bhattacherjee, M. Rahman, S. Ghosh, A. Kumar, A. K. Bagdi, G. V. Zyryanov, O. N. Chupakhin, P. Das and A. Hajra, Adv. Synth. Catal., 2021, 363, 1597 CrossRef CAS.
-
(a) W. Li and X.-F. Wu, Org. Biomol. Chem., 2015, 13, 509 Search PubMed;
(b) L. Akerbladh, P. Nordeman, M. Wejdemar, L. R. Odell and M. Larhed, J. Org. Chem., 2015, 80, 1464 CrossRef PubMed;
(c) C. Tan, P. Wang, H. Liu, X.-L. Zhao and Y. Liu, Chem. Commun., 2015, 51, 10871 RSC;
(d) G. Sun, M. Lei and L. Hu, RSC Adv., 2016, 6, 28442 RSC;
(e) K. Xiang, P. Tong, B. Yan, L. Long, C. Zhao, Y. Zhang and Y. Li, Org. Lett., 2019, 21, 412 CrossRef CAS PubMed;
(f) W. Mansour, R. Suleiman, M. Fettouhi and B. El Ali, ACS Omega, 2020, 5, 23687 CrossRef CAS PubMed.
-
(a) D. R. Pye, L.-J. Cheng and N. P. Mankad, Chem. Sci., 2017, 8, 4750 RSC;
(b) S. Sumino, T. Ui and I. Ryu, Org. Lett., 2013, 15, 3142 CrossRef CAS PubMed;
(c) J.-B. Peng, B. Chen, X. Qi, J. Ying and X.-F. Wu, Adv. Synth. Catal., 2018, 360, 4153 CrossRef CAS;
(d) Y. Weng, C. Zhang, Z. Tang, M. Shrestha, W. Huang, J. Qu and Y. Chen, Nat. Commun., 2020, 11, 392 CrossRef CAS PubMed;
(e) L.-J. Cheng and N. P. Mankand, Acc. Chem. Res., 2021, 54, 2261 CrossRef CAS PubMed.
-
(a) W. Ren and M. Yamane, J. Org. Chem., 2010, 75, 3017 CrossRef CAS PubMed;
(b) W. Ren and M. Yamane, J. Org. Chem., 2010, 75, 8410 CrossRef CAS PubMed;
(c) B. Roberts, D. Liptrot, L. Alcaraz, T. Luker and M. J. Stocks, Org. Lett., 2010, 12, 4280 CrossRef CAS PubMed;
(d) W. Ren, A. Emi and M. Yamane, Synthesis, 2011, 14, 2303 Search PubMed.
-
(a) B. Roberts, D. Liptrot, T. Luker, M. J. Stocks, C. Barber, N. Webb, R. Dods and B. Martin, Tetrahedron Lett., 2011, 52, 3793 CrossRef CAS;
(b) M. Nakka, R. Tadikonda, S. Nakka and S. Vidavalur, Adv. Synth. Catal., 2016, 358, 520 CrossRef CAS;
(c) J. S. Rao, A. V. Rao, T. Krishna, V. N. Murthy, J. Rajesh and A. Raghunadh, RSC Adv., 2016, 6, 67534 RSC.
-
(a) M. P. Darbem, C. H. A. Esteves, I. M. Oliveira, J. S. Reis, D. C. Pimenta and H. A. Stefani, RSC Adv., 2019, 9, 9468 RSC;
(b) H. A. Esteves, M. P. Darbem, D. C. Pimenta and H. A. Stefani, Eur. J. Org. Chem., 2019, 7384 CrossRef CAS;
(c) M. P. Darbem, K. S. Kanno, I. M. Oliveira, C. H. A. Esteves, D. C. Pimenta and H. A. Stefani, New J. Chem., 2019, 43, 696 RSC;
(d) M. P. Darbem, H. A. Esteves, I. M. Oliveira, D. C. Pimenta and H. A. Stefani, ChemCatChem, 2020, 12, 576 CrossRef CAS;
(e) A. A. Soares-Paulino and H. A. Stefani, Eur. J. Org. Chem., 2020, 3847 CrossRef CAS;
(f) M. P. Darbem, H. A. Esteves, I. M. Oliveira and H. A. Stefani, Eur. J. Org. Chem., 2020, 5220 CrossRef CAS.
- M. Malinowski, T. V. Tran, M. de Robichon, N. Lubin-Germain and A. Ferry, Adv. Synth. Catal., 2020, 362, 1184 CrossRef CAS.
-
(a) A. Bordessa, A. Ferry and N. Lubin-Germain, J. Org. Chem., 2016, 81, 12459 CrossRef CAS PubMed;
(b) M. Robichon, A. Bordessa, N. Lubin-Germain and A. Ferry, J. Org. Chem., 2019, 84, 3328 CrossRef PubMed;
(c) N. Hussain, M. Bhardwaj, A. Ahmed and D. Mukherjee, Org. Lett., 2019, 21, 3034–3037 CrossRef CAS PubMed;
(d) A. Ahmed, N. Hussain, M. Bhardwaj, A. K. Chhalodia, A. Kumar and D. Mukherjee, RSC Adv., 2019, 9, 22227 RSC.
- B. Godoi, R. F. Schumacher and G. Zeni, Chem. Rev., 2011, 111, 2937 CrossRef CAS PubMed.
-
(a) P. C. Ferriola, V. Cody and E. Middleton, Biochem. Pharmacol., 1989, 38, 1617 CrossRef CAS PubMed;
(b) A. K. Verma and R. Pratap, Tetrahedron, 2012, 68, 8523 CrossRef CAS;
(c) A. G. Golub, V. G. Bdzhola, O. V. Ostrynska, I. V. Kyshenia, V. M. Sapelkin, A. O. Prykhod'ko, O. P. Kukharenko and S. M. Yarmoluk, Med. Chem., 2013, 21, 6681 CAS;
(d) T. Yatabe, X. Jin, K. Yamaguchi and N. Mizuno, Angew. Chem., Int. Ed., 2015, 54, 13302 CrossRef CAS PubMed;
(e) C. Jiang, Z. Xiong, S. Jin, P. Gao, Y. Tang, Y. Wang, C. Du, X. Wang, Y. Liu, B. Lin, Y. Liu and M. A. Cheng, Chem. Commun., 2016, 52, 11516 RSC;
(f) X. Zhu, I. L. K. Wong, K.-F. Chan, J. Cui, M. C. Law, T. C. Chong, X. Hu, L. M. C. Chow and T. H. Chan, J. Med. Chem., 2019, 62, 8578 CrossRef CAS PubMed;
(g) A. M. de Matos, A. Martins, T. Man, D. Evans, M. Walter, M. C. Oliveira, O. López, J. G. F. Bolaños, P. Dätwyler, B. Ernst, M. P. Macedo, M. A. Contino, N. A. Colabufo and A. P. Raute, Pharmaceuticals, 2019, 12, 98 CrossRef CAS PubMed;
(h) N. Zhang, J. Yang, K. Li, J. Luo, S. Yang, J.-R. Song, C. Chen and W.-D. Pan, Molecules, 2019, 24, 2723 CrossRef CAS PubMed;
(i) A. H. E. Hassan, K.-T. Lee and Y. S. Lee, Eur. J. Med. Chem., 2020, 187, 111965 CrossRef CAS PubMed;
(j) S. Tian, T. Luo, Y. Zhu and J.-P. Wan, Chin. Chem. Lett., 2020, 31, 3073 CrossRef CAS.
-
(a) M. M. Caitlin, S. R. Matthew and E. Alexanian, Org. Lett., 2016, 18, 4148 CrossRef PubMed;
(b) R. P. Dominic, C. Li-Jie and N. P. Mankad, Chem. Sci., 2017, 8, 4750 RSC;
(c) K. L. Parvathi, V. M. Sarma, C. Sridhar and B. K. Raghu, ChemistrySelect, 2019, 4, 11553 CrossRef.
-
(a) K. Sangu, T. Watanabe, J. Takaya and N. Iwasawa, Synlet, 2007, 6, 929 Search PubMed;
(b) J. Takaya, K. Sangu and N. Iwasawa, Angew. Chem., Int. Ed., 2009, 48, 7090 CrossRef CAS PubMed;
(c) N. Iranpoor, H. Firouzabadi, S. Motevalli and M. Talebi, Tetrahedron, 2013, 69, 418 CrossRef CAS.
Footnote |
† Electronic supplementary information (ESI) available. CCDC 2085190. For ESI and crystallographic data in CIF or other electronic format see DOI: 10.1039/d1ra08388k |
|
This journal is © The Royal Society of Chemistry 2022 |
Click here to see how this site uses Cookies. View our privacy policy here.